ABSTRACT
The CCCryo Culture Collection of Cryophilic Algae is a diverse bioresource of mainly cold-adapted microalgae from polar environments. It currently comprises 518 strains of 178 species in 101 genera. Most strains were isolated from field samples collected during seven polar expeditions, mainly to Svalbard (Norway) and Antarctica. Almost 90% of the strains are cryophilic, with 33% of all strains being psychrophilic (obligate cryophilic) representing the “true” snow algae and 56% being psychrotrophic (non-obligate cryophilic), comprising other snow and permafrost algae from cold habitats. There are also some cultures of mosses, cyanobacteria, fungi and bacteria from cold or thermal environments. These strains are publicly available and serve as a rare bioresource for fundamental studies as well as for the development of commercial products. Basic studies address taxonomic and phylogenetic as well as physiological questions and genome and transcriptome research, whereas applied studies mostly address the capabilities of cryophilic algae to produce commercially interesting products for markets like food, feed and supplements, cosmetics, tools for molecular biology and diagnostics or food processing. Examples of strains studied for the production of valuable polyunsaturated omega-3 fatty acids like EPA, secondary carotenoids like astaxanthin, ice-structuring proteins, cold-active enzymes, UV-absorbing mycosporine-like amino acids and extracellular polymeric substances as form stabilizers are given and discussed in the context of customers’ acceptance, legal regulations for specific markets, technical feasibility of an industrial production process, potential profit and chances for the successful development of a commercial product.
Introduction
Biobanks culturing microalgae and cyanobacteria are distributed worldwide at many research institutions, but only about 20 of the databases or lists of strains are accessible via the internet and offer the strains commercially. Some well-known collections like collections like CCAP (Culture Collection of Algae and Protozoa), NIES (Microbial Culture Collection at the National Institute for Environmental Studies), SAG (Sammlung von Algenkulturen at the University of Göttingen) and UTEX (Culture Collection of Algae at The University of Texas) harbour a broad variety of photosynthetically active organisms from cyanobacteria to micro- and macroalgae, showing their general diversity, whilst others have their focus on a specific group of taxa, e.g. on cyanobacteria at the PCC (Pasteur Culture Collection of Cyanobacteria) or on Zygnematophyceae at the MZCH-SVCK (Microalgae and Zygnematophyceae Collection). Isolates from extreme environments such as hot springs or cold environments usually are not cultured in separate biobanks, but may be available either from the major collections in limited numbers or may be obtained from specialized research collections often maintained by individual researchers at universities, but usually these are not open to the public.
Having been merely a living repository of research objects in earlier times when they were founded, culture collections have developed into collections harbouring a good part of the known algal diversity for fundamental but also applied research. Initially, strains were mostly ordered for academic research at universities and schools. The use of algal and cyanobacterial strains for applied research and the development of industrial products was initially very unusual but has become increasingly important over the last few decades. These days algal collections play an important role in the preservation of algal and cyanobacterial biodiversity and the provision of strains for research, development, and commercial applications, and they (are expected to) function as reliable backup facilities for these valuable bioresources as well.
The CCCryo – how it started and what we are talking about
When we started our research on snow algae, we found that isolates from polar environments were hardly available. In particular, the so-called true snow algae were not listed at the algal collections. Taxonomic knowledge of these species was still scarce and was usually derived from research on field material and some rare isolates maintained at non-public collections. Soon the main reason for this gap in understanding algal diversity became clear – the major culture collections simply did not and still do not have culture cabinets at low temperatures near 0°C so that such isolates can be maintained properly. The CCCryo – Culture Collection of Cryophilic Algae at Potsdam (Germany) fills this gap and offers a safe home to psychrophilic and psychrotolerant algae and cyanobacteria, as well as to mosses and some bacteria and fungi from polar and high alpine environments.
The CCCryo was founded in 1999 by the author of this paper and the supervisor of his doctoral thesis, G.R. Fuhr, both employed at the Humboldt-Universität zu Berlin at that time. With the move of both of them to the Fraunhofer Gesellschaft and the erection of a new institute building in Potsdam, the CCCryo moved first to the Fraunhofer IBMT and then to the Fraunhofer IZI-BB in Potsdam near Berlin where it is still located. The word Cryo for “cryophilic” (= cold-loving) in the biobank’s acronym reflects its specialization. The collection’s first isolates were prepared during one of many scientific expeditions to Spitsbergen (Svalbard). The aim was to obtain living snow algae for our own laboratory experiments, but also to provide these isolates to the scientific community to promote snow algal research. At that time the truly psychrophilic (see below) snow algae were hardly available worldwide. Hence, basic research topics like taxonomy, phylogeny or physiology had scarcely addressed this group of extremophiles, even less had these algae entered the applied research field. Worldwide only a handful of scientists actively worked on cryophilic algae, mainly addressing taxonomic questions. By making strains available from around the turn of the millennium we aimed to move them more into focus for basic and applied research as a novel bioresource. We hypothesized that these algae might harbour some unknown characteristics worth exploration for industrial products.
Psychrophilic vs. psychrotolerant algae
At this point it needs to be clarified what is meant by snow algae. In general, isolates from cold habitats, termed cryophilic, can cope well with temperatures between 0 and 15°C. Depending on their degree of adaptation they can be categorized as psychrophilic (= obligate cryophilic) if they are strongly dependent on low temperatures and die when the temperature increases above a certain threshold (e.g. 8 or 12°C) – this applies to many algae from persistent snow and glacier habitats. If they prefer or can cope well with slightly higher temperatures they are categorized as psychrotolerant (= non-obligate cryophilic) – this applies to most of the algae from permafrost and alpine soils. The minimum temperature (Tmin) and the optimum temperature for growth (Topt) as well as the maximum temperatures (Tmax) above which growth or cell proliferation ceases are the most important parameters to classify the temperature requirements of algae and cyanobacteria from cold habitats. Morita (Citation1975), working on bacteria, provided a good framework and Hoham and Duval (Citation2001) adapted this to algae. It can be summarized (Leya, Citation2013) that if Tmin is around 0°C, Topt ≤ 15°C and Tmax = 15–20°C we can classify the strain as psychrophilic; if Tmin > 0°C and Topt > 15°C we should use the term psychrotolerant. There is a certain overlap between these two categories as strains from both can grow at freezing temperatures around 0°C (in contrast to the mesophilic Chlamydomonas reinhardtii), but optimum and upper temperature limits for growth are lower for psychrophiles compared to psychrotolerants (Russell et al., Citation1990). We can also refer to the habitat and say that snow and glacier algae are often psychrophilic and permafrost algae are instead psychrotolerant.
Materials and methods
The origin of strains, their maintenance, quality control and data organization at CCCryo
The majority of CCCryo strains were isolated from field samples, collected since 1997, originating from the Arctic Archipelago of Svalbard (Norway) and the antarctic King George Island (Antarctica). The author collected most of the samples during seven polar expeditions, and clones from single-cell isolates or picked colonies were mostly established during the cruises as storage of fresh samples is hardly possible for these temperature-sensitive algae. To obtain clonal cultures, field samples from green snow (mass occurrence of actively dividing algae), red snow (mass development of non-proliferating red cysts of Sanguina spp., but with other snow algal species intermingled) and the permafrost (rock, soil, moss, wood etc.) were placed into sterile 15 or 50 ml polyethylene vials and stored on snow in a cool box during the field trip. When back in the field station or on board the sailing yacht (the latter also served as our mobile field station, e.g. when circumnavigating Spitsbergen), fresh field samples were immediately used to isolate single cells using a binocular microscope. Agar plates with different culture media such as BBM, BG11, ESP, TAP, OHH, Spirulina and WEES and pH values between 5 and 9, agarized on 47 mm polystyrene petri dishes, were used for the multiple cell isolation steps [for recipes see CCCryo website at <http://cccryo.fraunhofer.de> or Anderson (Citation2005)]. Clonal strains were established from single-cell isolates or from round, supposedly clonal colonies after multiple streaking out steps on agar plates. These procedures were always carried out outside during the expeditions, as the ambient temperature usually provided the best conditions for this sensitive isolation step, i.e. cold temperatures below 10°C and very clean air. Once on agar plates, the isolates were cultured at ambient conditions during the remaining cruise, checked for growth regularly and, if necessary, protected from too high or freezing temperatures or too much light using snow or ice from the environment and placement in shady locations. Until return to our home lab the isolates were stored in cool boxes, usually used for organ transplants, to ensure a long-lasting chilling below 5°C until they could be stored and cultured safely in cool rooms or commercial fridges with a glass door for external irradiance (). The cultures were irradiated by fluorescent tubes (Osram 77 fluora, cool white, OSRAM Licht AG, Munich, Germany or Philips 840, Philips GmbH Market DACH, Hamburg, Germany) and later, to save energy, changed to LED tubes with a sunlight spectrum (Valoya model L-series, spectrum NS12, Valoya Oy, Helsinki, Finland). Photon fluence rate is maintained at 5–10 µmol m−2 s−1 (measured with a LI-COR LI-250 light metre with a planar quantum PAR sensor).
Figure 1. Stock cultures of CCCryo strains from cold locations are maintained in commercial fridges with glass doors for external irradiance at a temperature of 1–5°C. At the top right a small heated box for thermophilic cyanobacteria and red algae cultured at 35°C is visible, next to it are agar slants and culture flasks for isolates that prefer room temperature for growth.
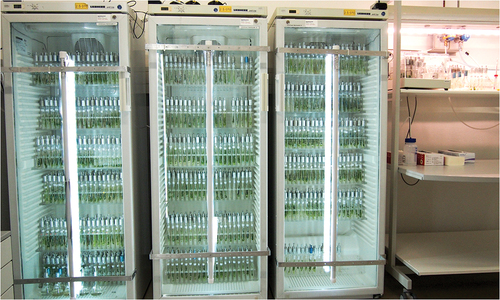
Culture temperature for all strains originating from cold locations was maintained at between 1 and 5°C to prevent any genetic drift by unintentionally selecting cells becoming tolerant to higher temperatures. This was a precautionary measure in favour of psychrophilic strains, disregarding the optimum temperature for growth of some strains, which may be higher. Only strains that proved to grow poorly at these low temperatures were cultured in fridges at 12–15°C. The agar plates carrying the original isolates were checked regularly for growth and once growth was stable, individual isolates/colonies were transferred to agar slants. At that point each strain was registered in a database, receiving a unique CCCryo strain number. All relevant information was stored, such as taxonomic designation, geographic origin, locality, collector’s name and date, isolator’s name and date, culture medium and pH, microscopic photos and many more details. All strains are numbered consecutively with the first 3 digits as the unique strain identifier and the 2-digit number after the hyphen indicating the year in which the strains entered the CCCryo biobank. A letter after the 3-digit number indicates that the strain was reisolated from a single cell a second time, so the genetic data may differ from the original isolate. Unculturable field samples of red or black snow carry a code with the prefix RS (red snow) or BS (black snow) followed by a 4-digit number as the unique sample identifier and ending with a 4-digit number as the year, in which the sample was collected. All data in the database are continuously updated – especially regarding taxonomy – and expanded with information on phylogenetic marker genes, growth characteristics, preferences for different culture media and temperatures, and metabolites produced, with the aim of providing information for potential commercial use of suitable strains. As far as the taxonomic classification above genus level is concerned, we follow the system of Guiry and Guiry (Citation2018) or, if necessary, the online taxonomy resources of the National Centre for Biotechnology Information NCBI (https://www.ncbi.nlm.nih.gov/). Our internal CCCryo database consists of one main table with each strain’s main data and 18 tables carrying further information on locations, metabolites, genetic data etc. making up the complete relational database. Apart from the algae and cyanobacteria isolated from cold habitats, some bacteria, fungi and mosses from polar locations are also cultured. Mesophilic and some thermophilic algae and cyanobacteria complement the CCCryo biobank.
The database also serves as the coordinating interface for curating the CCCryo biobank. Depending on their growth rates, strains must be maintained alive by serial transfer every 1–12 months using around 20 different culture media. Each month a list of 100–160 strains is generated, which need to be transferred to fresh agar slants. Before transfer each agar slant is inspected with a binocular microscope to detect contamination independently from the regular bacterial testing. That takes place at least every 2 years using nutrient-rich liquid media (ES-Fleisch, a basal medium with soil extract and 0.1% beef extract or BBM supplemented with 1.5% glucose, 2.0% peptone and 0.25% yeast extract) suitable for promoting growth of a wide variety of fungal and bacterial contaminants. This procedure ensures the high quality of the CCCryo strains. If strains became contaminated they are flagged “to be cleaned” or “under supervision” in the database and will be reisolated or cleaned by single cell isolation, multiple streaking, or pipette spraying. To promote growth, the freshly inoculated agar slants are initially placed in a culture fridge with a slightly higher irradiance (10–20 µmol m−2 s−1) for about 1–2 weeks and then they are transferred to their regular culture fridge whilst the oldest agar slants of the strains are disposed of. The CCCryo as a living bioresource carries four live generations of each strain for safety reasons (contamination or sudden death of a culture on a single agar slant). Some strains are also cryopreserved in either 5% v/v DMSO (dimethyl sulphoxide) or 5% v/v methanol. We found, however, that many psychrophilic strains of the genera Chlamydomonas and Chloromonas do not survive either the cryopreservation protocols or cryoprotective agents, and so we do not rely on this method to preserve this valuable bioresource.
Diversity of CCCryo strains
A list of all species together with temperature requirements or preferences is presented in . For many of these species multiple strains from different locations exist. Several of these species also exist as axenic or xenic strains. Further details on the origin of the single strains, sequence data etc. can be obtained from our external online database at <http://cccryo.fraunhofer.de>. Some of the species listed in or selected strains thereof, however, may not be found online. These are listed in our internal database only, as they are used for industrial applications or are authentic strains of new species for which descriptions have not yet been published. Several species listed in carry the abbreviation “cf.” (= confer, Latin: to discuss, to debate) in front of their genus name or species epithet, as their taxonomy so far is poorly resolved and their correct identity needs to be discussed. The species names listed are the best identifications possible according to current algal taxonomy. New species or genera have been, and will continue to be, described for several of these unusual species from cold habitats.
Table 1. List of all species cultured at CCCryo and their classification according to their temperature demands (temp.): pp = psychrophilic, pt = psychrotolerant, me = mesophilic, th = thermophilic, n.d. = not determined. For completeness of typical snow and glacier taxa the species of Chlamydomonas nivalis, Sanguina aurantia, S. nivaloides, Ancylonema nordenskiöldii and Mesotaenium berggrenii are listed as well. Of these taxa only field samples and/or cyst stages are stored at CCCryo as frozen archives, however, these cannot be ordered. The correct identity for some of the cryophilic taxa could not be determined finally and these carry “cf.” (= confer) in front of their genus name or species epithet and need to be studied in further, detailed phylogenetic analyses. Those unspecified listings carry a number or other code after the “sp.” and could only be described to genus level so far, however, different morphospecies had been identified and this is reflected by their unique species suffix. Classification according to Guiry (Citation2018).
The CCCryo also hosts some strains as duplicates (= same isolate) of other culture collections (ASIB, ASW, CCAP, CCALA, MZCH-SVCK, SAG, UTEX). Often those strains are cryophilic, but some are also mesophilic or thermophilic. They are maintained for comparative studies, but also to secure these rare bioresources. Furthermore, we maintain many species as multiple strains (= strain of the same species, but from different samples or locations). They are used to study genotypes, e.g. with the aim of identifying a strain of a particular species that is best adapted for a specific application. Taxonomic, strain, genetic and geographic distribution data from the CCCryo are used by external databases such as Global Biodiversity Information Facility (GBIF). CCCryo is registered at WFCC (World Federation of Culture Collections) as WDCM 940.
Nagoya protocol and “rights on the strains”
We observe regulations according to the Nagoya Protocol of Access and Benefit Sharing and follow the recommendations stated in the MIRRI manual (Verkley, Martin, & Smith, Citation2016). For those strains collected and isolated by us, we have full rights to use that bioresource as far as regulations according to the Nagoya Protocol are respected. Having unrestricted rights on most of the CCCryo strains helps and for industry it is often indispensable when intending to develop commercial products.
Biannual meetings of snow algal researchers at SAM
Over the last 20 years an increasing number of young scientists have started to study snow algae from very different perspectives, and because this specific research community still is small and poorly connected, we decided to organize a regular meeting to bring together the various expertise and to facilitate and initiate personal contacts. In 2016 we organized the first Snow Algal Meeting (SAM) together with L.G. Benning and S. Lutz (GFZ, Potsdam, Germany) and D. Remias (FH-Wels, Austria), and now this is run every second year.
Results and discussion
The scope of the CCCryo
Initially the alga responsible for the mass phenomenon of Red Snow was the focus – Chlamydomonas nivalis [recently transferred to Sanguina nivaloides (Procházková, Leya, Křížková, & Nedbalová, Citation2019), see remarks below]. Soon it became clear that the astaxanthin-rich red cysts that make up Red Snow cannot be cultured as they do not germinate in the lab and do not result in a growing isolate (see remarks further below). Luckily, we also always found several spots of the rather rare green snow (actively dividing algal taxa), which were the sources of our first isolates and so our primary focus at CCCryo was broadened out from one species to cryophilic microalgae from snow and glacier habitats in general. This was a good decision, as soon it became clear that the diversity of snow algae is much wider than expected, and we realized that such a diverse bioresource of cryophilic algae did not exist anywhere so far and it would be worth making it available for research. shows that most of the strains belong to the algal classes Chlorophyceae and Trebouxiophyceae. These make up almost 400 strains. In this context it must be stated that we culture many species in multiple strains from different locations, which explains that the CCCryo represents “only” 178 species in 101 genera whilst the total number of strains currently amounts to 518 isolates. The 256 strains of Chlorophyceae are mainly represented by the genera Chlamydomonas and Chloromonas, various species of which are typically found on the snow. Some of the species are extremely psychrophilic, but some rather psychrotolerant species seem to be able to cope with the harsh conditions of persistently cold snow and ice habitats. The various Raphidonema and Koliella spp. from the Trebouxiophyceae, which can be found on virtually every snow field around our planet, are typical species occurring on both snow or glacier fields and the surrounding permafrost soil. They inhabit both these habitats successfully as they prefer temperatures of 4–12°C; however, they grow in rather shady areas compared to Chloromonas and Chlamydomonas spp., which often build up massive green blooms in unshaded areas on snow fields. Raphidonema together with Stichococcus and Chlorella and Chlorella-like species make up most of the cryophilic Trebouxiophyceae. With 142 isolates this is the second largest algal class at the CCCryo. The 32 strains of Zygnematophyceae are mainly represented by different Zygnema spp. from permafrost. The other algal classes have poor representation in cold habitats. Among the Xanthophyceae we mainly find soil- and rock-inhabiting Heterococcus spp. Some as yet undescribed species of pennate diatoms can form large populations as brown snow and rarely Chrysophyceae can also be isolated from fast flowing rivulets crossing glaciers. Mosses were isolated from permafrost as they form the major plant biomass on polar permafrost and they grow easily in our growth cabinets. Occasionally fungi (Leotiomycetes) and bacteria were isolated from contaminated snow algal strains as these might be interesting for studies on species interactions and food and nutrient webs in cold habitats. The other classes contain strains from habitats other than snow and ice. In particular, many of the Cyanophyceae are represented by isolates from thermal habitats such as hot springs. However, as these are extremophiles, they also found a safe harbour at the CCCryo.
Colleagues still ask: Where are the strains of Chlamydomonas nivalis? Why can living strains of this well-known alga, the cause of red snow, a phenomenon known since CitationAristotle (350 BC, translated by D’Arcy Wentworth Thompson) not be ordered from the CCCryo? Indeed, this Red Snow is abundant in polar snow habitats but is only found in its cyst stage (= resting stages) world-wide. To my knowledge there exists no actively growing strain of this taxon. Some isolates carrying the name of Chlamydomonas nivalis were and still are maintained in various collections, but in many cases Ettl (Citation1988; U. Schlösser, pers. comm.) reidentified such strains as other species of Chlamydomonas or Chloromonas. Presumably, those not yet reidentified will also turn out to belong to taxa other than C. nivalis. Those reidentified strains were isolated from snow samples, but genetic analyses of phylogenetic markers showed that they differed from the taxon known as C. nivalis. 200 years after the first field samples of Red Snow were brought back from a voyage under the British Admiralty [Bauer (Citation1819, Citation1820); Ross (Citation1820/1819)], phylogenetic analyses by Procházková et al. (Citation2019) of samples collected between 1999 and 2018 from 42 locations worldwide revealed a separate clade comprising samples of the most common taxon of Red Snow, for which a new genus of green algae, Sanguina, was established, with two species S. nivaloides (dark red cysts) and S. aurantia (orange cysts). We are not able to make these cysts germinate to study their life cycles, but the necessity of their transfer from the genus Chlamydomonas to a separate genus, as previously suggested (Leya, Citation2003), was finally confirmed. We still do not understand the reasons why these cysts do not germinate, but Sanguina nivaloides and S. aurantia are listed on the CCCryo web site (without living strains) to provide at least some geographic and genetic information on their field samples. The same applies to the unculturable Ancylonema nordenskiöldii and Mesotaenium berggrenii, two filamentous algae of the Zygnematophyceae causing Black Snow.
When looking at the temperature requirements of the strains maintained at CCCryo nearly 90% of all strains are cryophilic. About a third of all strains (33%) are psychrophilic and require temperatures ≤ 15°C for optimal growth; 56% tolerate temperatures down to 0°C (psychrotolerant), but prefer > 15°C; 10% of strains prefer conditions of a temperate climate (mesophilic) and 1% require warmer conditions (thermophilic).
Regarding the quality of our strains, shows some key numbers. Of a total of 518 strains more than 80% are clonal, i.e. each of those strains is based on single-cell isolation, whilst about 14% were picked from clonal colonies. 60% of all strains are axenic, i.e. free from bacteria and fungi. Deliberately, some species at CCCryo exist as axenic and non-axenic strains to enable the study of interactions between algae and bacteria. Though certainly the natural ratio of the algae to the bacteria will have changed over time in the isolate, we presume that at least one bacterial “partner” from the original field sample might have survived, though others might have been outcompeted and proportions certainly will have changed drastically. Whilst some algal isolates cope very well with the bacterial partners, others do not. In the latter case we reisolate the alga to save it. The 29 entries for non-living resting stages refer to field samples of the taxa responsible for Red and Black Snow. Though we work continuously on identifying and updating strain names, the generic names of 71 strains (14%) and the species name of 82 strains (16%) are still unconfirmed. Finally, a small yet increasing number (8 strains) of newly described cryophilic strains have been deposited at CCCryo as authentic strains (= living strains of holotypes). As few other algal culture collections have culture facilities with temperatures below 10°C, the option of depositing authentic strains at CCCryo has closed a gap in securing the biodiversity of these extremophilic algae. depicts the number of strains in relation to their geographic origin with a clear focus on Svalbard and Antarctica from where we sampled and isolated the majority of the cryophilic strains.
Figure 3. Key data reflecting the quality of the CCCryo strains: axenic = free from bacteria and fungi; clonal = strain originated from a single cell, i.e. the strain is genetically clonal; colony pick = strain originated from a colony, i.e. presumably the strain is genetically clonal; non-alive = these listings comprise unculturable cysts or field material; however they are listed in the database to provide this rare information to the scientific community; “cf.” genus/species = strains with so far unclear identity on genus or species level; authentic strains = these strains represent type strains that are deposited at other collections, herbaria or museums in a metabolically inactive state.
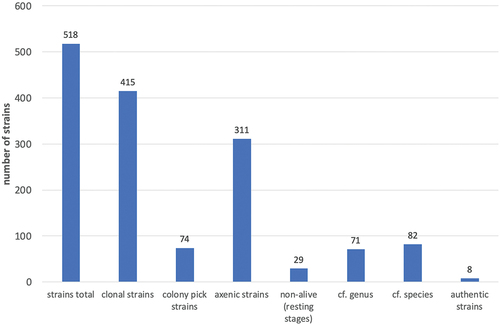
Figure 4. Number of CCCryo strains and their geographic origin. [earth-international = these 14 strains refer to isolates of a green alga (CCCryo 101–99) and Nostoc punctiforme from Spitsbergen (Svalbard) and Antarctica respectively that spent 469 days on the outside of the International Space Station ISS. To mark their stay outside of the Earth, they were labelled “origin: Earth-international” (J-P et al., Citation2019)].
![Figure 4. Number of CCCryo strains and their geographic origin. [earth-international = these 14 strains refer to isolates of a green alga (CCCryo 101–99) and Nostoc punctiforme from Spitsbergen (Svalbard) and Antarctica respectively that spent 469 days on the outside of the International Space Station ISS. To mark their stay outside of the Earth, they were labelled “origin: Earth-international” (J-P et al., Citation2019)].](/cms/asset/51ca51f2-89c0-47c5-ba50-3645349953d1/tapy_a_1753572_f0004_oc.jpg)
Cryopreservation of CCCryo strains
The CCCryo strains are maintained as living strains. Initially we started to cryopreserve strains but could not proceed with the experiments necessary to ensure sufficiently high survival ratios, basically because of insufficient staff. Nevertheless, more than half of all strains are cryopreserved in either 5% methanol or 5% DMSO or both, but survival rates, so far have been determined for only about 30 strains, range from 0 to 40% in Chloromonas and Chlamydomonas spp. and from 76 to 100% in many Trebouxiophycean algae (Raphidonema, Stichococcus, Tetracystis) and other green algae (e.g. Sphaerocystis). Initially it appeared astonishing that the strains of Chlamydomonas and Chloromonas did not survive though one would assume that psychrophilic species should be easier to cryopreserve than e.g. mesophilic species. But obviously surviving winter conditions on Svalbard at around −7°C below a protecting snow cover (Leya, Citation2004; Leya, Müller, Ling, & Fuhr, Citation2004) has nothing to do with surviving at −150°C in a cryoprotectant. It seems to be very important which cell stage is used for cryopreservation. In some experiments we found that aplanospores, e.g. of the psychrophilic Chlamydomonas klinobasis (CCCryo 050–99) survived cryopreservation, but not the motile flagellate cells (unpublished data) though this strain produces ice-structuring proteins (ISP, see below). Similar proteins found, e.g. in Antarctic fish are termed antifreeze proteins (AFP) and can evoke a freezing point depression by about 1°C, so that these fish can survive sea temperatures of −1.9°C (DeVries, Citation1983). It was assumed that AFPs or ISPs might favour survival at low temperatures, but the freezing point depression of snow algal ISPs is not as great (0.1°C) as that of fish AFP, and therefore, they appear unsuitable as cryoprotectants for storage below −150°C (Petasch, Citation2008; Rode, Citation2013).
Cryophilic Algae and their special adaptations
When we initially started our studies on cryophilic algae with our first collections and strain isolations on Spitsbergen, we faced critics who claimed that snow algae are nothing more than algae that can survive on snow. However, the fact that psychrophilic snow algae have a growth optimum of ≤ 15°C is very unusual. Currently we are doing in-depth analyses of various stress transcriptomes of two psychrophilic CCCryo strains to identify industrially interesting cold-active enzymes or other proteins. One early example is the ice-structuring protein of a Chlamydomonas strain. We already knew that only the psychrophilic Chlamydomonas or Chloromonas species produce ISP, while none of the psychrotolerant species at CCCryo do, moreover, the ISP-positive strains produce species-specific types of these proteins (Connor, Citation2011; Petasch, Citation2008; Speth, Citation2010). Furthermore, true (psychrophilic) snow algae seem to be remarkably well adapted to snow and glacier habitats, which are very low in nutrients (Spijkerman, Wacker, Weithoff, & Leya, Citation2012). The various snow and ice-inhabiting algal species produce unusual UV-absorbing substances (Remias et al., Citation2012), antioxidants (Leya, Rahn, Lãtz, & Remias, Citation2009), omega-3 fatty acids sought for supplementing the human diet (PUFAChain Consortium, Citation2017) or survive space- and Mars-like conditions as simulated on the outside of the International Space Station (de Vera et al. Citation2019). Analysing the growth and production characteristics of EPA (eicosapentaenoic acid)-producing algae, we could show that cryophilic strains perform better in outdoor photobioreactor plants in colder climates than strains not adapted to such climate conditions (Jorde et al., Citation2017; PUFAChain Consortium, Citation2017). Last but not least the CCCryo includes many algal species that need to be newly described or examined in more detail [for examples see Procházková et al. (Citation2019), Remias, Karsten, Lütz, and Leya (Citation2010) and Remias, Wastian, Lütz, and Leya (Citation2013)]. Some of the above aspects are described in Leya (Citation2013), others are outlined below.
CCCryo strains for fundamental studies
When we studied the first CCCryo strains we soon faced the problem that many isolates could not be identified to genus or species level as they did not meet the existing description of valid taxa. The status quo of strain names is the best to our current knowledge, yet in some cases species names have to be regarded as preliminary (see taxa with the prefix “cf.” in front of their genus or species name). In some rare cases we could not even find a suitable genus name for some strains, so that they could only be determined to a higher taxonomic level (e.g. indet. Chlorosarcinaceae). Sequencing of phylogenetic marker genes such as 18S, ITS or rbcL was very helpful and most of those are available in GenBank when searching for the string “CCCryo” or when checking the respective entries in our online database. Regarding the 18 S rRNA gene, information for about 90 strains is available; for the ITS gene this covers about 106 strains; and information on the rbcL gene is available for about 18 strains. Some intensive work has been done in our group (Bey, Citation2010). We also share our reference sequence lists of different marker genes with colleagues doing high-throughput sequencing of field samples to enable species allocation to their OTUs (e.g. Lutz, Procházková, Benning, Nedbalová, & Remias, Citation2019). Yet much more work is necessary to describe snow algal diversity in a taxonomically correct way, as many taxa must be revised or described anew. The same applies to many physiological, cell, cryo-biological or molecular questions, of which only a few have been addressed yet (Bley, Citation2006; Connor, Citation2011; Hulatt, Berecz, Egeland, Wijffels, & Kiron, Citation2017; Hulatt & Thomas, Citation2011; Kreische, Citation2014; Lenz, Citation2015; Leya, Citation2003, Citation2018; Leya, Müller, Ling, & Fuhr, Citation2001; Leya et al., Citation2009; Pawella, Citation2008; Remias et al., Citation2010, Citation2013; Spijkerman et al., Citation2012; Zacke, Citation2007).
Why are cryophilic algae interesting as a bioresource for industrial applications?
When asked why one should use cryophilic algae for industrial products, different strategies can be outlined. For public relations purposes, terms like “cryophilic algae” or “snow algae” are a unique or at least unusual selling point. Especially in cosmetics or food supplements this can have considerable marketing advantages. If outdoor algal mass production facilities are considered, cryophilic algae could show an advantage in growth rates if the geographic location and the respective climate are considered. In colder regions like Northern Europe or Canada, cryophilic algae have growth rates at outdoor temperatures that become comparable to those of mesophilic algae cultured in Middle or Southern Europe (Jorde et al., Citation2017). Especially if the metabolite production rates are considered, it could pay to use a cryophilic algal strain in a photobioreactor plant, e.g. to produce omega-3 fatty acids in Scandinavia. A mesophilic alga as a production strain might not yield sufficient quantities of the sought-after metabolite if grown under cold environmental conditions, but a psychrotrophic species does. For each prevailing production condition, a suitable algal strain best adapted to these conditions should be used. In permanently cold conditions it could be a cryophilic strain throughout the year, or a mesophilic strain could be used during summer and autumn and replaced by a cryophilic strain for winter and spring. We call this change of the production strain the algal crop rotation (ACR) principle. This way the overall productivity can be maximized. We demonstrated this during the EU-funded project PUFAChain (PUFAChain Consortium, Citation2017) when screening algal strains from the SAG and CCCryo for industrial production of polyunsaturated fatty acids, the omega-3 fatty acids EPA (eicosapentaenoic acid) and DHA (docosahexaenoic acid). shows that when growing the psychrophilic Raphidonema strain CCCryo 381–11 at colder temperatures below 12-13°C its EPA productivity is about ten-fold higher than that of Vischeria sp. (formerly Chloridella simplex) strain SAG 51.91. Especially under lower light intensities productivity of this Raphidonema strain nearly equals the Vischeria strain, as the low-light-adapted Raphidonema seems to utilize light energy more efficiently (). Also supplying additional CO2 to the culture more than doubled its EPA yield. At temperatures above 12–13°C the Vischeria strain was more productive, and seemed to be more efficient in producing the omega-3 fatty acid EPA (). Further information on the experiment can be found in the final report of the EU-project PUFAChain (PUFAChain Consortium, Citation2017). So, one can say that the Vischeria strain is more suitable if the production plant is operated in warmer regions with a warm climate over the whole year, e.g. in Southern Europe, however, if a company in Middle or Northern Europe decides to produce EPA with the aid of microalgae, it is well advised to choose an algal strain/species that is better adapted to the dominating colder and less sunny climate. This choice can also mean growing, e.g. a Vischeria strain during the warmer months and growing a cryophilic strain like the depicted Raphidonema strain during the colder months. Our ACR principle suggests that rotating the crop over the year to select the production strain best adapted to the prevailing culture conditions can enhance the overall economic efficiency of an algal production plant. This is one way to optimize production processes when planning to use algae as new bioresources to replace traditional sources. Regarding many algal products such an optimization is very important to become economically compatible with conventional sources and production processes.
Figure 5. Comparison of the psychrophilic Raphidonema nivale strain CCCryo 381–11 with the Vischeria sp. (formerly Chloridella simplex) strain SAG 51.91 regarding the production of the omega-3 fatty acid EPA (eicosapentaenoic acid) under different temperature and light conditions. (a) depicts EPA productivity taking the photon fluence rate E into account. (b) shows the productivity per litre and day. Cultures were bubbled with either sterile filtered air (closed symbols) or additional 3–5 v/v % CO2 (open symbols).
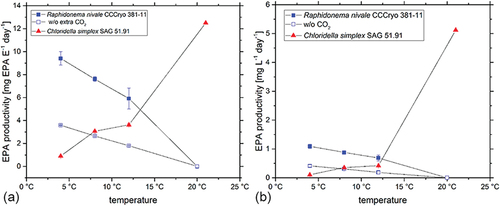
Hulatt et al. (Citation2017) addressed the problem of declining biomass and lipid yields in algal cultures in colder climates in his study of some psychrophilic strains of Chlamydomonas, Chloromonas and Raphidonema and also state that the use of cryophilic strains can provide an opportunity to increase yields at low temperatures. In general we can say that cryophilic algae show growth rates at cold temperatures that are comparable with those of mesophilic algae at warm temperatures (Hulatt et al., Citation2017). This becomes evident when looking at product yields (), but also is the case when comparing biomass growth rates. Of course cryophilic algae do not have an unbeatable advantage over other algae – it strongly depends on the target product, the revenues to be expected from the respective market, the location where the algae will be grown and where downstream processes will take place. Also, costs for personnel and energy need to be considered as these may vary greatly among different countries. So, reasons to use a cryophilic alga might reflect a certain PR-strategy, a specific location in a cold climate where the algae will be grown, but also possibly a product that is unique to psychrophilic algae (see specific examples below). Many algae-based products are already so well established that it would be nonsense to shift to cryophilic algae as an alternative resource. Many snow and permafrost algae produce astaxanthin, which is marketed as a valuable pigment for fish cultures and as an antioxidant in food supplements. However, the industrial production process has been developed and has been put into practice largely based on Haematococcus lacustris (formerly H. pluvialis) (Fábregas, Otero, Maseda, & Domínguez, Citation2001; Lorenz & Cysewski, Citation2000). There is no pressure to change this production process. However, if a carotenoid- and astaxanthin-producing strain is to be grown in a region with a colder climate, using a cryophilic strain might be more sensible. Some examples for industrial products from cryophilic algae will now be outlined.
Cosmetic ingredients in general
The cosmetics sector is a very trend-driven market. Promotion of novel products with new properties due to new ingredients with new effects is the big driver in this billion-dollar-market.Footnote1 Legal regulations are relatively easy to meet compared to other markets and companies are willing to develop new products if a unique selling point or marketable idea is offered. In 2008 we started to develop a special production process for a psychrophilic CCCryo strain for a company developing cosmetics. From the biomass produced that company developed the so-called “snow algae powder” as an ingredient for different lines of cosmetics and products for various brands. Having started with some test biomass in 2008 we have had a fruitful cooperation for more than 10 years. “Snow algae cosmetics” products are now available world-wide under different brands. This is a successful showcase for an industrial application for algae from snow, because the story behind the product, apart from the functional benefits, helped greatly in marketing the product and certainly was a market-opener for us.
Cold-active enzymes from psychrophilic snow algae
Psychrophilic algae have adapted their metabolism to low temperatures and their enzymes have high catalytic efficiencies at low temperature relative to their mesophilic and thermophilic counterparts (Varshney, Mikulic, Vonshak, Beardall, & Wangikar, Citation2015). For CCCryo strains 020–99 and 050–99 differential display studies comparing the proteome and transcriptome of these two psychrophilic strains have shown changes in their proteome and transcriptome patterns. Pawella (Citation2008) compared cultures grown at 2°C and 8°C and detected many proteins on 2D-SDS gels that were only produced by the 2°C culture. Bley (Citation2006) grew cultures of strain CCCryo 020–99 at 8°C and then exposed it to 2°C and vice versa and took samples for transcript analyses after 0.5, 2, 4 and 8 hours. Using arbitrary primers, she could prove an up- or downregulation of several transcripts 0.5–2 h after the temperature shock whilst the house-keeping enzyme GAPDH (glutaraldehyde 3-phospatase dehydrogenase) showed a constant transcript level. Zacke (Citation2007) compared the specific temperature optima of the MDHs (malate dehydrogenase) of several psychrophilic strains with that of the mesophilic Chlamydomonas reinhardtii strain CCCryo 152b-01 (= SAG 11–32b). He could demonstrate at the enzyme level that the MDH of strain CCCryo 020–99 has an optimum temperature for its relative activity 10 K lower than that of C. reinhardtii. Cold-active enzymes have been described for many prokaryotes (Cavicchioli, Siddiqui, Andrews, & Sowers, Citation2002 and citations therein; Gerday et al., Citation1997) and numerous biotechnological applications have been suggested (Cavicchioli et al., Citation2002; Margesin & Schinner, Citation1999). Psychrophilic eukaryotes like algae have hardly been studied yet, probably because suitable algal strains were not easily available from algal collections. Recently we have sequenced the transcriptomes of two psychrophilic algae after different types of stress and hope to find specific genes for cold-active enzymes with potential applications in different markets (unpublished). The gene for an ice-structuring protein has already been found in these transcriptome data. Markets suitable for cold-adapted enzymes from eukaryotic algae include food and feed processing, molecular diagnostics and biology, and the cosmetics sector.
Ice-structuring proteins from psychrophilic snow algae
Ice-structuring proteins (ISP), a special group of proteins formerly called antifreeze proteins (AFP) (Clarke, Buckley, & Lindner, Citation2002), are known from many prokaryotic and eukaryotic organisms (Leya, Citation2013) such as bacteria, fungi, green algae, diatoms, cyanobacteria, mosses, higher plants like grasses and grains, spiders, insects, and Antarctic fish. From the latter they were first described by DeVries (Citation1971). These proteins are also characteristic of the truly cryophilic, the psychrophilic snow algae, but not for the psychrotolerant (non-obligate psychrophiles) (Petasch, Citation2008; Speth, Citation2010). Though the proteins from snow algae hardly prevent freezing as their former name might suggest, they force water ice-crystals into a specific shape, thus controlling their normally unrestricted growth. We have tested ISP from the psychrophilic Chlamydomonas klinobasis strain CCCryo 050–99 in cryopreservation protocols as well, without identifying positive effects on survival (Rode, Citation2013). In the Raphidonema strain CCCryo 011a-99 increasing ISP-concentrations in the CPA (cryoprotective agent) failed to achieve survival rates induced by 5% methanol as the CPA alone. Even the addition of ISP to methanol as an CPA did not lead to an increase in survival rates. When trying to cryopreserve strain CCCryo 050–99 itself, only low survival rates of 3% were achieved with 5% methanol alone as the CPA, which decreased further when adding 50 µg ml−1 ISP produced by the alga itself. AFPs or ISPs have been investigated as CPAs or additives to CPAs in many cryopreservation protocols with diverse results. Koshimoto and Mazur (Citation2002) found deleterious effects when cryopreserving mouse spermatozoa; Carpenter and Hansen (Citation1992) observed indistinct effects on red blood cells; but positive effects were found when freezing Escherichia coli cells (Kawahara, Higa, Tatsukawa, & Obata, Citation2009), zebra fish embryos (Martínez-Páramo, Barbosa, Pérez-Cerezales, Robles, & Herráez, Citation2009), islets of Langerhans (Matsumoto et al., Citation2006) and rat hearts (Amir et al., Citation2004). Finding optimum concentrations of CPAs or combinations with additives and target-specific freezing protocols is required and needs more investigation.
Though known from so many organisms since the 1960s, it is surprising that only one industrial product has ever entered the market. Unilever (Daniel et al. Citation2007) used fish AFPs transgenically produced in yeast in some types of low-fat Breyers ice creams. The natural function of ISP in psychrophilic snow algae is not yet clear either. Possibly it functions as a natural binder for the microalgal cells to melt ice crystals during snow storms. This way the algae are passively but efficiently dispersed together with the snow crystals and at the same time the algal cells are close to their liquid water resource.
Polyunsaturated fatty acids (PUFAs) from psychrophilic snow and permafrost algae
In PUFAChain we studied many CCCryo strains for their ability to produce PUFAs, particularly EPA and DHA which are well suited to the food supplement market. As the currently used marine fish sources are diminishing, potent microalgae are coming into focus, in particular, some strains of Raphidonema and Koliella (Trebouxiophyceae) which are potential EPA producers. As shown in , strain CCCryo 381–11 of the psychrophilic Raphidonema nivale produced similar amounts of EPA when grown at 4°C as the cryotolerant/mesophilic SAG strain 51.91 of Vischeria sp. (formerly Chloridella simplex) grown at around 20°C. So again, psychrophilic strains show their potential when a production strain for photobioreactor plants in cold regions is sought. The EPA contents of >60 strains of CCCryo strains screened by PUFAChain were between 0.014 mg g−1 dry mass (for an unidentified strain of Pleurastrum sp.) and 28.75 mg g−1 for a snow diatom (Chamaepinnularia sp.). However, these EPA contents were topped by 29.00 mg g−1 for strain SAG 51.91 of Vischeria sp. and 90.00 mg g−1 for SAG 2.99 of Microchloropsis gaditana (formerly Nannochloropsis gaditana). Raphidonema and Koliella strains proved to be good producers of EPA with values ranging between 1.00 and 14.04 mg g−1 dry mass depending on the species and strain. shows a fatty acid profile of CCCryo strain 198–04 of a Koliella sp. (Schulze, Citation2011). „EPA (C20:5) and the very long-chain omega-9 fatty acids C22:1 and C24:1 are striking for this alga, the latter presumably being related to nervonic acid, which has been considered for the treatment of demyelinating disorders of neurons (Coupland & Langley, Citation1993; Tang et al., Citation2013). Psychrophilic Chlamydomonas and Chloromonas, though obligatorily adapted to low temperatures, did not produce any noticeable amounts of long or very long chain PUFAs. Hulatt et al. (Citation2017) studied five psychrophilic CCCryo strains and found that such cold-adapted strains could provide an opportunity to increase yields of algal biomass and oil products under low temperature conditions.
Figure 6. Fatty acid profile of strain CCCryo 198–04 of a Koliella sp. with long-chain fatty acids. (N+ = nitrate-containing medium, N- = nitrate-depleted medium); from Schulze, Citation2011).
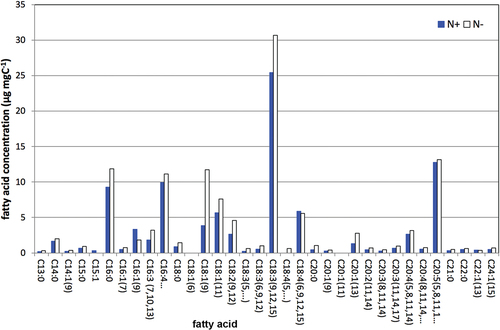
Pigments from psychrotolerant permafrost algae
The first metabolite that comes to mind when talking of snow algae might be carotenoids, especially astaxanthin. This ketocarotenoid has a high market price as a food supplement for humans and currently is produced industrially from Haematococcus lacustris biomass. It also has a big market in aquaculture as it gives salmonids and crustaceans the attractive salmon-like pigmentation, increases survival rates of fish and crustacean larvae during breeding and is used to pigment egg yolks (Christiansen, Lie, & Torrissen, Citation1995; Elwinger, Lignell, & Wilhelmson, Citation1997; Lorenz & Cysewski, Citation2000; Menasveta, Worawattanamateekul, Latscha, & Clark, Citation1993). Sanguina nivaloides produces large amounts of astaxanthin in its cysts, but as no living cultures exist, it cannot be used to produce it. Yet, many other cold-adapted algae, mainly from the permafrost habitats surrounding the snow and glacier fields, also produce various carotenoids including free and esterified astaxanthin when nutrient-depleted. depicts concentrations and ratios of carotenoids in a permafrost algal strain from the CCCryo. More than 50% of all carotenoids were astaxanthin and its esters and total astaxanthin amounted to 1.8 mg g−1 dry mass. From a biotechnological point of view culturing Haematococcus lacustris is much more efficient with astaxanthin concentrations around 40 mg g−1 dry weight (Lee & Soh, Citation1991); however, this mesophilic algal species would not grow and thus, not yield any astaxanthin in cold climates. Although the cryophilic CCCryo strain is less productive, it can cope well with low temperatures and low irradiance levels and yet produce considerable amounts of astaxanthin.
Figure 7. Carotenoid spectrum of a CCCryo strain from permafrost. (a) Carotenoids and chlorophylls as concentrations of dry mass. (b) Single carotenes and xanthophylls as ratios of all carotenoids detectable present. (data from the Fraunhofer-internal project MassCaro, unpublished).
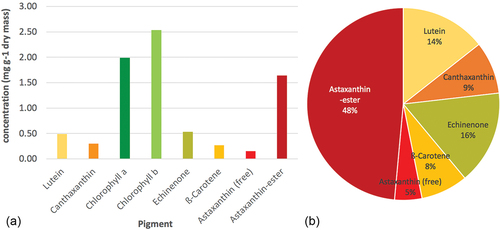
Together with the above example for EPA production in cold climates this example shows the advantages and disadvantages of cold-adapted algal strains for biotechnological production processes. They may not compete with mesophilic production strains at warm culture temperatures, but those mesophilic strains may themselves not compete with cryophilic ones at cold culture temperatures. An optimization of a production process is always necessary to increase yields and profit and though one can assume that in the end yields will be lower in a colder climate, such bio-based production processes in cold environments can be made possible by using cryophilic algal strains.
UV-absorbing substances from psychrotolerant permafrost algae
MAAs (mycosporine-like amino acids) are known as photoprotectants against UVR (ultraviolet radiation) obtained mainly from cyanobacteria (Richa et al., Citation2011), but they also have been found in a range of other organisms like fungi, macroalgae, phytoplankton communities (Sinha, Singh, & Häder, Citation2007) and in the Trebouxiophyceae (Hartmann, Holzinger, Ganzera, & Karsten, Citation2016; Karsten, Friedl, Schumann, Hoyer, & Lembcke, Citation2005). In the organisms themselves they protect intracellular structures and biomolecules from damage by converting the energy from UV-radiation into heat, but may have other functions (Bandaranayake, Citation1998; Oren & Gunde-Cimerman, Citation2007). They also decrease the sensitivity of organisms to UV (Ferroni, Klisch, Pancaldi, & Hader, Citation2010). Hotter et al. (Citation2018) studied many Trebouxiophyceae for their potential for producing MAAs, and as this group is well represented on polar snow fields we screened several strains of Koliella, Raphidonema and Stichococcus in this regard (Ettingshausen, Citation2019). The cosmetics market is interested in these natural sunscreens as alternative UV-protective additives in sunscreen creams (Carreto & Carignan, Citation2011; Richa et al., Citation2011), because the currently used chemicals seem to have considerably damaging effects on fish and corals in our marine environment (Coronado et al., Citation2008; Ladrière, Compère, Decloux, Vandewalle, & Poulicek, Citation2008) but there is no biotechnological process to produce these MAAs. Often, the main problem with bio-based products at industrial scale is to develop a production process that ensures reliable delivery of satisfactory amounts to the formulating cosmetics companies. Though it has been shown that MAAs can be synthesized in a heterologous expression system (Miyamoto, Komatsu, & Ikeda, Citation2014), this option is problematic for market entry as GMO ingredients for cosmetics are rarely acceptable though allowed in principle. However, as UV-protective creams are subject to different control regulations than regular cosmetics products, customers’ reservations in this respect might be allayed by realizing that special cosmetics require raw materials from different production processes than regular cosmetics. Yet, reliable natural sources and an easy production process would clearly be of advantage. Ettingshausen (Citation2019) identified a psychrotolerant CCCryo strain from an Iceland glacier presumably from the genus Stichococcus that produced a UV-absorbing substance with a broad absorption in the range of 320–335 nm. Interestingly induction by UV-light was not required for this substance to be synthesized by the alga, which could greatly facilitate a biotechnological production process. This substance might be identical with the MAA prasiolin or the prasiolin-like MAA reported by Hotter et al. (Citation2018). However, they found that prasiolin is rather unstable and this fact, together with the low amounts produced by the algae compared to the amounts sought by the cosmetics industry, could be hindering its market. Possibly this can only be overcome by production in an acceptable heterologous expression system.
Extracellular polymeric substances (EPS) from psychrotolerant permafrost algae
Many CCCryo strains from permafrost habitats belong to spore- and sporangia-forming taxa (cf. Coenochloris, Dictyococcus, Gloeocystis, Ploeotila, cf. Sphaerocystis and some Chloromonas species). When environmental conditions become unfavourable for growth in spring with dwindling water availability, with a decrease in nutrients, an increase in irradiation due to days with 24 hours of daylight or at the beginning of winter with freezing temperatures and decreasing daylengths, these algae form resting spores. Apart from large amounts of carotenoids accumulated in oil droplets by some taxa, the spores often remain arranged in multi-celled sporangia surrounded by a mucilage layer consisting of various sugars of different proportions (glucose, xylose, galactose, arabinose, mannose and rhamnose) and respective uronic acids (unpublished). These EPSs presumably prevent cells from desiccation and offer mechanical protection and defence against microscopic predators. Depending on the composition of sugars and overall formulation these EPSs seem to have varying gel properties. Strain CCCryo 147–01 of Gloeocystis sp. produced a gel-like, but form-stable EPS (). When the culture was centrifuged to harvest the EPS for analysis most of the algal cells pelleted at the bottom whilst some cells remained trapped in the EPS together with many air bubbles () also illustrating the form-stability of this EPS.
Figure 8. Extracellular polymeric substances (EPS) produced by strain CCCryo 147–01 of Gloeocystis sp., a psychrophilic isolate from snow. (a) Single cells and four-celled sporangia surrounded by EPS. (b) The mucilage layer made visible by negative staining with Burri’s ink. (c) When centrifuged some green cells remain trapped within a gel-like, form stable mass capable of trapping air bubbles.
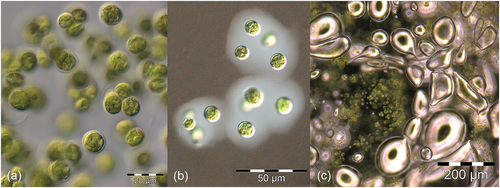
In the cosmetics sector many products from shower, shaving and hair gels to peelings, lipsticks and shampoos contain small microplastic beads or water soluble, liquid or wax- and gel-like plastics creating the desired gel state. Microplastics and other nanomaterials have been proven to be washed into our seas and to accumulate in fish and bivalves (Collard et al., Citation2017; Li, Yang, Li, Jabeen, & Shi, Citation2015) with toxic effect on human cerebral and epithelial cells when taken up (Schirinzi et al., Citation2017). Thus, the cosmetics sector is very keen on banning or at least reducing synthetic plastics. Algae-based EPSs with form- or structure-stabilizing effects e.g. in hair or shaving gels could be a bio-based alternative. However, again, effective production processes need to be developed to increase yields and reduce costs to overcome the competitive hurdles in comparison to conventional, synthetic additives.
The importance and future of the CCCryo biobank
The Culture Collection of Cryophilic Algae has become a valuable biobank over the last years. The scientific community of snow algae researchers has increased from not even a handful around the turn of the millennium to 11 participants from 7 institutions at the Snow Algae Meeting SAM2016 and to 26 participants from 17 institutions at SAM2018. Studies cover various aspects from field and basic laboratory studies to projects with applied aspects and product developments. The biobank has around 90 customers from 41 countries from the Americas, Europe and Asia. The CCCryo is home to a large diversity of mainly cryophilic algal isolates and is unique world-wide in its scope.
Generally, algae as a source of ingredients are well accepted in the food and food supplement sector and they are highly marketable, particularly as the trend for vegetarian and vegan food is big and algae are compliant with this. On the other side, we often face legislation problems that hinder the easy entry of so far unknown algae onto the food market. The EU novel food catalogue lists those organisms, products thereof and other substances that have authorization to enter as food products onto the European Union markets (European Commission, Citation2019). Likewise, it gives an orientation which bioresources need an approval under the Novel Food Regulation, before they can be marketed. A glance at the list showed that from the estimated 30,000 to over 1 million algal species (Guiry, Citation2012), only around 31 species from 22 genera, e.g. Aphanizomenon (1 species), Arthrospira (1), Ascophyllum (2) Chlorella (3), Ecklonia (1), Fucus (4) Laminaria (4), Saccharina (1), Ulva (1), Undaria (1)] are listed and authorized for food currently. Authorization of new species is time-consuming and expensive with costs estimated from some 10,000 to 1 million Euros (pers. communication). It is understandable that such costs hinder the development of novel, algal-based products for the food market. Despite low profit margins, also because production costs for algae generally are still high, the food market surely is an interesting market, but EU authorities should facilitate access via the novel food catalogue without risking safety issues for the customer. This could give extra growth to this market through new products. Cold-adapted algae will hardly be able to compete with mesophilic ones due to the pressure on prices unless the algal production conditions favour the use of cold-adapted strains, e.g. in outdoor facilities in Northern European climates.
The food supplement market promises better profits, but likewise is strictly controlled by either the novel food catalogue if a complete organism or preparation thereof is concerned, or by the REACH (Registration, Evaluation, Authorization and Restriction of Chemicals) regulation for the European Union market regarding extracted and refined substances. Such algal-based substances, e.g. polyunsaturated fatty acids, antioxidants like astaxanthin or dyes/pigments need a registration before being allowed to enter the market. Again, for registration extensive data covering technical information and extensive and costly toxicity and compliance tests are required.
CCCryo strains surely could be used to develop products for either of these markets, yet there can be a certain imbalance of costs for R&D (research and development) and the profit on the other side. For many readily available products like tablets of whole algal cells [“Spirulina” (Arthrospira) or Chlorella], astaxanthin (Haematococcus lacustris) or omega-3 fatty acids (Crypthecodinium, Schizochytrium, Nannochloropsis) the sources or production processes have been established already and companies like to stick to established processes if they are profitable. As the profit margins in these markets are rather low to medium compared to the cosmetics or pharmaceuticals sector, it would be rather hard to establish new processes based on cryophilic strains and there might be no need for that, unless the cryophilic algae would offer new types of PUFA valuable for human or animal consumption. In general, it is not advisable to work on a push-based strategy to place algal-based products on a market, i.e. having identified a metabolite and then search for a customer. Rather should one apply a pull-strategy monitoring the market for its demands and trends and then offer a suitable algal species or strain to the companies involved in that specific market.
The cosmetics market is a desirable and acceptable market in terms of regulations. Looking worldwide, regulation is realized by the so-called China compliance list, officially called: Inventory of Existing Cosmetic Ingredients in China – IECIC (Citation2014). In its current version it lists 8641 cosmetic ingredients. Regarding algae, single species and preparations or extracts thereof are listed, but also the entries “algae extract” or just “algae” exist. This gives a certain freedom to place algae and their products on the cosmetics market. The safety of such algae-based ingredients when applied on human skin yet needs to be proven. The first things tested by cosmetics companies when they develop new ingredients are their non-toxicity and non-allergenicity. These safety issues are necessary and one needs to have them in mind when doing research in this field.
The development of cold-active enzymes from eukaryotic algae is hardly existent except for a few with little importance for industrial applications (Chen et al., Citation2017; Di Martino Rigano et al., Citation2006; Yaish, Doxey, McConkey, Moffatt, & Griffith, Citation2006). This may be due to the rare availability of suitable, cold-adapted algal strains. More often psychrophilic bacteria have been investigated (Margesin & Schinner, Citation1999) and a multiple of cold-active enzymes have been identified (Cavicchioli et al., Citation2002 and citations therein) and find different applications in the food and food processing (Hamid & Mohiddin, Citation2018), chemical, detergent, and dairy industry and many more industrial and research sectors (Cavicchioli et al., Citation2002). Often energy in industrial processes can be saved as the use of enzymes that are optimally adapted to the specific process temperature have a higher catalysis turnover, or processes can be performed at lower process temperatures if suitable enzymes are used (Gerday et al., Citation1997). Yet, the public acceptance for algal enzymes, especially if used for food processing or cosmetics, might be higher if these enzymes were derived from algae/plants rather than from bacteria or fungi. With our current sequencing project on several stress transcriptomes (salt, red/blue light and low vs. high CO2) of two psychrophilic snow algal species we hope to add some valuable insights into cold-active enzymes from algae once the data are evaluated. Also, genetic information on proteins and enzymes that are non-existent in prokaryotes might be detected and further applications could be developed from them.
The hurdles for entering either of the above-mentioned markets with novel bioresources or their metabolites can be high and costly depending on the market you want to access. In the capitalistic economic system the final profit counts. Novel algae and their bio-based products can balance the immense initial costs of development if there is a high profit due to either a novel PR strategy, the solution to an existing problem or the creation of a new product. Special funding or cooperating companies willing to take the risk (and costs) are desirable to overcome this gap. This would facilitate approaches to launch algae and algal products, regardless if common or extremophile, on suitable industrial and consumer markets. The conversion of our industry from fossil resources to renewable bioresources is underway, but legislation can also be hindering. This bias must be conquered.
Acknowledgments
I am specifically thankful to Günter R. Fuhr for having made numerous scientific voyages to Svalbard and the Antarctic and many invaluable experiences in the Arctic possible. I also would like to thank Hau U. Ling who advised me on snow algae and how to isolate single cells during our expedition together to Spitsbergen in 1999. I am also grateful to students and co-workers who used strains from the CCCryo biobank for their projects at our institute and with whom we obtained valuable results. References are given where appropriate.
Disclosure statement
In accordance with Taylor & Francis policy and my ethical obligation as a researcher, I am reporting that I have no potential conflicts of interest to declare.
Additional information
Funding
Notes
1 For example the world-wide skin-care market volume was forecasted to reach 133 billion US-$ in 2019 (https://de.statista.com/themen/25/kosmetik/, accessed on 13 November 2019).
References
- Amir, G., Rubinsky, B., Horowitz, L., Miller, L., Leor, J., Kassif, Y., … Lavee, J. (2004). Prolonged 24-hour subzero preservation of heterotopically transplanted rat hearts using antifreeze proteins derived from arctic fish. The Annals of Thoracic Surgery, 77(5), 1648–1655.
- Andersen, R. A. (Ed). (2005). Algal culturing techniques. London: Elsevier Academic Press.
- Aristotle. ( 350 BC, translated by D’Arcy Wentworth Thompson). Chapter 19. In The works of Aristotle: History of animals, Book V. Chicago: University of Chicago.
- Bandaranayake, W. M. (1998). Mycosporines: Are they nature’s sunscreens? Natural Product Reports, 15(2), 159–172.
- Bauer, F. (1819). Microscopical observation on the red snow. Quarterly Journal of Science and Arts, 7, 222–229. (incl. plate VI).
- Bauer, F. (1820). Red snow of Baffin’s Bay. Amer J Sci Arts, 2(2), 356.
- Bey, T. D. (2010). ITS-Barcoding als zusätzliches Hilfsmittel zur Artdifferenzierung von Schneealgen ( Bachelor Thesis). Universität Potsdam, Potsdam, Germany.
- Bley, U. (2006). Differentielle Transkriptanalysen an der psychrophilen Schneealge Chloromonas sp. Stamm CCCryo020-99 (Chlamydomonadaceae, Chlorophyta) durch Hitze- und Kälteschockversuche. Student Internship. Berlin, Germany: Humboldt-Universität zu Berlin.
- Carpenter, J. F., & Hansen, T. N. (1992). Antifreeze protein modulates cell survival during cryopreservation: Mediation through influence on ice crystal growth .Proceedings of the National Academy of Sciences, 89(19), 8953–8957.
- Carreto, J. I., & Carignan, M. O. (2011). Mycosporine-like amino acids: Relevant secondary metabolites. chemical and ecological aspects. Marine Drugs, 9(3), 387–446.
- Cavicchioli, R., Siddiqui, K. S., Andrews, D., & Sowers, K. R. (2002). Low-temperature extremophiles and their applications. Current Opinion in Biotechnology, 13(3), 253–261.
- Chen, J., Cui, H., Hou, Y., Cui, Y., Wang, Y., Xu, Y., … Qin, S. (2017). Molecular cloning, expression and characterisation of glucokinase gene from the mixotrophic green alga Chlorella kessleri. Phycologia, 56(5), 477–486.
- Christiansen, R., Lie, O., & Torrissen, O. J. (1995). Growth and survival of Atlantic salmon, Salmo salar L., fed different dietary levels of astaxanthin. First-feeding fry. Aquaculture Nutrition, 1(3), 189–198.
- Clarke, C. J., Buckley, S. L., & Lindner, N. (2002). Ice structuring proteins - a new name for antifreeze proteins. Cryo Letters, 23(2), 89–92.
- Collard, F., Gilbert, B., Compère, P., Eppe, G., Das, K., Jauniaux, T., & Parmentier, E. (2017). Microplastics in livers of European anchovies (Engraulis encrasicolus, L.). Environmental Pollution, 229, 1000–1005.
- Connor, D. (2011). Molekulare Charakterisierung von eisstrukturierenden Proteinen (ISP) aus kryophilen Schneealgen (Master Thesis) Westfälische Wilhelms-Universität, Münster, Germany.
- Coronado, M., De Haro, H., Deng, X., Rempel, M. A., Lavado, R., & Schlenk, D. (2008). Estrogenic activity and reproductive effects of the UV-filter oxybenzone (2-hydroxy-4-methoxyphenyl-methanone) in fish. Aquatic Toxicology, 90(3), 182–187.
- Coupland, K., & Langley, N. A. (1993) Use of nervonic acid and long chain fatty acids for the treatment of demyelinating disorders. U.S.A. Patent 5,194,448, 16. 03.1993
- Daniel, A., Hoddle, A., Jones, A., Oldroyd, J. R., & Singleton, S. (2007) Ice cream confection containing an antifreeze protein. EU Patent EP 1 417 892 B1, 23.05.2007.
- DeVries, A. (1971). Glycoproteins as biological antifreeze agents in Antarctic fishes. Science, 172(3988), 1152–1155.
- DeVries, A. L. (1983). Antifreeze Peptides and Glycopeptides in Cold-Water Fishes. Annual Review of Physiology, 45(1), 245–260.
- Di Martino Rigano, V., Vona, V., Lobosco, O., Carillo, P., Lunn, J. E., Carfagna, S., … Rigano, C. (2006). Temperature dependence of nitrate reductase in the psychrophilic unicellular alga Koliella antarctica and the mesophilic alga Chlorella sorokiniana. Plant, Cell and Environment, 29(7), 1400–1409.
- Elwinger, K., Lignell, A., & Wilhelmson, M. (1997). Astaxanthin rich algal meal (Haematococcus pluvialis) as carotenoid source in feed for laying hens. In: Proceedings of the VII European Symposium on the Quality of Eggs and Egg Products, Poznan, Poland (pp 52–59).
- Ettingshausen, J. (2019). Screening of microalgae and cyanobacteria for UV-absorbing substances (Bachelor Thesis). Technical University of Berlin, Berlin, Germany.
- Ettl, H. (1988, pers. comm. U. Schlösser). Re-identification of various Chlamydomonadaceae strains at SAG, UTEX and other culture collections. unpublished .
- European Commission. (2019). Novel food catalogue. Retrieved 2019, 23 October, from https://ec.europa.eu/food/safety/novel_food/catalogue_en.
- Fábregas, J., Otero, A., Maseda, A., & Domínguez, A. (2001). Two-stage cultures for the production of Astaxanthin from Haematococcus pluvialis. Journal of Biotechnology, 89(1), 65–71.
- Ferroni, L., Klisch, M., Pancaldi, S., & Hader, D.-P. (2010). Complementary UV-absorption of mycosporine-like amino acids and scytonemin is responsible for the UV-insensitivity of photosynthesis in Nostoc flagelliforme. Marine Drugs, 8(1), 106–121.
- Gerday, C., Aittaleb, M., Arpigny, J. L., Baise, E., Chessa, J.-P., Garsoux, G., … Feller, G. (1997). Psychrophilic enzymes: A thermodynamic challenge. Biochimica Et Biophysica Acta (BBA) - Protein Structure and Molecular Enzymology, 1342(2), 119–131.
- Guiry, M. D. (2012). How many species of algae are there? Journal of Phycology, 48(5), 1057–1063.
- Guiry, M. D., & Guiry, G. M. (2018) AlgaeBase. World-wide electronic publication. National University of Ireland, Galway. Retrieved 2019, October 21, from http://www.algaebase.org.
- Hamid, B., & Mohiddin, F. A. (2018). Cold-active enzymes in food processing. In M. Kuddus (Ed.), Enzymes in Food Technology: Improvements and Innovations (pp. 383–400). Singapore: Springer Singapore. doi:10.1007/978-981-13-1933-4_19
- Hartmann, A., Holzinger, A., Ganzera, M., & Karsten, U. (2016). Prasiolin, a new UV-sunscreen compound in the terrestrial green macroalga Prasiola calophylla (Carmichael ex Greville) Kützing (Trebouxiophyceae, Chlorophyta). Planta, 243(1), 161–169.
- Hoham, R. W., & Duval, B. (2001). Microbial ecology of snow and freshwater ice with emphasis on snow algae. In H. G. Jones, J. W. Pomeroy, D. A. Walker, & R. W. Hoham (Eds.), Snow ecology: An interdisciplinary examination of snow-covered ecosystems (pp. 168–228). Cambridge, U.K.: Cambridge University Press.
- Hotter, V., Glaser, K., Hartmann, A., Ganzera, M., & Karsten, U. (2018). Polyols and UV-sunscreens in the Prasiola -clade (Trebouxiophyceae, Chlorophyta) as metabolites for stress response and chemotaxonomy. Journal of Phycology, 54(2), 264–274.
- Hulatt, C. J., Berecz, O., Egeland, E. S., Wijffels, R. H., & Kiron, V. (2017). Polar snow algae as a valuable source of lipids? Bioresource Technology, 235, 338–347.
- Hulatt, C. J., & Thomas, D. N. (2011). Productivity, Carbon Dioxide Uptake and Net Energy Return of Microalgal Bubble Column Photobioreactors. Bioresource Technology, 102(10), 5775.
- Inventory of Existing Cosmetic Ingredients in China – IECIC. (2014). China food and drug administration (CFDA). Retrieved from http://www.cirs-reach.com/Cosmetic_Inventory/China_IECIC_Inventory_of_Existing_Cosmetic_Ingredients_in_China.html.
- Jorde, F., Leya, T., Pereira, S., Badenes, S. M., Santos, E., Costa, L., … Kryvenda, A. (2017). Benefits of the Algae Crop Rotation principle in algal mass production. In: 11th international phycological congress (p.13). Szczecin, Poland. 19.09.2017.
- J-P, D. V., Alawi, M., Backhaus, T., Baqué, M., Billi, D., Böttger, U., … Zucconi, L. (2019). Limits of life and the habitability of Mars: The ESA space experiment BIOMEX on the ISS. Astrobiology, 19(2), 145–157.
- Karsten, U., Friedl, T., Schumann, R., Hoyer, K., & Lembcke, S. (2005). Mycosporine-like amino acids and phylogenies in green algae: Prasiola and its relatives from the Trebouxiophyceae (Chlorophyta). Journal of Phycology, 41(3), 557–566.
- Kawahara, H., Higa, S., Tatsukawa, H., & Obata, H. (2009). Cryoprotection and cryosterilization effects of type I antifreeze protein on E. coli cells. Biocontrol Science, 14(2), 49–54.
- Koshimoto, C., & Mazur, P. (2002). Effects of warming rate, temperature, and antifreeze proteins on the survival of mouse spermatozoa frozen at an optimal rate. Cryobiology, 45(1), 49–59.
- Kreische, M. (2014) Einfluss von Temperatur und Nitratverfügbarkeit auf die Bildung von EPA und anderen Fettsäuren der arktischen Süßwasseralge Hormidiospora verrucosa CCCryo 198-05 (Bachelor Thesis). Brandenburgische Technische Universität Cottbus-Senftenberg, Senftenberg, Germany.
- Ladrière, O., Compère, P., Decloux, N., Vandewalle, P., & Poulicek, M. (2008). Morphological alterations of zooxanthellae in bleached cnidarian hosts. Cahiers De Biologie Marine. doi:10.21411/CBM.A.DCAE14FF
- Lee, Y.-K., & Soh, C.-W. (1991). Accumulation of astaxanthin in Haematococcus lacustris (Chlorophyta). Journal of Phycology, 27(5), 575–577.
- Lenz, E. (2015). Einfluss von Eisen und Phosphat auf die Produktion von Eicosapentaensaure durch ein Isolat der Alge cf. Hormidiospora verrucosa aus Spitzbergen. Berlin (Germany: Technische Universitat Berlin.
- Leya, T. (2003). Feldstudien und genetische Untersuchungen zur Kryophilie der Schneealgen Nordwestspitzbergens (Doktorarbeit) Humboldt-Universität zu Berlin, Berlin - published by Shaker Verlag, Aachen (2004), ISBN 3-8322-2438-6.
- Leya, T. (2004). Feldstudien und genetische Untersuchungen zur Kryophilie der Schneealgen Nordwestspitzbergens. In Berichte aus der Biologie (pp. 145). Aachen: Shaker. Retrieved from https://www.shaker.de/de/content/catalogue/index.asp?lang=de&ID=8&ISBN=978-3-8322-2438-7
- Leya, T. (2013). Snow Algae: Adaptation strategies to survive on snow and ice. In J. Seckbach, A. Oren, & H. Stan-Lotter (Eds.), Polyextremophiles: Life under multiple forms of stress, vol 27. Cellular origin, life in extreme habitats and astrobiology (pp. 401–423). Dordrecht: Springer Netherlands. doi:10.1007/978-94-007-6488-0_17
- Leya, T. (2018). Psychrophilic snow algae: Model organisms for drought and salt stress. In Workshop “C. merolae as an emerging model organism”. Berlin, Germany. 08.-09.10.2018.
- Leya, T., Müller, T., Ling, H. U., & Fuhr, G. (2001). Psychrophilic microalgae from north-west spitsbergen, svalbard: Their taxonomy, ecology and preliminary studies of their cold adaptation using single cell electrorotation. Nova Hedwigia, Beiheft, 123, 551–570.
- Leya, T., Müller, T., Ling, H. U., & Fuhr, G. R. (2004). Snow algae from North-Western Spitsbergen (Svalbard). In Alfred-Wegener-Institut für Polar- und Meeresforschung (AWI-Bremerhaven) (Ed.), The coastal ecosystem of Kongsfjorden, Svalbard. Synopsis of biological research performed at the Koldewey Station in the years 1991-2003 (pp. 46–54). AWI-Bremerhaven, Bremerhaven,: Alfred-Wegener-Institut für Polar- und Meeresforschung.
- Leya, T., Rahn, A., Lãtz, C., & Remias, D. (2009). Response of arctic snow and permafrost algae to high light and nitrogen stress by changes in pigment composition and applied aspects for biotechnology. FEMS Microbiology Ecology, 67(3), 432–443.
- Li, J., Yang, D., Li, L., Jabeen, K., & Shi, H. (2015). Microplastics in commercial bivalves from China. Environmental Pollution, 207, 190–195.
- Lorenz, R. T., & Cysewski, G. R. (2000). Commercial potential for Haematococcus microalgae as a natural source of astaxanthin. Trends in Biotechnology, 18(4), 160–167.
- Lutz, S., Procházková, L., Benning, L. G., Nedbalová, L., & Remias, D. (2019). Evaluating amplicon high-throughput sequencing data of microalgae living in melting snow: Improvements and limitations. Fottea, 19(2), 115–131.
- Margesin, R., & Schinner, F. (Eds). (1999). Biotechnological applications of cold-adapted organisms. Berlin: Springer.
- Martínez-Páramo, S., Barbosa, V., Pérez-Cerezales, S., Robles, V., & Herráez, M. P. (2009). Cryoprotective effects of antifreeze proteins delivered into zebrafish embryos. Cryobiology, 58(2), 128–133.
- Matsumoto, S., Matsusita, M., Morita, T., Kamachi, H., Tsukiyama, S., Furukawa, Y., … Todo, S. (2006). Effects of synthetic antifreeze glycoprotein analogue on islet cell survival and function during cryopreservation. Cryobiology, 52(1), 90–98.
- Menasveta, P., Worawattanamateekul, W., Latscha, T., & Clark, J. S. (1993). Correction of black tiger prawn (Penaeus monodon Fabricius) coloration by astaxanthin. Aquacultural Engineering, 12(4), 203–213.
- Miyamoto, K. T., Komatsu, M., & Ikeda, H. (2014). Discovery of gene cluster for mycosporine-like amino acid biosynthesis from Actinomycetales microorganisms and production of a novel mycosporine-like amino acid by heterologous expression. Applied and Environmental Microbiology, 80(16), 5028.
- Morita, R. Y. (1975). Psychrophilic bacteria. Bacteriological Reviews, 39(2), 144–167.
- Oren, A., & Gunde-Cimerman, N. (2007). Mycosporines and mycosporine-like amino acids: UV protectants or multipurpose secondary metabolites? FEMS Microbiology Letters, 269(1), 1–10.
- Pawella, L. (2008) Differentielle Proteomanalyse löslicher und membranständiger Proteine psychrophiler Schneealgen mittels 2D-SDS-PAGE (Diploma Thesis). Technische Universität, Berlin, Berlin, Germany.
- Petasch, J. (2008). Gefrierschutzsubstanzen in Schneealgen (Bachelor Thesis). Universität Potsdam, Potsdam, Germany.
- Procházková, L., Leya, T., Křížková, H., & Nedbalová, L. (2019). Sanguina nivaloides and Sanguina aurantia gen. et spp. nov. (Chlorophyta): The taxonomy, phylogeny, biogeography and ecology of two newly recognised algae causing red and orange snow. FEMS Microbiology Ecology, 95(6). doi:10.1093/femsec/fiz064
- PUFAChain Consortium (2017) The value chain from microalgae to PUFA, project acronym: PUFAChain, grant no. 613303. FP7 Programme. Brussels: European Commission. Retrieved from https://cordis.europa.eu/project/rcn/110872
- Remias, D., Karsten, U., Lütz, C., & Leya, T. (2010). Physiological and morphological processes in the Alpine snow alga Chloromonas nivalis (Chlorophyceae) during cyst formation. Protoplasma, 243(1–4), 73–86.
- Remias, D., Schwaiger, S., Aigner, S., Leya, T., Stuppner, H., & Lütz, C. (2012). Characterization of an UV- and VIS-absorbing, purpurogallin-derived secondary pigment new to algae and highly abundant in Mesotaenium berggrenii (Zygnematophyceae, Chlorophyta), an extremophyte living on glaciers. FEMS Microbiology Ecology, 79(3), 638–648.
- Remias, D., Wastian, H., Lütz, C., & Leya, T. (2013). Insights into the biology and phylogeny of Chloromonas polyptera (Chlorophyta), an alga causing orange snow in maritime Antarctica. Antarctic Science, 25(5), 648–656.
- Richa, R. R. P., Kumari, S., Singh, K. L., Kannaujiya, V. K., Singh, G., Kesheri, M., & Sinha, R. P. (2011). Biotechnological potential of mycosporine-like amino acids and phycobiliproteins of cyanobacterial origin. Journal of Biotechnology, Bioinformatics and Bioengineering, 1(2), 159–171.
- Rode, S. (2013) Einfluss von Ice Structuring Proteins auf die Kryokonservierung von Algen. Potsdam, Germany: Schwerpunktpraktikum, Universität Potsdam.
- Ross, J. (1820/1819). Entdeckungsreise unter den Befehlen der Britischen Admiralität mit den Königlichen Schiffen Isabella und Alexander um Baffins-Bay auszuforschen und die Möglichkeit einer nordwestlichen Durchfahrt zu untersuchen. In P. A. Nemnich (Ed.), Friedrich fleischer (pp. 74–76 (i.a.)). Leipzig: Friedrich Fleischer.
- Russell, N. J., Harrisson, P., Johnston, I. A., Jaenicke, R., Zuber, M., Franks, F., & Wynn-Williams, D. (1990). Cold adaptation of microorganisms. Philosophical Transactions of the Royal Society B: Biological Sciences, 326(1237), 595–611.
- Schirinzi, G. F., Pérez-Pomeda, I., Sanchís, J., Rossini, C., Farré, M., & Barceló, D. (2017). Cytotoxic effects of commonly used nanomaterials and microplastics on cerebral and epithelial human cells. Environmental Research, 159, 579–587.
- Schulze, L. (2011). Reihenuntersuchung von Schnee- und Permafrostalgen auf die Bildung von Fettsäuren unter Stickstoffmangel. Berlin, Germany: Forschungsarbeit, Beuth Hochschule für Technik Berlin.
- Sinha, R. P., Singh, S. P., & Häder, D.-P. (2007). Database on mycosporines and mycosporine-like amino acids (MAAs) in fungi, cyanobacteria, macroalgae, phytoplankton and animals. Journal of Photochemistry and Photobiology B: Biology, 89(1), 29–35.
- Speth, J. (2010) Aktivität eisstrukturierender Proteine (ISP) ausgewählter Schneealgen - Analyse der Stabilität isolierter ISP aus Chlamydomonas pseudopulsatilla (Bachelor Thesis). Beuth Hochschule für Technik, Berlin, Germany.
- Spijkerman, E., Wacker, A., Weithoff, G., & Leya, T. (2012). Elemental and fatty acid composition of snow algae in Arctic habitats. Frontiers in Microbiology, 3, 380.
- Tang, T.-F., Liu, X.-M., Ling, M., Lai, F., Zhang, L., Zhou, Y.-H., & Sun, -R.-R. (2013). Constituents of the essential oil and fatty acid from Malania oleifera. Industrial Crops and Products, 43, 1–5.
- Varshney, P., Mikulic, P., Vonshak, A., Beardall, J., & Wangikar, P. P. (2015). Extremophilic micro-algae and their potential contribution in biotechnology. Bioresource Technology, 184, 363–372.
- Verkley, G., Martin, D., & Smith, D. (2016, May). MIRRI Best practice manual on access and benefit sharing. Braunschweig, Germany: Leibniz-Institut DSMZ.
- Yaish, M. W. F., Doxey, A. C., McConkey, B. J., Moffatt, B. A., & Griffith, M. (2006). Cold-active winter rye glucanases with ice-binding capacity. Plant Physiology, 141(4), 1459–1472.
- Zacke, T. (2007). Untersuchungen zu Aktivitätsmaxima von Enzymen aus Schneealgen (Diploma Thesis). Humboldt-Universität zu Berlin, Berlin, Germany.