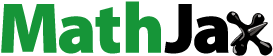
Abstract
The present study reports the synthesis of gold nanoparticles (AuNPs) using ‘sea grapes’ (Macroalgae) of Caulerpa racemosa extract in a simple, fast and environmentally friendly biogenic method. The formation of spherical, stable, polycrystalline nanoparticles with an average size of 18–45 nm was demonstrated by UV–vis spectroscopy, SEM, high-resolution transmission electron microscopy and X-ray diffraction measurements. Furthermore, FTIR analysis confirmed the presence of phytochemical constituents in the form of functional groups involved in AuNPs synthesis. Antimicrobial activities confirmed that Gram-negative bacteria, here represented by Escherichia coli, were found to be more sensitive to synthesized AuNPs than Gram-positive bacteria, represented by Staphylococcus aureus. The synthesized nanoparticles also showed cytotoxicity against human lung cancer cells (H460) with an IC50 value of 25 µg/mL. Furthermore, our findings show that AuNPs derived from C. racemosa extract are able to induce apoptosis activation via extrinsic and intrinsic pathways and mitochondrial pathways. These findings lay the groundwork for future research against emerging multi-drug resistant bacterial pathogens, as well as lung cancer treatment.
1. Introduction
Nanotechnology has been applied in diverse scientific fields, including physics, chemistry, pharmaceutical science, material science, medicine and agriculture (Narayanan & Sakthivel, Citation2011). Largely, nanoparticles have been widely used in systematical applications in medicine, cosmetics, drug-delivery systems, DNA labelling, sensors, catalysis and other fields (Ghorbani, Citation2014; Gopinath et al., Citation2019; Mohan et al., Citation2020). To date, several methods have been explored for the synthesis of nanoparticles. However, most of them require toxic reagents, harmful reducing agents, expensive techniques and severe reaction parameters (Usman, Aziz, & Noqta, Citation2019). Furthermore, a trace amount of unreacted substance could exert influence on the synthesis process and pollute the environment. As a result, developing an alternative synthetic route using greener methods to reduce environmental pollution is critical. Green synthesis of nanoparticles using microorganisms such as bacteria (Pantidos & Horsfall, Citation2014; Saravanan, Barik, MubarakAli, Prakash, & Pugazhendhi, Citation2018), fungi (Akther, Mathipi, Kumar, Davoodbasha, & Srinivasan, Citation2019; Suganya et al., Citation2015), algae (Mubarakali, Gopinath, Rameshbabu, & Thajuddin, Citation2012; Palaniappan, Sathishkumar, & Sankar, Citation2015), seaweed (Boomi et al., Citation2020) and plant extracts (Makarov et al., Citation2014; MubarakAli, Thajuddin, Jeganathan, & Gunasekaran, Citation2011) has been widely reported. However, the use of microorganisms necessitates proper handling and maintenance, which incurs costs. On the other hand, the use of plant and seaweed extracts actively mediates the ion reducing process in the formation of metallic nanoparticles, lowering maintenance costs, and acting as a stabilizing agent (Manikandakrishnan et al., Citation2019). Moreover, plant and seaweed borne natural compounds such as proteins, phenols, carbohydrates, flavonoids, alkaloids and amino acids are essential phytoconstituents used as precursors in the silver and gold ion reduction reactions within green synthesis processes (Goddard, Marín, Russell, & Searcey, Citation2020; Palaniappan et al., Citation2015), and also for the production of desirably sized nanoparticles (Makarov et al., Citation2014). These nanoparticles are being used for the evaluation of a wide range of pharmacological activities such as anti-cancer (Palaniappan et al., Citation2015), anti-bacterial (Subramanian et al., Citation2019), larvicidal (Mondal et al., Citation2014), anti-plasmodial (Ponarulselvam et al., Citation2012), anti-dermatophytic (Nisha et al., Citation2014) and other degenerative diseases.
Seaweed is a good reducing agent and has a high metal uptake capacity. It produces secondary metabolites, particularly bis-indole alkaloids and terpenoids, the latter primarily with an enol acetate functional group. Some of the compounds have antimicrobial, insecticidal, antifouling, ichthyotoxic, feeding deterrent, anti-inflammatory, cytotoxic and plant growth regulatory properties (P. Yang et al., Citation2015). Due to the rise of multiple antibiotic resistance strains, such as methicillin-resistant Staphylococcus aureus (MRSA) and Extended Spectrum Beta-Lactamases (ESBLs), treating for bacterial illness has recently become one of the most significant challenges and sources of great concern for both aquatic and human populations. Hence, treating bacterial pathogens with metal nanoparticles is a promising alternative to antibiotics in the clinical sector. A recent study reported that silver nanoparticles synthesized using Caulerpa racemose displayed strong antibacterial activity against different human pathogens (Kathiraven, Sundaramanickam, Shanmugam, & Balasubramanian, Citation2015). Similarly, other studies also reported that gold and zinc nanoparticles synthesized from Turbinaria conoides (Rajeshkumar et al., Citation2013), Caulerpa peltata, and Sargassum myriocystum (Nagarajan & Arumugam Kuppusamy, Citation2013) exhibited potential antibacterial activities against Gram-positive and Gram-negative bacteria, respectively. However, there have been very few reports on C. racemose-mediated AuNPs synthesis and their antibacterial applications.
Cancer is one of the major clinical diseases characterized by a progressive growth in the proportion of cell divisions (Kanchana & Balakrishna, Citation2011) that cause significant morbidity and mortality, as well as the world’s leading health issue (Devi, Bhimba, & Ratnam, Citation2012). The medical application of nanotechnology will be an alternative research tool for diagnosing and treating cancer disease, as well as repairing damaged tissues and cells, while lowering costs and minimizing side effects (Manikandakrishnan et al., Citation2019). Also, anticancer therapy using nanoparticles is becoming increasingly popular (Unno et al., Citation2005). Since nanoparticles are millions of times smaller than a human cell, they can interact with DNA, proteins, enzymes, and cell receptors both extracellularly and intracellularly (Behzadi et al., Citation2017). According to recent literature, gold nanoconjugates have been tested for antioxidant and cytotoxic activity in cervical cancer cell lines (HeLa cells), with findings suggesting that gold nanoparticles (AuNPs) could improve scavenging activity and promote apoptosis by increasing mitochondrial transmembrane permeation (Mahalakshmi & Kumar, Citation2019). Similarly, silver nanoparticles obtained from the seaweed (Cymodocea serrulata) have shown potential cytotoxicity against human lung cancer A549 cells lines (Palaniappan et al., Citation2015). However, cytotoxicity determinations of these gold nanoparticles from C. racemose are still limited. Therefore, the goal of this research was to develop a green synthesis method of gold nanoparticles using an aqueous extract of C. racemose seaweed. Furthermore, the cytotoxicity of synthesized gold nanoparticles against a human lung cancer cell line was investigated to develop new nano-drug formulations for cancer treatment.
2. Materials and methods
2.1. Sources of chemicals, bacterial cultures and cell lines
Chloroauric acid (HAuCl4), Dulbecco’s modified Eagle’s medium (DMEM), fetal bovine serum (FBS), penicillin, streptomycin, 3-(4,5-dimethyl-2, 5-diphenyl-2H-terazolium bromide (MTT), dimethyl sulfoxide (DMSO), Luria Bertani (LB) broth and Muller Hinton Agar were purchased from Himedia (Mumbai, India). All the chemicals and reagents used in this study were of the highest purity. The human lung cancer cell line (H460) was procured from the NCCS (National Center for Cell Science), Pune, India. The bacterial pathogens including Staphylococcus aureus and Escherichia coli used in this study were obtained from Bharathidasan University, Tamil Nadu, India.
2.2. Collection and preparation of Caulerpa racemosa extract
Caulerpa racemosa is a species of green algal seaweed in the Caulerpaceae family that is commonly referred to as ‘sea grapes’. It is found in many areas of shallow seawater in tropical and subtropical marine habitats, and there are about 60 species worldwide. There are many species and varieties of this genus in India, with the majority of them found in the Gulf of Mannar, the southeast coast of India, and the southeastern Chinese coastline, respectively.
Fresh C. racemosa samples were collected from the Gulf of Mannar and Rameswarem regions, southeast coast of Tamil Nadu, India. Collected seaweed was surface sterilized with distilled water to remove contaminants and shade dried for 14 days after which it was ground into a fine powder using a kitchen blender. The aqueous extract of seaweed was prepared following the method of Palaniappan et al. (Citation2015). Briefly, C. racemosa powder (10 g) was added to deionized water (100 mL). The resultant mixture suspension/solution (10%) was then heated at 60 °C for 20 min. The resultant suspension was filtered and stored at 4 °C for further studies.
2.3. Synthesis of gold nanoparticles
For the synthesis of gold nanoparticles, an aqueous solution of 1 mM chloroauric acid (HAuCl4) was prepared. Following this, 5 mL of C. racemosa extract was mixed with 45 mL of aqueous 1 mM chloroauric acid solution and left at room temperature for 10 min before boiling in a water bath at 60 °C for 20 min. The colour changes in the solution confirmed the synthesis of gold nanoparticles (AuNPs). Simultaneously, a control (without extract) was also maintained. Following the completion of the reaction, the solution of synthesized nanoparticles was centrifuged at 10,000 rpm for 30 min at 4 °C. After centrifugation, the colloidal AuNPs were washed in distilled water, collected and stored at 4 °C until further use.
2.4. Characterization studies of AuNPs
The UV–vis spectra of the green synthesized gold nanoparticles were recorded at periodic time intervals until the absorption maxima reached saturation. The absorption maxima were scanned using UV–Visible spectrophotometer (JASCO V-650) at a wavelength of 300–800 nm with a resolution of 1 nm using deionized water as a blank. The surface morphology and shape of the AuNPs were studied using scanning electron microscope (HITACHI-S3000H). Furthermore, the mean particle size of the synthesized AuNPs was measured using high-resolution transmission electron microscopy (HR-TEM; JEOL-JEM 2100). The surface plasmon resonance (SPR) peaks and crystalline nature of green synthesized AuNPs were observed using XRD (Xpert PRO XRD) measurements operating at a voltage of 40 kV and a current of 30 mA, respectively. The FTIR (Perkin Elmer spectrophotometer – RXI spectrum) spectra of green synthesized AuNPs were analyzed to identify potential functional groups for AuNPs formation. The spectrum was analyzed manually with all peaks identified based on the NIST-FTIR database.
2.5. Antibacterial activity
The antimicrobial activity of the AuNPs was determined using the disc diffusion method, as described by Kiehlbauch et al. (Citation2000). Briefly, the obtained cultures were grown overnight at 37 °C in 20 mL of Luria Bertani (LB) broth and then adjusted with sterile saline solution to achieve turbidity comparable to McFarland no. 5 standard (1.0 × 108) CFU/mL. Using a sterile cotton swab, each strain was swabbed uniformly onto the surface of the Mueller Hinton agar plate (Himedia Labs, Mumbai, India). Each disc was impregnated with a different concentration (50 μg, 100 μg and 150 μg) of aqueous solution of nanoparticles in solution and placed on Mueller Hinton agar plates along with standard antibiotic (30 μg). The plates were then incubated at 37 °C for 24 h and the zones of inhibition were measured.
2.6. Cell culture and MTT assay
The human lung cancer cell line, known as H460, was obtained from the National Centre for Cell Science (NCCS), which is located in Pune, India. The H460 cells that were obtained were cultured in DMEM, which was supplemented with 10% FBS and 1% penicillin/streptomycin. The cells were maintained in an incubator at 37 °C with a CO2 concentration of 5%. The MTT (dimethyl thiazolytetrazolium bromide) assay was performed according to the method described by N. Yang, WeiHong, and Hao (Citation2014). Briefly, the cells were seeded (1 × 104) in a 96-well plate and incubated for 24 h at 37 °C in a humidified atmosphere of 95% air and 5% CO2. Successively, the green synthesized AuNPs were treated with human lung H460 cancer cells at concentrations ranging from 5 to 35 μg/mL, while control cells were treated with DMSO. After 48 h, 20 µL of MTT solution was added to each well, and the cultures were further incubated for 4 h before being treated with 200 µL of DMSO. The absorbance was measured at 595 nm and the percentage of cell growth inhibition was calculated using the given formula,
2.7. Morphological changes
As previously described by Varunkumar et al. (Citation2020), the morphological effect of green synthesized AuNPs on H460 cells was confirmed using acridine orange (AO) and ethidium bromide (EB) staining. Briefly, a 6-cell plate with 5 × 105 cells/well was incubated overnight for attachment. After attachment, cells were given a lethal dose of synthesized nanoparticles in fresh medium. After 24 h, the cover slip was removed and stained with AO/EB (10 mL) for 30 min before being washed with 1× PBS. For ROS analysis, AuNP-treated cells were stained with 40 μM dichlorofluorescein-diacetate (DCFH-DA) for 30 min. Furthermore, the effect of AuNPs on the nuclei was observed by staining the treated cells with Hoechst 33258. Briefly, 1 × 105/mL-seeded cells were used in 6-well culture plates. After 60% confluency, rGO-Ag composites were added. After 24 h, 50 μL/mL Hoechst 33258 stain was added and incubated for 30 min at 37 °C in 5% CO2. The cells were imaged in a fluorescence microscope with ×20 and ×40, respectively.
2.8. Statistical analysis
For the MTT assay, Graph Pad Prism, version 6.04 (La Jolla, CA, USA) was used to perform one-way and two-way ANOVAs with Dunnett’s post hoc test. To be statistically significant, a value must be less than 0.05, 0.01, 0.001, or even 0.0001 to be marked as P1, P2, P3 or P4, respectively.
3. Results and discussion
Green synthesized nanoparticles are more acceptable because they do not use toxic chemicals. Due to their non-toxicity, seaweeds are considered as potential candidates for the synthesis of nanoparticles. Previous studies have indicated that green synthesized nanoparticles have excellent anti-microbial and anti-cancer efficacies, indicating their potential use as nanotherapeutics (Rahmat et al., Citation2021; Rathinavel et al. Citation2020). This study also tested the efficacy of gold nanoparticles synthesized using seaweed against bacterial pathogens, as well as lung cancer cells.
3.1. Green synthesis of gold nanoparticles and UV–visible spectroscopy
When the aqueous extracts of C. racemosa were added to flasks containing chloroauric acid solution and incubated for 20 min at two different temperatures (room temperature and 60 °C), the formation of AuNPs was confirmed in the flask incubated at 60 °C by the development of ruby red or pinkish colour, which is a distinctive characteristic of AuNPs (MubarakAli et al., Citation2011; Ramakrishna, Rajesh Babu, Gengan, Chandra, & Nageswara Rao, Citation2016). However, the aqueous solutions of algal extracts and chloroauric acid incubated at room temperature remained yellow and slightly yellow in colour, respectively.
Temperature is one of the important factors in the biosynthesis of nanoparticles (Hamed, Givianrad, & Moradi, Citation2015). The current study demonstrated the synthesis of bioactive AuNPs in a very short reaction time at a higher temperature (60 °C), correlating with our previous study in which silver nanoparticles were synthesized using a different seaweed, Cymodocea serrulata (Kamran, Bhatti, Iqbal, & Nazir, Citation2019; Palaniappan et al., Citation2015). In UV–visible spectroscopy, the synthesized AuNPs had an absorbance peak at 540 nm (), which was similar to other studies (Manjunath et al., Citation2017; MubarakAli et al., Citation2011). Furthermore, samples incubated at 60 °C had the highest absorbance spectra when compared to samples incubated at room temperature. Previously, the synthesis of gold nanoparticles using microorganisms required 24–48 h to complete the process. This is regarded as one of the disadvantages of using biological sources for nanoparticle synthesis (Priyadarshini, Pradhan, Sukla, & Panda, Citation2014). In contrast to previous research, we were able to synthesize gold nanoparticles within an hour by enhancing the incubation temperature, making this seaweed a new potential source for gold nanomaterials.
3.2. FTIR spectroscopy analysis
The FTIR results of both seaweed extract and synthesized AuNPs showed transmittance at different wavelengths (). The results support our hypotheses that various biomolecules, such as phenolic compounds, protein, tannin, flavonoid, alkaloids and terpenoids, are present in the C. racemosa extract and act as reducing and capping agents for the formation of AuNPs. A similar observation was reported when phenolic residues were depleted into phenoxide structures via oxidation for the purpose of gold ion reduction (Boomi et al., Citation2020). The dried C. racemosa FTIR spectra revealed absorption peaks at 3411, 2931, 2102, 1643, 1413, 1260, 1150, 1078, 1029, 706, 645 and 615 cm−1, corresponding to the N-H stretching vibration, SH structure (organo silicon compounds), C = C structure (alkanes) and C = S structure (stretching vibration sulphides) (). The AuNPs absorption peak frequencies were 3483, 2919, 2853, 1604, 1542, 1418, 1604, 1408, 1314, 1458, 1072, 714, 591, 441, 413 cm−1 (). The presence of an absorption band at 3483 cm−1 was due to peptide linkage N–H stretching vibrations and phenolic O–H stretching vibrations, indicating the presence of polyphenols and phenolic acids. Peaks at 1604 and 1408 cm−1 and 1072 cm−1, respectively, were indicative of carbonyl groups, C-O stretching of carboxylic and amines (Manikandakrishnan et al., Citation2019).
3.3. Electron microscopy and XRD analysis
SEM and HR-TEM analysis were used to investigate the size and shape of green synthesized AuNPs. and shows SEM and HR-TEM of AuNPs. They were mostly cubic and spherical in shape, with a few irregular patterns and this finding is consistent with previous research (Manikandakrishnan et al., Citation2019). Furthermore, the TEM image revealed that the synthesized gold nanoparticles ranged in diameter from 18.94 to 45.54 nm, with an average particle size of around 35 nm. In the previous study by Ramakrishna et al. (Citation2016), a similar phenomenon was observed.
Figure 4. High-resolution transmission electron microscopy images of gold nanoparticles: (a) 200 nm scale, (b) 100 nm scale, (c) 20 nm scale and (d) selected area diffraction pattern.
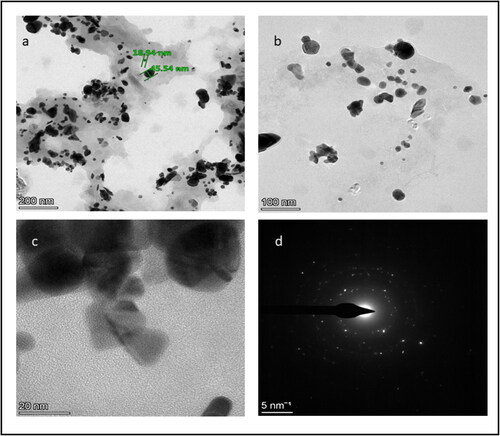
X-ray diffraction (XRD) was used to confirm the crystallinity and the characteristic peaks of AuNPs. The XRD patterns of the AuNPs prepared using the C. racemosa extracts and their simulated solution are shown in . The obtained diffraction peaks correspond to the (111), (200), (220) and (311) planes, which are indexed as the band for gold’s centred cubic structure. Thus, the XRD pattern clearly shows that the resultant nanoparticles were crystalline in nature. The highest relative intensity (%) revealed that chloroauric acid concentration was directly related to the formation of more AuNPs (Suganya et al., Citation2015; Yallappa, Manjanna, & Dhananjaya, Citation2015).
3.4. Antibacterial activity
The antibacterial activity of resultant nanoparticles was investigated in this study using a disc diffusion susceptibility assay against Gram-negative E. coli and Gram-positive S. aureus bacteria. The AuNPs exhibited a dose-dependent antibacterial activity against S. aureus and E. coli. At higher concentrations of AuNPs, treatment induced around 9 mm diameter zone of inhibition (). In addition, recent research demonstrated that the conjugation of the resultant nanoparticles (either gold or silver) with standard antibiotics would further enhance the antibacterial potential of up to three times (Ali, Perveen, Ali, et al., Citation2020; Ali, Perveen, Shah, et al., Citation2020).
Table 1. Inhibitory action of gold nanoparticles (AuNPs) synthesized using aqueous extracts of Caulerpa racemosa against human pathogenic bacteria.
Notably, E. coli had a higher susceptibility to AuNPs than S. aureus. This difference in susceptibility patterns between Gram negative and Gram positive bacteria can be attributed to differences in cell wall composition (MubarakAli et al., Citation2011). The antibacterial activity of the NPs depends upon various factors, including the absorption rate, release of metabolites, metabolic functions and distribution in the cell. The NPs attach to the microbial cell surface through active moieties and then diffused into the interior of the cell where they begin to interact with the cell’s different components (Rahmat et al., Citation2021). Furthermore, the electrostatic interaction and alternation of the bacterial cell membrane were the primary mechanisms by which the AuNPs induced antibacterial activity (Ramar et al., Citation2015).
3.5. Cytotoxic activity
According to the World Health Organization, lung cancer now ranks sixth among the leading causes of death globally (Bray et al., Citation2018). Due to their advanced pharmaceutical applications, algae-derived AuNPs have sparked considerable interest recently (Rathinavel et al., Citation2020). However, there has been very little research on the anticancer activity of AuNPs synthesized from C. racemosa extracts (Manikandakrishnan et al., Citation2019). To the best of our knowledge, this is the first attempt to investigate AuNPs’ anticancer activity against human lung cancer cells (H460). The results revealed that theH460 cells responded to the AuNPs cytotoxic assay in a dose-dependent manner from a concentration of 5 µg to 35 µg. This treatment reduced H460 cell viability. Similarly, silver nanoparticles from different seaweeds are also reported to exhibit cytotoxic activity against human breast cancer (MCF-7) (Devi et al., Citation2012), human colon cancer (HT29) (Ramar et al., Citation2015), human lung cancer (Palaniappan et al., Citation2015) and human cervical (HeLa) cancer cell lines (Mahalakshmi & Kumar, Citation2019).
The half-maximal inhibitory concentration (IC50) of AuNPs against H460 cells was determined to be 25 µg (). This cytotoxic activity was greater than that of AuNPs synthesized from the brown algae Cytoseira baccata and tested against colon cancer cells (González-Ballesteros, Prado-López, Rodríguez-González, Lastra, & Rodríguez-Argüelles, Citation2017), while lower than AuNPs synthesized from the green algae C. racemosa and used to treat human adenocarcinoma cells (Manikandakrishnan et al., Citation2019). This cytotoxic effect of AuNPs results from the active physicochemical interaction of gold atoms with functional groups of intercellular proteins and nitrogen bases and phosphate groups in DNA (Blagoi et al., Citation1991).
3.6. Morphological changes
Apoptosis is a normal process that leads to the death of cells and it is controlled by apoptotic proteins such as BAX, caspases and BCL-2, Bid (Ola, Nawaz, & Ahsan, Citation2011). Apoptotic morphological changes caused by synthesized AuNPs are depicted in . These changes were observed in H460 lung cells stained with AO and EB. After being stained with AO, H460 cells take on a uniformly green appearance, whereas the debris left behind by early apoptotic cells is yellow or yellowish green. After treatment with AuNPs, cells that had been stained with EB showed signs of apoptosis, including condensed nuclei, membrane blebbing and apoptotic bodies. When compared to untreated cells, these cytological changes suggest that cells treated with AuNPs are most likely to undergo apoptosis. Similar variations were seen in gold nanoparticles extracted from Marsdenia tenacissima, which had been green synthesized and tested against lung cancer cells A549 (Sun et al., Citation2019).
Figure 7. The effect of green synthesized AuNPs from C. racemosa on human lung cancer (H460) cells: (a) Morphology changes marked in arrow – AO/EB dual staining assay, (b) ROS generation – DCFH-DA staining, (c) nuclei condensation – Hoechst 33258 staining assay (scale: 100 μm).
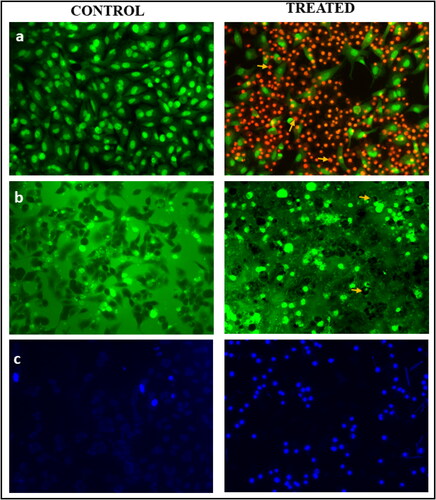
Furthermore, DCFH-DA staining was used to investigate the effect of AuNPs on ROS generation in H460 cells. In general, low levels of ROS are required for normal cancer cell proliferation, differentiation, cell cycle progression, invasion and migration. However, high ROS levels in cancer cells disrupt normal function and cause cell death (Redza-Dutordoir & Averill-Bates, Citation2016). In the current study, fluorescence absorbed by cells treated with synthesized AuNPs was found to be higher than that absorbed by cells untreated with AuNPs (), this indicates the synthesized AuNPs inhibit the expression of the anti-apoptotic gene in H460 cells, while increasing the expression of the pro-apoptotic gene (Varunkumar et al., Citation2020). This results in the activation of the p53-dependent intrinsic signalling apoptosis pathway. In addition, as shown in , 25 μg/mL AuNPs treatment resulted in clear nuclear damage as indicated by the presence of bright blue fluorescence intensity due to chromatin condensation, nuclear fragmentation and apoptotic bodies, whereas control H460 cells exhibited uniformly light blue nuclei. This further supports the notion that synthesized AuNPs are capable of inducing apoptosis through the regulation of the intrinsic apoptotic pathway.
4. Conclusion
In this study, we successfully synthesized gold nanoparticles (AuNPs) using a renewable bio-resource, the seaweed C. racemosa. The aqueous extracts of the seaweed reduce Au (III) ions to gold nanoparticles (AuNPs) at 60 °C within about 20 min. The synthesized nanomaterials have shown improved physico–chemical properties like size, shape and surface chemistry. The phenolic extracts of the seaweed actively reduced and stabilized the AuNPs. The AuNPs showed the highest antibacterial activities against Gram-negative bacteria (E. coli) than Gram-positive bacteria (S. aureus). Furthermore, cytotoxicity and apoptosis induction by the synthesized nanoparticles against human lung cancer cells (H460) was confirmed, indicating the potential of AuNPs as a natural medicine for lung cancer, potentially overcoming the negative effects of chemotherapy. Overall, the findings established a solid foundation for future research into the development of new biomedical drugs for cancer therapy.
Ethical approval
No related ethical issues are reported.
Acknowledgements
We acknowledge the UGC-MRP South Eastern Regional Office, Hyderabad for providing support for this work.
Disclosure statement
The authors declare that they have no conflict of interest.
Additional information
Funding
References
- Akther, T., Mathipi, V., Kumar, N. S., Davoodbasha, M., & Srinivasan, H. (2019). Fungal-mediated synthesis of pharmaceutically active silver nanoparticles and anticancer property against A549 cells through apoptosis. Environmental Science and Pollution Research International, 26(13), 13649–13657. doi:10.1007/s11356-019-04718-w.
- Ali, S., Perveen, S., Ali, M., Shah, M. R., Khan, E., Sharma, A. S., … Chen, Q. (2020). Nano-conjugates of Cefadroxil as efficient antibacterial agent against Staphylococcus aureus ATCC 11632. Journal of Cluster Science, 31(4), 811–821. doi:10.1007/s10876-019-01688-4
- Ali, S., Perveen, S., Shah, M. R., Zareef, M., Arslan, M., Basheer, S., … Ali, M. (2020). Bactericidal potentials of silver and gold nanoparticles stabilized with cefixime: A strategy against antibiotic-resistant bacteria. Journal of Nanoparticle Research, 22(7), 1–12. doi:10.1007/s11051-020-04939-y
- Behzadi, S., Serpooshan, V., Tao, W., Hamaly, M. A., Alkawareek, M. Y., Dreaden, E. C., … Mahmoudi, M. (2017). Cellular uptake of nanoparticles: journey inside the cell. Chemical Society Reviews, 46(14), 4218–4244. doi:10.1039/c6cs00636a.Cellular
- Blagoi, Y. P., Galkin, V. L., Gladchenko, G. O., Kornilova, S. V., Sorokin, V. A., & Shkorbatov, A. G. (1991). Metal complexes of nucleic acids in solutions. Kiev: Naukova Dumka. (in Russian).
- Boomi, P., Poorani, G. P., Selvam, S., Palanisamy, S., Jegatheeswaran, S., Anand, K., … Prabu, H. G. (2020). Green biosynthesis of gold nanoparticles using Croton sparsiflorus leaves extract and evaluation of UV protection, antibacterial and anticancer applications. Applied Organometallic Chemistry, 34(5), e5574. doi:10.1002/aoc.5574
- Bray, F., Ferlay, J., Soerjomataram, I., Siegel, R. L., Torre, L. A., & Jemal, A. (2018). Global cancer statistics 2018: GLOBOCAN estimates of incidence and mortality worldwide for 36 cancers in 185 countries. CA: A Cancer Journal for Clinicians, 68(6), 394–424. doi:10.3322/caac.21492
- Devi, J. S., Bhimba, B. V., & Ratnam, K. (2012). In vitro anticancer activity of silver nanoparticles synthesized using the extract of Gelidiella sp. International Journal of Pharmacy and Pharmaceutical Sciences, 4(4), 710–715.
- Ghorbani, H. R. (2014). A review of methods for synthesis of Al nanoparticles. Oriental Journal of Chemistry, 30(4), 1941–1949. doi:10.13005/ojc/300456
- Goddard, Z. R., Marín, M. J., Russell, D. A., & Searcey, M. (2020). Active targeting of gold nanoparticles as cancer therapeutics. Chemical Society Reviews, 49(23), 8774–8789.
- González-Ballesteros, N., Prado-López, S., Rodríguez-González, J. B., Lastra, M., & Rodríguez-Argüelles, M. C. (2017). Green synthesis of gold nanoparticles using brown algae Cystoseira baccata: Its activity in colon cancer cells. Colloids and Surfaces B, Biointerfaces, 153, 190–198. doi:10.1016/j.colsurfb.2017.02.020.
- Gopinath, V., Priyadarshini, S., MubarakAli, D., Loke, M. F., Thajuddin, N., Alharbi, N. S., … Vadivelu, J. (2019). Anti-Helicobacter pylori, cytotoxicity and catalytic activity of biosynthesized gold nanoparticles: Multifaceted application. Arabian Journal of Chemistry, 12(1), 33–40. doi:10.1016/j.arabjc.2016.02.005
- Hamed, M. R., Givianrad, M. H., & Moradi, A. M. (2015). Biosynthesis of silver nanoparticles using marine sponge Haliclona. Oriental Journal of Chemistry, 31(4), 1961–1967. doi:10.13005/ojc/310413
- Kamran, U., Bhatti, H. N., Iqbal, M., & Nazir, A. (2019). Green synthesis of metal nanoparticles and their applications in different fields: A review. Zeitschrift Für Physikalische Chemie, 233(9), 1325–1349. doi:10.1515/zpch-2018-1238
- Kanchana, A., & Balakrishna, M. (2011). Anti-cancer effect of saponins isolated from Solanum trilobatum leaf extract and induction of apoptosis in human larynx cancer cell lines. International Journal of Pharmacy and Pharmaceutical Sciences, 3(4), 356–364.
- Kathiraven, T., Sundaramanickam, A., Shanmugam, N., & Balasubramanian, T. (2015). Green synthesis of silver nanoparticles using marine algae Caulerpa racemosa and their antibacterial activity against some human pathogens. Applied Nanoscience, 5(4), 499–504. doi:10.1007/s13204-014-0341-2
- Kiehlbauch, J. A., Hannett, G. E., Salfinger, M., Archinal, W., Monserrat, C., & Carlyn, C. (2000). Use of the National Committee for Clinical Laboratory Standards guidelines for disk diffusion susceptibility testing in New York state laboratories. Journal of Clinical Microbiology, 38(9), 3341–3348.
- Mahalakshmi, M., & Kumar, P. (2019). Phloroglucinol-conjugated gold nanoparticles targeting mitochondrial membrane potential of human cervical (HeLa) cancer cell lines. Spectrochimica Acta Part A: Molecular and Biomolecular Spectroscopy, 219, 450–456. doi:10.1016/j.saa.2019.04.060
- Makarov, V. V., Love, A. J., Sinitsyna, O. V., Makarova, S. S., Yaminsky, I. V., Taliansky, M. E., & Kalinina, N. O. (2014). “Green” nanotechnologies: Synthesis of metal nanoparticles using plants. Acta Naturae, 6(1), 35–44. 20. doi:10.32607/20758251-2014-6-1-35-44
- Manikandakrishnan, M., Palanisamy, S., Vinosha, M., Kalanjiaraja, B., Mohandoss, S., Manikandan, R., … Prabhu, N. M. (2019). Facile green route synthesis of gold nanoparticles using Caulerpa racemosa for biomedical applications. Journal of Drug Delivery Science and Technology, 54, 101345. doi:10.1016/j.jddst.2019.101345
- Manjunath, M. H., Joshi, C. G., Danagoudar, A., Poyya, J., Kudva, A. K., & BL, D. (2017). Biogenic synthesis of gold nanoparticles by marine endophytic fungus-Cladosporium cladosporioides isolated from seaweed and evaluation of their antioxidant and antimicrobial properties. Process Biochemistry, 63, 137–144. doi:10.1016/j.procbio.2017.09.008
- Mohan, U. P., Sriram, B., Panneerselvam, T., Devaraj, S., MubarakAli, D., Parasuraman, P., … Kunjiappan, S. (2020). Utilization of plant-derived Myricetin molecule coupled with ultrasound for the synthesis of gold nanoparticles against breast cancer. Naunyn-Schmiedeberg’s Archives of Pharmacology, 393(10), 1963–1976. doi:10.1007/s00210-020-01874-6
- Mondal, N. K., Chowdhury, A., Dey, U., Mukhopadhya, P., Chatterjee, S., Das, K., & Datta, J. K. (2014). Green synthesis of silver nanoparticles and its application for mosquito control. Asian Pacific Journal of Tropical Disease, 4, S204–S210. doi:10.1016/S2222-1808(14)60440-0
- Mubarakali, D., Gopinath, V., Rameshbabu, N., & Thajuddin, N. (2012). Synthesis and characterization of CdS nanoparticles using C-phycoerythrin from the marine cyanobacteria. Materials Letters, 74, 8–11. doi:10.1016/j.matlet.2012.01.026
- MubarakAli, D., Thajuddin, N., Jeganathan, K., & Gunasekaran, M. (2011). Plant extract mediated synthesis of silver and gold nanoparticles and its antibacterial activity against clinically isolated pathogens. Colloids and Surfaces B, Biointerfaces, 85(2), 360–365. doi:10.1016/j.colsurfb.2011.03.009.
- Nagarajan, S., & Arumugam Kuppusamy, K. (2013). Extracellular synthesis of zinc oxide nanoparticle using seaweeds of Gulf of Mannar, India. Journal of Nanobiotechnology, 11(1), 39–11. doi:10.1186/1477-3155-11-39
- Narayanan, K. B., & Sakthivel, N. (2011). Green synthesis of biogenic metal nanoparticles by terrestrial and aquatic phototrophic and heterotrophic eukaryotes and biocompatible agents. Advances in Colloid and Interface Science, 169(2), 59–79.
- Nisha, S. N., Aysha, O. S., Rahaman, J. S. N., Kumar, P. V., Valli, S., Nirmala, P., & Reena, A. (2014). Lemon peels mediated synthesis of silver nanoparticles and its antidermatophytic activity. Spectrochimica Acta Part A: Molecular and Biomolecular Spectroscopy, 124, 194–198. doi:10.1016/j.saa.2013.12.019
- Ola, M. S., Nawaz, M., & Ahsan, H. (2011). Role of Bcl-2 family proteins and caspases in the regulation of apoptosis. Molecular and Cellular Biochemistry, 351(1–2), 41–58.
- Palaniappan, P., Sathishkumar, G., & Sankar, R. (2015). Fabrication of nano-silver particles using Cymodocea serrulata and its cytotoxicity effect against human lung cancer A549 cells line. Spectrochimica Acta Part A: Molecular and Biomolecular Spectroscopy, 138, 885–890. doi:10.1016/j.saa.2014.10.072
- Pantidos, N., & Horsfall, L. E. (2014). Biological synthesis of metallic nanoparticles by bacteria, fungi and plants. Journal of Nanomedicine & Nanotechnology, 05(05), 1. doi:10.4172/2157-7439.1000233
- Ponarulselvam, S., Panneerselvam, C., Murugan, K., Aarthi, N., Kalimuthu, K., & Thangamani, S. (2012). Synthesis of silver nanoparticles using leaves of Catharanthus roseus Linn. G. Don and their antiplasmodial activities. Asian Pacific Journal of Tropical Biomedicine, 2(7), 574–580. doi:10.1016/S2221-1691(12)60100-2
- Priyadarshini, E., Pradhan, N., Sukla, L. B., & Panda, P. K. (2014). Controlled synthesis of gold nanoparticles using Aspergillus terreus if0 and its antibacterial potential against gram negative pathogenic bacteria. Journal of Nanotechnology, 2014, 1–9. doi:10.1155/2014/653198
- Rahmat, M., Bhatti, H. N., Rehman, A., Chaudhry, H., Yameen, M., Iqbal, M., … Abbas, M. (2021). Bionanocomposite of Au decorated MnO2 via in situ green synthesis route and antimicrobial activity evaluation. Arabian Journal of Chemistry, 14(12), 103415. doi:10.1016/j.arabjc.2021.103415
- Rajeshkumar, S., Malarkodi, C., Gnanajobitha, G., Paulkumar, K., Vanaja, M., Kannan, C., & Annadurai, G. (2013). Seaweed-mediated synthesis of gold nanoparticles using Turbinaria conoides and its characterization. Journal of Nanostructure in Chemistry, 3(1), 1–7. doi:10.1016/j.ijcard.2006.04.033
- Ramakrishna, M., Rajesh Babu, D., Gengan, R. M., Chandra, S., & Nageswara Rao, G. (2016). Green synthesis of gold nanoparticles using marine algae and evaluation of their catalytic activity. Journal of Nanostructure in Chemistry, 6(1), 1–13. doi:10.1007/s40097-015-0173-y
- Ramar, M., Manikandan, B., Marimuthu, P. N., Raman, T., Mahalingam, A., Subramanian, P., … Munusamy, A. (2015). Synthesis of silver nanoparticles using Solanum trilobatum fruits extract and its antibacterial, cytotoxic activity against human breast cancer cell line MCF 7. Spectrochimica Acta Part A: Molecular and Biomolecular Spectroscopy, 140, 223–228. doi:10.1016/j.saa.2014.12.060
- Rathinavel, L., Jothinathan, D., Sivasankar, V., Mylsamy, P., Omine, K., &, Selvarajan, R. (2020). Algal nanoparticles: boon for antimicrobial therapeutic applications. In: R. Prasad, B. Siddhardha, & M. Dyavaiah (Eds.), Nanostructures for antimicrobial and antibiofilm applications (pp. 127–138). Cham: Springer. doi:10.1007/978-3-030-40337-9_15
- Redza-Dutordoir, M., & Averill-Bates, D. A. (2016). Activation of apoptosis signalling pathways by reactive oxygen species. Biochimica et Biophysica Acta (BBA) – Molecular Cell Research, 1863(12), 2977–2992. doi:10.1016/j.bbamcr.2016.09.012
- Saravanan, M., Barik, S. K., MubarakAli, D., Prakash, P., & Pugazhendhi, A. (2018). Synthesis of silver nanoparticles from Bacillus brevis (NCIM 2533) and their antibacterial activity against pathogenic bacteria. Microbial Pathogenesis, 116(January), 221–226. 10.1016/j.micpath.2018.01.038.
- Subramanian, P., Ravichandran, A., Manoharan, V., Muthukaruppan, R., Somasundaram, S., Pandi, B., … You, S. (2019). Synthesis of Oldenlandia umbellata stabilized silver nanoparticles and their antioxidant effect, antibacterial activity, and bio-compatibility using human lung fibroblast cell line WI-38. Process Biochemistry, 86, 196–204. doi:10.1016/j.procbio.2019.08.002
- Suganya, K. S. U., Govindaraju, K., Kumar, V. G., Dhas, T. S., Karthick, V., Singaravelu, G., & Elanchezhiyan, M. (2015). Blue green alga mediated synthesis of gold nanoparticles and its antibacterial efficacy against Gram positive organisms. Materials Science & Engineering C, Materials for Biological Applications, 47, 351–356.
- Sun, B., Hu, N., Han, L., Pi, Y., Gao, Y., & Chen, K. (2019). Anticancer activity of green synthesised gold nanoparticles from Marsdenia tenacissima inhibits A549 cell proliferation through the apoptotic pathway. Artificial Cells, Nanomedicine and Biotechnology, 47(1), 4012–4019. doi:10.1080/21691401.2019.1575844
- Unno, Y., Shino, Y., Kondo, F., Igarashi, N., Wang, G., Shimura, R., … Shirasawa, H. (2005). Oncolytic viral therapy for cervical and ovarian cancer cells by Sindbis virus AR339 strain. Clinical Cancer Research, 11(12), 4553–4560.
- Usman, A. I., Aziz, A. A., & Noqta, O. A. (2019). Application of green synthesis of gold nanoparticles: A review. Jurnal Teknologi, 81(1), 171–182. doi:10.11113/jt.v81.11409
- Varunkumar, K., Anusha, C., Saranya, T., Ramalingam, V., Raja, S., & Ravikumar, V. (2020). Avicennia marina engineered nanoparticles induce apoptosis in adenocarcinoma lung cancer cell line through p53 mediated signaling pathways. Process Biochemistry, 94(February), 349–358. doi:10.1016/j.procbio.2020.04.034
- Yallappa, S., Manjanna, J., & Dhananjaya, B. L. (2015). Phytosynthesis of stable Au, Ag and Au–Ag alloy nanoparticles using J. sambac leaves extract, and their enhanced antimicrobial activity in presence of organic antimicrobials. Spectrochimica Acta Part A: Molecular and Biomolecular Spectroscopy, 137, 236–243. doi:10.1016/j.saa.2014.08.030
- Yang, N., WeiHong, L., & Hao, L. (2014). Biosynthesis of Au nanoparticles using agricultural waste mango peel extract and its in vitro cytotoxic effect on two normal cells. Materials Letters, 134, 67–70. doi:10.1016/j.matlet.2014.07.025
- Yang, P., Liu, D.-Q., Liang, T.-J., Li, J., Zhang, H.-Y., Liu, A.-H., … Mao, S.-C. (2015). Bioactive constituents from the green alga Caulerpa racemosa. Bioorganic & Medicinal Chemistry, 23(1), 38–45. doi:10.1016/j.bmc.2014.11.031