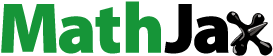
ABSTRACT
This work shows interesting results for a metal–organic complex as a green corrosion inhibitor. The study describes features of two passive films (PF) containing chrysin for mild steel in H2SO4 by means of electrochemical noise under a potentiostaic regime as a function of time. The features showed a current reduction due to the formation of a passive film with better stability and homogeneity when chrysin is absorbed at the optimal experimental potential. PF formed at 50 ppm presented a very high corrosion protection over seven hours reducing the current density seven orders of magnitude, and PF formed at 300 ppm showed two orders of magnitude decrease over 23 h. PF analysis by FT-IR spectra showed important stretch band shift vibrations in the carbonyl and hydroxyl groups as evidence of the presence of metal–organic complex. Moreover, the information provided by adsorption isotherms and quantum chemical calculations confirmed the coordinated bonds established by chemisorption process which correlates with the experimental results.
GRAPHICAL ABSTRACT
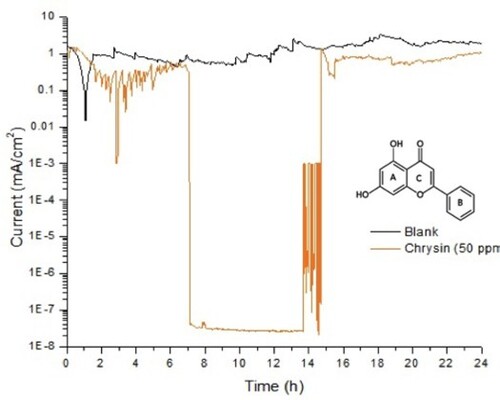
Introduction
The challenge of obtaining new alternatives to prevent corrosion of metallic materials used in industry and avoiding environmental damage to comply with environment protection regulations have motivated researchers to find new alternatives for the development of green corrosion inhibitors, and green anticorrosion coatings from several natural organic compounds [Citation1,Citation2]. As a result, natural sources have demonstrated good inhibition properties [Citation3–5]. Several natural compounds are being isolated to evaluate their anticorrosion properties [Citation6]. Therefore, it has been reported in our previous study that flavonoid chrysin possesses anticorrosion coating properties for carbon steel at a high overpotential in acidic media [Citation7]. Also, it was observed that homogenous oxide films become stable and reduce the pitting attack.
Furthermore, it is known that flavonoids, such as chrysin, are widely studied due to their properties as antioxidant, anti-cancer, antiallergic, antitumor activity, etc. [Citation8]. Chrysin’s structure with a carbonyl group, aromatic rings and hydroxyl groups has demonstrated capability to interact with other molecules by forming hydrogen bonds in order to improve physicochemical properties as solubility, stability, permeability, etc. [Citation9].
Therefore, the purpose of this work is to ascertain the passive film’s behavior as a function of time using electrochemical noise under potentiostatic regime, and the metal-chrysin interactions by FT-IR spectra, adsorption isotherms, and quantum chemical calculations.
Experimental procedure
Testing material and solution
The testing material was 1018 carbon steel (0.14%C, 0.90%Mn, 0.30%P and as balance) as cylindrical probes of 0.6 mm in diameter. They were welded with copper wire and encapsulated in commercial crystal polymeric resin. The 0.28 cm2 exposed surface of each probe was abraded with 600 up to 1200 SiC emery paper and washed with distilled water. A solution of H2SO4 0.5 M was used as a testing corrosive media. The flavonoid chrysin was purchased from Aldrich and used without further purification. It was weighed and diluted in 2 mL of methanol and distilled water to be added to the corrosive solution at the desired concentration (50 and 300 ppm) [Citation10].
Electrochemical techniques
Electrochemical noise measurements (EN) were performed under potentiostatic regime and stirring conditions (100 rpm) at room temperature. Each working electrode was immersed into the corrosive solution in the presence of chrysin and polarized to the desired potential (+216 and −370 mV) with a saturated Ag/AgCl electrode as a reference and a graphite rod as an auxiliary electrode. The measurements were recorded every second over 24-h period using a fully automated potentiostat/galvanostat from ACM Instrument, and an average of values from one minute of recording was plotted.
The Fourier transform infra-red (FT-IR) spectra were recorded in an ALPHA BRUKER spectrometer in the region of 4000–400 cm−1and obtained by scraping the metal surfaces from the working electrodes after they were tested by EN experiments.
Results and discussion
Electrochemical noise (EN)
EN assessments revealed that the corrosion process of bared mild steel in sulfuric acid over 24 h at corrosion potential (−370 mV) presented a current density around 1 mA, and it was carried out by uniform corrosion. This is a typical behavior for this material in an acid solution. shows the corrosion of mild steel during 24-h period where regular small amplitude oscillations were observed, due to hydrogen evolution events along the metal surface. However, current density in the presence of 50 ppm of chrysin showed three important aspects. First, between hours one and seven, it presented several oscillations with an average value of 0.4 mA/cm2 and a high amplitude fluctuation at hour three, possibly for the interaction of chrysin and the passive film as it formed on the metal surface. As a result, in the following seven hours the recorded current value was very low. It decreased approximately seven orders of magnitude, and no oscillations were observed by a smooth shape. This behavior confirms that passive film becomes more stable and therefore, less permeable to the aggressive media. However, at hour 14, several oscillations with very a high amplitude appeared, and the corrosion current variation was 3–7 orders of magnitude less compared to the blank. Finally, in the last nine hours, an average of 0.7 mA/cm2 of current density was registered with a similar shape to uniform corrosion in the blank sample.
Figure 1. Noise in current under potentiostatic regime for carbon stee in 0.5 M H2SO4 containing 50 ppm of chrysin, applying −370 mV (active state potential) during 24-h.
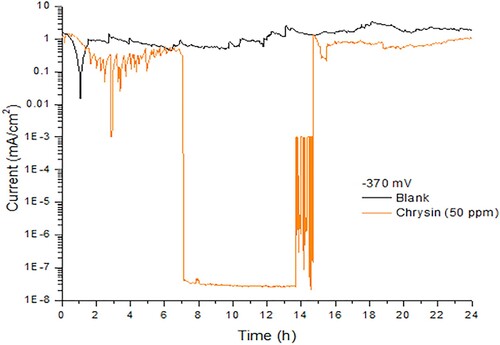
According to our previous report, at a high anodic overpotential (+350 mV) mild steel reacts with hydrogen ions (H+) to form a passive film. In addition, in the presence of chrysin, mild steel’s polarization potential shifted to +216 mV obtaining a lower current density [Citation7]. Therefore, displays the behavior of mild steel immersed in an acid solution and polarized at passivation overpotential +216 mV in the absence and in the presence of 300 ppm of chrysin as a function of the time over 24 h. The evolution of the passive film for the system in the absence of chrysin started at the first hour when the current density value decreases by around two orders of magnitude, reaching an average of 30 mA/cm2. Also, the curve presents a high amplitude transient at 5, 13, 17, 20 and 22 h where the current increased proximately three decades that reflects the breakdown of the oxides layer. On the other hand, the polarized system in the presence of chrysin shows a current decrement at the second hour with an average of 2 mA/cm2 of corrosion current. Short transients with similar size, and two with very high amplitude, returned to the average current value during the 24 h. This behavior confirms that the corrosion inhibition is due to the chrysin’s adsorption which controls the breakdown of the passive layer [Citation11] diminishing the current density two orders of magnitude.
FT-IR spectra
FT-IR spectra, a useful technique to determine functional groups whether organic or inorganic compounds in solid state [Citation12], was used to identify important shifts between chrysin structure and chrysin-mild steel adsorbed layer. After scraping the working electrode surfaces of the inhibition systems in the presence of chrysin, shows the FT-IR spectra of two passive films compared to a pure chrysin sample. Overall, the passive layers’ spectra showed the same typical peaks as pure chrysin’s spectra. That is attributed that no decomposition of chrysin occurred during the EN assessments [Citation13]. However, it is possible to see that there are important band shifts to reveal the presence of a Fe-chrysin complex. For instance, hydroxyl groups of chrysin are observed as a broad band in the region 3100–3600 cm−1, while both groups are absorbed on the passive film, they showed an increment in frequency between 3600 and 2800 cm−1 . Moreover, OH group changes are in accordance with what reported in the previous literature, where some chrysin complexes have been characterized by a broad band in the region 3675–2800 cm−1 [Citation14]. Furthermore, the shift of the O-H vibrational frequency at higher wavenumbers is important evidence that a metal–organic complex has been formed. Because flavonoids such as chrysin present a keto–enol equilibrium between the C = O and O–H groups on the part of the hydrogen atom, when metallic coordination occurs, as in this case, iron takes the place of the hydrogen causing it moves away from the C = O group, and now remaining independent to from part only of the hydroxyl group. Therefore, the characteristics of the stretching vibrations of the OH group change for the new organometallic structure [Citation15].
Figure 3. FT-IR spectra of chrysin and scraped surfaces of two obtained oxides films after EN tests.
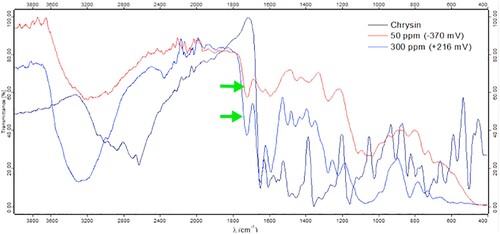
Another important shift was the stretching absorption band for carbonyl group (C = O) that appeared with a high intensity in the region of 1647 cm−1. For the obtained passive layers at corrosion potential and +216 mV, the C = O stretching band was observed with a higher frequency (1722 cm−1) compared to pure chrysin’s band. In addition, it is well known that shifts in C = O adsorption band could be determined by many factors, such physical state, electronic mass effects of neighboring substituents, conjugations, ring strain, etc. [Citation16]. Therefore, this change in the stretching band of the carbonyl group is considered as an evidence of a coordination between iron oxides and chrysin, as it has been observed in other metal complexes [Citation17,Citation18]. The information obtained from FT-IR is in accordance with some flavonoid that have demonstrated to be oxidizable in a range of −200 mV to +600 mV showing metal coordination with Fe(II) ion [Citation19].
summarizes FT-IR peaks for chrysin and the passive films, where is observed the Fe-ion influence on the aromatic ring C because of the interaction. Two complexes formed on the protective layers revealed the same typical bands for aromatic with slightly vibrations band changes. Regarding C–O–C group in a six-membered ring, chrysin shows a characteristic adsorption region in 1354–1310 cm−1, while C–O–C signals for the chrysin complexes moved to the regions 1380–1367 cm−1. Other important change regarding to a vibration band was seen in the O–H group in-plane which is observed in chrysin structure at 1023 cm−1, it was displaced for the complex between 1031–1077 cm−1.
Table 1. Infrared band (cm−1) of chrysin and two different obtained films (−370 mV and +216 mV). b, broad; m, medium; s, small.
Adsorption isotherms
Isotherms provide information about the adsorption process. There are two types of adsorptions, and they allow to classify the interactions between the inhibitor over a surface according to the free energy of adsorption (ΔGads). As suggested in the literature, when the heat of adsorption is low, ΔGads is −20 KJ/mol or less negative. Thus, the adsorption is classified as physisorption. On the other hand, when the heat of adsorption between inhibitor and metal surface shows a ΔGads of −40 KJ/mol or more negative, the process involves charge transfer from the inhibitor to metal, and it produces a coordination type bond, then, the process is classified as chemisorption [Citation20,Citation21].
The fractional coverage surface can be calculated assuming that steel is corroded uniformly. Therefore, it was calculated by current density data from potentiodinamic polarization curves at three different concentration (50, 300 and 500 ppm) of chrysin (that are discussed in our previous paper), for each potential (−370 and +216 mV) according to the following Equation (1):
(1)
(1) where Icorr is the corrosion current density for mild steel without chrysin as a representation of total corrosion sites on the tested surface; I'corr is the current density in the presence of the flavonoid and represents the number of corrosion sites once the flavonoid was adsorbed [Citation22]. In agreement with the surface coverage and isotherms models, shows the best isotherms correlations. For the system in active state (−370 mV), Langmuir isotherm obtained the best fit, while Freundlich isotherm was better suited for the system at a high overpotential (+216 mV). The equilibrium constant of adsorption (Kads) is related to the free energy ΔGads, as seen in Equation (2):
(2)
(2) where 55.5 denotes the concentration of water in the solution [Citation23]; R is the universal constant of gases, and T corresponds to the absolute temperature. It is possible to observe in that a system with chrysin-carbon steel at active state obeyed the Langmuir isotherm, which suggests that chrysin makes a monolayer, where each adsorbed molecules is independent of whether or not nearby sites are occupied, while in the case of mild steel at +216 mV passivation potential obeys the Freundlich model due to the enthalpy changed logarithmically as a consequence of the inhibitor concentration. Therefore, the sites are not equivalent, which means that adsorption can occur in the favored areas with the highest energy [Citation24]. This behavior could be related to the characteristic transformation of different iron oxides and the applied overpotential. In addition, it is well known that the heterogeneous iron passive layer is composed by maghemite and magnetite [Citation12].
Figure 4. Adsorption isotherms for two carbon steel systems: (a) best Langmuir correlation for active state potential (−370 mV), and (b) best Freundlich isotherm correlation for the system at passivation potential (+216 mV).
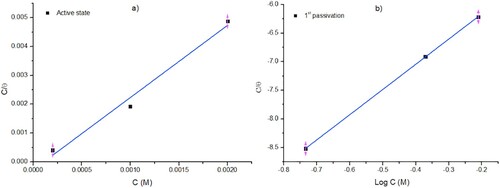
Table 2. Parameters from adsorptions isotherms for the two tested systems.
The isotherm models () showed a good correlation demonstrating correlation coefficients (R2) values close to 1. Furthermore, the large value in the equilibrium constant (Kads) obtained for the system with overpotential (+216 mV) denotes a good inhibition [Citation25]. Free energy values are in the range of −24 to −27 KJ/mol. It suggests that chrysin is adsorbed by both processes, physisorption and chemisorption [Citation26]. Commonly, the physisorption of organic molecules is a faster process than chemisorption that takes place once they replace the adsorbed water on the metal surface. Therefore, physisorption process in this system can be attributed by the displacement of water molecules by electrostatic interaction between chrysin and Fe0 atoms. On the other hand, organic molecules with phenolic groups can reduce Fe(III) to Fe [Citation27], which suggest that chemisorption of chrysin is caused by the existence of Fe(III) ions making a metal complex as shown in the FT-IR analysis.
In addition, physisorption over a surface is influenced by the metal charge and the nature of the aggressive media. The electrical charge of the metal is related to the corrosion potential (Ecorr), and it can be either positive or negative. When the surface is influenced by a negative polarization as in the case of our system at active state at −370 mV, mild steal develops an electrical double layer where the metal surface is negatively charged, and the species from the acid media (H+) are positively charged. In these conditions, chrysin is adsorbed over the double layer by physisorption. On the other hand, chrysin is adsorbed more than other negative specie when mild steel is polarized at positive overpotential (+216 mV) [Citation28]. According to previous studies, chrysin has demonstrated capability to complex metal ions through its oxidizable groups in the ring A [Citation29]. Thus, chrysin can interact with the oxide products being strongly adsorbed.
Quantum chemical calculation
As it is well known, electrostatic interactions are the consequence of electrical charges in the molecules, and many reactions are carried out by electron densities [Citation30]. Therefore, to correlate the obtained result with the structural nature of chrysin, quantum chemical interactions are considered important with the purpose to determine active sites when chrysin in under an acidic media. The charge density distributions were obtained by DFT at the B3LYP/6-31 level of theory. In shows the optimized structures of chrysin and iron-chrysin coordination as suggested in the FT-IR results.
Orbital HOMO and LUMO are often related to molecular reactivity. As it has seen in prelaminar studies of corrosion inhibitors, a high value of EHOMO is related to a good ability to donate electrons on a metal or oxide surface, due to the richness of electrons within the molecule [Citation31]. In is observed that The HOMO energy of chrysin is distributed around rings A and C due to the presence of oxygen atoms in the OH groups, and the carbonyl group as well [Citation32]. Regarding the LUMO orbitals, they are preferably distributed in the ring B, due to the aromatic ring acts as an electron donor to the electronegative groups. Therefore, the existence of an aromatic ring in the chrysin structure, makes the carbonyl and hydroxyl groups more reactive. In relation to the chrysin-Fe complex, is observed a displacement in the HOMO orbitals towards the Fe coordination site because of the electron donation from the electron-rich groups of chrysin, and LUMO orbitals are homogeneously distributed around the entire molecule, while Ring A for chrysin presented less LUMO orbitals. These shifts in LUMO energies could be attributed to the electron donation toward Fe-ion.
Figure 6. The HOMO and LUMO distributions and energies calculated for chrysin and chrysin complex (Fe-chrysin).
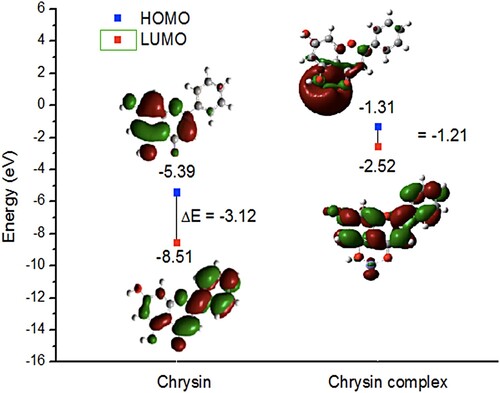
The total dipole moment is used to describe the molecular polarity and is related to the inhibition efficiency. Molecules with a high value of dipole moment are strongly adsorbed over the metal surface [Citation33]. The total dipole moment obtained for chrysin is μ = 5.36 D, where the direction of the negative charge is towards rings A and C influenced by the electronegativity of the oxygen atoms. Therefore, the total dipole moment suggests that carbonyl and hydroxyl groups, electron-rich sites, act as active receptors of Fe ions.
Moreover, an important parameter associated with the resistance to charge transfer is the global hardness (η), chrysin presented a low value of hardness (η = 1.56). Molecules with a low value of hardness are more reactive. That parameter is in accordance with the capability of chrysin to react with active sites over the metal surface. In addition, the number of transferred electrons (ΔN), has become an important tool to obtain information about the reactivity of inhibitors [Citation34,Citation35]. When ΔN < 0, the electron transference between the molecule and metal surface is not carried out. On the contrary when ΔN > 0, the electron transference occurs. ΔN is obtained by the following equation:
(3)
(3) where in the case of iron XFe = 4.28 eV and for chrysin Xchry = 6.95 eV that are the absolute electronegativity. The value of transferred electrons was a positive value of 3.78 eV. According to literature, this value suggests that chrysin possesses the capability to transfer electrons, and confirms metal coordination.
Conclusions
The electrochemical noise results showed that as a consequence of applied potential, chrysin is adsorbed on the oxide passive film allowing more stability and less current rate. Then, the passive film that afforded the highest stability during 24 h-period was the film formed with 300 ppm of chrysin at corrosion potential (−370 mV). However, the system that reached the best efficiency was the film obtained with 50 ppm at high overpotential (+216 mV). The adsorption isotherms confirmed a strong adsorption of chrysin over the passive film.
The FT-IR results and Quantum chemical calculations agreed that under both tested conditions (−370 mV and +216 mV), chrysin can donate electrons through its OH and C = O groups to obtain a Fe-complex making a more stable passive that reduced the corrosion rate. Therefore, at these potentials, chrysin could be a green corrosion inhibitor for mild steel in acid media.
Disclosure statement
No potential conflict of interest was reported by the author(s).
Additional information
Funding
References
- Sørensen PA, Kiil S, Dam-Johansen K, et al. Anticorrosive coatings: a review. J Coatings Technol Res. 2009;6(2):135–176. doi:10.1007/s11998-008-9144-2.
- Chávez Diaz G, Uruchurtu Chavarín J, Valladares-Cisneros MG. Evaluation of curcuma longa as corrosion green inhibitor for 1018 steel in media chloride. ECS Trans. 2021;101(1):213–224. doi:10.1149/10101.0213ecst.
- Díaz-Cardenas MY, Lagunas-Rivera S, Salinas-Bravo VM, et al. Peumus Boldus extract as corrosion inhibitor for carbon steel in 0.5 M sulfuric acid. Green Chem Lett Rev. 2017;10(4):257–268. doi:10.1080/17518253.2017.1369167.
- Negm NA, Kandile NG, Aiad IA, et al. New eco-friendly cationic surfactants: synthesis, characterization and applicability as corrosion inhibitors for carbon steel in 1N HCl. Colloids Surf A Physicochem Eng Asp. 2011;391(1–3):224–233. doi:10.1016/j.colsurfa.2011.09.032.
- Rodríguez Torres A, Valladares Cisneros MG, Uruchurtu Chavarín J, et al. The use of Brugmansia Arborea as a green corrosion inhibitor for AISI 1018 carbon steel in acid media. Green Chem Lett Rev. 2021;14(1):107–117. doi:10.1080/17518253.2020.1862924.
- Shahid M. Corrosion protection with eco-friendly inhibitors. Adv Nat Sci Nanosci Nanotechnol. 2011;2(4). doi:10.1088/20436262/2/4/043001.
- Díaz MY, Valladares MG, Menchaca EC, et al. Electrochemical evaluation of chrysin flavonoid as corrosion inhibitor for mild steel in sulphuric acid solution. Eur J Eng Res Sci. 2020;5(11):1389–1393. doi:10.24018/ejers.2020.5.11.2247.
- Cárdenas M, Marder M, Blank VC, et al. Antitumor activity of some natural flavonoids and synthetic derivatives on various human and murine cancer cell lines. Bioorganic Med Chem. 2006;14(9):2966–2971. doi:10.1016/j.bmc.2005.12.021.
- Chadha R, Bhalla Y, Nandan A, et al. Chrysin cocrystals: characterization and evaluation. J Pharm Biomed Anal 2017;134:361–371. doi:10.1016/j.jpba.2016.10.020.
- Zhou L, Zhang P, Yang G, Lin R, Wang W, Liu T, & Zhang J. Solubility of Chrysin in Ethanol and Water Mixtures. J. Chem. Eng. Data. 2014;59(7):2215–2220. https://doi.org/10.1021/je5001654.
- Kuznetsov YI. Physico-chemical aspects of protection of metals by organic corrosion inhibitors. Prot Met Phys Chem Surfaces. 2015;51(7):1111–1121. doi:10.1134/S2070205115070102.
- Namduri H, Nasrazadani S. Quantitative analysis of iron oxides using Fourier transform infrared spectrophotometry. Corros Sci. 2008;50(9):2493–2497. doi:10.1016/j.corsci.2008.06.034.
- Mondal PK, Mirmehrabi M, Rohani S. Insight into the formation of heteromolecular hydrogen bonds between dasatinib and GRAS molecules. Org Process Res Dev. 2021;25(7):1579–1588. doi:10.1021/acs.oprd.0c00549.
- Pusz J, Wolowiec S. Solid compounds of Ce(III), Pr(III), Nd(III), and Sm(III) ions with chrysin. J Therm Anal Calorim. 2012;110(2):813–821. doi:10.1007/s10973-011-1989-4.
- Yin H, Shi Y. Theoretical investigation on the excited state intramolecular proton transfer in Me2N substituted flavonoid by the time-dependent density functional theory method. Chinese Phys B. 2018;27(5). doi:10.1088/1674-1056/27/5/058201.
- Silverstein RM, Webster FX, Kiemle DJ. Spectrometric identification of organic compounds. Hoboken: NJ: John Wiley & Sons; 2005.
- He F, Hu R, Li R, et al. The complexation mode of metal ions with Langmuir monolayers of nitrogen-containing flavonoid glycoside-based surfactants derived from Rutin. Colloids Surf B Biointer. 2011;84(1):233–240. doi:10.1016/j.colsurfb.2011.01.005.
- Malešev D, Kuntić V. Investigation of metal-flavonoid chelates and the determination of flavonoids via metal-flavonoid complexing reactions. J Serbian Chem Soc. 2007;72(10):921–939. doi:10.2298/JSC0710921M.
- Van Acker SABE, Van Den Berg D, Tromp MNJL, et al. Structural aspects of antioxidant activity of flavonoids. Free Radic Biol Med. 1996;20(3):331–342. doi:10.1016/0891-5849(95)02047-0.
- Tezcan F, Yerlikaya G, Mahmood A, et al. A novel thiophene schiff base as an efficient corrosion inhibitor for mild steel in 1.0 M HCl: electrochemical and quantum chemical studies. J Mol Liq. 2018;269:398–406. doi:10.1016/j.molliq.2018.08.025.
- Muthukrishnan P, Jeyaprabha B, Prakash P. Corrosion inhibition and adsorption behavior of Setaria Verticillata leaf extract in 1M sulphuric acid. J Mater Eng Perform. 2013;22(December):3792–3800. doi:10.1007/s11665-013-0700-2.
- Durnie W, De Marco R, Jefferson A, et al. Development of a structure-activity relationship for oil field corrosion inhibitors. J Electrochem Soc. 1999;146(5):1751–1756. doi:10.1149/1.1391837.
- Zingales A, Quartarone G, Bellomi T, et al. Using indole to inhibit copper corrosion in aerated 0.5 M sulfuric acid. Corrosion. 2010;54(8):606–618. doi:10.5006/1.3287636.
- Do DD. Adsorption analysis: equilibria and kinetics; Series on Chemical Engineering. Vol. 2. Imperial College Press; 1998. doi:10.1142/9781860943829.
- Obi-Egbedi NO, Obot IB. Inhibitive properties, thermodynamic and quantum chemical studies of alloxazine on mild steel corrosion in H2SO4. Corros Sci. 2011;53(1):263–275. doi:10.1016/j.corsci.2010.09.020.
- Baskar R, Gopiraman M, Kesavan D, et al. Synthesis, characterization, and electrochemical studies of novel biphenyl based compounds. Ind Eng Chem Res. 2012;51(10):3966–3974. doi:10.1021/ie201470j.
- Sravanthi K, Ayodhya D, Yadgiri Swamy P. Green synthesis, characterization of biomaterial-supported zero-valent iron nanoparticles for contaminated water treatment. J Anal Sci Technol. 2018;9(1). doi:10.1186/s40543-017-0134-9.
- Harvey TJ, Walsh FC, Nahlé AH. A Review of inhibitors for the corrosion of transition metals in aqueous acids. J Mol Liq. 2018;266:160–175. doi:10.1016/j.molliq.2018.06.014.
- Engelmann MD, Hutcheson R, Cheng IF. Stability of ferric complexes with 3-hydroxyflavone (Flavonol), 5,7-Dihydroxyflavone (Chrysin), and 3′,4′-Dihydroxyflavone. J Agric Food Chem 2005;53(8):2953–2960. doi:10.1021/jf048298q.
- Gece G. The use of quantum chemical methods in corrosion inhibitor studies. Corros Sci. 2008;50(11):2981–2992. doi:10.1016/j.corsci.2008.08.043.
- Obot IB, Macdonald DD, Gasem ZM. Density functional theory (DFT) as a powerful tool for designing new organic corrosion inhibitors. Part 1: an overview. Corros Sci 2015;99:1–30. doi:10.1016/j.corsci.2015.01.037.
- Munõz VA, Ferrari GV, Sancho MI, et al. Spectroscopic and thermodynamic study of chrysin and quercetin complexes with Cu(II). J Chem Eng Data. 2016;61(2):987–995. doi:10.1021/acs.jced.5b00837.
- Al-Amiery AA, Majedy YKA, Kadhum AAH, et al. New Coumarin derivative as an eco-friendly inhibitor of corrosion of mild steel in acid medium. Molecules. 2015;20(1):366–383. doi:10.3390/molecules20010366.
- Issa RM, Awad MK, Atlam FM. Quantum chemical studies on the inhibition of corrosion of copper surface by substituted uracils. Appl Surf Sci. 2008;255(5 PART 1):2433–2441. doi:10.1016/j.apsusc.2008.07.155.
- Khadom AA. Dual function of benzotriazole as copper alloy corrosion inhibitor and hydrochloric acid flow improver. Surf Eng Appl Electrochem. 2014;50(2):157–172. doi:10.3103/s1068375514020069.