ABSTRACT
Cyanobacteria are ubiquitously distributed oxygenic photosynthetic prokaryotes, present even in extreme environments. Many cyanobacteria have been found to be excellent sources of proteins and bioactive compounds. Their cosmopolitan availability, abundant important bioactive compounds and their associated health benefits make cyanobacteria an ideal target for therapeutic applications. Cyanobacteria have a potential role in nutraceutics and are also important in drug development. Several studies have advocated the use of cyanobacteria and their active principles in the treatment of various diseases because cyanobacteria display anti-cancer, antibacterial, anti-immunosuppressive, anti-tumour, neuroprotective, and antiviral activities. Thus, in this review, we have summarized and updated the therapeutic applications of cyanobacteria in drug development, with the introduction of the new term “cyanotherapeutics”.
Introduction
Natural compounds derived from living organisms have traditionally been used as medicine. Our ancestors relied on natural products to fight several diseases. Examples of applications of plant extracts are those of Raulfia serpintina (Apocynaceae) against snakebite (Dey & De, Citation2012; Lobay, Citation2015; Upasani et al., Citation2017), Phyllanthus niruri against liver diseases (Al Zarzour et al., Citation2017; Amin et al., Citation2012; Hong et al., Citation2015) and Centella asiatica for memory improvement (Puttarak et al., Citation2017; Veerendra Kumar & Gupta, Citation2003). Following the emergence of molecular biology and combinatorial chemistry, the use of natural products declined due to the formulation of chemical compounds that mimicked their function. However, the application of ethnobotany in disease cures has continued in the background (Heinrich, Citation2003; Jima & Megersa, Citation2018). Over the past few decades, there has been an increasing interest in drug development from natural compounds and research into the effect of natural compounds on the human body in health and disease (Ji, Li, & Zhang, Citation2009; Singh et al., Citation2017). In recent years several pharmaceutical companies have been working on “nutraceuticals”, the term being a combination of the words “nutrition” and “pharmaceuticals” (Defelice, Citation1989) which can be defined as a food or food product that possesses health benefits and has medicinal properties in the prevention/treatment of disease (Dominguez, Citation2013; Kalra, Citation2003). Cyanobacteria are an important target due to their cosmopolitan availability and their bioactive compounds such as polyunsaturated fatty acids (PUFAs), phycobiliproteins (PBPs), carotenoids, several enzymes and vitamins, with a long history of use (Ciferri & Tiboni, Citation1985; Ghosh et al., Citation2016) due to their beneficial properties (Burja, Banaigs, Abou-Mansour, Burgess, & Wright, Citation2001; Nunnery, Mevers, & Gerwick, Citation2010; Singh, Kate, & Banerjee, Citation2005). Available evidence suggests that cyanobacteria have been used since 1500 BC to treat gout, fistula and forms of cancer (Abarzua, Jakubowski, Eckert, & Fuchs, Citation1999; Bhadury & Wright, Citation2004; Dahms, Ying, & Pfeiffer, Citation2006).
Cyanobacteria are Gram-negative, oxygenic photosynthetic bacteria, diversified in a wide range of habitats ranging from the ice caps of Antarctica to hot springs and rock surfaces (Kulasooriya, Citation2012; Whitton & Potts, Citation2000). They are thought to be one of the most ancient groups of organisms and the ancestor of higher plant plastids (Knoll, Citation2008; Komarek, Kastovsky, Mares, & Johansen, Citation2014; Ughy, Nagy, & Kós, Citation2015). The bioactive compounds available in cyanobacteria possess several health benefits () and have some specific applications such as their use in the formation of medicinal drugs, fuels, cosmetics, food additives and plastics. Cyanobacteria have biochemical pathways that produce unique bioactive molecules with potential commercial and medical applications based on their antifungal, antibiotic, antimicrobial, immunosuppressant, anti-inflammatory, anticancer, antiviral, antibacterial, anticoagulant, antimalarial, antiprotozoal, antituberculosis, anti-HIV (human immunodeficiency virus) and antitumour activities () (Abed, Dobretsov, & Sudesh, Citation2009; Bhadury & Wright, Citation2004; Burja et al., Citation2001; Devillers et al., Citation2007; Jaspars & Lawton, Citation1998; Kajiyama, Kanzaki, Kawazu, & Kobayashi, Citation1998; Koehn, Lomgley, & Reed, Citation1992; Matthew, Salvador, Schupp, Paul, & Luesch, Citation2010; Shimizu, Citation2003). The anti-HIV activity was due to Cyanovirin-N, a carbohydrate binding protein from Nostocellipsosporum (Botos & Wlodawer, Citation2003; Boyd et al., Citation1997).
Table 1. Bioactive compounds obtained from cyanobacteria
Figure 1. Cyanobacteria and their potential applications (Gerwick et al., Citation2001; Jha & Zi-Rong, Citation2004; Senthilkumar & John, Citation2008; Xiong et al., Citation2006)
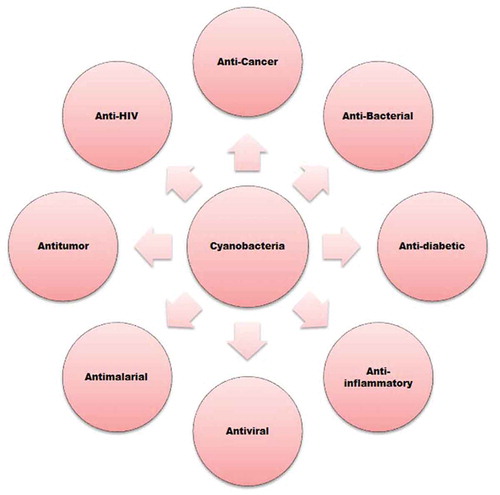
Metabolites such as sulphated polysaccharides, which have antiviral activity, and which have also been reported to have anti-cancer properties (Raposo, de Morais, & Bernardo de Morais, Citation2013), are produced by Arthrospira sp. (Patterson, Larsen, & Moore, Citation1994). Arthrospira sp. also forms gamma-linolenic acid (GLA) that is useful in the control of cholesterol levels, lowering blood pressure and protecting the cardiovascular system (Białek & Rutkowska, Citation2015; Furmaniak et al., Citation2017). Apratoxins (Nostoc sp.), lynbyabellin (Lyngbya majuscula) and curacin (L. majuscula) were successfully used in drug discovery for different cancers (Vijayakumar, Citation2015). These properties result from the availability of carotenoids, chlorophylls, phycocyanins, various amino acids and minerals (Singh et al., Citation2005).
Cyanobacteria are an ideal model system for research on topics ranging from ecophysiology to molecular regulation of cellular events. Cyanobacteria have notable genetic plasticity and variability, making many strains easy to transform (Kühl et al., Citation2012a) (Kühl et al., Citation2012b). Many cyanobacterial genomes have been fully sequenced and are available in the databases (Cyano Clust, http://cyanoclust.c.u-tokyo.ac.jp/), Kazusa (http://www.kazusa.or.jp/), DOE Joint Genome Institute in the USA (http://www.jgi.doe.gov/), CyanoBase, CYORF (http://cyano. genome.jp/), Cyanosite (http://www-cyanosite.bio.purdue edu/). Thus, this review gathers together the information on the application of cyanobacteria for health benefits under the new term “cyanotherapeutics”.
Cyanobacteria as a source of food and nutraceuticals
Some cyanobacterial strains can be eaten directly without performing purification. Cyanobacteria contain 60% protein and are rich in beta-carotene, thiamine, riboflavin and Vitamin B12. Nostoc commune contains fibre and proteins and plays an important role in nutritionally rich human diets (Burja et al., Citation2001; Devillers et al., Citation2007; Jaspars & Lawton, Citation1998).
Cyanobacteria such as Spirulina, Anabaena and Nostoc are commonly used as a food source in countries such as Mexico, Chile, and the Philippines (Abed et al., Citation2009). Arthrospira platensis, commonly known as Spirulina, is one of the most popular cyanobacteria used as a health supplement in the form of powder, tablets or capsules. Spirulina is a rich source of proteins (60%), β-carotene and vitamin B12 (Abed et al., Citation2009). NASA and the European Space Agency have remarked on the health value of Spirulina and stated that Spirulina can serve as a major source of food/food supplements and nutrition (Cornet & Dubertret, Citation1990; Mathur, Citation2018). Several other cyanobacterial species are also used as a food source and as nutraceuticals (Singh, Tiwari, Rai, & Mohapatra, Citation2011). Consumption of cyanobacteria results in several health benefits so they have traditionally been used as a “food for fitness”. Arthospira platensis is a rich source of β-carotene and several other biomolecules of nutraceutical value are popularly regarded as “food for the future” (Desai & Sivakami, Citation2004; Khan, Bhadouria, & Bisen, Citation2005; Mohan, Misra, Srivastav, Umapathy, & Kumar, Citation2014).
The pharmacological properties of cyanobacteria
As discussed above cyanobacteria are a source of several secondary metabolites/bioactive compounds (i.e., PUFAs, PBPs, carotenoids, and various enzymes and vitamins; Ghosh et al., Citation2016) and have great potential to be used as pharmacological agents in various disease conditions (Dunlap et al., Citation2007; Gademann & Portmann, Citation2008; Tan, Citation2007). Properties of bioactive compounds isolated from cyanobacteria include anti-cancer, anti-inflammatory, anti-malarial, anti-bacterial and neuroprotective activity (Abed et al., Citation2009; Bhadury & Wright, Citation2004; Burja et al., Citation2001; Devillers et al., Citation2007; Jaspars & Lawton, Citation1998; Kajiyama et al., Citation1998; Koehn et al., Citation1992; Matthew et al., Citation2010; Shimizu, Citation2003) suggesting a tremendous potential for the pharmacological industry in drug development. Some of these potential applications are discussed in the following sections.
Cyanobacteria as a neuroprotective agent
The photosynthetic accessory PBP pigments of cyanobacteria such as phycocyanin (PC), allophycocyanin (APC) and phycoerythrin (PE) have pharmacological properties such as antioxidant, anti-inflammatory, antitumour/cancer and neuroprotective activities (Bannu et al., Citation2019; Diyana, Plamen, Detelina, Rumen, & MIvanka, Citation2018; Jiang et al., Citation2017). Recently, Arthrospira platensis has been found to have immunostimulant, antiviral, hypocholesterolemic and hypoglycaemic activities and has high antioxidant activity due to c-phycocyanin (C-PC), GLA and phenolic compounds (Abdel-Daim, Abuzead, & Halawa, Citation2013; Kumar, Mella-Herrera, & Golden, Citation2010). C-PC is an active component of Spirulina which shows neuroprotective activity by inhibiting cyclooxygenase 2 (COX-2) and it has been suggested that the apoprotein associated with phycocyanin plays a key role in the inhibition of COX-2 (Reddy et al., Citation2000; Romay, Gonzalez, Ledon, Remirez, & Rimbau, Citation2003). Reactive Oxygen Species (ROS) play a vital role in the induction of neurodegeneration, and phycocyanin (C-PC) and phycocyanobilin show strong antioxidant activity, reduce ROS and help in protection from neurodegenerative conditions (Cervantes-Llanos et al., Citation2018; Pentón-Rol, Marín-Prida, & Falcón-Cama, Citation2018). Furthermore, it has been shown that dietary supplementation of Spirulina protects tyrosine hydroxylase positive neurons in an intra-striatal 6-OHDA lesion model of Parkinson’s disease (Chattopadhyaya et al., Citation2015; Stramberg, Gemma, Vila, & Bickford, Citation2005). The available report demonstrated that microglia play a key role in generating neurodegenerative diseases and supplementation of Spirulina shows a neuroprotective effect by down-regulating MHC II expression in microglia (Pabon et al., Citation2012). Bachstetter et al. (Citation2010) found that Spirulina protected hippocampus neural progenitor cells against lipopolysaccharide (LPS) induced acute systemic inflammation. Patro, Sharma, Kariaya, and Patro (Citation2011) have shown that Arthospira platensis suppressed glia activation and improved motor function in collagen-induced arthritic rats. Furthermore, Arthospira platensis reduces the accumulation of NF200 in the spinal cord neurons of arthritic rats, which inhibits neurodegeneration (Patro et al., Citation2011). Perez-Juarez, Chamorro, Alva-Sanchez, Paniagua-Castro, and Pacheco-Rosado (Citation2016) have shown that administration of kainic acid induces neurodegeneration by causing neuronal damage in the CA3 hippocampal region which is reduced by supplementation of Spirulina. It was also demonstrated that supplementation of Spirulina reduces oxidative stress in the hippocampal region resulting in a neuroprotective effect (Hwang et al., Citation2011; Perez-Juarez et al., Citation2016).
Cyanobacterial LPSs present on the outer cell membrane of cyanobacteria possess immunostimulatory activity (Liu & Bing, Citation2011). Several studies have been conducted to evaluate the biological activity of cynobacterial LPSs (Durai, Batool, & Choi, Citation2015; Ianaro, Tersigni, & D’Acquisto, Citation2009; Liu & Bing, Citation2011; Moosová et al., Citation2019; Stewart, Webb, Schluter, & Shaw, Citation2006). The most characterized cyanobacterial LPS is “cyanobacterial product” (CyP) from Oscillatoria planktothrix FP1 which shows a neuroprotective effect by its antagonist activity to Toll-Like Receptor (TLR)-4 (Gemma, Molteni, & Rossetti, Citation2016; Molteni, Bosi, & Rossetti, Citation2018). A report from our group demonstrated that dietary supplementation by Spirulina improved locomotor activity and increased the lifespan in paraquat (herbicide) sensitive DJ-1βΔ93flies (Parkinson’s disease model in Drosophila) (Kumar, Christian, Panchal, Guruprasad, & Tiwari, Citation2017). Down the line, other studies from our group have also shown the neuroprotective activity of Spirulina using Drosophila models of Alzheimer’s disease (Authors’ unpublished data).
In addition to various biologically active compounds cyanobacteria also produce many toxic compounds (“cyanotoxins”) such as microcystins (from Microcystis, Anabaena, Hapalosiphon, Nostoc, Oscillatoria, and Synechocystis), nodularin (Nodularia), saxitoxins (STXs) (Anabaena, Aphanizomenon, Lyngbya, Cylindrospermopsis), anatoxins-a (Anabaena, Planktothrix, Microcystis), cylindrospermopsin (Cylindrospermopsis) and aplysiatoxins and lyngbyatoxin-a (Lyngbya) (Burja et al., Citation2001; Dias, Paulino, & Pereira, Citation2015; Ferrão-Filho & Kozlowsky-Suzuki, Citation2011; Rastogi, Sonani, & Madamwar, Citation2015; Singh et al., Citation2005; van Apeldoorn, van Egmond, Speijers, & Bakker, Citation2007).
Anti-cancer activity of cyanobacteria
It has been demonstrated that cyanobacteria possess several bioactive derivatives that have the potential to kill cancer cells. Curacin A and cryptophycins isolated from cyanobacteria possess cytotoxic activity through an interaction with microtubule-binding proteins, which arrests cell division and induces apoptosis (Gerwick, Tan, & Sitachitta, Citation2001; Han, Kim, & Kim, Citation2008; Jordan & Wilson, Citation1998; Moore, Corbett, Patterson, & Valeriote, Citation1996). Oftedal et al., (Citation2010) have shown that extracts from Anabaena sp. M27, M30 and M44 result in the induction of cell death in acute myeloid leukaemia (AML) in humans. Cryptophycin isolated from cyanobacteria, such as Nostoc sp. GSV224 (a Nostoc species discovered at the University of Hawaii to be a cryptophycin producer), possess anti-cancer activity against KB human nasopharyngeal cancer cells and LoVo human colorectal cancer cells (Patterson et al., Citation1991; Yasin et al., Citation2019). The cytotoxicity induced by cryptophycins was due to its interaction with microtubule-binding proteins disrupting tubulin dynamics and eventually causing death of tumour cells (Panda, Himes, Moore, Wilson, & Jordan, Citation1997). Cryptophycin acts as a tubulin-destabilizing agent and arrests tumour cells into the G-M phase of the cell cycle, resulting in hyper-phosphorylation of Bcl-2 and apoptotic signalling (Dias et al., Citation2015; Drew, Fine, Do, Douglas, & Petrylak, Citation2002; Smith, Zhang, Mooberry, Patterson, & Moore, Citation1994). However, the Phase II clinical trial of a synthetic analogue “crytophycin 52” failed the initial tests and showed severe toxicity, thus, it was abandoned for further anti-cancer drug development (Edelman et al., Citation2003).
Curacin and dolastatin have been shown to possess cytotoxic activity (Gerwick et al., Citation2001; Jordan & Wilson, Citation1998; Moore et al., Citation1996) and have potential for anti-cancer drug development (Chaganty, Golakoti, Heltzel, Moore, & Yoshida, Citation2004; Moore et al., Citation1996). Curacin-A was first isolated from Lyngbya majuscula (Gerwick, Roberts, Proteau, & Chen, Citation1994) and acts as an inhibitor of cell growth and mitosis activity by interacting with tubulin (Verdier-Pinard et al., Citation1998; Xiong, O’Keefe, Byrd, & McMahon, Citation2006; Yasin et al., Citation2019). Nostoc sp. has been shown to be more potent than currently available drugs for cancer such as taxol and vinblastine (Moore et al., Citation1996). Dolastatin-10, a metabolite isolated from cyanobacteria living in symbiosis with the sea hare Dolabella auricularia (Luesch, Moore, Paul, Mooberry, & Corbett, Citation2001) is a potent anti-proliferative agent, resulting from the presence of four amino acid residues, dolavaline, dolaisoleucine, dolaproline and dolaphenine, which bind with tubulin to arrest the cell into the G2/M phase, and also induce apoptosis (Maki et al., Citation1995). Dolastatin-10 failed the clinical trial (Phase II) due to its low yield but several dolastatin-10 synthetic derivatives have shown potential as an antiproliferative agent. Several studies have suggested that semisynthetic or synthetic derivatives of dolastatin, i.e., auristatin PE (TZT–1027, soblidotin) (Gradishar et al., Citation2012), synthadotin (ILX651; Rafiei & Haddadi, Citation2017) and cematodin (LU–103793; Ercolak, Sahin, Gunaldi, Duman, & Afsar, Citation2015)) possess better pharmacological and pharmacokinetic properties (Lichota, Citation2018; Mita et al., Citation2006; Watanabe, Minami, & Kobayashi, Citation2006). Several other cyanobacterial bioactive compounds have been tested in different human tumour cell lines for their cytotoxic activity. Apratoxin D, an analogue of apratoxin (apratoxin family of cytotoxins isolated from a Lyngbya sp.) from Lyngbya majascula is cytotoxic to human lung cancer cells (Gutierrez et al., Citation2008; Masuda et al., Citation2014; Thornburg et al., Citation2013) and symplocamide A from Symploca sp. possesses cytotoxic activity against lung cancer and neuroblastoma cells (Linington et al., Citation2008; Tan, Citation2010).
Bioactive compounds isolated from cyanobacteria have the ability to induce apoptosis and autophagy in the cancerous cell (Han et al., Citation2008; Kim et al., Citation2012). It was shown that HL-60 (a human leukaemia cell line) cells incubated with an extract from Synechocystis and Synechococcus sp. showed cell shrinkage and apoptosis (Martins et al., Citation2008). Biselyngbyaside (a macrolide glycoside) from Lyngbya sp. and an extract from marine benthic Anabaena sp. have been shown to induce cell death in mature osteoclasts (Teruya, Sasaki, Kitamura, Nakayama, & Suenaga, Citation2009; Yonezawa et al., Citation2012) and in an AML cell line (Oftedal et al., Citation2010).
There are other cyanobacterial bioactive compounds that have the potential to inhibit cancer by arresting the cell cycle, inducing mitochondrial fragmentation, oxidative damage, alteration of apoptotic signalling pathways, modulation of caspase signalling and via sodium channels (). In a study by Gunasekera, Ross, Paul, Matthew, and Luesch (Citation2008), dragonamide C and dragonamide D isolated from Lyngbya polychroa displayed anti-cancerous activity similar to that of dragonamides against leishmaniasis (Balunas et al., Citation2009; Jimenez & Scheuer, Citation2001; McPhail et al., Citation2007). Calothrixin A, an indolophenanthridine from Calothrix sp., induces cell cycle arrest in the G2/M phase in human cancer cell lines (Chen, Smith, & Waring, Citation2003). Dolastatins isolated from cyanobacterial strains can arrest cell cycles (Luesch et al., Citation2001). Hectochlorin and lyngbyabellins, lipopeptides isolated from the genus Lyngbya, can induce arrest in G2/M phase in a human Burkitt lymphoma cell line (Marquez et al., Citation2002).
Figure 2. Anti-cancer activity associated with cyanobacteria (Luesch et al., Citation2001; Xiong et al., Citation2006; Yonezawa et al., Citation2012; Marquez et al., Citation2002; Drew et al., 2002)
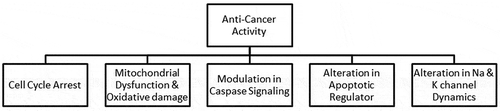
Aurilides A and B isolated from marine cyanobacteria can induce mitochondrial dysfunction (Sato et al., Citation2011). Since mitochondria play a key role in the generation of oxidative stress, mitochondrial fragmentation in cancerous cells will affect the oxidative stress level and may cause oxidative damage to the cancerous cells. There are several cyanobacterial compounds that can alter mitochondrial dynamics and increase oxidative damage to cancerous cells. Calothrixin A has been shown to increase intracellular ROS, which induces oxidative stress and DNA fragmentation in Jurkat human T cells (Chen et al., Citation2003). Cryptophycins 1 and 52 have also been shown to induce caspase-1 and caspase-3 dependent cell death and DNA fragmentation (Drew, Fine, Do, Douglas, & Petrylak, Citation2002; Mooberry, Busquets, & Tien, Citation1997). A study by the National Cancer Institute demonstrated that β-carotene is anticarcinogenic in nature with strong antioxidant properties. Spirulina was used for the treatment of radiation sickness after the Chernobyl disaster in Russia (Loseva & Dardynskaya, Citation1993).
Cyanobacteria and diabetes
Diabetes mellitus is a major threat to the global population, and one of the leading causes of human death after cancer and cardiovascular diseases (Senthilkumar & John, Citation2008). It is a metabolic disorder in which insulin deficiency occurs and blood glucose/blood sugar remains high for a prolonged time (Pandurangan & Kim, Citation2016), ultimately resulting in the onset of hypertension, nephropathy, retinopathy and cardiomyopathy (Senthilkumar & John, Citation2008). There are two types of diabetes, type 1 and type 2. In type 1 diabetes, insulin production is inhibited by the body, while in type 2 diabetes, body cells are not able to respond to insulin resulting in insulin resistance (Lauritano & Ianora, Citation2016; Pandurangan & Kim, Citation2016). The number of diabetic patients is increasing significantly across the globe, which is of immediate concern.
Due to several health-related problems caused by the prolonged use of allopathic medicines, researchers are interested in using traditional medicines to cure/improve diabetic conditions. Thus, several efforts have been taken to identify plants or plant-based components with anti-diabetic properties (Białek & Rutkowska, Citation2015; Kar, Maharana, Pattnaik, & Dash, Citation2006). The possible application of cyanobacteria for anti-diabetic activity has been investigated (Gerwick, Mrozek, Moghaddam, & Agarwal, Citation1989; Layam & Reddy, Citation2006; Pandurangan & Kim, Citation2016; Priatni et al., Citation2016). Some cyanobacterial such as Chroococcus minor, Synechocystis pavalakki, Phormidium corium, Arthrospira platensis, Oscillatoria chalybea, Spirulina labyrinthiformis, and Fischerella sp. show hypoglycaemic effects (Ahmed, Badawi, Mostafa, & Higazy, Citation2018; Senthilkumar & John, Citation2008). Arthrospira platensis, in particular, forms phytochemicals such as flavonoids, phytopigments, and sterols which have shown a strong hypoglycaemic effect, helping to reduce the blood glucose, Serum Glutamic Oxaloacetc Transaminase (SGOT), Serum Glutamic Pyruvic Transaminase (SGPT), Acid phosphatase (ACP) and Alkaline phosphatase (ALP) levels in alloxan-induced diabetic rats, and suggesting that Spirulina has antihyperglycaemic activities (Senthilkumar & John, Citation2008). Further, the supplementation of Spirulina helps in the prevention of complications of type 2 diabetes by lowering blood glucose levels during fasting and post-prandially (after a meal), i.e., haemoglobin A1 c (HbA1 c) level (Parikh, Mani, & Iyer, Citation2001). Additionally, Spirulina also helps in the regulation of lipid profiles in type 2 diabetes (Parikh et al., Citation2001). Pandurangan and Kim (Citation2016) have shown that oral administration of the cyanobacterial extract to streptozotocin diabetic rats for up to 60 days showed reduced blood glucose levels and lipid peroxidation in diabetic rats. They showed that reduction in blood glucose level was due to increased plasma insulin, serum C-peptide and improved glycolytic enzymes resulting from cyanobacterial administration in diabetic rats. Layam and Reddy (Citation2006) showed that supplementation with Spirulina increases weight gain in diabetic rats due to the improved function of plasma insulin, C-peptide and hexokinase activity. Thus, they proposed an antihyperglycaemic effect of Spirulina. Further, an in vitro study by Ghosh et al. (Citation2016) demonstrated the antihyperglycaemic effect of cyanobacterial pigments such as C-phycoerytherin (C-PE), phycocyanin (C-PC), lycopene and myxoxanthophyll. Their study suggested that the antihyperglycaemic effect was due to the inhibition of α-glucosidase by said cyanobacterial pigments.
Cyanobacteria and anti-viral activity
Several studies have suggested that antiviral activity is associated with cyanobacteria (Luescher-Mattli, Citation2003; Nowotny, Mentel, Wegner, Mundt, & Lindequist, Citation1997). Nowotny et al. (Citation1997) demonstrated that a crude extract from Microcystis aeruginosa has antiviral activity against Influenza A virus; bioactive compounds from Lyngbya lagerheimii and Phormidium tenue possess anti-HIV activity (Gustafson et al., Citation1989; Schaeffer & Krylov, Citation2000; Vijayakumar, Citation2015); extract from Spirulina has the potential to inhibit the replication of viruses (o measles and HIV-1; Cohen, Jorgensen, Revsbech, & Poplawski, Citation1986; Jha & Zi-Rong, Citation2004). Chen et al. (Citation2016) have shown anti-viral properties associated with cold water extract of A. platensis. The group demonstrated that viral plaque formation in a broad range of influenza viruses, including oseltamivir-resistant strains, was inhibited by the A. platensis extract. Furthermore, they observed that Spirulina extracts increased the lifespan of influenza-infected mice. The mechanism identified was inhibition of the influenza haemagglutination at an early stage of infection that decreased the virus yields in cells. Patterson et al. (Citation1994) demonstrated that Chroococcales commonly produce antiviral agents. Extracts from 694 strains representing 334 species of cyanobacteria were examined for anti-HIV-1 activity and 529 strains were examined for anti-HSV-2 and anti-RSV activity. Approximately 10% of extracts exhibited antiviral activity against HSV-2 or HIV-1, whereas ~2% showed activity against RSV. They observed that antiviral activity was more commonly associated with lipophilic extracts than with hydrophilic extracts (Patterson et al., Citation1994). A study by Hernandez-Corona, Nieves, Meckes, Chamorro, & Barron (Citation2002) demonstrated the antiviral activity of hot water extract of Spirulina maxima against Herpes simplex virus type 2 (HSV-2). Several cyanobacterial species release acidic polysaccharides, such as calcium spirulan (Ca-SP) from Arthospira platensis, which act as potent inhibitors of herpes simplex virus-1 (Hayashi, Hayashi, & Kojima, Citation1996; Kanekiyo et al., Citation2005), and a fucoidan from the sporophyll of Undaria pinnatifida (Hayashi, Citation2008). Ichthyopeptins A and B, two cyclic depsipeptide compounds released from Microcystis ichthyoblabe, show antiviral activity against influenza A virus (Zainuddin et al., Citation2007). Calcium spirulan (Ca-Sp) prevents several viruses from replicating and exhibits antiviral activity against the human immunodeficiency virus type 1 (HIV-1), HSV-1, influenza virus, human cytomegalovirus (HVMV), mumps virus and measles virus (Barrón, Martín Torres-Valencia, Chamorro-Cevallos, & Zúñiga-Estrada, Citation2007; Hayashi, Citation2008; Ramakrishnan, Citation2013; Simpore et al., Citation2005).
El-Baz, El-Senousy, El-Sayed, and Kamel (Citation2013) have demonstrated that 53.3%, 66.7%, 76.7%, 56.7% and 50% ethanol extract of Arthospira platensis reduces infectious units of adenovirus type 7, Coxsackievirus B4, astrovirus type 1, rotavirus Wa strainand adenovirus type 40, respectively, in vitro. Further, five freshwater cyanobacterial species, Anabaena sphaerica, Chroococcus turgidus, Oscillatoria limnetica, and Arthospira platensis exhibit antiviral activity against adenovirus type 40 (Abdo, Hetta, El-Senousy, Din, & Ali, Citation2012).
Cyanobacteria and immunomodulatory activity
Several studies have suggested immunomodulatory and anti-inflammatory activities associated with Spirulina (Abdel-Daim et al., Citation2013; Finamore, Palmery, Bensehaila, & Peluso, Citation2017; Ou, Lin, Yang, Pan, & Cheng, Citation2013; Wu et al., Citation2016). A study by Abdel-Daim et al. (Citation2013) showed that supplementation of Arthospira platensis powder (500 and 1000 mg kg−1) to mice decreases tumour necrosis factor-alpha (TNF-α) in serum and alters the expression of oxidative stress markers such as nitric oxide (NO), superoxide dismutase (SOD), malondialdehyde (MDA), catalase (CAT), reduced glutathione (GSH) and glutathione peroxidase (GPX) in hepatic, renal and brain tissues (Abdel-Daim et al., Citation2013). Their work has shown the anti-inflammatory and immunomodulatory effect of Spirulina in acetic acid induced ulcerative colitis rats. Finamore et al. (Citation2017) showed that supplementation of Spirulina affects the innate immune response and promotes the activity of natural killer cells thus having anti-hypersensitive, anti-inflammatory and immunostimulating effects (Ou et al., Citation2013). Further, a study by Wu et al. (Citation2016) has suggested that the immunomodulatory and anti-inflammatory response are due to the active components of Spirulina such as phycocyanin and β-carotene and their regulatory effect on ERK1/2, JNK, p38 and IkB signalling pathway.
Bio-economic aspects of the use of cyanobacteria in medicines
There is a continuous increase in the world’s population which may reach nine billion by 2050 (Singh et al., Citation2017). The increase in population with limited natural resources will have major impacts on food and health. Moreover, dependency on agricultural crops in limited land space will be insufficient to meet the requirements of growing populations (Huisman et al., Citation2018; Zahra et al., Citation2020). It requires us to explore cost-effective alternative resources to meet the continuously increasing demand of a global population growing in terms of nutrition and healthcare. Cyanobacteria and algae can help to fulfil the growing demand of increasing populations (Mulkidjanian et al., Citation2006; Pisciotta et al., Citation2010). In this review, we have shown the potential application of Cyanobacteria for human welfare and in medicines. As is known very well, the use of allopathic medicines for most health issues has been of immense value for quick relief of health problems. However, being a symptomatic cure system, allopathic medicines have severe side effects on other cells and eventually on the human body as a whole. It has been reported that continuous and prolonged use of allopathic medicine may affect the immune system and give rise to the development of multidrug resistance (Aslam et al., Citation2018; Karam, Chastre, Wilcox, & Vincent, Citation2016). So, the total cost of the use of allopathic medicine to treat a particular health-related issue remains higher as compared to the involvement of natural therapy or ayurvedic treatment. It can be better explained by citing the prolonged use of Paracetamol to cure a fever that triggers a threat of liver damage (McCrae, Morrison, MacIntyre, Dear, & Webb, Citation2018). Similarly, use of chemotherapy for cancer patients culminates in problems such as excessive hair loss, weaker immune system, improper metabolism, dermatological disorders (Aslam et al., Citation2018; Biswal & Mehta, Citation2018). While the use of ayurvedic/herbal medicine has no known side effects, it takes relatively longer but has great potential to cure several health-related issues. In terms of the bio-economic use of allopathic vs. ayurvedic medicine, the latter is much cheaper in the long run. It is pertinent to mention that among all the medicinal plants currently used for therapeutic purposes, some of the unique characteristics of cyanobacteria such as being the store house of a wide variety of bioactive compounds with a wide range of applications in pharmaceuticals, not requiring much nutrition for their growth and can be grown with little/residual nutrient with high production in terms of lipids (60–65% of dry weight), proteins (33–p50%), carbohydrates, etc. as compared to the agricultural land-based crops with a high cost of cultivation. They have fast-growing potential with no requirement of agricultural land, for cultivation, only a tank containing water and nutrient is sufficient for their luxuriant growth, easy to harvest and transport along with harbouring medically important biomolecules. Also, cyanobacteria have a higher yield per area as compared to agricultural crops and the ability to grow on non-productive and non-arable lands and different water conditions (Zahra et al. 2020). These features make cyanobacteria bio-economically good potential candidates for further exploration towards therapeutics purposes.
Allopathic medicines can be good in the case of any medical emergency such as heart attack, brain haemorrhage and serious accidents. However, for the treatment of chronic cases, allopathic medicines may not be the best choice because use may increase the treatment cost many-fold and worsen the situation over time. Many drugs have been launched onto the market but after years of use, it was noticed that there were serious side effects so they were banned.
Being the factories for several bioactive compounds, cyanobacteria may be a potential target to treat several diseases. There have been many studies investigating the pharmacological properties associated with cyanobacteria and their bioactive components. However, to bring cyanobacteria and their active components into translational mode, detailed in vivo and in vitro studies using different animal models and clinical studies will be essential (El Semary & Fouda, Citation2015; Guihéneuf, Khan, & Tran, Citation2016; Malathi, Ramesh Babu, Mounika, & Digamber Rao, Citation2015; Mukund & Sivasubramanian, Citation2014; Volk & Furkert, Citation2006).
Acknowledgments
The financial assistance from Gujarat Council of Scientific and Technology (GUJCOST/MRP/2015-16/2680), India to AKT is duly acknowledged. The authors are thankful to the Puri Foundation for Education in India for Infrastructure support.
Disclosure statement
The authors declare that there are no conflicts of interest.
References
- Abarzua, S., Jakubowski, S., Eckert, S., & Fuchs, P. (1999). Biotechnological investigation for the prevention of marine biofouling II. Blue-green algae as potential producers of biogenic agents for the growth inhibition of microfouling organisms. Botanica Marina, 42, 459–465.
- Abdel-Daim, M. M., Abuzead, S. M. M., & Halawa, S. M. (2013). Protective role of Spirulina platensis against acute deltamethrin-induced toxicity in rats. PloS One, 8, e72991.
- Abdo, S. M., Hetta, M. H., El-Senousy, W. M., Din, R. A., & Ali, G. H. (2012). Antiviral activity of freshwater algae. Journal of Applied Pharmaceutical Science, 2(2), 21–25.
- Abed, R. M., Dobretsov, S., & Sudesh, K. (2009). Applications of cyanobacteria in biotechnology. Journal of Applied Microbiology, 106, 1–12.
- Ahmed, B. E., Badawi, M. H., Mostafa, S. S., & Higazy, A. M. (2018). Human anticancer and antidiabetic activities of the cyanobacterium Fischerella sp. BS1-EG isolated from River Nile, Egypt. International Journal of Current Microbiology and Applied Sciences, 7, 3473–3485.
- Al Zarzour, R., Ahmad, M., Asmawi, M., Kaur, G., Saeed, M., Al-Mansoub, M., … Yam, M. (2017). Phyllanthus niruri standardized extract alleviates the progression of non-alcoholic fatty liver disease and decreases atherosclerotic risk in Sprague–Dawley Rats. Nutrients, 9, 766.
- Amao, Y., & Nakamura, N. (2006). Biohydrogen production with the light-harvesting function of grana from Spirulina and colloidal platinum. International Journal of Hydrogen Energy, 31, 39–42.
- Amin, Z. A., Bilgen, M., Alshawsh, M. A., Ali, H. M., Hadi, A. H., & Abdulla, M. A. (2012). Protective role of phyllanthus niruri extract against thioacetamide-induced liver cirrhosis in rat model. Evidence-based Complementary and Alternative Medicine: eCAM, 241583. doi:10.1155/2012/241583
- Aslam, B., Wang, W., Arshad, M. I., Khurshid, M., Muzammil, S., Rasool, M. H., … Baloch, Z. (2018). Antibiotic resistance: A rundown of a global crisis. Infection and Drug Resistance, 11, 1645–1658.
- Bachstetter, A. D., Jernberg, J., Schlunk, A., Vila, J. L., Hudson, C., Cole, M. J., … Bickford, P. C. (2010). Spirulina promotes stem cell genesis and protects against LPS induced declines in neural stem cell proliferation. PloS One, 5, e10496.
- Back, S. (2005). Production of cryptophycin from blue green algae. J Young Investig, 12.
- Balunas, M. J., Linington, R. G., Tidgewell, K., Fenner, A. M., Urena, L.-D., Togna, G. D., … Gerwick, W. H. (2009). Dragonamide E, a modified linear lipopeptide from Lyngbya majuscula with antileishmanial activity. Journal of Natural Products, 73, 60–66.
- Banker, R., & Carmeli, S. (1998). Tenuecyclamides A-D, cyclic hexapeptides from the cyanobacterium Nostoc spongiaformae var tenue. Journal of Natural Products, 61, 1248–1251.
- Bannu, S. M., Lomada, D., Gulla, S., Chandrasekhar, T., Reddanna, P., & Reddy, M. C. (2019). Potential Therapeutic Applications of C-Phycocyanin. Current Drug Metabolism, 20, 967–976.
- Barrón, B. L., Martín Torres-Valencia, J., Chamorro-Cevallos, G., & Zúñiga-Estrada, A. (2007). Chapter 11. Spirulina as an antiviral agent. Spirulina in Human Nutrition and Health, 227–242.
- Bhadury, P., & Wright, P. C. (2004). Exploitation of marine algae: Biogenic compounds for potential antifouling applications. Planta, 219, 561–578.
- Bhaskar, V., Law, D. A., Ibsen, E., Breinberg. D., Cass, K. M., DuBridge, R. B., Evangelista, F., Henshall, S. M., Hevezi, P., Miller, J. C., Pong, M., Powers, R., Senter, P., Stockett. D., Sutherland, R. L., von Freeden-Jeffry, U., Willhite, D., Murray, R., Afar, D. E., Ramakrishnan, V. (2003). E-selectin up-regulation allows for targeted drug delivery in prostate cancer. Cancer Res, 63(19),6387–6394.
- Białek, M., & Rutkowska, J. (2015). The importance of γ-linolenic acid in the prevention and treatment. Postepy Higieny I Medycyny Doswiadczalnej (Online), 69, 892–904.
- Biswal, S. G., & Mehta, R. D. (2018). Cutaneous adverse reactions of chemotherapy in cancer patients: A clinicoepidemiological study. Indian Journal of Dermatology, 63, 41–46.
- Botos, I., & Wlodawer, A. (2003). Cyanovirin-N: A sugar-binding antiviral protein with a new twist. Cellular and Molecular Life Sciences CMLS, 60, 277–287.
- Boyd, M. R., Gustafson, K. R., McMahon, J. B., Shoemaker, R. H., O’Keefe, B. R., Mori, T., … Laurencot, C. M. (1997). Discovery of cyanovirin-N, a novel human immunodeficiency virus-inactivating protein that binds viral surface envelope glycoprotein gp120: Potential applications to microbicide development. Antimicrobial Agents and Chemotherapy, 41, 1521–1530.
- Burja, A. M., Banaigs, B., Abou-Mansour, E., Burgess, J. G., & Wright, P. C. (2001). Marine cyanobacteria prolific source of natural products. Tetrahedron, 57, 9347–9377.
- Cardellina, J. H., Marner, F. J., & Moore, R. E. (1979). Seaweed dermatitis: structure of lyngbyatoxin A. Science, 204, 193–195.
- Cardozo, K. H. M., Guaratini, T., Barros, M. P., Falcao, V. D. R., Tonon, A. P., Lopes, N. P., Campos, S., Torres, M. A., Souza, A. O., & Colepicolo, P. (2007). Metabolites from algae with economical impact. Comparative Biochemistry and Physiology Part C: Toxicology & Pharmacology, 146, 60–78.
- Carte, B. K., (1996). Biomedical potential of marine natural products. Bioscience, 46, 271–286.
- Cervantes-Llanos, M., Lagumersindez-Denis, N., Marín-Prida, J., Pavón-Fuentes, N., Falcon-Cama, V., Piniella-Matamoros, B., … Raíces-Cruz, I. (2018). Beneficial effects of oral administration of C-Phycocyanin and Phycocyanobilin in rodent models of experimental autoimmune encephalomyelitis. Life Sciences, 194, 130–138.
- Chaganty, S., Golakoti, T., Heltzel, C., Moore, R. E., & Yoshida, W. Y. (2004). Isolation and structure determination of cryptophycins 38, 326, and 327 from the terrestrial cyanobacterium Nostoc sp. GSV 224. Journal of Natural Products, 67, 1403–1406.
- Chattopadhyaya, I., Gupta, S., Mohammed, A., Mushtaq, N., Chauhan, S., & Ghosh, S. (2015). Neuroprotective effect of Spirulina fusiformis and amantadine in the 6-OHDA induced Parkinsonism in rats. BMC Complementary and Alternative Medicine, 15, 296.
- Chen, X. X. S., Smith, G. D., & Waring, P. (2003). Human cancer cell (Jurkat) killing by the cyanobacterial metabolite calothrixin A. Journal of Applied Phycology, 15, 269–277.
- Chen, Y. H., Chang, G. K., Kuo, S. M., Huang, S. Y., Hu, I. C., Lo, Y. L., & Shih, S. R. (2016). Well-tolerated Spirulina extract inhibits influenza virus replication and reduces virus-induced mortality. Scientific Reports, 6, 24253.
- Ciferri, O., & Tiboni, O. (1985). The biochemistry and industrial potential of Spirulina. Annual Review of Microbiology, 39, 503–526.
- Cohen, Y., Jorgensen, B. B., Revsbech, N. P., & Poplawski, R. (1986). Adaptation to hydrogen sulfide of oxygenic and anoxygenic photosynthesis among cyanobacteria. Applied and Environmental Microbiology, 51, 398–407.
- Cornet, J., & Dubertret, G. 1990. The cyanobacterium Spirulina in the photosynthetic compartment of the MELISSA artificial ecosystem. Workshop on artificial ecological systems, DARA-CNES, Marseille, France, October 24–26.
- Dahms, H.-U., Ying, X., & Pfeiffer, C. (2006). Antifouling potential of cyanobacteria: A mini-review. Biofouling, 22, 317–327.
- Defelice, S. L. (1989). The nutraceutical revolution: Fueling a powerful, new international market. Como, Italy: Harvard University Advanced Management Program in Biomedical Research and Development.
- Desai, K., & Sivakami, S. (2004). Spirulina: The wonder food of the 21st Century. Asia-Pacific Biotech News, 8, 1298–1302.
- Devillers, J., Dore, J. C., Guyot, M., Poroikov, V., Gloriozova, T., Lagunin, A., & Filimonov, D. (2007). Prediction of biological activity profiles of cyanobacterial secondary metabolites. SAR and QSAR in Environmental Research, 18, 629–643.
- Dewi, I. C., Falaise, C., Hellio, C., Bourgougnon, N., & Mouget J. L. (2018). Anticancer, Antiviral, Antibacterial, and Antifungal Properties in Microalgae. Microalgae in Health and Disease Prevention, 235–261.
- Dey, A., & De, J. N. (2012). Traditional use of plants against snakebite in Indian subcontinent: A review of the recent literature. African Journal Of Traditional, Complementary, And Alternative Medicines : Ajtcam / African Networks on Ethnomedicines, 9, 153–174.
- Dias, E., Paulino, S., & Pereira, P. (2015). Cyanotoxins: From poisoning to healing–a possible pathway? Limnetica, 34, 159–172.
- Diyana, B. D. M., Plamen, S., Detelina, B., Rumen, M., & MIvanka, T. (2018). Content of phycoerythrin, phycocyanin, allophycocyanin and phycoerythrocyanin in some cyanobacterial strains: Applications. Engineering in Life Sciences, 18, 861–866.
- Dominguez, H. (2013). Functional ingredients from algae for foods and nutraceuticals. Woodhead Publishing Limited.
- Drew, L., Fine, R. L., Do, T. N., Douglas, G. P., & Petrylak, D. P. (2002). The novel antimicrotubule agent cryptophycin 52 (LY355703) induces apoptosis via multiple pathways in human prostate cancer cells. Clinical Cancer Research, 8, 3922–3932.
- Dunlap, W. C., Battershill, C. N., Liptrot, C. H., Cobb, R. E., Bourne, D. G., Jaspars, M., … Newman, D. J. (2007). Biomedicinals from the phytosymbionts of marine invertebrates: A molecular approach. Methods, 42, 358–376.
- Durai, P., Batool, M., & Choi, S. (2015). Structure and effects of cyanobacterial lipopolysaccharides. Marine Drugs, 13, 4217–4230.
- Edelman, M. J., Gandara, D. R., Hausner, P., Israel, V., Thornton, D., DeSanto, J., & Doyle, L. A. (2003). Phase 2 study of cryptophycin 52 (LY355703) in patients previously treated with platinum based chemotherapy for advanced non-small cell lung cancer. Lung Cancer (Amsterdam, Netherlands), 39, 197–199.
- El Semary, N. A., & Fouda, M. (2015). Anticancer activity of Cyanothece sp. strain extracts from Egypt: First record. Asian Pacific Journal of Tropical Biomedicine, 5, 992–995.
- El-Baz, F. K. E.-S., El-Senousy, W. M., El-Sayed, A. B., & Kamel, M. M. (2013). In vitro antiviral and antimicrobial activities of Spirulina platensis extract. Journal of Applied Pharmaceutical Science, 3, 052–056.
- Ercolak, V., Sahin, B., Gunaldi, M., Duman, B. B., & Afsar, C. U. (2015). Efficacy of paclitaxel in the treatment of Kaposi sarcoma. European Review for Medical and Pharmacological Sciences, 19, 4095–4100.
- Feldman, S. C., Reynaldi, S., Stortz, C. A., Cerezo, A. S., & Damont, E. B. (1999). Antiviral properties of fucoidan fractions from Leathesia difformis. Phytomedicine, 6, 335–340.
- Ferrão-Filho, A., & Kozlowsky-Suzuki, B. (2011). Cyanotoxins: Bioaccumulation and effects on aquatic animals. Marine Drugs, 9, 2729–2772.
- Finamore, A., Palmery, M., Bensehaila, S., & Peluso, I. (2017). Antioxidant, immunomodulating, and microbial-modulating activities of the sustainable and ecofriendly spirulina. Oxidative Medicine and Cellular Longevity, 2017, 1–14.
- Furmaniak, M. A., Misztak, A. E., Franczuk, M. D., Wilmotte, A., Waleron, M., & Waleron, K. F. (2017). Edible cyanobacterial genus Arthrospira: Actual state of the art in cultivation methods, genetics, and application in medicine. Frontiers in Microbiology, 8, 2541.
- Gademann, K., & Portmann, C. (2008). Secondary metabolites from cyanobacteria: Complex structures and powerful bioactivities. Current Organic Chemistry, 12, 326–341.
- Gemma, S., Molteni, M., & Rossetti, C. (2016). Lipopolysaccharides in Cyanobacteria: A brief overview. Advances in Microbiology, 6, 391.
- Gerwick, W., Mrozek, C., Moghaddam, M., & Agarwal, S. (1989). Novel cytotoxic peptides from the tropical marine cyanobacterium Hormothamnion enteromorphoides 1. Discovery, isolation and initial chemical and biological characterization of the hormothamnins from wild and cultured material. Experientia, 45, 115–121.
- Gerwick, W. H., Tan, L. T., & Sitachitta, N. (2001). Nitrogen containing metabolites from marine cyanobacteria. In G. A. Cordell (Ed.), In the alkaloids:. Chemistry of biology (Vol. 57, pp. 75–184). San Diego: Academic Press.
- Gerwick, W. H., Roberts, M. A., Proteau, P. J., & Chen, J. L. (1994). Screening cultured marine microalgae for anticancer-type activity. Journal of Applied Phycology, 6, 143–149.
- Ghosh, T., Bhayani, K., Paliwal, C., Maurya, R., Chokshi, K., Pancha, I., & Mishra, S. (2016). Cyanobacterial pigments as natural anti-hyperglycemic agents: An in vitro study. Frontiers in Marine Science, 3, 146.
- Gradishar, W. J., Krasnojon, D., Cheporov, S., Makhson, A. N., Manikhas, G. M., Clawson, A., … Iglesias, J. (2012). Phase II trial of nab-paclitaxel compared with docetaxel as first-line chemotherapy in patients with metastatic breast cancer: Final analysis of overall survival. Clinical Breast Cancer, 12, 313–321.
- Grinberg, M., Sarig, R., Zaltsman, Y., Frumkin, D., Grammatikakis, N., Reuveny, E., & Gross, A. (2002). tBID Homooligomerizes in the mitochondrial membrane to induce apoptosis. Journal of Biological Chemistry, 277, 12237–12245.
- Guihéneuf, F., Khan, A., & Tran, L.-S. P. (2016). Genetic engineering: A promising tool to engender physiological, biochemical, and molecular stress resilience in green microalgae. Frontiers in Plant Science, 7, 400.
- Gunasekera, S. P., Ross, C., Paul, V. J., Matthew, S., & Luesch, H. (2008). Dragonamides C and D, linear lipopeptides from the marine cyanobacterium brown Lyngbya polychroa. Journal of Natural Products, 71, 887–890.
- Gupta, C., Prakash, D., Garg, A. P., & Gupta, S. (2012). Whey Proteins: A novel source of Bioceuticals. Middle East Journal of Scientific Research, 12, 365–375.
- Gustafson, K. R., Cardellina, J. H., Fuller, R. W., Weislow, O. S., Kiser, R. F., Snader, K. M., … Boyd, M. R. (1989). AIDS-antiviral sulfolipids from cyanobacteria (blue-green algae). JNCI: Journal of the National Cancer Institute, 81, 1254–1258.
- Gutierrez, M., Suyama, T. L., Engene, N., Wingerd, J. S., Matainaho, T., & Gerwick, W. H. (2008). Apratoxin D, a potent cytotoxic cyclodepsipeptide from Papua New Guinea collections of the marine cyanobacteria Lyngbya majuscula and Lyngbya sordida. Journal of Natural Products, 71, 1099–1103.
- Han, S. I., Kim, Y. S., & Kim, T. H. (2008). Role of apoptotic and necrotic cell death under physiologic conditions. BMB Reports, 41, 1–10.
- Hayashi, K., Hayashi, T., & Kojima, I. (1996). A natural sulfated polysaccharide, calcium spirulan, isolated from Spirulina platensis: In vitro and ex vivo evaluation of anti-herpes simplex virus and anti-human immunodeficiency virus activities. AIDS Research and Human Retroviruses, 12, 1463–1471.
- Hayashi, T. (2008). Studies on evaluation of natural products for antiviral effects and their applications. Yakugaku Zasshi: Journal of the Pharmaceutical Society of Japan, 128, 61–79.
- Heinrich, M. (2003). Ethnobotany and natural products: The search for new molecules, new treatments of old diseases or a better understanding of indigenous cultures? Current Topics in Medicinal Chemistry, 3, 141–154.
- Hernandez-Corona, A., Nieves, I., Meckes, M., Chamorro, G., & Barron, B. L. (2002). Antiviral activity of Spirulina maxima against herpes simplex virus type 2. Antiviral Research, 56, 279–285.
- Hong, M., Li, S., Tan, H., Wang, N., Tsao, S.-W., & Feng, Y. (2015). Current status of herbal medicines in chronic liver disease therapy: The biological effects, molecular targets and future prospects. International Journal of Molecular Sciences, 16, 28705–28745.
- Huisman, J., Codd., G. A., Paerl, H. W., Ibelings, B. W., Verspagen, J. M. H., & Visser, P. M. (2018). Cyanobacterial blooms. Nature Reviews Microbiology, 16, 471–483.
- Hwang, J.-H., Lee, I.-T., Jeng, K.-C., Wang, M.-F., Hou, R. C.-W., Wu, S.-M., & Chan, Y.-C. (2011). Spirulina prevents memory dysfunction, reduces oxidative stress damage and augments antioxidant activity in senescence-accelerated mice. Journal of Nutritional Science and Vitaminology, 57, 186–191.
- Ianaro, A., Tersigni, M., & D’Acquisto, F. (2009). New insight in LPS antagonist. Mini Reviews in Medicinal Chemistry, 9, 306–317.
- Jaspars, M., & Lawton, L. A. (1998). Cyanobacteria - a novel source of pharmaceuticals. Current Opinion in Drug Discovery & Development, 1, 77–84.
- Jha, R. K., & Zi-Rong, X. (2004). Biomedical compounds from marine organisms. Marine Drugs, 2, 123–146.
- Ji, H. F., Li, X. J., & Zhang, H. Y. (2009). Natural products and drug discovery. Can thousands of years of ancient medical knowledge lead us to new and powerful drug combinations in the fight against cancer and dementia? EMBO Reports, 10, 194–200.
- Jiang, L., Wang, Y., Yin, Q., Liu, G., Liu, H., Huang, Y., & Li, B. (2017). Phycocyanin: A potential drug for cancer treatment. Journal of Cancer, 8, 3416–3429.
- Jima, T. T., & Megersa, M. (2018). Ethnobotanical study of medicinal plants used to treat human diseases in Berbere district, Bale zone of Oromia regional state, south east Ethiopia. Evidence-Based Complementary and Alternative Medicine, 2018, 1–16.
- Jimenez, J. I., & Scheuer, P. J. (2001). New lipopeptides from the caribbean cyanobacterium lyngbya majuscula. Journal of Natural Products, 64, 200–203.
- Jordan, M. A., & Wilson, L. (1998). Microtubules and actin filaments: Dynamic targets for cancer chemotherapy. Current Opinion in Cell Biology, 10, 123–130.
- Kajiyama, S.-I., Kanzaki, H., Kawazu, K., & Kobayashi, A. (1998). Nostofungicidine, an antifungal lipopeptide from the field-grown terrestrial blue-green alga Nostoc commune. Tetrahedron Letters, 39, 3737–3740.
- Kalra, E. K. (2003). Nutraceutical-definition and introduction. AAPS PharmSci, 5, 27–28.
- Kanekiyo, K., Lee, J. B., Hayashi, K., Takenaka, H., Hayakawa, Y., Endo, S., & Hayashi, T. (2005). Isolation of an antiviral polysaccharide, nostoflan, from a terrestrial cyanobacterium, Nostoc flagelliforme. Journal of Natural Products, 68, 1037–1041.
- Kar, D., Maharana, L., Pattnaik, S., & Dash, G. (2006). Studies on hypoglycaemic activity of Solanum xanthocarpum Schrad. & Wendl. fruit extract in rats. Journal of Ethnopharmacology, 108, 251–256.
- Karam, G., Chastre, J., Wilcox, M. H., & Vincent, J. L. (2016). Antibiotic strategies in the era of multidrug resistance. Critical Care (London, England), 20, 136.
- Khan, Z., Bhadouria, P., & Bisen, P. (2005). Nutritional and therapeutic potential of Spirulina. Current Pharmaceutical Biotechnology, 6, 373–379.
- Kim, M. K., Suh, D. H., Seoung, J., Kim, H. S., Chung, H. H., & Song, Y. S. (2012). Autophagy as a target for anticancer therapy and its modulation by phytochemicals. J Food Drug Anal, 20, 241–245.
- Klebanov, G. I., Kapitanov, A. B., Teselkin, Yu. O., Babenkova, IV., Zhambalova, B. A., Lyubitsky, O. B., Nesterova, O. A., Vasil’eva, O. V., Popov, I. N., Lewin, G., & Vladimirov, Yu. A. (1998). The antioxidant properties of lycopene. Membr Cell Biol, 12, 287–300.
- Klisch, M., & Hader, D. P. (2008). Mycosporine-like amino acids and marine toxins-the common and the different. Mar Drugs, 6, 147–163.
- Knoll, A. H. (2008). Cyanobacteria and earth history. The Cyanobacteria: Molecular Biology, Genomics, and Evolution, 1–20.
- Koehn, F. E., Lomgley, R. E., & Reed, J. K. (1992). Microcolins A and B, new immunosuppressive peptide from the bluegreen algae Lyngbya majuscula. Journal of Natural Products, 55, 613–619.
- Komarek, J., Kastovsky, J., Mares, J., & Johansen, J. R. (2014). Taxonomic classification of cyanoprokaryotes (cyanobacterial genera) 2014, using a polyphasic approach. Preslia, 86, 295–335.
- Kühl, M., Behrendt, L., ECLvET, T., Qvortrup, K., Schreiber, U., Borisov, S. M., … Larkum, A. W. D. (2012a). Microenvironmental ecology of the chlorophyll b-containing symbiotic cyanobacterium Prochloron in the didemnid ascidian Lissoclinum patella. Frontiers in Microbiology, 3, 402.
- Kühl, M., Behrendt, L., Trampe, E. C. L. E., Qvortrup, K., Schreiber, U., Borisov, S. M., … Larkum, A. W. (2012b). Microenvironmental ecology of the chlorophyll b-containing symbiotic cyanobacterium Prochloron in the didemnid ascidian Lissoclinum patella. Frontiers in Microbiology, 3, 402.
- Kulasooriya, S. A. (2012). Cyanobacteria: Pioneers of planet earth. Ceylon Journal of Science (Biological Sciences), 40, 71.
- Kumar, A., Christian, P. K., Panchal, K., Guruprasad, B. R., & Tiwari, A. K. (2017). Supplementation of Spirulina (Arthrospira platensis) Improves Lifespan and Locomotor Activity in Paraquat-Sensitive DJ-1betaDelta93 Flies, a Parkinson’s Disease Model in Drosophila melanogaster. Journal of Dietary Supplements, 14, 573–588.
- Kumar, K., Mella-Herrera, R. A., & Golden, J. W. (2010). Cyanobacterial heterocysts. Cold Spring Harbor Perspectives in Biology, 2, a000315.
- Lauritano, C., & Ianora, A. (2016). Marine organisms with anti-diabetes properties. Marine Drugs, 14, 220.
- Layam, A., & Reddy, C. L. K. (2006). Antidiabetic property of spirulina. Diabetologia Croatica, 35, 29–33.
- Lichota, A. G. K. (2018). Anticancer activity of natural compounds from plant and marine environment. International Journal of Molecular Sciences, 19, 1–38.
- Linington, R. G., Edwards, D. J., Shuman, C. F., McPhail, K. L., Matainaho, T., & Gerwick, W. H. (2008). Symplocamide A, a potent cytotoxin and chymotrypsin inhibitor from the marine Cyanobacterium Symploca sp. Journal of Natural Products, 71, 22–27.
- Liu, M., & Bing, G. (2011). Lipopolysaccharide animal models for Parkinson’s disease. Parkinson’s Disease, 2011, 1–7.
- Lobay, D. (2015). Rauwolfia in the Treatment of Hypertension. Integrative Medicine (Encinitas, Calif.), 14, 40–46.
- Loseva, L. P., & Dardynskaya, I. V. 1993. Spirulina-natural sorbent of radionucleides. Research institute of radiation medicine, Minsk, Belarus. In. 6th Intl Congress of Applied Algology, Czech Republic.
- Luesch, H., Moore, R. E., Paul, V. J., Mooberry, S. L., & Corbett, T. H. (2001). Isolation of dolastatin 10 from the marine cyanobacterium Symploca species VP642 and total stereochemistry and biological evaluation of its analogue symplostatin 1. Journal of Natural Products, 64, 907–910.
- Luescher-Mattli, M. (2003). Algae, a possible source for new drugs in the treatment of HIV and other viral diseases. Current Medicinal Chemistry-Anti-Infective Agents, 2, 219–225.
- Maki, A., Diwakaran, H., Redman, B., Al-Asfar, S., Pettit, G. R., Mohammad, R. M., & Al-Katib, A. (1995). The bcl-2 and p53 oncoproteins can be modulated by bryostatin 1 and dolastatins in human diffuse large cell lymphoma. Anti-cancer Drugs, 6, 392–397.
- Malathi, T., Ramesh Babu, M., Mounika, T., & Digamber Rao, B. (2015). Antimicrobial activity of blue-green algae, Calothrix braunii (A. Br.) Bornet et Flahault. International Journal of Innovative Science, Engineering & Technology, 2, 104–112.
- Marquez, B. L., Watts, K. S., Yokochi, A., Roberts, M. A., Verdier-Pinard, P., Jimenez, J. I., … Gerwick, W. H. (2002). Structure and absolute stereochemistry of hectochlorin, a potent stimulator of actin assembly. Journal of Natural Products, 65, 866–871.
- Martins, R. F., Ramos, M. F., Herfindal, L., Sousa, J. A., Skaerven, K., & Vasconcelos, V. M. (2008). Antimicrobial and cytotoxic assessment of marine cyanobacteria - Synechocystis and Synechococcus. Marine Drugs, 6, 1–11.
- Masuda, Y., Suzuki, J., Onda, Y., Fujino, Y., Yoshida, M., & Doi, T. (2014). Total synthesis and conformational analysis of apratoxin C. The Journal of Organic Chemistry, 79, 8000–8009.
- Mathur, M. (2018). Bioactive molecules of spirulina: A food supplement. Bioactive Molecules in Food, 1–22. doi:10.1007/978-3-319-54528-8_97-1
- Matthew, S., Salvador, L. A., Schupp, P. J., Paul, V. J., & Luesch, H. (2010). Cytotoxic halogenated macrolides and modified peptides from the apratoxin-producing marine cyanobacterium Lyngbya bouillonii from Guam. Journal of Natural Products, 73, 1544–1552.
- McCrae, J. C., Morrison, E. E., MacIntyre, I. M., Dear, J. W., & Webb, D. J. (2018). Long-term adverse effects of paracetamol - a review. British Journal of Clinical Pharmacology, 84, 2218–2230.
- McPhail, K. L., Correa, J., Linington, R. G., Gonzalez, J., Ortega-Barria, E., Capson, T. L., & Gerwick, W. H. (2007). Antimalarial linear lipopeptides from a Panamanian strain of the marine cyanobacterium Lyngbya majuscula. Journal of Natural Products, 70, 984–988.
- Medina, R. A., Goeger, D. E., Hills, P., Mooberry, S. L., Huang, N., Romero, L. I., Ortega-Barria, E., Gerwick, W. H., & McPhail, K. L. (2008). Coibamide A, a potent antiproliferative cyclic depsipeptide from the Panamanian marine cyanobacterium Leptolyngbya sp. J Am Chem Soc, 130, 6324–6325.
- Mita, A. C., Hammond, L. A., Bonate, P. L., Weiss, G., McCreery, H., Syed, S., … Rowinsky, E. K. (2006). Phase I and pharmacokinetic study of tasidotin hydrochloride (ILX651), a third-generation dolastatin-15 analogue, administered weekly for 3 weeks every 28 days in patients with advanced solid tumors. Clinical Cancer Research : An Official Journal of the American Association for Cancer Research, 12, 5207–5215.
- Mohan, A., Misra, N., Srivastav, D., Umapathy, D., & Kumar, S. (2014). Spirulina, the nature’s wonder: A review. Lipids, 5, 7–10.
- Molteni, M., Bosi, A., & Rossetti, C. (2018). Natural products with toll-like receptor 4 antagonist activity. International Journal of Inflammation, 2018. doi:10.1155/2018/2859135
- Mooberry, S. L., Busquets, L., & Tien, G. (1997). Induction of apoptosis by cryptophycin 1, a new antimicrotubule agent. International Journal of Cancer. Journal International Du Cancer, 73, 440–448.
- Moore, R. E., Corbett, T. H., Patterson, G. M. L., & Valeriote, F. A. (1996). The search for new antitumor drugs from blue-green algae. Current Pharmaceutical Design, 2, 317–330.
- Moosová, Z., Šindlerová, L., Ambrůzová, B., Ambrožová, G., Vašíček, O., Velki, M., … Kubala, L. (2019). Lipopolysaccharides from Microcystis cyanobacteria-dominated water bloom and from laboratory cultures trigger human immune innate response. Toxins, 11, 218.
- Mukund, S., & Sivasubramanian, V. (2014). Anticancer activity of oscillatoria terebrieormis cyanobacteria in human lung cancer cell line a549. International Journal of Applied Biology and Pharmaceutical Technology, 5, 34–45.
- Mulkidjanian, A. Y., Koonin, E. V., Makarova, K. S., Mekhedov, S. L., Sorokin, A., Wolf, Y. I., Dufresne, A., Partensky, Fdr., Burd, H., & Kaznadzey, D. (2006). The cyanobacterial genome core and the origin of photosynthesis. Proceedings of the National Academy of Sciences, 103, 13126–13131.
- Natsume, T., Watanabe, J., Koh, Y., Fujio, N., Ohe, Y., Horiuchi, T., Saijo, N., Nishio, K., Kobayashi, M. (2003). Antitumor activity of TZT-1027 (Soblidotin) against vascular endothelial growth factor-secreting human lung cancer in vivo. Cancer Sci, 94, 826–833.
- Nowotny, A., Mentel, R., Wegner, U., Mundt, S., & Lindequist, U. (1997). Antiviral activity of an aqueous extract of the cyanobacterium Microcystis aeruginosa. Phytotherapy Research: An International Journal Devoted to Medical and Scientific Research on Plants and Plant Products, 11, 93–96.
- Nunnery, J. K., Mevers, E., & Gerwick, W. H. (2010). Biologically active secondary metabolites from marine cyanobacteria. Current Opinion in Biotechnology, 21, 787–793.
- Oftedal, L., Selheim, F., Wahlsten, M., Sivonen, K., Doskeland, S. O., & Herfindal, L. (2010). Marine benthic cyanobacteria contain apoptosis-inducing activity synergizing with daunorubicin to kill leukemia cells, but not cardiomyocytes. Marine Drugs, 8, 2659–2672.
- Oren, A., & Gunde-Cimerman, N. (2007). Mycosporines and mycosporine-like amino acids: UV protectants or multipurpose secondary metabolites? FEMS Microbiol Lett, 269, 1–10.
- Ou, Y., Lin, L., Yang, X., Pan, Q., & Cheng, X. (2013). Antidiabetic potential of phycocyanin: Effects on KKAy mice. Pharmaceutical Biology, 51, 539–544.
- Pabon, M. M., Jernberg, J. N., Morganti, J., Contreras, J., Hudson, C. E., Klein, R. L., & Bickford, P. C. (2012). A spirulina-enhanced diet provides neuroprotection in an alpha-synuclein model of Parkinson’s disease. PloS One, 7, e45256.
- Panda, D., Himes, R. H., Moore, R. E., Wilson, L., & Jordan, M. A. (1997). Mechanism of action of the unusually potent microtubule inhibitor cryptophycin 1. Biochemistry, 36, 12948–12953.
- Pandurangan, M., & Kim, D. H. (2016). Therapeutic potential of cyanobacteria against streptozotocin-induced diabetic rats. 3 Biotech, 6, 94.
- Parikh, P., Mani, U., & Iyer, U. (2001). Role of spirulina in the control of glycemia and lipidemia in type 2 diabetes mellitus. Journal of Medicinal Food, 4, 193–199.
- Patro, N., Sharma, A., Kariaya, K., & Patro, I. (2011). Spirulina platensis protects neurons via suppression of glial activation and peripheral sensitization leading to restoration of motor function in collagen-induced arthritic rats. Indian Journal of Experimental Biology, 49, 739–748.
- Patterson, G. M., Baldwin, C. L., Bolis, C. M., Caplan, F. R., Karuso, H., Larsen, L. K., … Tschappat, K. D. (1991). Antineoplastic activity of cultured blue‐green algae (cyanophyta) 1. Journal of Phycology, 27, 530–536.
- Patterson, G. M. L., Larsen, L. K., & Moore, R. E. (1994). Bioactive natural products from blue-green algae. Journal of Applied Phycology, 6, 151–157.
- Pentón-Rol, G., Marín-Prida, J., & Falcón-Cama, V. (2018). C-phycocyanin and phycocyanobilin as remyelination therapies for enhancing recovery in multiple sclerosis and ischemic stroke: A preclinical perspective. Behavioral Sciences, 8, 15.
- Perez-Juarez, A., Chamorro, G., Alva-Sanchez, C., Paniagua-Castro, N., & Pacheco-Rosado, J. (2016). Neuroprotective effect of Arthrospira (Spirulina) platensis against kainic acid-neuronal death. Pharmaceutical Biology, 54, 1408–1412.
- Pisciotta, J. M., Zou, Y., & Baskakov, IV. (2010). Light-dependent electrogenic activity of cyanobacteria. PloS One, 5, 1–10.
- Prasanna, R., Sood, A., Jaiswal, P., Nayak, S., Gupta, V., Chaudhary, V., Joshi, M., & Natarajan, C. (2010). Rediscovering cyanobacteria as valuable sources of bioactive compounds. Prikl Biokhim Mikrobiol, 46, 133–147.
- Priatni, S., Budiwati, T. A., Ratnaningrum, D., Kosasih, W., Andryani, R., Susanti, H., & Susilaningsih, D. (2016). Antidiabetic screening of some Indonesian marine cyanobacteria collection. Biodiversitas Journal of Biological Diversity, 17. doi:10.13057/biodiv/d170236
- Puttarak, P., Dilokthornsakul, P., Saokaew, S., Dhippayom, T., Kongkaew, C., Sruamsiri, R., … Chaiyakunapruk, N. (2017). Effects of Centella asiatica (L.) Urb. on cognitive function and mood related outcomes: A systematic review and meta-analysis. Scientific Reports, 7, 10646.
- Rafiei, P., & Haddadi, A. (2017). Docetaxel-loaded PLGA and PLGA-PEG nanoparticles for intravenous application: Pharmacokinetics and biodistribution profile. International Journal of Nanomedicine, 12, 935.
- Ramakrishnan, R. (2013). Antiviral properties of Cyanobacterium, Spirulina platensis a review. International Journal of Medicine and Pharmaceutical Science, 3, 1–10.
- Raposo, M. F., de Morais, R. M., & Bernardo de Morais, A. M. (2013). Bioactivity and applications of sulphated polysaccharides from marine microalgae. Marine Drugs, 11, 233–252.
- Rastogi, R. P., Sonani, R. R., & Madamwar, D. (2015). Cyanobacterial sunscreen scytonemin: Role in photoprotection and biomedical research. Applied Biochemistry and Biotechnology, 176, 1551–1563.
- Reddy, C. M., Bhat, V. B., Kiranmai, G., Reddy, M. N., Reddanna, P., & Madyastha, K. (2000). Selective inhibition of cyclooxygenase-2 by C-phycocyanin, a biliprotein from Spirulina platensis. Biochemical and Biophysical Research Communications, 277, 599–603.
- Romay, C., Gonzalez, R., Ledon, N., Remirez, D., & Rimbau, V. (2003). C-phycocyanin: A biliprotein with antioxidant, anti-inflammatory and neuroprotective effects. Current Protein and Peptide Science, 4, 207–216.
- Sanchez, L. M., Lopez, D., Vesely, B. A., Togna, G. D., Gerwick, W. H., Kyle, D. E., & Linington, R. G. (2010). Almiramides A-C: discovery and development of a new class of leishmaniasis lead compounds. Journal of Medicinal Chemistry, 53, 4187–4197.
- Sato, S., Murata, A., Orihara, T., Shirakawa, T., Suenaga, K., Kigoshi, H., & Uesugi, M. (2011). Marine natural product aurilide activates the OPA1-mediated apoptosis by binding to prohibitin. Chemistry & Biology, 18, 131–139.
- Schaeffer, D. J., & Krylov, V. S. (2000). Anti-HIV activity of extracts and compounds from algae and cyanobacteria. Ecotoxicology and Environmental Safety, 45, 208–227.
- Senthilkumar, R., & John, S. (2008). Hypoglycaemic activity of marine cyanobacteria in alloxan induced diabetic rats. Pharmacol Online, 2, 704–714.
- Shi, S. R., Cote, R. J., & Taylor, C. R. (1999). Standardization and further development of antigen retrieval immunohistochemistry: Strategies and future goals. Journal of Histotechnology, 22, 177–192.
- Shimizu, Y. (2003). Microalgal metabolites. Current Opinion in Microbiology, 6, 236–243.
- Sielaff, H., Christiansen, G., & Schwecke, T. (2006). Natural products from cyanobacteria: Exploiting a new source for drug discovery. IDrugs, 9, 119–127.
- Singh, S. P., Klisch, M., Sinha, R. P., & Hader, D. P. (2010). Genome mining of mycosporine-like amino acid (MAA) synthesizing and non-synthesizing cyanobacteria: A bioinformatics study. Genomics, 95, 120–128.
- Simpore, J., Zongo, F., Kabore, F., Dansou, D., Bere, A., Nikiema, J. B., … Musumeci, S. (2005). Nutrition rehabilitation of HIV-infected and HIV-negative undernourished children utilizing spirulina. Annals of Nutrition & Metabolism, 49, 373–380.
- Singh, R., Parihar, P., Singh, M., Bajguz, A., Kumar, J., Singh, S., … Prasad, S. M. (2017). Uncovering potential applications of cyanobacteria and algal metabolites in biology, agriculture and medicine: Current status and future prospects. Front Microbiology, 8, 515.
- Singh, R. K., Tiwari, S. P., Rai, A. K., & Mohapatra, T. M. (2011). Cyanobacteria: An emerging source for drug discovery. The Journal of Antibiotics, 64, 401–412.
- Singh, S., Kate, B. N., & Banerjee, U. (2005). Bioactive compounds from cyanobacteria and microalgae: An overview. Critical Reviews in Biotechnology, 25, 73–95.
- Smith, C. D., Zhang, X., Mooberry, S. L., Patterson, G. M. L., & Moore, R. E. (1994). Cryptophycin: A new antimicrotubule agent active against drug-resistant cells. Cancer Research, 54, 3779–3784.
- Stevenson, C. S., Capper, E. A., Roshak, A. K., Marquez, B., Eichman, C., Jackson, J. R., Mattern, M., Gerwick, W. H., Jacobs, R. S., Marshall, L. A. (2002). The identification and characterization of the marine natural product scytonemin as a novel antiproliferative pharmacophore. J Pharmacol Exp Ther, 303, 858–866.
- Stewart, I., Webb, P. M., Schluter, P. J., & Shaw, G. R. (2006). Recreational and occupational field exposure to freshwater cyanobacteria–a review of anecdotal and case reports, epidemiological studies and the challenges for epidemiologic assessment. Environmental Health, 5, 6.
- Stramberg, I., Gemma, C., Vila, J., & Bickford, P. C. (2005). Blueberry-and spirulina-enriched diets enhance striatal dopamine recovery and induce a rapid, transient microglia activation after injury of the rat nigrostriatal dopamine system. Experimental Neurology, 196, 298–307.
- Tan, L. T. (2007). Bioactive natural products from marine cyanobacteria for drug discovery. Phytochemistry, 68, 954–979.
- Tan, L. T. (2010). Filamentous tropical marine cyanobacteria: A rich source of natural products for anticancer drug discovery. Journal of Applied Phycology, 22, 659–676.
- Teruya, T., Sasaki, H., Kitamura, K., Nakayama, T., & Suenaga, K. (2009). Biselyngbyaside, a macrolide glycoside from the marine cyanobacterium Lyngbya sp. Organic Letters, 11, 2421–2424.
- Thornburg, C. C., Cowley, E. S., Sikorska, J., Shaala, L. A., Ishmael, J. E., Youssef, D. T., & McPhail, K. L. (2013). Apratoxin H and apratoxin A sulfoxide from the Red Sea cyanobacterium Moorea producens. Journal of Natural Products, 76, 1781–1788.
- Ughy, B., Nagy, C. I., & Kós, P. B. (2015). Biomedical potential of cyanobacteria and algae. Acta Biologica Szegediensis, 59, 203–224.
- Upasani, S. V., Beldar, V. G., Tatiya, A. U., Upasani, M. S., Surana, S. J., & Patil, D. S. (2017). Ethnomedicinal plants used for snakebite in India: A brief overview. Integrative Medicine Research, 6, 114–130.
- van Apeldoorn, M. E., van Egmond, H. P., Speijers, G. J., & Bakker, G. J. (2007). Toxins of cyanobacteria. Molecular Nutrition & Food Research, 51, 7–60.
- Veerendra Kumar, M. H., & Gupta, Y. K. (2003). Effect of Centella asiatica on cognition and oxidative stress in an intracerebroventricular streptozotocin model of Alzheimer’s disease in rats. Clinical and Experimental Pharmacology & Physiology, 30, 336–342.
- Verdier-Pinard, P., Lai, J.-Y., Yoo, H.-D., Yu, J., Marquez, B., Nagle, D. G., … Gerwick, W. H. (1998). Structure-activity analysis of the interaction of curacin A, the potent colchicine site antimitotic agent, with tubulin and effects of analogs on the growth of MCF-7 breast cancer cells. Molecular Pharmacology, 53, 62–76.
- Vijayakumar, S. M. M. (2015). Pharmaceutical applications of cyanobacteria-A review. Journal of Acute Medicine, 5, 15–23.
- Volk, R.-B., & Furkert, F. H. (2006). Antialgal, antibacterial and antifungal activity of two metabolites produced and excreted by cyanobacteria during growth. Microbiological Research, 161, 180–186.
- Watanabe, J., Minami, M., & Kobayashi, M. (2006). Antitumor activity of TZT-1027 (Soblidotin). Anticancer Research, 26, 1973–1981.
- Whitton, B. A., & Potts, M. (2000). The ecology of cyanobacteria: Their diversity in time and space. Springer Science & Business Media, Springer 2000 edition.
- Wu, Q., Liu, L., Miron, A., Klímová, B., Wan, D., & Kuča, K. (2016). The antioxidant, immunomodulatory, and anti-inflammatory activities of Spirulina: An overview. Archives of Toxicology, 90, 1817–1840.
- Xiong, C., O’Keefe, B. R., Byrd, R. A., & McMahon, J. B. (2006). Potent anti-HIV activity of scytovirin domain 1 peptide. Peptides, 27, 1668–1675.
- Yasin, D., Zafaryab, M., Ansari, S., Ahmad, N., Khan, N. F., Zaki, A., … Fatma, T. (2019). Evaluation of antioxidant and anti-proliferative efficacy of Nostoc muscorum NCCU-442. Biocatalysis and Agricultural Biotechnology, 17, 284–293.
- Yonezawa, T., Mase, N., Sasaki, H., Teruya, T., Hasegawa, S., Cha, B. Y., … Woo, J. T. (2012). Biselyngbyaside, isolated from marine cyanobacteria, inhibits osteoclastogenesis and induces apoptosis in mature osteoclasts. Journal of Cellular Biochemistry, 113, 440–448.
- Zahra, Z., Choo, D. H., Lee, H., & Parveen, A. (2020). Cyanobacteria: Review of Current Potentials and Applications. Environments, 7, 13.
- Zainuddin, E. N., Mentel, R., Wray, V., Jansen, R., Nimtz, M., Lalk, M., & Mundt, S. (2007). Cyclic depsipeptides, ichthyopeptins A and B, from Microcystis ichthyoblabe. Journal of Natural Products, 70, 1084–1088.