Abstract
Rheumatoid arthritis is a chronic form of arthritis that causes bone destruction in joints such as the knees and fingers. Over the past two decades, the clinical outcomes of rheumatoid arthritis have improved substantially with the development of biological agents and Janus kinase inhibitors. Osteoclasts are myeloid lineage cells with a unique bone-destroying ability that can lead to joint destruction. On the other hand, osteoclasts play an important role in skeletal homeostasis by supporting bone remodeling together with osteoblasts in the bone marrow under steady-state conditions. However, the same osteoclasts are considered to participate in physiological bone remodeling and joint destruction. We found that pathological osteoclasts have different differentiation pathways and regulatory transcription factors compared to physiological osteoclasts. We also identified arthritis-associated osteoclastogenic macrophages (AtoMs), which are common progenitors of pathological osteoclasts in mice and humans that develop specifically in inflamed synovial tissue. This review presents details of the newly identified AtoMs and the original intravital imaging systems that can visualize synovial tissue and pathological osteoclasts at the pannus–bone interface.
1. Introduction
Rheumatoid arthritis (RA) is a chronic autoimmune disease that causes joint and cartilage destruction as a result of persistent inflammation of the synovial tissue. Osteoclasts are myeloid lineage multinuclear cells that play an important role in skeletal homeostasis by supporting bone remodeling, together with osteoblasts in the bone marrow (BM) under steady-state conditions [Citation1]. In contrast to this physiological role, osteoclasts are also involved in pathological osteolysis in RA. Some reports show that osteoclasts involved in pathological bone destruction are possibly induced by different subsets and differentiation pathways of physiological osteoclasts. For example, monocyte-derived immature dendritic cells enhance trans-differentiation into osteoclasts in the presence of inflammatory cytokines such as TNF-α and IL-1α [Citation2,Citation3], and mouse BM-derived macrophages possibly differentiate into osteoclasts in the presence of TNF-α and M-CSF in the absence of osteoclast differentiation factors (ODF/RANKL–RANK system) [Citation4]. However, the subset of osteoclast precursors and specific cytokines involved in pathological bone destruction in RA remain unknown. The hypertrophied synovium (called the pannus) attaches to the bone surface on the bare area [Citation5,Citation6], which is especially vulnerable to bone destruction. BM-derived monocytes are thought to differentiate into mature osteoclasts in the inflamed synovium [Citation7,Citation8]. However, no detailed analyses of osteoclasts or osteoclast precursors in the synovium have been reported, mainly due to the technical difficulties associated with isolating the miniscule amounts of synovial tissues from the bare areas [Citation9].
In this review, we discuss the origin and characteristics of newly identified arthritis-associated osteoclastogenic macrophages (AtoMs) [Citation10] in synovial tissues. We also introduce the multiphoton intravital imaging systems established in our laboratory to visualize synovial tissues and pathological osteoclasts at the pannus–bone interface [Citation11].
2. Osteoclasts/osteoclast precursors in inflamed synovium are derived from BM
To visualize mature osteoclasts and osteoclast precursors in a living mouse simultaneously, we developed the fluorescent-labeled double transgenic mouse (CX3CR1-EGFP/TRAP-tdTomato mouse) by crossing CX3CR1-enhanced green fluorescent protein (EGFP) knock-in mice with tartrate-resistant acid phosphatase (TRAP)-tdTomato transgenic mice [Citation12–14]. CX3CR1 is a fractalkine (CX3CL1) receptor and a marker of monocyte lineage cells, including osteoclast precursors in the BM. TRAP is a marker of mature osteoclasts.
We used a well-established collagen-induced arthritis (CIA) model and backcrossed the CX3CR1-EGFP/TRAP-tdTomato mouse onto the DBA/1j background to investigate the osteoclast precursor population in the inflamed synovium. We also developed an original protocol to expose the inflamed synovium on the bare area of knee joints by removing the patella, patellar ligaments, and quadriceps femoris muscles using micro-scissors under a stereoscopic microscope [Citation10]. With these methods, synovial tissues were isolated from the inflamed joints, and a cell culture observation system revealed that CX3CR1+ cells gradually express TRAP-tdTomato and fuse with other TRAP+ cells to differentiate into mature osteoclasts.
In BM chimeric mice, synovial osteoclasts and osteoclast precursors are derived exclusively from BM cells. To determine whether these osteoclast precursors enter the synovium via the circulation or invade the pannus–bone interface directly through tiny holes in the bone, we used wild-type and CX3CR1-EGFP/TRAP-tdTomato double transgenic parabiotic mice. As a result, CX3CR1+ osteoclast precursors and TRAP+ mature osteoclasts were detected in the wild-type parabiont, suggesting that these cells are derived from BM and migrate to the pannus via the circulation.
3. Macrophages in the inflamed synovium have high osteoclast differentiation potential
To analyze CX3CR1+ cells within the inflamed synovium in more detail, we performed flow cytometry analysis of the blood, BM, and synovium of CIA model mice 1 week after the onset of arthritis. CX3CR1lo Ly6Chi cells, known as inflammatory monocytes [Citation15], were significantly increased in the blood and BM cells in CIA. In the inflamed synovium, CX3CR1lo Ly6Chi cells were detected along with a CX3CR1hi Ly6Cint population. The parabiosis and BM chimeric models suggested that CX3CR1lo Ly6Chicells in the blood (R1) migrate into the synovium (R2) and differentiate into CX3CR1hi Ly6Cint cells in the synovial microenvironment. Detailed analysis of these populations showed that R3 cells are relatively large and express surface makers of both macrophages and dendritic cells, such as F4/80, I-A/I-E, CD11c, and RANK. Since some cells are in transition between R2 and R3 cell populations, we redefined R2′ cells and R3′ cells based on the level of F4/80 expression. May–Giemsa staining showed that R3′ cells have macrophage morphology, such as abundant foamy cytoplasm and cytoplasmic vacuoles. In comparison, R2′ cells had peripherally located kidney-shaped nuclei, suggesting that, without an additional trigger, differentiation into macrophages is not guaranteed for monocytes after they enter the synovium. Combined stimulation with RANKL and TNF-α proved most efficient for inducing the osteoclastogenesis of R3 cells. These results suggest that R3′ cells are osteoclast precursors in the inflamed synovium; we called these cells AtoMs (). In contrast to the physiological osteoclast precursors in the BM, AtoMs express surface markers related to antigen presentation, such as CD80/86 and I-A/I-E, and may share functional characteristics with osteoclast precursors and dendritic cells. This is reasonable because AtoMs can aggravate inflammation by presenting auto-antigens, which can promote osteoclastogenesis at the pannus–bone interface. Mature osteoclasts in the BM are in close contact with osteoblasts and migrate slowly on the bone surface to renew the bone. On the other hand, mature osteoblasts do not exist at the pannus–bone interface, and pathological osteoclasts remain in the same position to eventually form a complete hole. This mode of osteolysis may be beneficial in pyogenic arthritis as rapid bone resorption is critical for removing the scaffold needed for bacterial proliferation.
4. Foxm1 contributes to the bone destruction caused by AtoMs
We performed RNA sequencing (RNA-Seq) transcriptomic analysis of R1, R2′, and R3′ (AtoMs) cells, and predicted upstream regulators through bioinformatics analysis performed using Ingenuity Pathway Analysis. These analyses showed that Foxm1, a transcription factor that plays a major role in carcinogenesis by inducing the epithelial–mesenchymal transition [Citation16], also has a regulatory role in AtoMs. Because the invasive phenotype of a tumor is analogous to aberrant bone destruction in arthritis, these correlations prompted us to explore its function in arthritis. Articular bone erosion was partially alleviated in the global knock-out mice (Foxm1flx/flxRosa26CreERT2), while the severity of joint swelling was reduced, implying an indirect effect against bone destruction by suppressing inflammation. Furthermore, we used a single-cell RNA-Seq technique (scRNA-Seq) to identify the specific subpopulation that differentiates into pathological osteoclasts in situ. This revealed that about 10% of AtoMs (approximately 1,000 cells per mouse) differentiate into mature osteoclasts at the pannus − bone interface; this small subpopulation selectively expressed Foxm1, demonstrating its role in pathological osteoclastogenesis.
We next investigated the osteoclast precursors in the inflamed synovium of human RA patients. Because Ly6C is not expressed in human cells, we used CX3CR1 and HLA-DR to identify the subset with high osteoclastogenic potential. CX3CR1+ HLA-DRhi CD14+ CD64+ cells exist in the synovial fluid and synovium of RA patients but not in their blood. Mouse AtoMs do not exist in the blood. CX3CR1lo Ly6Chi cells in the blood (R1) migrate into the synovium (R2) and then differentiate into AtoMs within the synovial microenvironment. Therefore, these results are compatible with human experiments showing that human AtoMs do not exist in the peripheral blood. We subdivided the CX3CR1+ HLA-DRhi cells into CX3CR1+ HLA-DRhi CD11c+ CD86− and CX3CR1+ HLA-DRhi CD11c+ CD86+ cells. The latter had higher potential for osteoclast formation, and thiostrepton [Citation17–19], a FoxM1 inhibitor, significantly suppressed osteoclastogenesis by these cells. Although direct evidence supporting the differentiation of AtoMs into mature osteoclasts in RA patients is lacking, future studies focusing on single-cell analysis of human AtoMs via pseudotime trajectory analyses may provide some insight.
5. Intravital imaging of pathological osteoclasts in the inflamed synovium
Because the inflamed synovium is characterized by hypervascularity and a multilayered structure, an excitation laser cannot reach the articular bone surface, which prevents the observation of pathological bone destruction in vivo. Therefore, we established a novel system for intravital imaging of the pannus–bone interface, visualizing pathological osteoclasts in the inflamed synovium using multiphoton microscopy () [Citation11]. We observed the third meta phalangeal joint of the forepaw, which is thin enough to permit penetration of a near-infrared excitation laser from multiphoton microscopy into the pannus–bone interface [Citation20,Citation21]. Under a stereoscopic microscope, we directly observed and exposed the bare area by incising the skin and extensor tendon of the middle digit using micro-scissors. We then observed the exposed bare area of CX3CR1-EGFP/TRAP-tdTomato double transgenic mice with inverted multiphoton microscopy. Bone tissue was visualized using a nonlinear second harmonic generation optical process [Citation22,Citation23]. We observed small resorption pits (diameter = 20–50 µm), and we succeeded in directly visualizing pathological osteolysis caused by osteoclasts at the pannus–bone interface (). Using this imaging system, we found that the biological agent CTLA-4 Ig (abatacept) [Citation24,Citation25] bound to CX3CR1+ macrophages and CD140a (PDGFRa)+ fibroblast-like synoviocytes [Citation7] in the inflamed synovium in vivo.
Figure 2. Intravital imaging of pathological osteoclasts in the inflamed synovium. (A) Schematic diagram of multiphoton intravital imaging of the inflamed synovium. (B) An intravital image of the inflamed synovium of a CX3CR1-EGFP/TRAP-tdTomato mouse. Green: AtoMs; Red: pathological osteoclasts; Blue: second harmonic generation (SHG), including the synovium and bones. Arrowheads show representative resorption pits at the pannus–bone interface. Scale bar: 100 µm.
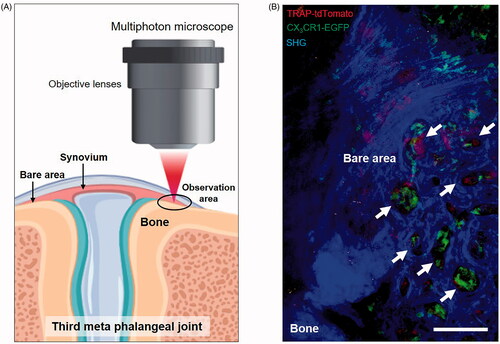
6. Conclusion
The assumption that osteoclasts in the BM and synovial tissue share the same differentiation trajectory is generally accepted. However, we identified pathological osteoclast precursors, called AtoMs, that follow a differentiation trajectory distinct from that of physiological osteoclasts in the BM. This discovery is expected to be a major stepping stone toward elucidating the detailed mechanism of pathological osteolysis, such as bone erosion in RA. Because AtoMs do not exist in other organs under homeostatic conditions, their physiological significance remains unknown. However, articular bone destruction is also induced in pyogenic arthritis, and AtoMs may contribute to biological defense of the synovium against bacterial invasion by removing the bony scaffold necessary for bacterial proliferation. The identification of AtoMs and their differentiation trajectory provides several clues for identifying new targets to suppress joint destruction in RA patients. Because osteoclast precursors in the synovium originate from the circulation, chemokines involved in their ingress into the synovium are potential targets, including CX3CL1 (fractalkine). Indeed, clinical trials of an anti-fractalkine monoclonal antibody demonstrated an effective clinical response in active RA patients [Citation26]. In addition, M-CSF and Foxm1 are possible candidates to target pathological osteoclastogenesis, and drug screening of these inhibitors is underway. Currently, disease-modifying anti-rheumatic drugs such as methotrexate, CTLA-4 Ig (abatacept), TNF inhibitors (infliximab, etanercept), anti-IL-6 receptor antibodies (tocilizumab, sarilumab), and the Janus Kinase inhibitor (targeting JAK-STAT pathway) are used to treat patients with RA. However, one-third of RA patients are still refractory to conventional treatment because every agent has side effects, and the efficacy of the agents differs among patients. Further investigation of AtoMs may lead to the development of fundamental treatments for RA in the near future.
Acknowledgments
We would like to thank BioRender for creating the figures.
Disclosure statement
The authors declare no competing financial interests.
References
- Teitelbaum S, Ross F. Genetic regulation of osteoclast development and function. Nat Rev Genet. 2003;4(8):638–649.
- Rivollier A, Mazzorana M, Tebib J, et al. Immature dendritic cell transdifferentiation into osteoclasts: a novel pathway sustained by the rheumatoid arthritis microenvironment. Blood. 2004;104(13):4029–4037.
- Nomura K, Kuroda S, Yoshikawa H, et al. Inflammatory osteoclastogenesis can be induced by GM-CSF and activated under TNF immunity. Biochem Biophys Res Commun. 2008;367(4):881–887.
- Kobayashi K, Takahashi N, Jimi E, et al. Tumor necrosis factor alpha stimulates osteoclast differentiation by a mechanism independent of the ODF/RANKL-RANK interaction. J Exp Med. 2000;191(2):275–286.
- Schett G, Gravallese E. Bone erosion in rheumatoid arthritis: mechanisms, diagnosis and treatment. Nat Rev Rheumatol. 2012;8(11):656–664.
- McInnes IB, Schett G. The pathogenesis of rheumatoid arthritis. N Engl J Med. 2011;365(23):2205–2219.
- Charles JF, Hsu L-Y, Niemi EC, et al. Inflammatory arthritis increases mouse osteoclast precursors with myeloid suppressor function. J Clin Invest. 2012;122(12):4592–4505.
- Ibáñez L, Abou-Ezzi G, Ciucci T, et al. Inflammatory osteoclasts prime TNFα-producing CD4+ T cells and express CX3 CR1. J Bone Miner Res. 2016;31(10):1899–1808.
- Werner D, Simon D, Englbrecht M, et al. Early changes of the cortical micro-channel system in the bare area of the joints of patients with rheumatoid arthritis. Arthritis Rheumatol. 2017;69(8):1580–1587.
- Hasegawa T, Kikuta J, Sudo T, et al. Identification of a novel arthritis-associated osteoclast precursor macrophage regulated by FoxM1. Nat Immunol. 2019;20(12):1631–1643.
- Hasegawa T, Kikuta J, Sudo T, et al. Development of an intravital imaging system for the synovial tissue reveals the dynamics of CTLA-4 Ig in vivo. Sci Rep. 2020;10(1):13480.
- Ishii M, Egen J, Klauschen F, et al. Sphingosine-1-phosphate mobilizes osteoclast precursors and regulates bone homeostasis. Nature. 2009;458(7237):524–528.
- Ishii M, Kikuta J, Shimazu Y, et al. Chemorepulsion by blood S1P regulates osteoclast precursor mobilization and bone remodeling in vivo. J Exp Med. 2010;207(13):2793–2798.
- Kikuta J, Wada Y, Kowada T, et al. Dynamic visualization of RANKL and Th17-mediated osteoclast function. J Clin Invest. 2013;123(2):866–873.
- Geissmann F, Jung S, Littman DR. Blood monocytes consist of two principal subsets with distinct migratory properties. Immunity. 2003;19(1):71–82.
- Lam EF, Brosens J, Gomes A, et al. Forkhead box proteins: tuning forks for transcriptional harmony. Nat Rev Cancer. 2013;13(7):482–495.
- Hegde N, Sanders D, Rodriguez R, et al. The transcription factor FOXM1 is a cellular target of the natural product thiostrepton. Nat Chem. 2011;3(9):725–731.
- Halasi M, Gartel AL. A novel mode of FoxM1 regulation: positive auto-regulatory loop. Cell Cycle. 2009;8(12):1966–1967.
- Kwok JM-M, Myatt SS, Marson MC, et al. Thiostrepton selectively targets breast cancer cells through inhibition of forkhead box M1 expression. Mol Cancer Ther. 2008;7(7):2022–2032.
- Byrne R, Rath E, Hladik A, et al. A dynamic real time in vivo and static ex vivo analysis of granulomonocytic cell migration in the collagen-induced arthritis model. PLoS One. 2012;7(4):e35194.
- Prendergast CT, Patakas A, Al-Khabouri S, et al. Visualising the interaction of CD4 T cells and DCs in the evolution of inflammatory arthritis. Ann Rheum Dis. 2018;77(4):579–588.
- Houle MA, Couture CA, Bancelin S, et al. Analysis of forward and backward second harmonic generation images to probe the nanoscale structure of collagen within bone and cartilage. J Biophotonics. 2015;8(11–12):993–901.
- Chen X, Nadiarynkh O, Plotnikov S, et al. Second harmonic generation microscopy for quantitative analysis of collagen fibrillar structure. Nat Protoc. 2012;7(4):654–669.
- Linsley PS, Wallace PM, Johnson J, et al. Immunosuppression in vivo by a soluble form of the CTLA-4 T cell activation molecule. Science. 1992;257(5071):792–795.
- Knoerzer DB, Karr RW, Schwartz BD, et al. Collagen-induced arthritis in the BB rat. Prevention of disease by treatment with CTLA-4-Ig. J Clin Invest. 1995;96(2):987–993.
- Tanaka Y, Takeuchi T, Umehara H, et al. Safety, pharmacokinetics, and efficacy of E6011, an antifractalkine monoclonal antibody, in a first-in-patient phase 1/2 study on rheumatoid arthritis. Mod Rheumatol. 2018;28(1):58–65.