Abstract
Infections with parasites, especially those involving nematodes that invade tissues, induce a strong Th2-type immune response, which increases immunoglobulin E and eosinophil levels in the blood and tissues. Eosinophils are not effective against all possible helminth infections, but are known to be effective against nematode larvae. In particular, when a host is re-infected by a species of nematode that it previously encountered, the activation of acquired immunity causes robust accumulation and activation of eosinophils that damages the nematode larvae. Eosinophil production and activation processes are mainly induced by interleukin (IL)-5, which is produced by Th2 cells and group 2 innate lymphoid cells, and eosinophils have been shown to generally participate in host defense, inflammation, and immunomodulation. Recently, several papers have reported host defense by non-antigen-specific immune activation, in which group 2 innate lymphoid cells and Th2 cells produce interleukin (IL)-5 and IL-13 in response to IL-33 stimulation. This immune activation is produced by migrating larvae of a species that differs from the species previously encountered. Eosinophils also play an important role in the eradication of migrating larvae. Thus, eosinophils contribute to host defense in both antigen-specific and non-antigen-specific manners.
1. Parasitic infections
Parasitic infections are not common in developed countries, but a World Health Organization survey revealed that intestinal parasites continue to infect billions of people in tropical and subtropical areas [Citation1]. Thus, intestinal parasitic infections are regarded as a medically important “Neglected tropical diseases”. Depending on the type and number of parasites, parasitic infection often causes severe anemia and malnutrition, and becomes chronic. Infections in children cause developmental disabilities, delays in cognitive development, and potential mortality. Moreover, parasitic infections have economic consequences due to treatment cost and loss of work time [Citation2].
Parasites can be classified into small protozoa, which constitute unicellular eukaryotes (e.g., malaria, toxoplasma, and amoeba) and large multicellular helminths (e.g., trematodes, cestodes, and nematodes). Parasites infect via various routes and in various sites, and the corresponding host immune responses vary depending on the properties of the particular parasites, as the amounts of circulating IgE and eosinophils increase due to helminthic infections. Nevertheless, parasitic infections often become chronic, as many worms acquire the ability to evade host immune responses. Here, we discuss the role of eosinophils in host defense against helminth infections.
2. Immune responses to nematodes
Immune responses to parasites exhibit some general trends. First, T cells are essential, as demonstrated by the inability of nude mice to successfully eliminate parasitic infections [Citation3–5]. Typically, a Th1-type immune response is induced by protozoal infection [Citation6], whereas a Th2-type immune response is induced by helminthic infection [Citation7]. For example, studies using Leishmania have shown the importance of the activation of phagocytes (e.g., macrophages) by interferon (IFN)-γ produced by Th1 cells, to control protozoal infections [Citation6]. In contrast, to restrict the growth and activity of large helminths that cannot be phagocytosed by macrophages, various effector factors are activated by cytokines produced by Th2 cells. In particular, the activation of effector antibodies and granulocytes (e.g., eosinophils, basophils, and mast cells), as well as mucus secretion and contraction of smooth muscle in mucosal tissues, such as gastrointestinal tract and trachea, promote the expulsion of helminths from the body [Citation8–10]. Mice with a predominately Th2-type immune response are resistant to infection by Trichuris muris, whereas mice with a predominately Th1-type immune response are susceptible to infection by T. muris [Citation11]. While it remains unclear why naïve T cells differentiate into Th2 cells in hosts infected with helminths, there are at least two possible reasons.
First, helminths have few Toll-like receptor ligands that induce dendritic cells to produce interleukin (IL)-12, which is important for Th1 cell differentiation. Second, parasites produce excretory/secretion molecules that suppress IL-12 production while enhancing the production of Th2 cell-inducing cytokines (thymic stromal lymphopoietin [TSLP], IL-25, and IL-33) from non-hematopoietic epithelial cells [Citation12–15].
The role of innate immunity against helminth infection is also a focus of active investigations. In particular, helminths damage epithelial and endothelial cells in various tissues when they infect or move in the host; these damaged cells release cytokines such as TSLP, IL-25, and IL-33, which activate group 2 innate lymphocyte cells (ILC2s) and stimulate the production of large amounts of Th2 cytokines [Citation16,Citation17]. ILC2s are regarded as important sources of Th2 cytokines because the production and activation of Th2 cells requires a considerable amount of time; it is not sufficiently rapid to control primary or early stages of infection [Citation18].
Th2 cells and ILC2s produce Th2 cytokines such as IL-4, IL-13, IL-9, and IL-5. IL-4 is mainly produced by Th2 cells and is important for Th2 cell differentiation, as well as for the activation of the class switching mechanism in B cells that enables IgE production. The receptor for IL-4 consists of IL-4Rα and the common γ-chain, expressed on hematopoietic cells; it uses Signal Transducer and Activator of Transcription 6 (STAT6) and insulin receptor substrate-1 for intracellular signal transduction [Citation19]. Notably, IL-13 signal transduction also involves IL-4Rα and STAT6; thus, its actions partially overlap with those of IL-4. However, the receptor for IL-13 uses IL-13Rα, largely expressed on non-hematopoietic cells, instead of the common γ-chain; therefore, the actions of IL-13 differ from those of IL-4 [Citation20,Citation21]. IL-4 efficiently stimulates hematopoietic cells, whereas IL-13 mainly contributes to the activation of non-hematopoietic cells [Citation20]. IL-4 and IL-13 induce (1) the differentiation of mucosal epithelial cells into goblet cells for mucus secretion [Citation22]; (2) the proliferation of epithelial cells, thereby promoting epithelial turnover [Citation23]; (3) the production of antimicrobial peptides from epithelial cells [Citation24] and Paneth cells [Citation25], as well as the production of IL-25 from tuft cells [Citation26–28]; (4) the contraction of smooth muscle in mucosal tissue, thereby enhancing peristaltic movement [Citation29]; (5) the production of chemokines such as C-C Motif Chemokine Ligand (CCL) 11, CCL17, CCL22, and CCL24 from various cells [Citation30,Citation31]; (6) the differentiation of M2 macrophages [Citation32]; and (7) the activation and class switching of B cells [Citation33]. The actions of IL-4 and IL-13 are important for the elimination of the rodent intestinal parasitic nematode, Nippostrongylus brasiliensis [Citation8]. In addition, IL-9 enhances the proliferation, survival, and activation of mast cells, T cells, and ILC2s [Citation34]; IL-5 is a growth and survival factor for eosinophils, which is essential for eosinophil-mediated host defense [Citation35].
3. Proliferation and activation of eosinophils
Eosinophils are multinucleated leukocytes with granules that are rich in basic proteins; the numbers of these cells increase in blood and sites of inflammation during allergic reactions and helminth infections. IL-5 secretion from Th2 cells and ILC2s is important for eosinophil proliferation and survival. Th2 cells and ILC2s also produce IL-13, which facilitates recruitment of eosinophils to the site of infection or the site of allergen entry by stimulating the production of chemokines (e.g., CCL11 and CCL24) from various cells [Citation30,Citation36]. IL-33 is produced by various cells, such as epithelial cells, endothelial cells, and fibroblasts; this cytokine strongly stimulates the production of Th2 cytokines from Th2 cells and ILC2s [Citation37–40]. IL-33 also has the capacity to directly activate recruited eosinophils at sites of infection and allergen entry [Citation41].
The function of eosinophils as a defense against parasites is well-known; however, eosinophils have been reported to have additional roles in allergic inflammation and tissue repair, as well as immunomodulation [Citation42–44]. Regarding the eosinophil-mediated mechanism of defense against parasites, binding to the worm larvae through antibody or complement, followed by release of intracellular granules, is presumed to cause injury to invading worms [Citation45–49]. The numbers of eosinophils increase with helminth infection. Löffler found a link between parasite infection and pulmonary eosinophilia (Löffler’s syndrome) [Citation50]. Although the mechanism underlying this eosinophil accumulation has been unknown for many years, we elucidated the mechanism using a Strongyloides venezuelensis infection model [Citation38]. Some parasitic enteric nematode larvae, including S. venezuelensis and N. brasiliensis, do not migrate directly to the gut upon percutaneous or oral infection. Instead, they migrate to the lungs via the bloodstream, then penetrate the alveolar cavity and travel to the throat, where they are swallowed. Finally, they reach the small intestine and mature [Citation51,Citation52]. As the larvae pass through the lungs, they cause damage to lung tissue, stimulating the release of IL-33 from type II alveolar epithelial cells. IL-33-deficient mice showed a marked reduction in eosinophil accumulation upon S. venezuelensis infection. Conversely, nasal administration of IL-33 can induce pulmonary eosinophilia even in Rag2-deficient mice that lack both T cells and B cells [Citation38]. IL-33 stimulates ILC2s to proliferate and accumulate in the lungs, and induces production of IL-5 and IL-13 by ILC2s. Excretory/secretory products from N. brasiliensis stimulate neuronal production of neuromedin U, which stimulates ILC2s to produce Th2 cytokines. These combined actions result in the onset of lung eosinophilic inflammation [Citation53].
4. Eosinophils in host defense
Because the functions of IL-5 and eosinophils appeared to be closely related, their in vivo functions in eosinophil-mediated host defense has been clarified in studies using IL-5-deficient mice [Citation54,Citation55] and neutralizing antibodies against IL-5 [Citation56]. Neutralization or deficiency of IL-5 strongly suppresses eosinophil accumulation during helminth infection; however, some eosinophils remain in mouse tissues. Therefore, eosinophil-deficient mice have been developed and used for experimental analyses [Citation42,Citation57]. Eosinophils were first shown to have anti-parasitic activity through in vitro experiments on schistosome larvae [Citation46]. When eosinophils and schistosome larvae were cultured in the presence of IgE, IgG or complement, the eosinophils exhibited damaging activity against the larvae [Citation46,Citation48,Citation58]. However, the role of IgE-mediated eosinophil activation in vivo remains unclear, as high-affinity FcεRIα is often not expressed on the surface of eosinophils in mice, which are the most common experimental animal model [Citation59]. In addition, even eosinophil-deficient mice infected with schistosomes exhibit phenotypes similar to wild-type mice. Following scenario account for the differences in vitro and in vivo: At the initial stage of infection, infecting Schistosoma mansoni larvae induce a Th1-type immune response in mice without increasing the number of eosinophils, subsequently the host immune response changes to Th2-type after the larvae mature to adults and begin to lay eggs [Citation60]. The adult worms, different from larvae, are resistant to attack from eosinophils even though the number of eosinophils increases by the action of Th2 cytokines.
In addition, the absence of eosinophils had no particular effect in the mouse intestinal helminth T. muris infection model [Citation61]. T. muris is transmitted by the oral intake of eggs; larvae hatch in the intestine and become adults without migration to other tissues [Citation62]. In contrast, Heligmosomoides polygyrus infection occurs through oral ingestion of the larvae; these larvae invade the intestinal lining, where they undergo some growth before returning to the lumen and becoming adults. When eosinophil-deficient mice were infected with H. polygyrus, the intestines of these mice showed more worms than those of wild-type mice. In a model of microfilariae infection induced by intravenous administration of microfilariae of Brugia malayi, the number of microfilariae decreases immediately after infection in wild-type mice, but remains high after infection in eosinophil-deficient mice. When eosinophil-deficient mice were infected with the tissue-migrating intestinal nematode N. brasiliensis, the number of adults found in the intestinal tract was similar to that of wild-type mice; however, eosinophil-deficient mice showed increased numbers of eggs in feces, compared with the numbers of eggs observed in wild-type mice. Thus, eosinophils act to protect against intestinal parasitic nematodes that invade tissues.
5. Immune modulation by eosinophils
However, it has also been reported that eosinophils may promote infection. New larvae of Trichinella spiralis form in the intestinal tract; they then migrate to muscles, where they invade muscle fibers and become capsular larvae [Citation63]. At the stage of new larva infection, IL-10-producing CD4+ T cells or dendritic cells are activated by eosinophil-derived IL-10. Subsequently, CD4+ T/dendritic cell-derived IL-10 suppresses the production of nitric oxide (NO), such that larvae can survive [Citation64]. In contrast, eosinophil-deficient mice exhibit lower numbers of cystic larvae due to insufficient differentiation of IL-10-producing CD4+ T cells, as well as enhanced expression of IFN-γ and enhanced production of NO [Citation65]. Because these results were not observed in mice with neutralizing antibodies against IL-5, and were not observed in mice deficient in IL-5, the increased number of larval infections caused by eosinophils is thought to be due to the presence of resident eosinophils, those are not affected by IL-5 [Citation66–70]. These results are potentially related to the ability of parasites to exploit the immunoregulatory functions of eosinophils.
Resident eosinophils are thus presumed to have an immunoregulatory function; they are present in non-infection/non-allergy (i.e., steady state) conditions [Citation43]. Because they have the ability to enhance or suppress immunity, they may be associated with various diseases.
6. Eosinophil-mediated host protection in acquired immunity
When a host is infected with a parasite, the host acquires immunity to the parasite by retaining memory of specific antigens and thus becomes resistant to reinfection by the same parasite. Re-infecting wild-type mice with N. brasiliensis results in the capturing of parasite larvae in the skin and fewer larvae subsequently migrate to the lungs [Citation71]; however, many larvae reach the lungs in eosinophil-deficient mice [Citation72] ().
Figure 1. Primary and secondary immune responses in helminth infections. (Primary infection) Nematode infection induces differentiation of Th2 cells to induce Th2-type immune responses, such as mucus production and mastocytosis, as well as proliferation and accumulation of eosinophils and basophils, production of nematode antigen-specific antibodies, and differentiation of M2 macrophages. Some nematodes are expelled by these effectors, while others evade these effectors and develop chronic infection. Some nematodes exhibit a lung stage before they become adults in the intestines. When larvae penetrate the lung, they damage lung tissue, which leads to the release of epithelial cell-related cytokines (e.g., IL-33, TSLP, and IL-25) from epithelial and stromal cells; these cytokines induce proliferation of group 2 innate lymphoid cells and subsequent production of cytokines (e.g., IL-5 and IL-13), resulting in the accumulation of eosinophils. Thus, nematode infection induces pulmonary eosinophilia at 1–2 weeks after infection. However, eosinophils cannot damage larvae in the lungs, because nematodes have already moved to the intestines and developed into adult worms. (Secondary infection) If a host is re-infected by a species of nematode that it has previously encountered, Th2 cells and antibodies respond to the parasites robustly and promptly, thus trapping and damaging them at the sites of infection (e.g., skin) and at the lungs, through the actions of effector cells such as basophils and macrophages, prior to migration of the larvae to the intestines. If a host is infected by a species of nematode that it has not previously encountered, Th2 cells and antibodies cannot respond to the parasite antigen with effectiveness similar to that of the response to the sensitizing parasite; thus, the larvae can migrate to the lungs. Following tissue damage by migrating larva, stromal cells produce IL-33. The lungs of an infected host have large numbers of Th2 cells and/or ILC2s, which rapidly react to IL-33 by producing Th2 cytokines. Thus, infected hosts exhibit non-antigen-specific resistance by attacking migratory larvae through the actions of rapidly accumulated eosinophils.
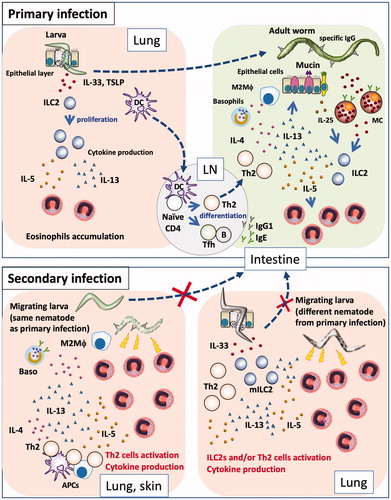
Eosinophils support T. spiralis survival during primary infection as described above. However, during reinfection, eosinophils inhibit the migration of new larvae [Citation73]. Parasite-specific antibodies are critical for eosinophil-mediated resistance to infection. Antibodies enhance the in vitro activity of eosinophils against T. spiralis larvae [Citation74]. These antibodies have also been shown to synergize with basophils and M2 macrophages to inhibit the migration of N. brasiliensis and H. polygyrus [Citation71,Citation75].
7. Non-antigen-specific host defense
Recently, we found that innate immunity against nematode infection also involves a memory component. S. venezuelensis-experienced mice were resistant to the same nematode infection through the actions of Th2 cells [Citation76], but were also resistant to infection by another parasite, N. brasiliensis, regardless of the presence of CD4+ T cells [Citation77]. This resistance was dependent on ILC2s: the ILC2s of mice that had experienced S. venezuelensis infection remained highly reactive even after expulsion of the nematode; they then responded rapidly and robustly to N. brasiliensis infection. In primary infections, the activation of ILC2s is slow, such that when ILC2s produce IL-5 and eosinophils accumulate in the lung, the nematode has already moved from the lung to the intestine; therefore, eosinophils are not very effective against parasites at this stage of infection. However, in infection-experienced mice, large amounts of IL-5 and IL-13 are promptly produced by memory-like ILC2s, which results in rapid accumulation of eosinophils at the site of infection during the early stage of infection; these eosinophils then attack the invading larvae. Importantly, eosinophils are essential for this resistance. Moreover, IL-33 is required for antigen-non-specific resistance, as IL-33-deficient mice did not exhibit protection against secondary infection. Similarly, Martinez-Gonzalez et al. reported that treatment with allergen or IL-33 induced the differentiation of memory-like ILC2s in the lung [Citation78]. They demonstrated that IL-33 alone was sufficient for the induction of memory-like ILC2s; however, in our experiments, IL-33 alone could not induce differentiation of these cells. Thus, the role of IL-33 in the induction of memory-like cell differentiation is controversial. Such parasite species-nonspecific responses are also exhibited by Th2 cells. In response to IL-33 stimulation, Th2 cells produced IL-5 and IL-13 in an antigen-independent manner, which facilitated resistance to N. brasiliensis infection following infection by another lung migratory nematode, Ascaris suum [Citation79] (). In addition to nematode infection, allergens can induce non-antigen-specific host defense.
Gazzinelli-Guimaraes et al. recently reported that house dust mite sensitization significantly limit the number and size of A. suum larva in the lungs, in an eosinophil-dependent manner [Citation80]. Furthermore, Filbey et al. demonstrated that mice could acquire CD4+ T cells/eosinophil dependent resistance against N.brasiliensis infection following infections by H.polygyrus or T. muris, although the life cycles of these species do not include a lung stage [Citation81]. These data are in contrast to the findings of previous reports in which helminth infections were shown to suppress the inflammatory responses in several autoimmune diseases [Citation82–84]. Thus, helminths exhibit complicated immune modulation, and further studies are needed to determine the mechanisms involved in the control of inflammatory diseases and helminth infections.
Thus, eosinophils do not work effectively against every helminth; however, in certain conditions, they contribute to host defense against parasites, especially nematode larvae. In particular, during reinfection, eosinophils participate in anti-parasite immunity by accumulating at the site where larvae are present, before the larvae mature into adults; therefore, it is important that eosinophils gather at the appropriate time and place.
Acknowledgments
The authors thank Ryan Chastain-Gross, Ph.D., from Edanz Group (www.edanzediting.com/ac) for editing a draft of this manuscript.
Disclosure statement
No potential conflict of interest was reported by the authors.
Additional information
Funding
References
- World Health Organization. Research priorities for helminth infections technical report of the TDR disease reference group on helminth infections introduction. WHO technical report series; 972. Geneva, Switzerland: WHO; 2012.
- De Neve J-W, Andriantavison RL, Croke K, et al. Health, financial, and education gains of investing in preventive chemotherapy for schistosomiasis, soil-transmitted helminthiases, and lymphatic filariasis in Madagascar: a modeling study. PLoS Negl Trop Dis. 2018;12(12):e0007002.
- Mitchell GF, Hogarth-Scott RS, Edwards RD, et al. Studies on immune responses to parasite antigens in mice. III. Nippostrongylus brasiliensis infections in hypothymic nu/nu mice. Int Arch Allergy Immunol. 1976;52(1-4):95–104.
- Prowse SJ, Mitchell GF, Ey PL, et al. Nematospiroides dubius: susceptibility to infection and the development of resistance in hypothymic (nude) BALB/c mice. Aust J Exp Biol Med Sci. 1978;56(5):561–570.
- Ruitenberg EJ, Elgersma A, Kruizinga W, et al. Trichinella spiralis infection in congenitally athymic (nude) mice. Parasitological, serological and haematological studies with observations on intestinal pathology. Immunology. 1977;33(4):581–587.
- Kemp M, Kurtzhals JA, Kharazmi A, et al. Interferon-gamma and interleukin-4 in human Leishmania donovani infections. Immunol Cell Biol. 1993;71 (6):583–587.
- Urban JF, Madden KB, Svetica A, et al. The importance of Th2 cytokines in protective immunity to nematodes. Immunol Rev. 1992;127(1):205–220.
- Finkelman FD, Shea-Donohue T, Morris SC, et al. Interleukin-4- and interleukin-13-mediated host protection against intestinal nematode parasites. Immunol Rev. 2004;201(1):139–155.
- Grencis RK. Immunity to helminths: resistance, regulation, and susceptibility to gastrointestinal nematodes. Annu Rev Immunol. 2015;33(1):201–225.
- Yasuda K, Nakanishi K. Host responses to intestinal nematodes. Int Immunol. 2018;30(3):93–102.
- Else KJ, Finkelman FD, Maliszewski CR, et al. Cytokine-mediated regulation of chronic intestinal helminth infection. J Exp Med. 1994;179(1):347–351.
- Bouchery T, Kyle R, Ronchese F, et al. The differentiation of CD4(+) T-helper cell subsets in the context of helminth parasite infection. Front Immunol. 2014;5:487.
- White RR, Artavanis-Tsakonas K. How helminths use excretory secretory fractions to modulate dendritic cells. Virulence. 2012;3(7):668–677.
- Laan LC, Williams AR, Stavenhagen K, et al. The whipworm (Trichuris suis) secretes prostaglandin E2 to suppress proinflammatory properties in human dendritic cells. Faseb J. 2017;31(2):719–731.
- Hammad H, Lambrecht BN. Barrier epithelial cells and the control of Type 2 immunity. Immunity. 2015;43(1):29–40.
- Neill DR, Wong SH, Bellosi A, et al. Nuocytes represent a new innate effector leukocyte that mediates type-2 immunity. Nature. 2010;464(7293):1367–1370.
- Roediger B, Weninger W. Group 2 innate lymphoid cells in the regulation of immune responses. Adv Immunol. 2015;125:111–154.
- Moro K, Kabata H, Tanabe M, et al. Interferon and IL-27 antagonize the function of group 2 innate lymphoid cells and type 2 innate immune responses. Nat Immunol. 2016;17(1):76–86.
- Paul WE. History of interleukin-4. Cytokine. 2015;75(1):3–7.
- Bao K, Reinhardt RL. The differential expression of IL-4 and IL-13 and its impact on type-2 immunity. Cytokine. 2015;75(1):25–37.
- LaPorte SL, Juo ZS, Vaclavikova J, et al. Molecular and structural basis of cytokine receptor pleiotropy in the interleukin-4/13 system. Cell. 2008;132(2):259–272.
- Grünig G, Warnock M, Wakil AE, et al. Requirement for IL-13 independently of IL-4 in experimental asthma. Science. 1998;282(5397):2261–2263.
- Cliffe LJ, et al. Accelerated intestinal epithelial cell turnover: a new mechanism of parasite expulsion. Science. 2005;308(5727):1463–1465.
- Artis D, Wang ML, Keilbaugh SA, et al. RELMbeta/FIZZ2 is a goblet cell-specific immune-effector molecule in the gastrointestinal tract. Proc Natl Acad Sci USA. 2004;101(37):13596–13600.
- Stockinger S, Albers T, Duerr CU, et al. Interleukin-13-mediated paneth cell degranulation and antimicrobial peptide release. J Innate Immun. 2014;6(4):530–541.
- Gerbe F, Sidot E, Smyth DJ, et al. Intestinal epithelial tuft cells initiate type 2 mucosal immunity to helminth parasites. Nature. 2016;529(7585):226–230.
- Howitt MR, Lavoie S, Michaud M, et al. Tuft cells, taste-chemosensory cells, orchestrate parasite type 2 immunity in the gut. Science. 2016;351(6279):1329–1333.
- von Moltke J, Ji M, Liang HE, et al. Tuft-cell-derived IL-25 regulates an intestinal ILC2-epithelial response circuit. Nature. 2016;529(7585):221–225.
- Akiho H, Blennerhassett P, Deng Y, et al. Role of IL-4, IL-13, and STAT6 in inflammation-induced hypercontractility of murine smooth muscle cells. Am J Physiol Gastrointest Liver Physiol. 2002;282(2):G226–232.
- Li L, Xia Y, Nguyen A, et al. Effects of Th2 cytokines on chemokine expression in the lung: IL-13 potently induces eotaxin expression by airway epithelial cells. J Immunol. 1999;162(5):2477–2487.
- Zhu Z, Ma B, Zheng T, et al. IL-13-induced chemokine responses in the lung: role of CCR2 in the pathogenesis of IL-13-induced inflammation and remodeling. J Immunol. 2002;168(6):2953–2962.
- Van Dyken SJ, Locksley RM. Interleukin-4- and interleukin-13-mediated alternatively activated macrophages: roles in homeostasis and disease. Annu Rev Immunol. 2013;31(1):317–343.
- Coffman RL, Savelkoul HF, Lebman DA. Cytokine regulation of immunoglobulin isotype switching and expression. Semin Immunol. 1989;1(1):55–63.
- Goswami R, Kaplan MH. A brief history of IL-9. J Immunol. 2011;186(6):3283–3288.
- Dougan M, Dranoff G, Dougan SK. GM-CSF, IL-3, and IL-5 family of cytokines: regulators of inflammation. Immunity. 2019;50(4):796–811.
- Fulkerson PC, Fischetti CA, McBride ML, et al. A central regulatory role for eosinophils and the eotaxin/CCR3 axis in chronic experimental allergic airway inflammation. Proc Natl Acad Sci USA. 2006;103(44):16418–16423.
- Schmitz J, Owyang A, Oldham E, et al. IL-33, an interleukin-1-like cytokine that signals via the IL-1 receptor-related protein ST2 and induces T helper type 2-associated cytokines. Immunity. 2005;23(5):479–490.
- Yasuda K, Muto T, Kawagoe T, et al. Contribution of IL-33-activated type II innate lymphoid cells to pulmonary eosinophilia in intestinal nematode-infected mice. Proc Natl Acad Sci USA. 2012;109(9):3451–3456.
- Cayrol C, Girard JP. IL-33: an alarmin cytokine with crucial roles in innate immunity, inflammation and allergy. Curr Opin Immunol. 2014;31:31–37.
- Dahlgren MW, Jones SW, Cautivo KM, et al. Adventitial stromal cells define group 2 innate lymphoid cell tissue niches. Immunity. 2019;50(3):707–722.e706.
- Matsuba-Kitamura S, Yoshimoto T, Yasuda K, et al. Contribution of IL-33 to induction and augmentation of experimental allergic conjunctivitis. Int Immunol. 2010;22(6):479–489.
- Huang L, Appleton JA. Eosinophils in helminth infection: defenders and dupes. Trends Parasitol. 2016;32(10):798–807.
- Weller PF, Spencer LA. Functions of tissue-resident eosinophils. Nat Rev Immunol. 2017;17(12):746–760.
- Travers J, Rothenberg ME. Eosinophils in mucosal immune responses. Mucosal Immunol. 2015;8(3):464–475.
- Giacomin PR, Gordon DL, Botto M, et al. The role of complement in innate, adaptive and eosinophil-dependent immunity to the nematode Nippostrongylus brasiliensis. Mol Immunol. 2008;45(2):446–455.
- Ramalho-Pinto FJ, McLaren DJ, Smithers SR. Complement-mediated killing of schistosomula of Schistosoma mansoni by rat eosinophils in vitro. J Exp Med. 1978;147(1):147–156.
- Gounni AS, Lamkhioued B, Ochiai K, et al. High-affinity IgE receptor on eosinophils is involved in defence against parasites. Nature. 1994;367(6459):183–186.
- Khalife J, Dunne DW, Richardson BA, et al. Functional role of human IgG subclasses in eosinophil-mediated killing of schistosomula of Schistosoma mansoni. J Immunol. 1989;142(12):4422–4427.
- Shamri R, Xenakis JJ, Spencer LA. Eosinophils in innate immunity: an evolving story. Cell Tissue Res. 2011;343(1):57–83.
- Löffler W. Flüchtige Lungeninfiltrate mit Eosinophilie. Klin Wochenschr. 1935;14(9):297–299.
- Tindall NR, Wilson PA. An extended proof of migration routes of immature parasites inside hosts: pathways of Nippostrongylus brasiliensis and Strongyloides ratti in the rat are mutually exclusive. Parasitology. 1990;100(2):281–288.
- Takamure A. Migration route of Strongyloides venezuelensis in rodents. Int J Parasitol. 1995;25(8):907–911.
- Cardoso V, Chesné J, Ribeiro H, et al. Neuronal regulation of type 2 innate lymphoid cells via neuromedin U. Nature. 2017;549(7671):277–281.
- Kopf M, Brombacher F, Hodgkin PD, et al. IL-5-deficient mice have a developmental defect in CD5+ B-1 cells and lack eosinophilia but have normal antibody and cytotoxic T cell responses. Immunity. 1996;4(1):15–24.
- Klion AD, Nutman TB. The role of eosinophils in host defense against helminth parasites. J Allergy Clin Immunol. 2004;113(1):30–37.
- Coffman RL, Seymour BW, Hudak S, et al. Antibody to interleukin-5 inhibits helminth-induced eosinophilia in mice. Science. 1989;245(4915):308–310.
- Swartz JM, Dyer KD, Cheever AW, et al. Schistosoma mansoni infection in eosinophil lineage-ablated mice. Blood. 2006;108(7):2420–2427.
- Capron M, Bazin H, Joseph M, et al. Evidence for IgE-dependent cytotoxicity by rat eosinophils. J Immunol. 1981;126(5):1764–1768.
- de Andres B, Rakasz E, Hagen M, et al. Lack of Fc-epsilon receptors on murine eosinophils: implications for the functional significance of elevated IgE and eosinophils in parasitic infections. Blood. 1997;89(10):3826–3836.
- Pearce EJ, Caspar P, Grzych JM, et al. Downregulation of Th1 cytokine production accompanies induction of Th2 responses by a parasitic helminth, Schistosoma mansoni. J Exp Med. 1991;173(1):159–166.
- Svensson M, Bell L, Little MC, et al. Accumulation of eosinophils in intestine-draining mesenteric lymph nodes occurs after Trichuris muris infection. Parasite Immunol. 2011;33(1):1–11.
- Hurst RJ, Else KJ. Trichuris muris research revisited: a journey through time. Parasitology. 2013;140(11):1325–1339.
- Fabre V, Beiting DP, Bliss SK, et al. Eosinophil deficiency compromises parasite survival in chronic nematode infection. J Immunol. 2009;182(3):1577–1583.
- Huang L, Gebreselassie NG, Gagliardo LF, et al. Eosinophil-derived IL-10 supports chronic nematode infection. Ji . 2014;193(8):4178–4187.
- Gebreselassie NG, Moorhead AR, Fabre V, et al. Eosinophils preserve parasitic nematode larvae by regulating local immunity. J Immunol. 2012;188(1):417–425.
- Herndon FJ, Kayes SG. Depletion of eosinophils by anti-IL-5 monoclonal antibody treatment of mice infected with Trichinella spiralis does not alter parasite burden or immunologic resistance to reinfection. J Immunol. 1992;149(11):3642–3647.
- Vallance BA, Matthaei KI, Sanovic S, et al. Interleukin-5 deficient mice exhibit impaired host defence against challenge Trichinella spiralis infections. Parasite Immunol. 2000;22(10):487–492.
- Martin C, Al-Qaoud KM, Ungeheuer M-N, et al. IL-5 is essential for vaccine-induced protection and for resolution of primary infection in murine filariasis. Med Microbiol Immunol. 2000;189(2):67–74.
- Le Goff L, Loke P, Ali HF, et al. J. E. Interleukin-5 is essential for vaccine-mediated immunity but not innate resistance to a filarial parasite. Infect Immun. 2000;68(5):2513–2517.
- Mesnil C, Raulier S, Paulissen G, et al. Lung-resident eosinophils represent a distinct regulatory eosinophil subset. J Clin Invest. 2016;126(9):3279–3295.
- Obata-Ninomiya K, Ishiwata K, Tsutsui H, et al. The skin is an important bulwark of acquired immunity against intestinal helminths. J Exp Med. 2013;210(12):2583–2595.
- Knott ML, Matthaei KI, Giacomin PR, et al. Impaired resistance in early secondary Nippostrongylus brasiliensis infections in mice with defective eosinophilopoeisis. Int J Parasitol. 2007;37(12):1367–1378.
- Huang L, Gebreselassie NG, Gagliardo LF, et al. Eosinophils mediate protective immunity against secondary nematode infection. J Immunol. 2015;194(1):283–290.
- Bass DA, Szejda P. Eosinophils versus neutrophils in host defense. Killing of newborn larvae of Trichinella spiralis by human granulocytes in vitro. J Clin Invest. 1979;64(5):1415–1422.
- Schwartz C, Turqueti-Neves A, Hartmann S, et al. Basophil-mediated protection against gastrointestinal helminths requires IgE-induced cytokine secretion. Proc Natl Acad Sci USA. 2014;111(48):E5169–5177.
- Mukai K, Karasuyama H, Kabashima K, et al. Differences in the importance of mast cells, basophils, IgE, and IgG versus that of CD4+ T cells and ILC2 cells in primary and secondary immunity to Strongyloides venezuelensis. Infect Immun. 2017;85(5):pii: e00053-17.
- Yasuda K, Adachi T, Koida A, et al. Nematode-infected mice acquire resistance to subsequent infection with unrelated nematode by inducing highly responsive group 2 innate lymphoid cells in the lung. Front Immunol. 2018;9:2132.
- Martinez-Gonzalez I, Mathä L, Steer CA, et al. Allergen-experienced group 2 innate lymphoid cells acquire memory-like properties and enhance allergic lung inflammation. Immunity. 2016;45(1):198–208.
- Guo L, Huang Y, Chen X, et al. Innate immunological function of TH2 cells in vivo. Nat Immunol. 2015;16(10):1051–1059.
- Gazzinelli-Guimaraes PH, et al. Allergen presensitization drives an eosinophil-dependent arrest in lung-specific helminth development. J Clin Invest. 2019;130:3686–3701.
- Filbey KJ, Camberis M, Chandler J, et al. Intestinal helminth infection promotes IL-5- and CD4. Mucosal Immunol. 2019;12(2):352–362.
- Gazzinelli-Guimaraes PH, Nutman TB. Helminth parasites and immune regulation. F1000Res. 2018;7:1685.
- Smallwood TB, Giacomin PR, Loukas A, et al. Helminth immunomodulation in autoimmune disease. Front Immunol. 2017;8:453.
- Varyani F, Fleming JO, Maizels RM. Helminths in the gastrointestinal tract as modulators of immunity and pathology. Am J Physiol Gastrointest Liver Physiol. 2017;312(6):G537–G549.