ABSTRACT
Urea formaldehyde (UF) was modified by the soybean protein hydrolysate (SPH) to adjust the rate of nitrogen, phosphorus, and potassium (NPK) release. The SPH section can copolymerize into the UF molecules. The crystallinity of modified UF fertilizer decreased with increasing SPH content. The surface of SPH-modified UF fertilizer was loose and porous. The nitrogen content of SPH-modified UF fertilizer decreased a little, but the nitrogen, phosphorus and potassium release rate was improved with increasing SPH content in modified fertilizer. The grain number per ear, thousand-grain weight and total yield were improved by the SPH-modified UF fertilizer. Compared to UF and control, protein, starch, fat, soluble sugar and vitamin C in the maize were increased by applying SPH-modified UF fertilizer. The results indicated that the nutrient release behavior can be adjusted by the introduced SPH to match the maize needs.
1. Introduction
Urea formaldehyde (UF) slow release fertilizer is condensation product formed by the reaction of urea and formaldehyde, which release of nitrogen mainly occurs by the enzyme attack [Citation1–Citation4]. It is particularly utilized in flowers, decorative plant, lawn fertilization, and in high-value cash crops and vegetables [Citation5].
However, the nitrogen release rate of the low molecular weight in UF fertilizer is higher than the crops required at the initial stage of growth, whereas the nitrogen release rate of the high molecular weight fraction is in slower rate than the crops required during the later stage of growth [Citation5]. It is for this reason that quantity demanded of UF slow release fertilizer declined. Therefore, it is necessary to change the molecular structure of urea-formaldehyde to match the crop needs [Citation6].
As is known, the control of the structure and its molecular weight of UF fertilizer are extremely difficult by regulating pH, temperature and urea/formaldehyde molar ratio. Soybean protein (SP) are available from renewable resources, which can ensure future sustainability. The impact on the natural environment is low during the growing, processing and utilization procedure [Citation7]. It has been reported that the application of soybean protein hydrolysate (SPH) and its degraded product can improve the quantity and activity of microorganisms in soil and increase crop yields [Citation8,Citation9].
SP is compact molecules, possessing primary, secondary, tertiary and quaternary structures, which is able to be unfolded by physical, chemical, or enzymatic methods [Citation10–Citation14]. The unfolded SP has the specific groups, such as primary amine, secondary amine, guanidyl, indolyl, etc. The formed groups in SPH can react with formaldehyde [Citation15,Citation16]. Therefore, urea can be partially substituted by SPH.
In this study, the SPH was applied to modify UF fertilizer via copolymerization process to adjust the rate of nutrient release. SPH was chemical modified by potassium hydroxide solution to unfold the quaternary structure and expose the reactive groups. The SPH was applied to react with formaldehyde and synthesis SPH-UF diblock copolymer to avoid the formation of high molecular weight urea-formaldehyde. The structure of the SPH-modified UF fertilizer was characterized by Fourier transform infrared spectroscopy (FTIR), thermal gravity (TG), X-ray diffraction (XRD) and scanning electron microscope (SEM). The nutrient release rate of the SPH-modified UF fertilizer in soil was also measured. The effects of SPH on the properties of SPH-modified UF fertilizers were analyzed, and a release mechanism was also proposed. The effect of SPH-modified UF fertilizer on the growth of maize was characterized.
2. Experimental
2.1. Materials
SP was purchased from Anyang Detianli food Co., Ltd, Henan Province, China. Urea (≥99.0%) was purchased from Beijing Chemical Plant. Potassium hydroxide (≥96.0%) and phosphoric acid (≥85.0%) were purchased from Tianjin Jinke Fine Chemical Research Institute, China. Formaldehyde (37.5 %) was purchased from Shantou Xilong Chemical Factory, Guangdong, China. All reagents and chemicals used in this study were of analytical grade and used without further purification.
2.2. Methods
2.2.1. The preparation of SPH
SPH was chemical modified with potassium hydroxide aqueous solutions (0.56 wt %) at 90°C for 1 h at a mixing speed of 500 rpm (solids content 13.04%).
2.2.2. The reactivity with formaldehyde
SPH (100 g) and formaldehyde (50 g) were added in a sealed flask and stirred at 40°C for 2 h. The remaining formaldehyde was measured according to a modified sodium sulfite method [Citation17].
2.2.3. The preparation of SPH-modified UF fertilizer
The SPH-modified UF fertilizer was synthesized in the followings:
Step I: methylolation. 37% aqueous formaldehyde solution, urea and SPH were added into a flask. The resulting solution was stirred at 40°C for 1.5 h. The pH of the solution was adjusted to 7.6 using potassium hydroxide aqueous solution (30 wt%).
Step II: polycondensation. Then the pH of the solution was adjusted to 4.5–5.0 by phosphoric acid aqueous solution (25 wt%), and stirred for 1.5 h. Then the obtained white turbid solution was filtered and dried at 60°C.
The material formulations of SPH-modified UF fertilizer is listed in . The possible mechanism is proposed in . The SPH-modified UF is a mixture of various length of molecular chains.
Table 1. The material formulations of UF and SPH-modified UF fertilizers.
2.2.4. Alkaline protease hydrolysis
The II fertilizers were washed with phosphate buffer solution (pH 8.0) at 55°C. Then, about 0.5 g fertilizers and 25 mg alkaline protease were added to 15 mL phosphate buffer solution (pH 8.0), and reacted at 55°C. The weight loss of the original and enzyme hydrolyzed samples was measured with an analytical balance.
2.2.5. Nutrient release rate of the fertilizers in soil
The soil columns were made of acrylic cylinders with 10.5 cm in diameter and 30 cm in height, and the bottom of the columns were covered with a 200 nylon mesh screen to prevent soil loss. Soil samples were placed at room temperature for 2 weeks. SPH-modified UF fertilizer was well mixed with dry soil (1 g N per 1 Kg dry soil). Then the soil mixed with and without fertilizer was kept in soil columns. After that, water was added to the columns until saturation. Throughout the experiment, the soil was incubated at 30°C. Three replications were done for each fertilizer. The columns were flushed with 200 mL deionized water at 0, 1, 2, …, 9 weeks. And, the leachate samples were collected from the outlet at the bottom of the columns. We minus the nutrient existed in the control sample. After that, the nitrogen content was estimated by the Kjeldahl method [Citation18]. The phosphate was determined by spectrophotometry. The potassium was characterized by flame photometry.
2.2.6 Trial layout and treatments
A field experiment was carried out in Jiangsu Academy of Agricultural Sciences, China (32°2ʹ northern latitude, 118°52ʹ east longitude) from April to September. There were five treatments: 1 (I), 2 (II), 3 (III), 4 (UF), 5 (a control without fertilizer). All fertilizers were applied when sow the seeds. And the four treatment received the same amount of N (140 kg/ha), P (70 kg/ha) and K (125 kg/ha). The soil and plantanalyzer development (SPAD) units for 10 plants per plot were measured throughout the growing season using a SPAD-502 Plus manual leaf chlorophyll meter.
2.2.7 Maize yield measurement
Grain yield was measured by harvesting two central rows (1.4 m × 10 m). Five plants were selected at random in the harvested plants, and then divided into grains and stover, and weighed. The samples were put into oven to deactivate enzymes at 105°C for 30 min, and then oven-dried at 80°C for 72 h to determine dry matter yield.
2.2.8 Maize quality measurement
The content of protein was measured by a K9840 Kjeldahl Analyzer (Jinan Hanon instrument co. LTD, China). Water soluble sugar was determined using the gravimetric Fehling’s method [Citation19]. Anthrone colorimetry method was applied to measure the contents of starch. Vitamin C was analyzed by the 2, 6-dichlorophenolindophenol titrimetric method [Citation20].
2.2.9 Statistical analysis
Data were analyzed with one-way ANOVA with fertilizer type as one factor using the SPSS software (Version 17.0). The least significant difference (LSD) was calculated to compare the differences between means in each treatment. Values are means ±SD of three replicates.
2.3. Measurement of SPH-modified UF fertilizers
The samples were characterized by FTIR spectrophotometer (Nicolet iS10, Thermo Scientific, America).
Thermal properties of fertilizers were characterized by a SII-7200 (Japan). And the temperature was in the range of 50–600°C.
The XRD patterns of fertilizers were performed with a Bruker D8 Advance diffractometer (Germany) with Cu Kα radiation (λ = 1.5418 Å) at room temperature.
A Quanta-200 SEM (Czech Republic) was used to obtain micrographs of the fertilizer granules.
3. Results
3.1. The preparation of soybean protein hydrolysate and its reactivity with formaldehyde
After SPH was chemical modified with potassium hydroxide, the spatial structure of SP was partially disrupted. And the reactive groups that are able to react with HCHO are exposed after hydrolysis [Citation15,Citation16]. The reactivity of SPH with formaldehyde is 21 mg HCHO per 1 g SPH.
3.2. FTIR of soybean protein before and after hydrolysis
The FTIR spectra of SP before and after hydrolysis are shown in . The peak at around 3274 cm−1 is attributed to the hydrogen bond association among molecules of SPHs, and hydroxyl group, amine stretching of the SPHs [Citation21–Citation23]. The peaks of C-C and C-O at 1238 cm−1 and 1073 cm−1 move to 1244 cm−1 and 1103 cm−1. The C-H off-plane coiling vibration band is observed at 979 cm−1. The occurrence peak at 979 cm−1 suggests that SP structure is unfolded after KOH hydrolysis [Citation15,Citation16]. SPH still possess original structure, β-sheets, on the basis of the amide I (1,605–1,640 cm−1) [Citation24].
3.3. ATR-FTIR spectra of unmodified and modified UF fertilizers
shows the ATR-FTIR spectra of unmodified UF and SPH modified UF fertilizers. It is noticeable that the spectra of the SPH-modified UF fertilizers are high similarity with that of the unmodified UF fertilizer. The reason is that the SPH and HCHO form the same functional groups as UF (). In addition, the functional groups of SPH [NH2CHR1CO(NHCHR2CO)nNHCHR3COOH] are also nearly the same as UF. The peak at around 3328 cm−1 is attributed to the hydrogen bond association among molecules, and hydroxyl group, amine stretching [Citation15,Citation16]. The absorption peak of C-H is measured at 2969 cm−1. The absorption band at 1621 cm−1 is due to the -C=O stretching vibration. The absorption band of the N-H is observed at 1550 cm−1 [Citation15,Citation16]. The absorption peak of the -CH2- is seen at 1439 cm−1. And the absorption of -NH2- is observed at 1388 cm−1. The absorbance of the -C-N- signals is measured at 1244 and 1137 cm−1. The absorption band at 1033 cm−1 is assigned to the C-O [Citation25]. The absorption peak at 3328 cm−1 become sharper with the increasing amount of SPH, which means that strong interaction is formed between SPH and UF molecular chains. The characteristic absorption peaks of the SPH-modified UF fertilizers are the same as those of unmodified UF fertilizers. The results illustrate that SPH is able to incorporate in the molecules of UF and form the block copolymer (-SPH-UF-). The result also can be proved by alkaline protease hydrolysis.
3.4. Alkaline protease hydrolysis
In order to demonstrate that the block copolymer (-SPH-UF-) is formed, the alkaline protease is applied to hydrolyze the SPH-modified UF fertilizers. The mass loss of different fertilizers hydrolyzed with alkaline protease as a function of time is shown in . The maximum mass loss of SPH-modified UF fertilizers treatment with and without alkaline protease was 18.51% and 1.62%, respectively. While, the maximum weight loss of UF hydrolyzed with and without alkaline protease was 1.75% and 1.47%, respectively. The theoretical percentage of SPH in II fertilizers is 10.18%. The mass loss of modified UF is more than the theoretical percentage of SPH from modified fertilizers after alkaline protease hydrolysis.
3.5. XRD characterization of different fertilizers
The XRD patterns of different fertilizers are presented in . It can be seen that the crystallization peaks of UF and SPH-UF are located at 22.5°, 24.7° and 31.8°. That is to say that the fertilizers before and after modification have a low degree of crystallinity because of the hydrogen bonding among molecules [Citation26,Citation27]. This result is in accordance with previous studies [Citation28,Citation22,Citation23]. In addition, the peaks of crystalline region of SPH-modified UF fertilizers decrease with increasing amount of SPH. The reason is that the order degree of UF molecules is disturbed by the blocked SPH. And the hydrogen intermolecular bonds among UF are weakened by the large molecules of SPH. The more SPH is introduced, the more crystalline regions of UF are destroyed.
3.6. Thermal analysis of UF and SPH-modified UF fertilizers
shows the thermal stability curves of the fertilizers. The charred residue of UF fertilizer is higher than that of modified UF fertilizers above 400°C. Moreover, the higher mole ratio of U/F in SPH-modified UF fertilizer contributes to the fewer residues. In other words, the SPH-modified UF fertilizers with higher amount of SPH possess less charred residues. In DTG curves, the peak of UF is sharper than that of modified UF. The temperature of the endothermic peak of UF, I, II and III is 275.1, 292.3, 292.4 and 295.5°C, respectively, which is due to the thermal decomposition of the methylenediurea in fertilizers [Citation29]. The endothermic peak temperature of modified UF fertilizer moves to higher temperature because of the introduced SPH. The area of endothermic peak for modified UF fertilizer become larger.
3.7. Morphology of UF and SPH-modified UF fertilizers
The surface morphologies of the unmodified and modified UF fertilizers are shown in . The SPH-modified UF fertilizer possess a more loose and porous surface than that of unmodified UF. And the pores of modified UF are in the size range of 0.3–2.5 μm. The interconnected channels are existed, which structurally increase the surface area of modified UF fertilizer. Therefore, water can be absorbed and preserved easily by the SPH-modified UF fertilizer.
3.8. The slow release behavior of UF and SPH-modified UF fertilizers in soil
The nitrogen content of UF, I, II and III fertilizers is 37.2%, 34.8%, 32.6% and 30.5%, respectively. Compared with UF, the nitrogen content of SPH-modified UF fertilizers decreases a little. The reason is that the nitrogen content of SPH is 14.1%, which is lower than that of UF. Activity index (AI) of UF is 41%, which is determined according to AOAC method [Citation18].
One of the most important characteristics of SPH-modified UF fertilizer is its slow-release properties. The release rate of different fertilizers in soil is monitored and the slow release behavior is shown in . The rate of nitrogen release from modified UF fertilizer decreases compared with the unmodified UF fertilizer within 3 weeks (). In addition, the nitrogen release rate of modified UF fertilizers decreases with an increase in the amount of SPH. The potassium and phosphorus release rate show similar trends (). The reason is the low molecular weight UF that degraded in the early stage copolymerize with SPH, which reduce the degradation rate at the initial stage of growth. And more and more low molecular weight UF react with SPH as the SPH content increases. The low molecular UF in SPH-modified UF fertilizer is degraded slowly because the intermolecular forces increase and the surface area to contact with enzymes decreases. Then potassium and phosphorus was released with the degradation of modified UF. However, the release rate of the SPH modified UF fertilizer is higher from 3 weeks to the end of the experiment. Moreover, the nitrogen release rate of modified UF fertilizers increases with the increasing amount of SPH. The nitrogen in I, II, III and UF fertilizer have released out 69.9%, 78.1%, 84.9% and 61.6% within 9 weeks, respectively.
Figure 7. (a) The N release behavior of different fertilizers (I, II, III: the ratio of U/F was 1.3, 1.35, 1.4). (b) The P release behavior of different fertilizers (I, II, III: the ratio of U/F was 1.3, 1.35, 1.4). (c) The K release behavior of different fertilizers (I, II, III: the ratio of U/F was 1.3, 1.35, 1.4).
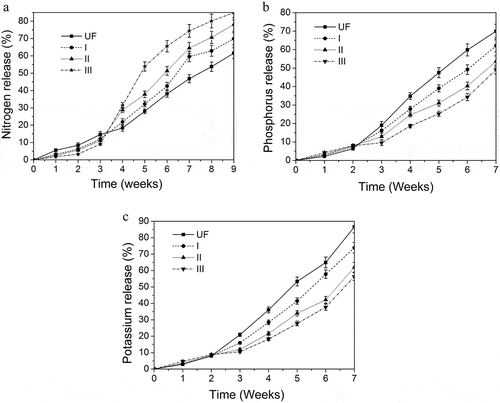
3.9. Effects of different fertilizer treatments on SPAD index of maize leaves
Average SPAD units varied at different growth stages and followed a similar trend to grain yield (). Compared to control, the fertilizer treatments increased the SPAD index of maize. The SPAD index of maize leaves treated with modified UF fertilizers was higher than unmodified UF. The results had significant differences as compared with that of UF.
Table 2. Effects of different fertilizer treatments on SPAD index of maize leaves.
3.10. Effects of different fertilizer treatments on yield
The effect of fertilizer type on the maize yield was listed in . The grain number per ear, thousand-grain weight and total yield were improved by the SPH-modified UF fertilizer. Grains per ear play an important role in the yield. Significant differences in grains per ear and thousand-grain weight were obtained. And the modified fertilizer II can significantly increase the maize yield. Compared to UF, the total yield of I, II and III increased 4.55%, 10.14% and 2.83%, respectively. The grain number per ear and total yield of SPH-modified UF had significant differences as compared with that of UF. Except II, there is no significant difference in thousand-grain weight between SPH-modified UF and UF. The total yield between the UF and II reached statistically significant level. That is to say that the maize yield can be improved by applying SPH-modified UF fertilizer II.
Table 3. Effects of different fertilizer treatments on yield.
3.11. Effects of different fertilizer treatments on quality
Compared to UF and control, protein, starch, fat, soluble sugar and vitamin C in the maize are increased by applying SPH-modified UF fertilizer, especially the II show more obvious effect (). Treatment II improve protein, starch, fat, soluble sugar and vitamin C by 50.70%, 20.49%, 5.93%, 1.43% and 10.33% than UF. There is no significant difference in soluble sugar among different fertilizer treatment. While, protein, starch, fat and vitamin C between II and UF reached statistically significant level. In a word, the modified UF can improve the quality of maize. The reason maybe the nitrogen release rate can match the crop need.
Table 4. Effects of different fertilizer treatments on quality of maize.
4. Discussion
SPH was applied to regulate the nitrogen release rate of UF fertilizers. In order to obtain the active groups of SP, the three-dimensional structure is partially unfolded after hydrolysis. The exposed -NH2 groups are able to react with HCHO and form the methylol derivatives [Citation15,Citation16,Citation30]. Thereby, SPH segments have the potential to copolymerize into the UF molecules. The strong interaction formed between SPH and UF molecules and the same characteristic absorption peaks of modified UF and unmodified UF indicate that SPH-formaldehyde-urea block copolymer is formed. Moreover, the results of alkaline protease hydrolysis of the modified UF provide further evidence for the formation of covalent bond between SPH and UF resin (block copolymer -SPH-UF-). The mass loss of modified UF is more than the theoretical percentage of SPH from modified fertilizers after alkaline protease hydrolysis. The result indicates that SPH-modified UF fertilizers can be hydrolyzed by the alkaline protease. And the blocked UF come off from the modified fertilizers after the SPH molecules are attacked by alkaline protease. Moreover, the decomposition of UF is promoted by the degradation of SPH during the nitrogen release. Lower molecular weight UF is easier to be mineralized by the microorganisms.
The more SPH is added in the synthesis process, the lower crystallinity is obtained. The less-crystalline regions result in more accessibility of the enzyme to the substrate. The modified fertilizer with lower crystallinity can be degraded easily by organisms and transformed into source of nitrogen for crops. Then the nitrogen release period of modified UF is shortened, which is benefit to improve the utilization efficiency of nitrogen. The loose and porous of modified UF fertilizer can increase the water holding ability of soil, reduce water and nutrient loss, enhance the drought resistance of maize. In addition, the increased surface area provides more effective contact area for various enzymes.
The higher amount of SPH was added in the modified UF fertilizers, the less charred residues was obtained from thermal analysis. The reason is that the SPH form less residues when the temperature is higher than 400°C. Therefore, the SPH-modified UF fertilizers with higher amount of SPH possess less charred residues. Because the molecular weight of the SPH is higher than that of UF, the endothermic peak temperature of modified UF fertilizer moves to higher temperature. The area of endothermic peak for modified UF fertilizer become larger due to more methylene ether bridges existed in SPH-modified UF fertilizer [Citation15,Citation16]. The peak of UF is sharper than that of modified UF. The reason is that the molecular weight distribution of SPH is wide. A conclusion can be drawn that the chemical structure is altered because the wide molecular weight distribution of SPH is block copolymerized with UF moleculars.
The nitrogen release rate is lower than UF within first 3 weeks, and the rate is higher after 3 weeks. The reason is the low molecular weight UF that degraded in the early stage copolymerize with SPH, which reduce the degradation rate at the initial stage of growth. And more and more low molecular weight UF react with SPH as the SPH content increases. The low molecular UF in SPH-modified UF fertilizer is degraded slowly because the intermolecular forces increase and the surface area to contact with enzymes decreases. The nitrogen release mechanism of SPH-modified UF fertilizer is illustrated in . The SPH, protein peptide, is easily accessed and attacked by enzymes derived from microorganisms. The produced amino acid can be directly utilized by plant. The blocked SPH is firstly decomposed and then the dropped UF is mineralized simultaneously by microorganisms [Citation31,Citation32]. The molecular weight of remaining UF is lower, which is easily decomposed by the microorganisms [Citation33]. The more SPH is added, the more blocked UF is formed. And, the molecular of blocked UF is lower, which is easily decomposed by microorganisms. Therefore, the nitrogen release rate of SPH-modified UF is improved with increasing SPH content in modified fertilizer.
In addition, the carbohydrate of SPH in modified UF fertilizer can offer carbon nutrients to soil microorganisms. The degraded SPH is able to improve the amount and activity of microorganisms. The modified UF fertilizer can absorb more water due to the abundant hydroxyl groups in carbohydrate, the abundant pore structure and large specific surface area. The water and the abundant microorganisms are beneficial to decompose the fertilizer and transform into source of nitrogen for crops.
Fertilization improved SPAD index in comparison to control, and modified UF fertilizers increased more (). The nitrogen content can be monitored by SPAD index [Citation34]. The results show that the nitrogen release of modified UF is in accordance with the nutrient requirement characteristics of maize. The effects of different fertilizer treatments on maize yield were tested. The results showed that statistically significant level in total yield between the UF and II were obtained. That is to say the nutrient release rate of modified UF fertilizer II could better match the maize growth curve. In addition, modified UF treatment improve protein, starch, fat, soluble sugar and vitamin C compared with unmodified UF, which is in accordance with the results of SPAD index and yield ( ). The management of soil nutrient at sufficient level is important for maintaining high quality [Citation35–Citation37]. The blocked SPH and its degraded products can improve the quantity and activity of microorganisms in soil and increase crop yields [Citation8,Citation38,Citation39]. In summary, the release pattern of modified UF fertilizer can be regulated by SPH to match the maize needs. The products are inexpensive and eco-friendly, which could be widely used in agriculture and horticulture.
5. Conclusions
The SPH was applied to modify UF fertilizer via copolymerization process to adjust the rate of nitrogen release. The SPH segments copolymerized with urea and formaldehyde. Because of the introduced SPH, the crystallinity decreased and the endothermic peak temperature moved to higher temperature. The surface of SPH-modified UF fertilizers became loose and porous. The nitrogen content of SPH-modified UF fertilizers decreased a little, while the nitrogen, phosphorus, and potassium (NPK) release rate increased with the increasing amount of SPH in modified fertilizers. The release pattern of SPH-modified UF fertilizer can be regulated by SPH to match the crop needs. Both the maize yield and quality can be improved by the SPH-modified UF fertilizer. It was worth noting that SPH-modified UF fertilizer was expected to be applied in agriculture to improve the maize yield and alleviate the environment pollution.
Acknowledgments
This work has been financially supported by Regional collaborative innovation project (science and technology assistance Xinjiang project) (Grant No. 2018E02035); the National Natural Science Foundation of China (Grant No. 11605077); China scholarship council (Grant No. 201808320076).
Disclosure statement
No potential conflict of interest was reported by the authors.
Additional information
Funding
References
- Guo Y, Zhang M, Liu Z, et al. Modeling and optimizing the synthesis of urea-formaldehyde fertilizers and analyses of factors affecting these processes. Sci Rep. 2018;8:4504.
- Azeem B, KuShaari KZ, Man ZB, et al. Review on materials & methods to produce controlled release coated urea fertilizer. J Control Release. 2014;181:11–21.
- Timilsena YP, Adhikari R, Casey P, et al. Enhanced efficiency fertilisers: a review of formulation and nutrient release patterns. J Sci Food Agric. 2015;95:1131–1142.
- Giroto AS, Guimarães GGF, Ribeiro C. A novel, simple route to produce urea: urea–formaldehydecomposites for controlled release of fertilizers. J Polym Environ. 2017;6:1–11.
- Alexander A, Helm HU. Ureaform as a slow release fertilizer: a review. J Plant Nutr Soil Sci. 1990;153:249–255.
- Senna AM, Carmo JBD, Silva JMSD, et al. Synthesis, characterization and application of hydrogel derived from cellulose acetate as a substrate for slow-release NPK fertilizer and water retention in soil. J Environ Chem Eng. 2015;3:996–1002.
- Calabria L, Vieceli N, Bianchi O, et al. Soy protein isolate/poly (lactic acid) injection-molded biodegradable blends for slow release of fertilizers. Ind Crop Prod. 2012;36:41–46.
- Wang J, Liu Z, Wang Y, et al. Production of a water-soluble fertilizer containing amino acids by solid-state fermentation of soybean meal and evaluation of its efficacy on the rapeseed growth. J Biotechnol. 2014;187:34–42.
- Rosiane LSL, Liv SS, Ligia RS, et al. Blends of castor meal and castor husks for optimized use as organic fertilizer. Ind Crop Prod. 2011;33:364–368.
- Cui C, Zhao M, Yuan B, et al. Effect of pH and pepsin limited hydrolysis on the structure and functional properties of soybean protein hydrolysates. J Food Sci. 2013;78:1871–1877.
- Jong L. Influence of protein hydrolysis on the mechanical properties of natural rubber composites reinforced with soy protein particles. Ind Crop Prod. 2015;65:102–109.
- Liu D, Zhu C, Peng K, et al. Facile preparation of soy protein/poly (vinyl alcohol) blend fibers with high mechanical performance by wet-spinning. Ind Eng Chem Res. 2013;52:6177–6181.
- Orliac O, Rouilly A, Silvestre F, et al. Effects of various plasticizers on the mechanical properties, water resistance and aging of thermo-moulded films made from sunflower proteins. Ind Crop Prod. 2003;18:91–100.
- Mirmoghtadaie L, Aliabadi SS, Hosseini SM. Recent approaches in physical modification of protein functionality. Food Chem. 2016;199:619–627.
- Qu P, Huang H, Wu G, et al. Hydrolyzed soy protein isolates modified urea-formaldehyde resins as adhesives and its biodegradability. J Adhes Sci Technol. 2015;29:2381–2398.
- Qu P, Huang H, Wu G, et al. Effects of hydrolysis degree of soy protein isolate on the structure and performance of resin. J Appl Polym Sci. 2015. DOI:10.1002/app.41469
- Park BD, Jeong HW. Hydrolytic stability and crystallinity of cured urea-formaldehyde resin adhesives with different formaldehyde/urea mole ratios. Int J Adhes Adhes. 2011;31:524–529.
- Horwitz W. Official methods of analysis of AOAC International. 16th ed. New York: AOAC International; 1999.
- Williams S. Official methods of analysis of AOAC. 14th ed. New York: AOAC International; 1984. p. 830–886.
- Milošević T, Milošević N. Apple fruit quality, yield and leaf macronutrients content as affected by fertilizer treatment. J Soil Sci Plant Nut. 2015;15:76–83.
- Liu H, Li C, Sun XS. Improved water resistance in undecylenic acid (UA)-modified soy protein isolate (SPI)-based adhesives. Ind Crop Prod. 2015;74:577–584.
- Chen J, Chen X, Zhu Q, et al. Determination of the domain structure of the 7S and 11S globulins from soy proteins by XRD and FTIR. J Sci Food Agric. 2013;93:1687–1691.
- Chen XY, Chen C, Zhang ZJ, et al. Nitrogen-doped porous carbon prepared from urea formaldehyde resins by template carbonization method for supercapacitors. Ind Eng Chem Res. 2013;52:10181–10188.
- Chen L, Subirade M. Elaboration and characterization of soy/zein protein microspheres for controlled nutraceutical delivery. Biomacromolecules. 2009;10:3327–3334.
- Zhang YH, Gu JY, Tan HY, et al. Fabrication, performances, and reaction mechanism of urea–formaldehyde resin adhesive with isocyanate. J Adhes Sci Technol. 2013;27:2191–2203.
- Park BD, Causin V. Crystallinity and domain size of cured urea–formaldehyde resin adhesives with different formaldehyde/urea mole ratios. Eur Polym J. 2013;49:532–537.
- Yamamoto CF, Pereira EI, Mattoso LH, et al. Slow release fertilizers based on urea/urea–formaldehyde polymer nanocomposites. Chem Eng J. 2016;287:390–397.
- Despres A, Pizzi A. Colloidal aggregation of aminoplastic polycondensation resins: urea–formaldehyde versus melamine–formaldehyde and melamine–urea–formaldehyde resins. J Appl Polym Sci. 2006;100:1406–1412.
- Samaržija-Jovanović S, Jovanović V, Konstantinović S, et al. Thermal behavior of modified urea–formaldehyde resins. J Therm Anal Calorim. 2011;104:1159–1166.
- Bacigalupe A, Poliszuk AK, Eisenberg P, et al. Rheological behavior and bonding performance of an alkaline soy protein suspension. Int J Adhes Adhes. 2015;62:1–6.
- Pan P, Jiang HM, Zhang JF, et al. Shifts in soil bacterial communities induced by the controlled-release fertilizer coatings. J Integr Agric. 2016;15:2855–2864.
- Jahns T, Kaltwasser H. Mechanism of microbial degradation of slow-release fertilizers. J Polym Environ. 2000;8:11–16.
- Yang Z, Shi Y, Sun Y, et al. The study on biodegradation of methylene urea by activated sludge. Polym Degrad Stab. 2016;128:107–114.
- Berenguer P, Santiveri F, Boixadera J, et al. Nitrogen fertilisation of irrigated maize under Mediterranean conditions. Eur J Agron. 2009;30:163–171.
- Sobia N, Muhammad Y, Muhammad N, et al. Use of controlled release phosphatic fertilizer to improve growth, yield and phosphorus use efficiency of wheat crop. Pak J Agric Sci. 2017;54:541–547.
- Zhang JH, Huang B, Chen L, et al. Characteristics of biochar produced from yak manure at different pyrolysis temperatures and its effects on the yield and growth of highland barley. Chem Spec Bioavailab. 2018;30:57–67.
- Yu XJ, Tian XF, Lu YY, et al. Combined effects of straw-derived biochar and bio-based polymer-coated urea on nitrogen use efficiency and cotton yield. Chem Spec Bioavailab. 2018;30:112–122.
- Kunito T, Hiruta N, Miyagishi Y, et al. Changes in phosphorus fractions caused by increased microbial activity in forest soil in a short-term incubation study. Chem Spec Bioavailab. 2018;30:9–13.
- Lima RLS, Severino LS, Sampaio LR, et al. Blends of castor meal and castor husks for optimized use as organic fertilizer. Ind Crop Prod. 2011;33:364–368.