Abstract
Antibodies specific for cardiolipin (CL)-β2-glycoprotein I (β2GPI) are known to induce tissue factor (TF) expression by monocytes and endothelial cells which leads to a prothrombotic state in antiphospholipid syndrome (APS), but the mechanism is not fully elucidated. Previously, we reported that the mouse monoclonal anti-CL-β2GPI antibody WB-6 cross-reacts with DNA, enters monocytes via binding to cell surface DNA, and induces TF expression. The current study aimed to identify the intracellular signaling pathways involved in this process. The binding of WB-6 to CL-β2GPI or DNA, and endocytosis was not prevented by chloroquine, but pre-treatment of the cells with chloroquine significantly suppressed TF expression. TLR9 inhibitory oligodeoxynucleotide also suppressed the WB-6-induced TF expression, suggesting a pivotal role of the TLR9 pathway in TF production. Serum antibodies obtained from a patient with APS accompanying systemic lupus erythematosus (SLE) bound to both CL-β2GPI and DNA, and induced TF in normal monocytes. This effect was suppressed by chloroquine, and abolished by removal of the DNA-binding activity. These results suggest that induction of TF expression results from TLR9 activation by DNA which was internalized together with cross-reactive antibodies produced in secondary APS accompanying SLE.
1. Introduction
Antiphospholipid syndrome (APS) is characterized by recurrent arterial thrombosis, venous thrombosis, pregnancy morbidity, and the presence of antiphospholipid antibodies [Citation1,Citation2]. About a half of APS cases occur without underlying disease and are designated primary APS, whereas secondary APS is associated with other diseases, most commonly systemic lupus erythematosus (SLE) [Citation3]. Of the different antiphospholipid antibodies, the presence of those which indirectly bind to phospholipids via phospholipid-binding proteins such as β2-glycoprotein I (β2GPI), but not those binding directly, has been reported to highly correlate with thrombotic episodes [Citation4]. Anti-β2GPI antibodies are believed to cause a prothrombotic state by several different mechanisms including inhibition of natural anticoagulant and fibrinolytic systems, and activation of monocytes, neutrophils, platelets and vascular endothelial cells [Citation5]. Of these mechanisms, induction of tissue factor (TF) expression on the surface of monocytes or endothelial cells is a major contributor to the pathogenesis, but the precise mechanism by which anti-β2GPI antibodies stimulate the cells to produce TF has not been fully elucidated.
β2GPI is a plasma protein of approximately 50 kDa consisting of five homologous sushi-domains, and has a closed circular form. Once the cluster of positively charged amino acids at the end of the C-terminus fifth domain binds to anionic phospholipids, such as cardiolipin (CL) (used in laboratory tests), or phosphatidylserine on the surface of apoptotic cells, the β2GPI converts to an open fishhook-like conformation resulting in the exposure to clinically relevant anti-β2GPI antibodies of the cryptic epitope in the first domain [Citation6–8]. Thus, anti-β2GPI antibodies bind to apoptotic cells and act as opsonins to facilitate the clearance and processing of these cells [Citation9]. Although the receptor for β2GPI has not been clearly identified, anti-β2GPI antibodies are also known to bind to β2GPI on live cells and exert biological effects. For example, these antibodies have been suggested to cross-link Toll-like receptor (TLR) 4 which may be interacting with β2GPI, and activate intracellular signaling pathways leading to expression of TF on monocytes [Citation10–12]. Another study suggested that antiphospholipid antibodies stimulate monocytes to express TNFα, which is a potent TF inducer, through activation of the TLR7 and TLR8 pathways [Citation13]. Furthermore, endocytosis of antiphospholipid antibodies associated with TLR2 was reported to be essential for expression of TF and TNFα by monocytes [Citation14]. Thus, different antibodies and assay conditions seem to have led to different conclusions in terms of the involvement of Toll-like receptors in monocyte activation.
Previously, we generated a mouse monoclonal antibody designated WB-6 from a lupus-prone male (NZW x BXSB)F1 mouse, and reported that it binds to CL-β2GPI, induces TF on normal human as well as mouse monocytes, and induces a prothrombotic state in an in vivo mouse model of APS [Citation15]. During studies on how WB-6 interacts with monocytes, we found that this antibody binds to DNA as well as to CL-β2GPI, and enters living cells via binding to the cell surface DNA [Citation16]. Based on these findings, we proposed a novel hypothesis that some anti-CL-β2-GPI antibodies are endocytosed into living monocytes together with the bound DNA, and thus stimulate intracellular DNA sensors, leading to expression of TF on the cells. In the current study, we extend the previous work to further dissect this mechanism, and demonstrate that the reactivity of WB-6 results not from indirect binding via DNA, but from direct cross-reactivity of the antibody to DNA in addition to CL-β2GPI. Induction of TF expression by WB-6 was dependent on the presence of DNA. It was suppressed by chloroquine (CQ) and by TLR9 inhibitory oligodeoxynucleotide (ODN), suggesting a pivotal role of the TLR9 pathway in these events. Furthermore, IgG from a patient was found to bind to both CL-β2GPI and DNA, and to induce TF expression on normal monocytes dependently on this DNA-binding activity, suggesting the relevance of the TLR9 pathway especially in secondary APS associated with SLE.
2. Materials and methods
2.1. Cells and antibodies
The study protocol was approved by TMDU Faculty of Medicine Ethics Committee (M2000-1480). SLE and APS were defined by standard classification criteria [Citation17,Citation18], and serum samples obtained from patients with written informed consent were provided to this study stripped of the clinical findings. Peripheral blood mononuclear cells (PBMCs) from healthy volunteers were isolated by density gradient centrifugation over Ficoll-Conray solution.
Monoclonal antibodies WB-6 and 2C10 were generated from an (NZW x BXSB)F1 mouse [Citation15], and an MRL/lpr mouse [Citation19], respectively. MRL/lpr-derived H241 was a gift from Dr David Stollar [Citation20]. Isotype of these antibodies are IgG2b, κ. Monoclonal antibodies were purified from hybridoma culture supernatant by salting out in half-saturated ammonium sulfate followed by Protein A or Protein G column chromatography. For some experiments, they were further purified by the method described by Fenton et al. [Citation21] with modification. Briefly, WB-6 or H241 bound to Protein A-Sepharose (Sigma Aldrich, St Louis, MO, USA) was washed with high-salt alkaline buffer (3 M NaCl, 1.5 M glycine, pH 8.9) followed by washing with PBS (pH 7.4), and then treated with 20 U/ml DNase I (Sigma Aldrich) in 40 mM Tris buffer containing 6 mM MgCl2 (pH 7.5) or 500 U/ml S1 nuclease (Takara Bio, Kusatsu, Japan) in 30 mM acetate buffer containing 280 mM NaCl and 1 mM ZnSO4 (pH 4.6) for 1 h at room temperature. After washing with PBS, antibodies were eluted with 0.1 M citrate buffer (pH 2.7) and dialyzed against PBS. Antibody purity was confirmed by SDS-PAGE and UV spectrum analysis. Antibody concentration was determined by Bradford protein assay. Monoclonal isotype-matched control IgG (IC IgG) was purchased from R&D Systems (Minneapolis, MN, USA). Final concentrations of lipopolysaccharides (LPS) in culture media derived from antibody preparations were, if any, confirmed to be <50 pg/ml by Limulus Color KY Test (FUJIFILM Wako Chemical, Osaka, Japan).
2.2. Estimation of antibody activity
Antibody binding to CL-β2GPI was determined by enzyme-linked immunosorbent assay (ELISA) as described previously [Citation15]. DNA binding was also determined by ELISA as described [Citation16]. Calf-thymus DNA (Sigma Aldrich) was dissolved in Tris-buffered saline (TBS; 25 mM Tris, 140 mM NaCl, pH 7.4) containing 5 mM EDTA, and used as double-stranded (ds) DNA. After denaturing in boiling water for 5 min followed by rapid chilling in iced water, it was used as single-stranded (ss) DNA. In inhibition assays, antibodies were preincubated in microtubes with the inhibitors for 30 min, and then introduced into the antigen-coated ELISA plates.
2.3. Detection of internalized antibodies
PBMCs were seeded in 48-well plates (3 × 105 cells/well) in RPMI-1640 containing 10% fetal bovine serum, 100 U/ml penicillin, 100 µg/ml streptomycin, and 10 mM non-essential amino acids. After incubation with 0.5–20 µg/ml 2C10, H241, WB-6, or IC IgG for 2–5 h at 37 °C in a CO2 incubator, unbound antibody was removed by washing with ice-cold PBS, and the cells were fixed with 2% paraformaldehyde-PBS for 5 min, washed with PBS, and permeabilized with 0.2% Tween 20-PBS. After blocking with 1% BSA-PBS, the cells were stained with Alexa Fluor 488-labeled goat anti-mouse IgG (Abcam, Cambridge, MA, USA) for 30 min at room temperature, then analyzed by flow cytometry (CytoFLEX, Beckman Coulter, Bream, CA, USA). For immunocytochemistry, after nuclear staining with Hoechst 33342, cells were imaged using a fluorescence microscope (Keyence, Osaka, Japan) or a confocal laser scanning microscope TCS SP8 (Leica, Tokyo, Japan).
2.4. Detection of TF
After incubation of PBMCs with anti-DNA antibodies as above, or LPS as a positive control, the cells were washed with cold PBS and incubated with phycoerythrin-conjugated anti-human CD142 antibody (Thermo Fisher Scientific, Waltham, MA, USA) for 20 min. After washing with 1% BSA-PBS, the cells were analyzed by flow cytometry.
2.5. Tests for the effect of CQ
PBMCs were preincubated with CQ diphosphate (50 µM in , or 100 µM in ; R&D Systems) for 30 min at 37 °C in a CO2 incubator. After washing with fresh medium, cells were re-seeded and incubated with anti-DNA antibodies as described above. (cf. In clinical usage, blood concentrations of chloroquine or hydroxychloroquine is usually less than 1 µM.)
2.6. Tests for the effect of a TLR9 antagonist
TLR9 inhibitory phosphorothioated oligodeoxynucleotide (ODN) IRS869 (5′-TCCTGGAGGGGTTGT-3′) [Citation22] or the control phosphorothioated ODN (5′-TCCTGCAGGTTAAGT-3′) (both from Sigma Aldrich) was mixed with DOTAP liposomal transfection reagent (Roche Diagnostics, Mannheim, Germany) in liposome buffer and incubated for 10 min at room temperature according to the manufacturer’s protocol. The mixture was gently added to the cell culture and incubated for 30 min. The final concentration of the ODN was 1.25 pmol/µl. After replacing with fresh medium, cells were incubated with anti-DNA antibodies as described above.
2.7. Absorption of DNA-binding activity in patient serum
Serum samples were obtained from patients with APS and antibody activity to DNA and CL-β2GPI was tested using the same protocol as for the monoclonal antibodies except that the second antibody was anti-human IgG-alkaline phosphatase. To remove the DNA-binding antibodies from one of the sera which showed the highest reactivity to both DNA and CL-β2GPI, poly-L-lysine (PLL) was coupled to cyanogen bromide-activated Sepharose 4B (GE Healthcare) according to the manufacturer’s protocol. 5 mg of salmon testes DNA (Sigma Aldrich) was attached by rolling overnight with 1 mL of the PLL-Sepharose gel in 25 mM phosphate buffer (pH 7.0). To remove anti-DNA antibodies in the patient serum, 24 µL of the serum was incubated with 100 µL of the DNA-PLL-Sepharose gel suspended in 600 µL RPMI complete medium for 30 min. After centrifugation, the supernatant was used in the experiments.
2.8. Statistical analyses
Data were analyzed by two-tailed unpaired parametric t-test for data shown in , or Tukey’s multiple comparisons test for . p < .05 was considered significant.
3. Results
3.1. Highly purified WB-6 binds to both DNA and CL-β2GPI
Previously, based on the results of direct binding ELISA, we had reported that the monoclonal antibody WB-6 purified using a Protein-G column binds to both DNA and CL-β2GPI [Citation16]. Although this was reproducible, we were not fully confident of the reactivity because one partner was nucleic acid and the other protein; do they share a common structure? Therefore, here we first confirmed the reactivity of WB-6 in a direct binding ELISA. WB-6 bound preferentially to CL-β2GPI in a dose-dependent manner and more strongly than to CL alone or β2GPI alone (), consistent with our previous findings. Furthermore, data from an inhibition ELISA showed that the binding of WB-6 to CL-β2GPI was inhibited by ssDNA (), suggested the presence of a negatively charged epitope shared between the sugar-phosphate backbone of DNA and the open form of β2GPI. Anti-DNA antibodies, however, are known to exist as immune complexes both in vivo and in vitro, and so we wondered whether WB-6 binds directly to the antigens. To resolve this problem, WB-6 purified by a conventional method using protein A or protein G (C-WB-6) was further purified by washing with high-salt alkaline buffer and enzyme digestion with DNase 1 (P1-WB-6) or S1 nuclease (P2-WB-6). By ultraviolet absorbance spectrometry, P1-WB-6 and P2-WB-6 showed higher A280/A260 ratios than C-WB-6, indicating that the latter carried substantial amounts of nucleic acids (). Using these highly purified preparations, we retested the antibody and found that both P1-WB-6 and P2-WB-6 bound to DNA and CL-β2GPI at similar concentrations to C-WB-6. This indicated that the binding pattern of WB-6 definitely arose from direct cross-reactivity ().
Figure 1. Highly purified WB-6 cross-reacts with CL-β2GPI and DNA. (A) Reactivity of WB-6 (purified by Protein G) to [CL + β2GPI], [CL + TBS (vehicle for β2GPI)], [Ethanol (vehicle for CL) + β2GPI] was tested by ELISA. (B) Binding activity of WB-6 (purified by Protein G) to [CL + β2GPI] was tested after pre-incubation with ssDNA or dsDNA. (C) After spectrophotometric analysis, the A280/A260 ratio was plotted for conventionally purified C-WB-6 (purified by Protein G), P1-WB-6 (purified with high-salt alkaline wash followed by DNase 1 treatment), and P2-WB-6 (purified with high-salt alkaline wash followed by S1nuclease treatment). (D) Binding activity of highly purified P1-WB-6 (circles) and P2-WB-6 (triangles) to ssDNA (open symbols) and dsDNA (closed symbols). (E) Binding activity of highly purified P1-WB-6 (open circles) and P2-WB-6 (closed circles) to CL-β2GPI.
![Figure 1. Highly purified WB-6 cross-reacts with CL-β2GPI and DNA. (A) Reactivity of WB-6 (purified by Protein G) to [CL + β2GPI], [CL + TBS (vehicle for β2GPI)], [Ethanol (vehicle for CL) + β2GPI] was tested by ELISA. (B) Binding activity of WB-6 (purified by Protein G) to [CL + β2GPI] was tested after pre-incubation with ssDNA or dsDNA. (C) After spectrophotometric analysis, the A280/A260 ratio was plotted for conventionally purified C-WB-6 (purified by Protein G), P1-WB-6 (purified with high-salt alkaline wash followed by DNase 1 treatment), and P2-WB-6 (purified with high-salt alkaline wash followed by S1nuclease treatment). (D) Binding activity of highly purified P1-WB-6 (circles) and P2-WB-6 (triangles) to ssDNA (open symbols) and dsDNA (closed symbols). (E) Binding activity of highly purified P1-WB-6 (open circles) and P2-WB-6 (closed circles) to CL-β2GPI.](/cms/asset/ffdc1bbd-7efb-42d4-979f-4ccb6945c37a/timm_a_1796285_f0001_c.jpg)
3.2. Kinetic properties of anti-DNA antibody internalization
WB-6 enters THP-1 cells, monocytes and endothelial cells [Citation16]. In the present study, we investigated the kinetics of this phenomenon using PBMCs from healthy volunteers comparing WB-6 with other monoclonal anti-DNA antibodies. 2C10 is a dsDNA-specific antibody which does not react with ssDNA or CL-β2GPI. H241 binds to both dsDNA and ssDNA, and is not reactive to CL-β2GPI (data not shown). All antibodies tested were preferentially incorporated into slightly larger cells in PBMCs which correspond to monocytes (). By flow cytometry, we confirmed that CD14+ cells are the major population which incorporated the antibodies. Kinetics of antibody incorporation by monocytes were different for the different antibodies. During the 5 h observation period, the fraction of 2C10-positive monocytes stayed constant at 50% or above, but although WB-6-positive monocytes also peaked at about 50% at 3 h, the number of positive cells decreased thereafter. In contrast, the number of H241-positive monocytes peaked already at 2 h, and also declined later ().
Figure 2. Internalization of monoclonal anti-DNA antibodies. (A) PBMCs were cultured with WB-6 (20 µg/ml) for 3 h and imaged by Alexa Fluor 488-labeled anti-mouse IgG after fixation and permeabilization. (B) PBMCs were cultured with 5 µg/ml 2C10, 20 µg/ml WB-6, 0.5 µg/ml H241, or IC IgG for 2, 3, 4 or 5 h, and after fixation and permeabilization, IgG-positive monocytes was estimated by flow cytometry gated on the CD14+ population.
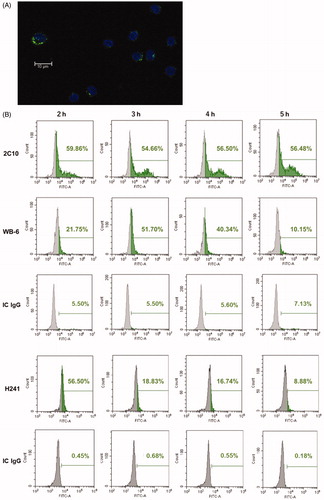
3.3. Wb-6 but not 2C10 or H241 induces TF expression on monocytes
WB-6 is known to induce TF expression on monocytes [Citation16]. To test whether this is a unique property of WB-6 or usual for anti-DNA antibodies, we compared the effects of three monoclonal antibodies all of which were confirmed to enter live monocytes as shown above. As indicated in , when the cells were incubated for 3 h with 20 µg/ml WB-6, the percentage of WB-6-positive monocytes was comparable to that of the cells incubated with 5 µg/ml 2C10. Therefore, we first compared the effect of 20 µg/ml WB-6 and 5 µg/ml 2C10 (). In unstimulated monocytes or those incubated with 20 µg/ml IC IgG, TF expression remained essentially absent during the 5 h incubation period. In contrast, WB-6 induced TF expression over time and reached a maximum at 5 h. Interestingly, 2C10 did not induce TF significantly despite also being internalized. To exclude the possibility that the higher concentration of WB-6 than 2C10 caused this difference, we tested the effect of 5 µg/ml WB-6 and confirmed that WB-6 still significantly induced TF expression (). In contrast, 2C10 did not induce TF expression even at a higher concentration (). Although not statistically significant, a high concentration of H241 showed a tendency to induce TF (). These results indicate that only some, not all, anti-DNA antibodies which can be internalized by monocytes are able to induce TF.
Figure 3. WB-6, but not 2C10 or H241, induces TF expression. PBMCs were incubated for 2-5 h with 5 µg/ml 2C10, 20 µg/ml WB-6 or 20 µg/ml IC IgG and TF expression on monocytes were estimated by flow cytometry (A). PBMCs were incubated for 5 h with 5 or 20 µg/ml WB-6 (B), 2C10 (C) or H241 (D) and TF expression on monocytes were estimated. Each bar shows mean ± SEM of the triplicate assay. *p < .05, **p < .01, ***p < .001.
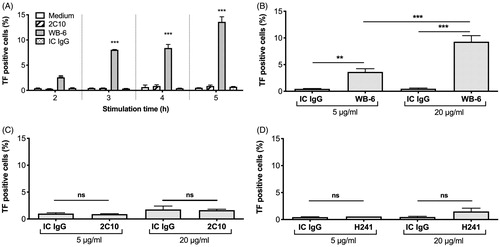
3.4. Wb-6-induced TF expression is suppressed by CQ and TLR9 inhibitory ODN
Endocytosis of WB-6 into monocytes is DNA-dependent because pre-treatment of cells with DNase I reduces the internalization [Citation16]. In addition, currently we observed that highly-purified P1-WB-6 and P2-WB-6 induced less amount of TF than C-WB-6 (Supplementary Figure). These results suggest involvement of intracellular DNA sensors of the innate immune system in TF induction by WB-6. To clarify this, we treated the cells with CQ, which is known to inhibit CpG-stimulated activation of the TLR9 pathway [Citation23], before incubation with WB-6. We found that this treatment nearly completely suppressed TF induction by WB-6, suggesting a pivotal role of the TLR9 pathway (). In contrast, TF induction by LPS, which is thought to stimulate cells mainly through the TLR4 pathway, was not suppressed by the same concentration of CQ.
Figure 4. Effect of chloroquine (CQ) and TLR9-inhibitory oligodeoxynucleotide (ODN) on WB-6-induced TF expression. (A) After treatment with 50 µM CQ for 30 min and washing with fresh medium, PBMCs were incubated with 20 µg/ml WB-6, IC IgG, or 100 ng/ml LPS for 5 h and TF-positive monocytes were quantified by flow cytometry. Each bar shows mean ± SEM of the triplicate assay. ***p < .001. (B) Incorporation of IgG by monocytes after incubation as above was estimated by fluorescence microscopy, and representative images are shown. (C) 20 µg/ml WB-6 was pre-incubated for 30 min with the indicated concentrations of CQ, and incubated on ELISA plates coated with CL-β2GPI (left panel), ssDNA or dsDNA (right panel). (D) After treatment with IRS869, control ODN (Ctrl) or distilled water (DW), PBMCs were incubated with 20 µg/ml WB-6 for 5 h and TF-positive monocytes were quantified by flow cytometry. Each bar shows mean ± SEM of the triplicate assay. **p < .01.
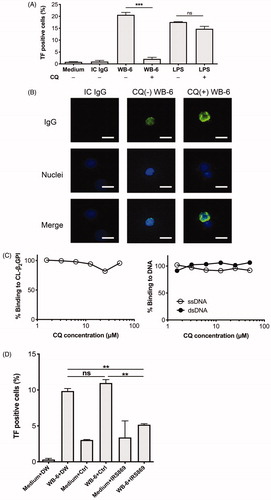
CQ is not necessarily a specific inhibitor of TLR9 and is known to have several different loci of action. For example, one study has shown that CQ inhibited caveola-mediated endocytosis of Japanese encephalitis virus [Citation24]. We therefore tested whether CQ inhibits internalization of WB-6, but found that this was not the case (). Rather, cytoplasmic fluorescence intensity tended to be enhanced by pre-treatment of the cells with CQ. We have not yet determined the mechanism responsible for this effect. Another study reported that hydroxychloroquine reduces the formation of antibody-β2GPI-phospholipid complexes [Citation25]. Thus, we tested whether this is the case with WB-6, but found that CQ did not inhibit the binding of WB-6 to CL-β2GPI; neither did it inhibit the binding of WB-6 to DNA ().
To further confirm the involvement of the TLR9 pathway in WB-6-induced TF expression, we tested the effect of a TLR9 antagonist. We found that the inhibitory ODN, IRS869, but not the control ODN, suppressed TF induction by WB-6 ().
3.5. Cross-reactive polyclonal antibodies from a patient also induce TF through TLR9
We were interested to determine whether the above results are limited to the properties of a particular monoclonal antibody or are shared with antibodies from patients. We obtained a serum sample from a patient with APS associated with SLE and found that her polyclonal IgG bound to both CL-β2GPI and DNA. This serum stimulated normal monocytes to express TF, which was also suppressed by CQ (). The DNA binding activity was almost completely removed by incubating this serum with DNA-Sepharose (). CL-β2GPI binding activity was also drastically reduced by this procedure, but still some binding activity remained suggesting that this IgG contains cross-reactive antibodies to CL-β2GPI and DNA, and also antibodies that are more specific for CL-β2GPI. Interestingly, depletion of the DNA-binding activity abolished the TF-inducing activity of this serum, suggesting the involvement of the TLR9 pathway analogous to the case of WB-6 ().
Figure 5. Induction of TF-expression by antibodies from a patient. (A) PBMCs obtained from healthy volunteers were incubated with 2% patient serum or 10 ng/ml LPS for 5 h, and the percentage of TF-positive monocytes was quantified by flow cytometry. Histograms show representative results of three independent experiments. In the lower panels, PBMCs were pre-treated with chloroquine (CQ), and a bar graph shows an analysis of the triplicate assay of the representative experiment. Each bar shows mean ± SEM. **p < .01. (B) Before and after removal of DNA-binding antibodies, binding activity of the patient serum, along with control serum from a healthy volunteer, to DNA (left panel) and CL-β2GPI (right panel) was tested by ELISA. (C) Before and after removal of DNA-binding antibodies, TF-inducing activity of the patient serum, along with control serum from a healthy volunteer, was tested by flow cytometry and a representative result of triplicate assay is shown. Each bar shows mean ± SEM. ***p < .001.
4. Discussion
In the current study, by stringently purifying the monoclonal antibody WB-6 we demonstrated that it is truly cross-reactive to CL-β2GPI and DNA. Other tested anti-DNA antibodies 2C10 and H241 were not cross-reactive to CL-β2GPI. Although with different kinetics, these three antibodies were incorporated mainly by monocytes when incubated with PBMCs. As a result, WB-6 induced expression of TF, but 2C10 or H241 did not. This effect of WB-6 was dependent on the presence of DNA, and was suppressed by CQ as well as by ODN IRS869, indicating the involvement of the TLR9 signaling pathway. Serum polyclonal IgG antibodies obtained from a patient with APS and SLE bound to CL-β2GPI and DNA, and induced TF expression on normal monocytes as well, and this effect was also abolished by abrogating DNA-binding activity. These results suggest the presence of WB-6-like cross-reactive antibodies in at least some patients with secondary APS complicated with SLE.
It is well known that polyclonal anti-DNA antibodies in SLE sera cross-react with a variety of nucleic acids. This is also the case with monoclonal anti-DNA antibodies, and many examples of such cross-reactivity have been published using diverse forms of DNA including synthetic polynucleotides [Citation26]. In addition, certain anti-DNA antibodies bind to some types of lipids, sugars or proteins [Citation27,Citation28]. However, anti-DNA antibodies inevitably exist as immune complexes with DNA in vitro and in vivo. Therefore, we were cautious in our interpretation of dual-reactivity of WB-6; i.e. to distinguish whether it resulted from direct reaction between the antibody and the antigens, or indirect reaction through DNA, nucleosome, other nucleic acids, or may be some DNA-binding proteins. With this in mind, we thoroughly purified the antibody for some experiments by washing with high-salt alkaline buffer followed by nuclease digestion. Nonetheless, we found that the highly purified WB-6 still bound to both CL-β2GPI and DNA, suggesting the presence of a common epitope shared between open-form β2GPI and DNA. This kind of cross-reactivity is not impossible and has in fact been predicted by others by using a computer model of residue-residue interactions across the interface of anti-DNA antibodies and β2GPI [Citation29].
Why WB-6 induces TF but 2C10 or H241 do not is a puzzle. Recently we reported that 2C10-DNA complex is incorporated by monocytes through macropinocytosis, and that the DNA stimulates the TLR9 and AIM-2 pathways resulting in expression of several inflammatory cytokines relevant to SLE pathogenesis [Citation30]. We usually observe that 2C10 enters live cells more readily than WB-6 even at low concentrations as shown in . WB-6 binds to β 2GPI but 2C10 does not, so it is therefore possible that the binding of WB-6 to cell surface β 2GPI and activation of interactive TLR pathways other than TLR9 alone is also needed to fully activate the cells to express TF. Supporting this hypothesis, TF induction by WB-6 was decreased in serum-free (i.e. β2GPI-free) medium (not shown). However, further experiments are required to reach a definitive conclusion on this issue because absence of serum in culture may affect many cellular functions. Another possible explanation is also implicated by the specificity of the antibodies. A major part of the cell-free DNA in culture medium or in plasma is present as nucleosomes in which dsDNA is protected from degradation by tight association with histones. 2C10 recognizes the tertiary structure of dsDNA and forms immune complexes with nucleosomes [Citation31]. On the other hand, recent studies have revealed the presence of ssDNA in cancer patients’ plasma and, at lower concentrations, in healthy subjects [Citation32]. Furthermore, another study reported abundant fragmented and partially single-stranded mitochondrial DNA in plasma of organ transplant recipients [Citation33], although to the best of our knowledge, this issue has not been examined in SLE. Because WB-6 has a much higher affinity for ssDNA than dsDNA (), it seems plausible that it carries degraded DNA originating from mitochondria released from apoptotic cells. Mammalian nucleosome-derived DNA is not an appropriate TLR9 ligand, but mitochondrial DNA is, because it contains abundant unmethylated CpG. Accordingly, induction of TF expression by CpG DNA, but not by mammalian DNA, has been observed by others [Citation34]. Because pathological conditions of SLE and APS include increased formation of neutrophil extracellular traps, increased generation of reactive oxygen species, and increased release of microparticles, it is likely that mitochondrial DNA is involved in the pathogenesis of these disorders as one of the key players [Citation35].
It is noteworthy that Brandt et al. [Citation14] have argued that TLR2 and its co-receptors, TLR1 and TLR6, are endocytosed by monocytes accompanying with CD14, β2GPI and anti-β2GPI antibodies. This resulted in the induction of TF and TNF, and was suppressed by blocking antibodies to TLR2, TLR1 or TLR6, but not to TLR4. They used IgG obtained from patients mostly with primary APS, and anti-DNA activity was not mentioned. On the other hand, Prinz et al. [Citation13] observed that human monoclonal anti-phospholipid antibodies accumulated in the endosomes of monocytes and plasmacytoid dendritic cells. This resulted in activation of endosomal NADPH oxidase and the generation of superoxide, which then sensitized TLR7 or TLR8 to their natural ligands, leading to production of inflammatory cytokines. Their antibodies are described not to bind to β2GPI, DNA, nor RNA. Thus, multiple antiphospholipid antibodies have been reported to cause a prothrombotic state through activation of different intracellular signaling pathways. However, investigations focused on secondary APS are very limited, and the current study provides a novel view of pathogenic mechanisms of APS especially associated with SLE. One of the limitations is we have analyzed only one clinical sample. A future research plan to compare the TF-inducing effect of serum antibodies from primary APS and secondary APS, along with exploration of the intracellular signaling molecules activated by each group, would be fascinating.
Hydroxychloroquine is widely prescribed for SLE, and has been demonstrated to be effective through interfering with inflammatory signaling pathways, reducing anti-DNA antibodies and normalizing complement activity [Citation36]. Effects of hydroxychloroquine on APS remain to be established, although one noteworthy study showed a reduction of serum soluble TF levels after 3 months’ treatment [Citation37]. Signaling pathways activated by antiphospholipid antibodies are heterogeneous as mentioned above, and additionally, loci of action of CQ or hydroxychloroquine are not completely understood. Nonetheless, the current study suggests that therapeutic strategies targeting the TLR9 pathway may be beneficial for treating secondary APS accompanying SLE.
Supplemental Material
Download MS Word (716.2 KB)Disclosure statement
All the authors have no conflicts of interest to disclose.
Additional information
Funding
References
- Svenungsson E, Antovic A. The antiphospholipid syndrome – often overlooked cause of vascular occlusions? J Intern Med. 2020;287(4):349–372.
- Linnemann B. Antiphospholipid syndrome – an update. VASA. 2018;47(6):451–464.
- Cervera R, Piette J-C, Font J, et al. Antiphospholipid syndrome: clinical and immunologic manifestations and patterns of disease expression in a cohort of 1,000 patients. Arthritis Rheum. 2002;46(4):1019–1027.
- de Laat HB, Derksen R, Urbanus RT, et al. beta2-glycoprotein I-dependent lupus anticoagulant highly correlates with thrombosis in the antiphospholipid syndrome. Blood. 2004;104(12):3598–3602.
- Chaturvedi S, McCrae KR. Diagnosis and management of the antiphospholipid syndrome. Blood Rev. 2017;31(6):406–417.
- de Laat B, Derksen R, Urbanus RT, et al. IgG antibodies that recognize epitope Gly40-Arg43 in domain I of beta 2-glycoprotein I cause LAC, and their presence correlates strongly with thrombosis. Blood. 2005;105(4):1540–1545.
- Agar Ç, van Os GMA, Mörgelin M, et al. Beta2-glycoprotein I can exist in 2 conformations: implications for our understanding of the antiphospholipid syndrome. Blood. 2010;116(8):1336–1343.
- Mahler M, Norman GL, Meroni PL, et al. Autoantibodies to domain 1 of beta 2 glycoprotein 1: a promising candidate biomarker for risk management in antiphospholipid syndrome. Autoimmun Rev. 2012;12(2):313–317.
- Cocca BA, Seal SN, D'Agnillo P, et al. Structural basis for autoantibody recognition of phosphatidylserine-beta 2 glycoprotein I and apoptotic cells. Proc Natl Acad Sci USA. 2001;98(24):13826–13831.
- Sorice M, Longo A, Capozzi A, et al. Anti-beta2-glycoprotein I antibodies induce monocyte release of tumor necrosis factor alpha and tissue factor by signal transduction pathways involving lipid rafts. Arthritis Rheum. 2007;56(8):2687–2697.
- Zhou H, Yan Y, Xu G, et al. Toll-like receptor (TLR)-4 mediates anti-β2GPI/β2GPI-induced tissue factor expression in THP-1 cells. Clin Exp Immunol. 2011;163(2):189–198.
- Meroni PL, Borghi MO, Raschi E, et al. Pathogenesis of antiphospholipid syndrome: understanding the antibodies. Nat Rev Rheumatol. 2011;7(6):330–339.
- Prinz N, Clemens N, Strand D, et al. Antiphospholipid antibodies induce translocation of TLR7 and TLR8 to the endosome in human monocytes and plasmacytoid dendritic cells. Blood. 2011;118(8):2322–2332.
- Brandt KJ, Fickentscher C, Boehlen F, et al. NF-κB is activated from endosomal compartments in antiphospholipid antibodies-treated human monocytes. J Thromb Haemost. 2014;12(5):779–791.
- Nishimura M, Nii T, Trimova G, et al. The NF-kB specific inhibitor DHMEQ prevents thrombus formation in a mouse model of antiphospholipid syndrome. J Nephropathol. 2013;2(2):114–121.
- Virachith S, Saito M, Watanabe Y, et al. Anti-β2 -glycoprotein I antibody with DNA binding activity enters living monocytes via cell surface DNA and induces tissue factor expression. Clin Exp Immunol. 2019;195(2):167–178.
- Aringer M, Costenbader K, Daikh D, et al. 2019 European League against rheumatism/American College of Rheumatology classification criteria for systemic lupus erythematosus. Ann Rheum Dis. 2019;78(9):1151–1159.
- Miyakis S, Lockshin MD, Atsumi T, et al. International consensus statement on an update of the classification criteria for definite antiphospholipid syndrome (APS). J Thromb Haemost. 2006;4(2):295–306.
- Kubota T, Watanabe N, Kanai Y, et al. Enhancement of oxidative cleavage of DNA by the binding sites of two anti-double-stranded DNA antibodies. J Biol Chem. 1996;271(11):6555–6561.
- Stollar BD, Zon G, Pastor RW. A recognition site on synthetic helical oligonucleotides for monoclonal anti-native DNA autoantibody. Proc Natl Acad Sci Usa. 1986;83(12):4469–4473.
- Fenton KA, Tømmerås B, Marion TN, et al. Pure anti-dsDNA mAbs need chromatin structures to promote glomerular mesangial deposits in BALB/c mice. Autoimmunity. 2010;43(2):179–188.
- Lenert PS. Classification, mechanisms of action, and therapeutic applications of inhibitory oligonucleotides for Toll-like receptors (TLR) 7 and 9. Mediators Inflamm. 2010;2010:986596.
- Torigoe M, Sakata K, Ishii A, et al. Hydroxychloroquine efficiently suppresses inflammatory responses of human class-switched memory B cells via Toll-like receptor 9 inhibition. Clin Immunol. 2018;195:1–7.
- Zhu Y-Z, Xu Q-Q, Wu D-G, et al. Japanese encephalitis virus enters rat neuroblastoma cells via a pH-dependent, dynamin and caveola-mediated endocytosis pathway. J Virol. 2012;86(24):13407–13422.
- Rand JH, Wu X-X, Quinn AS, et al. Hydroxychloroquine directly reduces the binding of antiphospholipid antibody-beta2-glycoprotein I complexes to phospholipid bilayers. Blood. 2008;112(5):1687–1695.
- Shoenfeld Y, Rauch J, Massicotte H, et al. Polyspecificity of monoclonal lupus autoantibodies produced by human-human hybridomas. N Engl J Med. 1983;308(8):414–420.
- Radic MZ, Weigert M. Genetic and structural evidence for antigen selection of anti-DNA antibodies. Annu Rev Immunol. 1994;12:487–520.
- Stemmer C, Richalet-Sécordel P, van Bruggen M, et al. Dual reactivity of several monoclonal anti-nucleosome autoantibodies for double-stranded DNA and a short segment of histone H3. J Biol Chem. 1996;271(35):21257–21261.
- Kumar S, Nagl S, Kalsi JK, et al. Anti-cardiolipin/beta-2 glycoprotein activities co-exist on human anti-DNA antibody light chains. Mol Immunol. 2003;40(8):517–530.530.
- Inoue K, Ishizawa M, Kubota T. Monoclonal anti-dsDNA antibody 2C10 escorts DNA to intracellular DNA sensors in normal mononuclear cells and stimulates secretion of multiple cytokines implicated in lupus pathogenesis. Clin Exp Immunol. 2020;199(2):150–162.
- Lu Q, Kanai Y, Kubota T. The emergence of anti-dsDNA antibodies precedes nucleosome-specific antibodies in MRL/lpr and MRL/+ mice. J Med Dent Sci. 2003;50(1):9–15.
- Ermakov KV, Bukhvostov AA, Vedenkin AS, et al. The unique single-stranded cfDNA species in retinoblastoma patients blood plasma: beyond new HPLC technology. Biomark J. 2019;5(3):1–4.
- Burnham P, Kim MS, Agbor-Enoh S, et al. Single-stranded DNA library preparation uncovers the origin and diversity of ultrashort cell-free DNA in plasma. Sci Rep. 2016;6:27859.
- El Kebir D, Damlaj A, Makhezer N, et al. Toll-like receptor 9 signaling regulates tissue factor and tissue factor pathway inhibitor expression in human endothelial cells and coagulation in mice. Crit Care Med. 2015;43(6):e179–e189.
- Mustelin T, Lood C, Giltiay NV. Sources of pathogenic nucleic acids in systemic lupus erythematosus. Front Immunol. 2019;10:1028.
- Monzavi SM, Alirezaei A, Shariati-Sarabi Z, et al. Efficacy analysis of hydroxychloroquine therapy in systemic lupus erythematosus: a study on disease activity and immunological biomarkers. Inflammopharmacol. 2018;26(5):1175–1182.
- Schreiber K, Breen K, Parmar K, et al. The effect of hydroxychloroquine on haemostasis, complement, inflammation and angiogenesis in patients with antiphospholipid antibodies. Rheumatology (Oxford)). 2018;57(1):120–124.