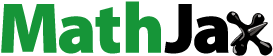
ABSTRACT
Selected three soil (yellow cinnamon soil, manual loessial soil and aeolian sandy soil) to study the kinetics and isotherms sorption of V(Ⅴ), and further explore the differences in the adsorption mechanism caused by initial V (V) concentration, ionic strength (IS), pH and different reaction times. It was observed that V(Ⅴ) adsorption onto the soil had three processes and completed rapid adsorption in 60, 100 and 120 min. The maximum adsorbing capacity of V(Ⅴ) on yellow cinnamon soil, manual loessial soil and aeolian sandy soil reached stable in pH range from 4 to 8. The adsorption capacity decreased with the increase of IS. V(Ⅴ) adsorption onto soil varied greatly with mineralogy and properties of soil, the surface area, soil organic carbon content and composition of particulate matter. This research enhanced the understanding of how soil properties affect vanadium (V) sorption onto agricultural soil.To investigate the reaction mechanism between vanadium (Ⅴ) and soil, and further explore the differences in the adsorption mechanism caused by initial V(V) concentration, ionic strength, pH and different reaction times. Selected three soil (yellow cinnamon soil, manual loessial soil and aeolian sandy soil) which are the typical agricultural soil from Shaanxi province to study the kinetics and isotherms sorption of V(Ⅴ). SEM study is used to probe the morphological features of the soil in the presence of vanadium and to investigate the change of surface functional groups on the three soils after adsorption by the FTIR spectra. It was observed that V(Ⅴ) adsorption onto the soil had three processes and completed rapid adsorption in 60 min, 100 min and 120 min. The maximum adsorption concentration of V(Ⅴ) derived from experimental data for the yellow cinnamon soil, manual loessial soil and aeolian sandy soil were 1124.6mg kg, 854.4mg kg and 748.6mg kg, respectively. The maximum adsorbing capacity of V(Ⅴ) on yellow cinnamon soil, manual loessial soil and aeolian sandy soil reached stable in the pH range from 4.0 to 8.0. The adsorption capacity decreased with the increase of ionic strength. V(Ⅴ) adsorption onto soil varied greatly with mineralogy and physical–chemical properties of soil, the BET surface area, soil organic carbon content and composition of particulate matter. This research enhanced the understanding of how soil properties affect vanadium (V) sorption onto agricultural soil.
KEYWORDS:
1. Introduction
Vanadium (V) is an indispensable trace mineral nutrient for the normal growth of human, animals and plants [Citation1]. However, V intake of more than 10 mg per day or foods containing 10–20 ug per gram V can cause toxic effects [Citation2]. In recent years, due to the large-scale industrial use of vanadium and the lack of thorough effluent treatment, wastewater containing vanadium is discharged to the environment. Continuous release of V can result in surface and groundwater contamination affecting biological functions of living organisms. Excessive Vanadium (V) in the human body can cause acute or chronic poisoning, damage to heart and kidney [Citation3]. Therefore, research on the mobility, toxicity and bioavailability of vanadium has remarkable significance to the environment and global health.
Vanadium predominantly exists in the divalent (V(II)) and pentavalent (V(Ⅴ)) forms. The V(II) and V(III) in the air are less common because of its susceptibility to oxidation [Citation4]. V(Ⅴ) mainly exists in the form of VO3− and can combine with other biological substances to form a complex that is easily absorbed by plants [Citation5]. Studies on the adsorption of vanadium(V) by different adsorbents manifested that the adsorption kinetics and isotherms submitted to second-order and Freundlich models, respectively [Citation6–Citation8]. Soil is a complex substrate containing inorganic and organic components that can act as adsorption sites for heavy metals. The factors involved in heavy metal adsorption in soils include clays, metal oxides, soil organic matter, soil pH, exchangeable cation concentration, metal speciation, metal concentration and ionic strength, etc., [Citation9–Citation12]. There is some study about the adsorption of V(Ⅴ) on soil content. For example, vanadium adsorption on natural soil colloids were pH-dependent with high capacity of 41.5–286 mg g−1 [Citation13], and clay minerals such as montmorillonite and kaolinite have low affinity for V(Ⅴ) with maximum adsorption capacity of 0.98 mg g−1 and 0.78 mg g−1, respectively [Citation14].It has been reported that V(Ⅴ) in a multi-element solution was less retained onto montmorillonite in trace concentrations (0.05 mg L−1) [Citation15]. The adsorption of V(Ⅴ) on goethite at pH 6–9 has a concentration dependence that mainly occurred by reflects the formation of polynuclear complexes in solution [Citation16]. Manohar et al. [Citation17] observed that adsorption of V(IV) was affected by the pH and ionic strength. Although previous studies have extensively examined the adsorption characteristic of vanadium on various adsorbents, the adsorption behavior research of V(Ⅴ) on the agricultural soil is not very comprehensive.
In China, vanadium ore resources are mainly stone, coal and vanadium–titanium magnetite. The extraction process of V ore produces wastewater containing vanadium dust and aerosol particles, which can pollute the surrounding soil, and bring health risks and potential harm to local residents. In the present study, we selected three soils which were the typical agricultural soil from Shaanxi province to study the kinetics and isotherms sorption of V(Ⅴ). The effects of ionic strength and pH with wide range (2–10) on the V(Ⅴ) adsorption were also examined. The kinetic characteristics of V(Ⅴ) adsorption were delineated using pseudo-first-order, pseudo-second-order, Elovich and the intra-particle diffusion models, and the isotherm adsorption was applied to various models such as Langmuir, Freundlich and Temkin. The consequences of this paper are very advantageous to predict vanadium transport and can be used to estimate the role of vanadium on the agricultural soil.
2. Materials and methods
2.1. Soil and reagents
3 soils were selected at the 0–20 cm depths from wheat fields in Shaanxi Province, China, including yellow cinnamon soil (Fengxian), manual loessial soil (Yangling) and aeolian sandy soil (Shenmu). The soils belonged to the major soil order Cambisol, Anthrosol and Arenosol, respectively (according to FAO/ISRIC/ISSS taxonomy [Citation18]). After removal of plant residues and air-drying, the soils were ground and screened using the 1 mm sieve.
Analytical grade reagents were used in this study. A vanadium standard reserve liquid of 1000 mg L−1 was purchased from the analysis and testing center of Non-ferrous metals and electronic materials (Beijing, China). Vanadium (NH4VO3) was purchased from Chengdu kelon chemical reagent factory (Chengdu, China). The HNO3, NaOH and NaNO3 were obtained from Guangdong Guanghua Chemical Factory Company, Ltd (Guangzhou, China).
2.2 Properties of soil
The Field Emission Scanning Electron Microscope (FESEM) (S-4800, Tokyo, Japan) was used to analyze the exterior surface morphology and elemental proportion of the three soils. Fourier transform infrared (FT-IR) spectrometer (Tensor27, Bruker, Germany) were used to analyze the soil surface functional groups. For this, the soils were ground with the KBr (1:100). To explore the crystallographic structures of the sample, X-ray diffraction (XRD) analysis was executed using a Powder X-ray Diffractometer (D/RAPID II, Rigaku, Japan) and the scan range was set at 2θ = 5°~90° with a scan rate of 8° min−1. The sample specific surface area was obtained by the BET analysis (ASAP 2000, Micromeritics, USA) with N2 gas adsorption.
2.3 Experiments methods
Sorption isotherm experiments of vanadium (V) on yellow cinnamon soil, manual loessial soil and aeolian sandy soil were performed using a range of concentrations of NH4VO3 (0, 2, 4, 8, 15, 25, 50, 100, 150, 200, 250 and 500 mg L−1). One gram of soil was added to 50 ml plastic centrifuge tubes with 25 ml vanadium (V) solution. Kinetic studies were carried out in room temperature (25°C) by adding 50 mg L−1 V solution and was analyzed for V after 5 min, 10 min, 20 min, 0.5 h, 1 h, 2 h, 4 h, 6 h, 8 h, 12 h, 20 h, 28 h, 36 h, and 48 h. The initial pH of the V(V) solution was adjusted to 2, 4, 6, 8 and 10 using 0.1 M HNO3 and 0.1 M NaOH. The effect of different ionic strength conditions was determined by using 0.1, 0.01 and 0.001 M NaNO3 as a supporting electrolyte. The liquid suspensions in centrifuge tubes were capped and shaken on an reciprocating oscillator with 170 r/min at 25°C for 24 h [Citation19,Citation20]. When the sorption reached the equilibration stage, the centrifuge tubes were centrifuged at 4000 r min−1 for about 10 min. The vanadium(V) concentrations in the supernatants were measured and recorded by the Flame atomic absorption (Z-2000, Japan) [Citation21]. The adsorbed amount of V(Ⅴ) was subsequently calculated by the difference between initially added V(Ⅴ) and final V(Ⅴ) concentration in solution. Each sample was duplicated.
2.4 Analysis methods
The adsorbed amount of vanadium(V) was determined on the basis of initially added and final vanadium(V) concentrations in the supernatants by the following equation:
where S stands for the amount adsorbed (mg kg−1), Co and Ce stand for the beginning and final V(V) concentrations (mg L−1), respectively.
Sorption kinetics was fitted by simulation models of Pseudo-first-order model [Citation22], Pseudo-second-order model [Citation23], Elovich model [Citation24]and intra-particle diffusion model [Citation25]. The adsorption isotherm is critical in determining the adsorption capacity of adsorbent and in optimizing the amount of adsorbent used. In this research, the following three equations were used to elucidate the experimental results.
Langmuir model [Citation26]
Freundlich model [Citation27]
Temkin model [Citation28]
where Se and St are the adsorbed amounts of metal (mg kg−1) at equilibrium and at time t (min). Sm is the maximum sorption amount (mg g−1). k1, k2, ki, KL, and KF are the equilibrium rate constants. The parameters a, b, c, n, aT and bT were the equation constant obtained from the slope and intercept of the trendline. The correspondingly high R2 value predicted which model better followed the V(Ⅴ) sorption. Statistical analysis of all data was conducted using Origin 8.5 and Excel 2010 for Windows.
3. Results and discussion
3.1 Soil characterization
The physicochemical properties of the yellow cinnamon soil, manual loessial soil and aeolian sandy soil are shown in . The BET surface area measurements acquired in accordance with N2 adsorption isotherm indicated that yellow cinnamon soil (24.791 m2 g−1) and manual loessial soil (16.756 m2 g−1) had higher surface area compared to that of aeolian sandy soil (4.075 m2 g−1). Consequently, more interlayer spaces of the yellow cinnamon soil and manual loessial soil were generated from the microporous system which may result in favorable adsorption sites to vanadium. Yellow cinnamon soil and manual loessial soil had a lower percentage of particulate matter in the 1.0–0.05 nm range (11.25% and 24.25%, respectively) compared to Aeolian sandy soil (83.94%) This may account for the differences in adsorption of V by these soils. An SEM study was used to probe the morphological features of the soil in the presence of vanadium (). Elemental analysis data indicated that the content of vanadium present was in lower amounts. The surface morphology and pore of yellow cinnamon soil, manual loessial soil and aeolian sandy soil were different. The yellow cinnamon soil and manual loessial soil appeared as irregular shaped crystals with a fluffy appearance, indicating higher adsorption capabilities. In comparison, aeolian sandy soil present relatively smooth surface.
Table 1. Basic physicochemical properties of the soil.
Figure 1. The SEM scanning of the yellow cinnamon soil (a, YS), manual loessial soil (b, MS) and aeolian sandy soil (c, AS).
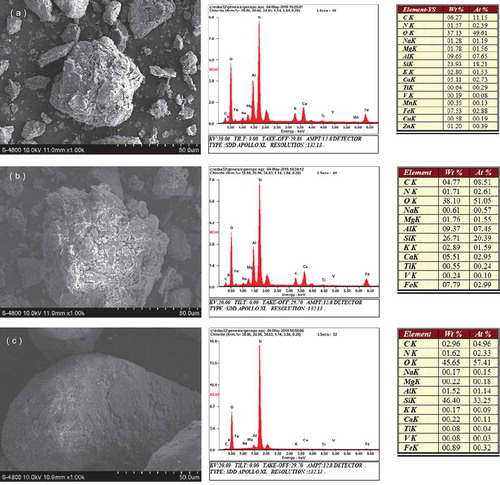
In order to further investigate the change of surface functional groups on the three soils after adsorption, the FTIR spectra from 4000 cm−1 to 500 cm−1 was measured (). The vibration of OH− was observed at 3441.43 cm−1 for yellow cinnamon soil [Citation29]. The bands at 1435 cm−1 was consistent with CH2 bending in pre- and post-sorption yellow cinnamon soil and manual loessial soil [Citation30]. Bands at 985.46 cm−1 represents the dissymmetric vibration of Si-O-(Al) and bands at 778 cm−1 and 992.69 cm−1 was due to the symmetry vibration and dissymmetric vibration of Si-O-Si [Citation31].
Figure 2. FTIR spectra and XRD diffractograms of the yellow cinnamon soil (YS), manual loessial soil (MS) and aeolian sandy soil (AS).
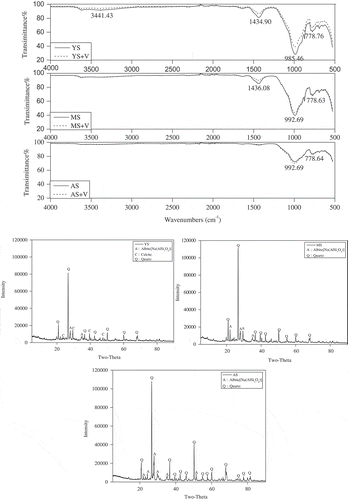
The consequence of elemental analysis and FT-IR were proved by analysis results of XRD shown in , which indicated that quartz and albite (Na (AlSi3O8)) were the main phase presented in the soil. In addition, the yellow cinnamon soil had the other mineral, calcite.
3.2 Adsorption kinetics
The fast adsorption stage of V by yellow cinnamon soil was from 0 to 240 min, which was similar to that of manual loessial soil and aeolian sandy soil (). Adsorption process of yellow cinnamon soil, manual loessial soil and aeolian sandy soil was mainly affected by the fast adsorption stage because the absorption of metavanadate mainly occurred in the first 4 h. Thereafter, another dynamic adsorption equilibrium phase was observed in the later stage since the active site of the soil was fully occupied. For example, about 37.2% adsorption amount of V(Ⅴ) by yellow cinnamon soil was completed in the first hour of reaction; however, only 7.4% of additional adsorption amount reacted in the remaining 23 h. The slower adsorption was because of the reduced concentration difference between the solution and the soil surface, due to which the amount of the V(Ⅴ) transported to the soil surface decreased. For the adsorption of V(Ⅴ) by manual loessial soil and aeolian sandy soil, a similar phenomenon was observed, however, the adsorption rate of the fast adsorption stage on other soils were achieved at 39.6% and 20.7% within 100 min and 120 min, respectively. At equilibrium, about 44.6%, 43.7% and 29.5% of the V(Ⅴ) were adsorbed by yellow cinnamon soil, manual loessial soil and aeolian sandy soil. On the other hand, it means about 55.4%, 56.3% and 70.5% of the V(Ⅴ) remained in solution, which may be the reason why the environmental quality standards of vanadium were based off the behavior of vanadium in the Shaanxi soils that has a comparatively higher concentration of V.
Figure 3. The adsorption kinetics model of V(Ⅴ) on the yellow cinnamon soil (a), manual loessial soil (b) and aeolian sandy soil (c) (initial V(Ⅴ) concentration of 50 mg L−1 and solution pH 7.0 ± 0.2).
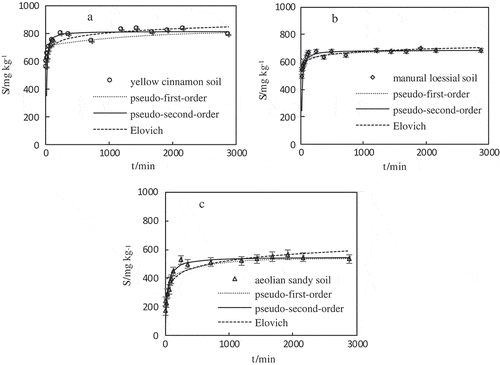
The kinetic data were simulated by several common kinetic models in . The linear regression coefficients (R2) of kinetic equations calculated for V(Ⅴ) adsorption onto the soil are shown in . According to the R2 values of kinetic equations, the pseudo-first-order equation fails to explain the kinetic data. In contrast, the kinetic result was well described by the Elovich and pseudo-second-order equations. The pseudo-second-order equation was observed to best describe the adsorption kinetic of V(Ⅴ) onto the three soils at 25°C. Similar observations were made in other studies where the pseudo-second-order equation was more suitable than other equations for the characterization of kinetic data of anionic adsorption onto soils or other materials [Citation32–Citation34]. The Elovich equation confirmed that chemisorption process is the main adsorption process in the studied sorption system. The trend followed by a and b were similar to the solution system that has been confirmed to be dominated by chemisorption, which showed that b had an available number of active adsorption sites [Citation35]. It was clear that the aeolian sandy soil (b = 0.26) had the least active adsorption sites in . In addition, the Pseudo-second-order model [Citation23] was applied to the dynamic data fitting, on the basis of hypothesis that the adsorption rate was controlled by the chemical adsorption mechanism and the adsorption rate was related to the number of activated sites on the adsorbent surface. Therefore, the V(Ⅴ) sorption onto yellow cinnamon soil, manual loessial soil and aeolian sandy soil were chemisorption at the water solution. In addition, in , parameters for pseudo-second-order kinetic equation were also presented. The pseudo-second-order reaction rate (K2) of yellow cinnamon soil, manual loessial soil and aeolian sandy soil were 0.1855 g mg−1 min−1, 0.1612 g mg−1 min−1 and 0.0649 g mg−1 min−1, respectively, which were in accordance with the change trend in .
Table 2. Parameters of kinetic models for the adsorption of V(Ⅴ) onto yellow cinnamon soil, manual loessial soil and aeolian sandy soil.
Diffusion mechanism played an important role in the overall adsorption rate of heavy metals, especially from the solid surface into the interior. Though the pseudo-second-order model could well fit the experimental result, the fitting results could not effectively explain the diffusion mechanism [Citation36,Citation37]. It was frequently noticed that the diffusion in the interior of the particles was the rate-governing step, and this ordinarily could be verified by using the intraparticle model equation. The kinetic data fitted by using the intraparticle diffusion model was showed in , which indicated that the V(Ⅴ) adsorption process of the three adsorbents had three successive steps. The results were consistent with the research of Roy et al. [Citation38], they pointed out that adsorption process was usually commanded by three steps: boundary film diffusion, intraparticle diffusion, and binding to the active site of the adsorbent. also presented that yellow cinnamon soil ranged from 0 to 1 h for the fast adsorption stage of V(Ⅴ), which was different from manual loessial soil and aeolian sandy soil within 100 min and 120 min.
Figure 4. The intraparticle diffusion model (a–c) and Boyd plots (d) of V(Ⅴ) sorption on the yellow cinnamon soil (YS), manual loessial soil (MS) and aeolian sandy soil (AS) (initial V(Ⅴ) concentration of 50 mg L−1 and solution pH 7.0 ± 0.2).
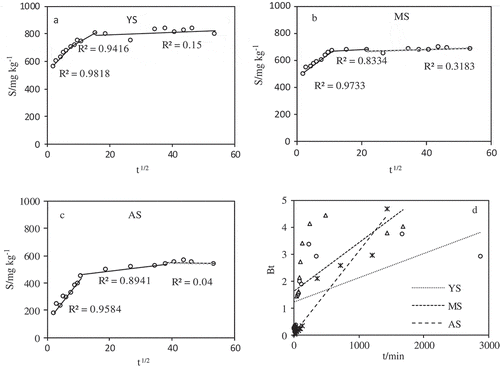
The diffusion of heavy metals in the adsorption process would be affected by the boundary layer resistance. Boyd and Webber models [Citation39] were widely used for estimating the main boundary layer resistance for soil particles, or pore diffusion resistance. The model [Citation40]is expressed as:
Bt is diffusivity constant, but each Bt value cannot be calculated by using the same method. So, the following approximation was obtained:
For Q > 0.85,
For Q < 0.85, Bt =
where Q is calculated from Q = St/Se. In ), if the plot of Bt against time is linear with zero intercept, then the rate of substance transfer was controlled by pore diffusion. If the intercept of plot is not zero, then it is indicated that the adsorption rate was controlled by film diffusion or site adsorption. Therefore, considering soil characterization data and , and it could be considered that the adsorption mechanism of V(Ⅴ) on yellow cinnamon soil and manual loessial soil was contributed by film-diffusion or chemical reaction which was different from that of aeolian sandy soil with pore-diffusion.
3.3 Isothermal adsorption
The fitting results of the experimental data for the three soils (), indicates that the adsorption behavior of vanadium on soil is completely different. Sorption of V onto yellow cinnamon soil, manual loessial soil and aeolian sandy soil increased with increasing concentration in solution, and the equilibrium curves showed a slow upward trend when the equilibrium concentration was close to 150 mg L−1. At the initial reaction stage of the equilibrium curve (below 100 mg L−1), sorption amounts of three soils were comparable and the isotherm could be grouped into L-type of Giles’ categories [Citation41]. At V(Ⅴ) concentrations more than 100 mg L−1, sorption amounts of V(Ⅴ) reached a stabile value with aeolian sandy soil followed by manual loessial soil and yellow cinnamon soil. On the other hand, the experimental consequences also revealed that the adsorption ratio of V(Ⅴ) decreased with increase in initial vanadium concentration. For instance, the adsorption ratio of yellow cinnamon soil decreased from 97.1% at 5.5 mg L−1 to 7.4% at 616.9 mg L−1 and correspondingly the adsorption amount increased from 133.6 mg kg−1 at 5.5 mg L−1 to 1147.4 mg kg−1 at 616.9 mg L−1. The reason for this was the presence of energetically less favorable sites that resulted in a decrease in V(Ⅴ) adsorption with increase in V(Ⅴ) concentration, while most of the V(Ⅴ) ions is easier to combine with the adsorption site at lower concentrations resulting in higher adsorption. The adsorption ratio of manual loessial soil range from 54.8% to 7.7%, while from 64.6% to 3.8% of aeolian sandy soil. The maximum adsorption amount of V(Ⅴ) on soil ranked as yellow cinnamon soil (1147.4 mg kg−1) > manual loessial soil (854.4 mg kg−1) > aeolian sandy soil (748.6 mg kg−1). This was because at the same initial concentration of V(Ⅴ) different surface morphology and physical-chemical properties including soil organic matter, clay and Fe/Al hydr(oxide) minerals could result in a large difference in the adsorption capacity among various soil [Citation42].
Figure 5. The adsorption ratio and isotherm models of V(Ⅴ) sorption on the yellow cinnamon soil (YS), manual loessial soil (MS) and aeolian sandy soil (AS) at solution pH 7.0 ± 0.2.
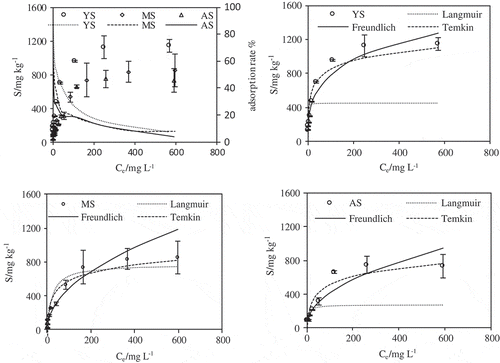
The analysis of equilibrium data played an important role in understanding the adsorption process and could compare the characteristics of different adsorbents through different experimental conditions. The equilibrium experimental data of V(Ⅴ) was analyzed commonly by the Langmuir (Eq.2), Freundlich (Eq.3) and Temkin (Eq.4) adsorption isotherm equations. The Langmuir isotherm model assumes a monolayer adsorbed on the surface and each molecule has the equal activation energy [Citation26,Citation43]. Freundlich model can well simulate multilayer adsorption on a heterogeneous adsorbent surface due to the difference of sorption sites or the different properties of the metal ions, such as free or hydrolyzed metal ions [Citation27]. The Temkin isotherm equation assumes that the heat of adsorption decreases linearly with the increase of molecular coverage of adsorbent surface because of adsorbent–adsorbate interactions and that the adsorption is characterized by a uniform distribution of the binding energies until the maximum binding energy was reached [Citation44].
The results of these three isotherm models simulated to the V(Ⅴ) adsorption on the yellow cinnamon soil, manual loessial soil and aeolian sandy soil were presented in . The parameters and correlation coefficients (R2) of these models were demonstrated in . From and , it could be concluded that three isotherm models could well fit the adsorption of V(Ⅴ) onto the manual loessial soil, and the Freundlich isotherm model was observed to yield the best fits to the experimental data of the yellow cinnamon soil, manual loessial soil and aeolian sandy soil due to the highest R2 value of 0.953, 0.947 and 0.923, respectively. The best fit of experimental data to the Freundlich isotherm indicated that the binding site with higher activity was first occupied by V(Ⅴ) and the binding strength decreases with the increase of occupied binding sites [Citation45]. Parameters of Freundlich isotherm model were presented in , yellow cinnamon soil (n = 3.291), manual loessial soil (n = 2.015) and aeolian sandy soil (n = 2.134) >1 indicated the adsorption process was only less inhibited at lower equilibrium concentrations due to the heterogeneity characteristics of the adsorbent surface without interacting with other particles [Citation46]. The greater KF value showed the higher degree of adsorption reaction. The kF values of these three soils were in the order of yellow cinnamon soil (0.193) > manual loessial soil (0.05) > aeolian sandy soil (0.047), which demonstrated that the yellow cinnamon soil had higher adsorption capacity compared to the manual loessial and aeolian sandy soil, which correspond to the results of the adsorption isotherms. Knowing the KF value, the free energy change (ΔG) of V(Ⅴ) adsorption onto the soil can be calculated by using the equation: ΔG = -RT ln (KF × 1000) [Citation32]. The calculated ΔG value was −13045.3, −9697.2 and −9543.8 kJ/mol, the negative free energy value indicated that the adsorption process is feasible and the adsorption of V(Ⅴ) onto the yellow cinnamon soil, manual loessial soil and aeolian sandy soil is spontaneous.
Table 3. Parameters of isotherm models for the adsorption of V(Ⅴ) onto yellow cinnamon soil, manual loessial soil and aeolian sandy soil.
On the other hand, the adsorption capacity of this soil for V(Ⅴ) was compared with previously reported adsorbents. The result showed that the maximum adsorption amount of the yellow cinnamon soil, manual loessial soil and aeolian sandy soil (1124.6 mg kg−1, 854.4 mg kg−1 and 748.6 mg kg−1, respectively) were different from other soil types, such as German soils (range 1.7–143 mg kg−1), alliticudic ferrisols (130 mg kg−1) and Aluminum-pillared Bentonite (24.16 mg g−1) [Citation6,Citation17,Citation47]. This was because of the specific surface area and the proportion of colloids in the adsorbent.
3.3 Effect of pH and ionic strength
The pH of soil solution also had significant impacts on the adsorption of V(Ⅴ) as it directly controlled the species of vanadium, the surface charge of soil colloid and iron aluminum oxides generated. The batch equilibrium experiments were conducted in initial pH (2–10) of a wide range to determine the effect of pH on the adsorption of V(Ⅴ) onto the three soil in ). The tendency of V(Ⅴ) adsorption increased first and then decreased with increasing pH, which shows that the adsorption amount of vanadium was significantly little in strong acid and strong alkali conditions. Under different pH conditions, V(Ⅴ) presents as 12 different morphological species including cationic, neutral and anionic species [Citation7]. When cationic VO2+ appeared in pH<4, neutral and anionic species (H3VO4) existed in pH from 4 to 6. The dominant species between pH 6 and 10 are H2VO4− and HVO42- [Citation42]. It was well known that soil colloidal particles were negatively charged at alkaline pH; thus, the interaction between anionic V species (H2VO4− and HVO42-) and the soil was more difficult, which was why the adsorption of V(Ⅴ) decreased as pH continued to increase at alkaline pH. Hence, the adsorption of V(Ⅴ) reached a maximum adsorption amount at pH about 4–8. Therefore, the effect of pH was attributed to (1) Charge repulsion or attraction [Citation48]; (2) Effect of pH on soil colloid potential [Citation49]; (3) Effect of pH on soil organic matter due to the adsorption capacity and the ion exchange capacity [Citation50]. Additionally, lower adsorption capacity under acidic conditions was due to competition between the hydrogen ion and VO2+ on the adsorption site, which hydrogen ions are derived from nitric acid used to adjust pH [Citation51]. At a higher pH, the OH− may compete with the vanadate species for adsorption site.
Figure 6. Effects of pH (a) and ion strength (b) on the adsorption of V(Ⅴ) by the yellow cinnamon soil (YS), manual loessial soil (MS) and aeolian sandy soil (AS).
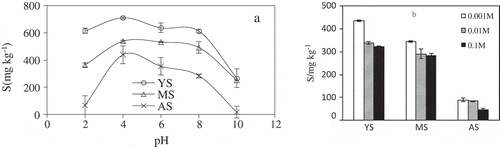
The ionic strength has a significant effect on the adsorption of V(Ⅴ). The effect of ionic strength (0.1, 0.01 and 0.001 M NaNO3 solution) of V(Ⅴ) adsorption onto yellow cinnamon soil, manual loessial soil and aeolian sandy soil were surveyed at pH 7.0 in ). The result showed that the sorption of V(Ⅴ) decreased with the increase of ionic strength. The effect of ionic strength was attributed to: (1) The activity of V (V) or the properties of the electrical double layer is changed by the ionic strength [Citation52]; (2) the competition for adsorption sites on soil surface between high concentrations of anionics and V(Ⅴ). On the other hand, increasing ionic strength will provide more Na+ ions that may occupy the site [Citation53]; (3) The increase of ionic strength accelerated the soil colloid coagulated, the soil colloid had a significant effect on the absorption of metal cations [Citation54] Additionally, based on the surface chemistry theory developed by Santen et al. [Citation55], sorbate species previously enter electrical diffused double layer of solid adsorbent, and the electrical diffused double layer will be compressed by the high ionic strength which resulted in more easily release of adsorbed V(Ⅴ). The adsorption of V(Ⅴ) on aeolian sandy soil in was less affected by ionic strength, whereas the adsorption of V(Ⅴ) on yellow cinnamon soil and manual loessial soil significantly decreased with increasing ionic strength. The results implied that the effect of ionic strength in adsorption V(Ⅴ) may depend on nature of the soil with different mineralogy and physicochemical properties. Similar results were also obtained from the adsorption of arsenic in soils by Feng et al. [Citation46].
4. Conclusions
On the basis of the above results and discussion, a good understanding to the role of different physicochemical parameters such as soil pH, ionic strength (NaNO3), initial V(Ⅴ) concentration and reaction time onto yellow cinnamon soil, manual loessial soil and aeolian sandy soil were obtained using the batch experiment method. Adsorption of V(Ⅴ) showed an increasing trend up to a rapid adsorption reaction time of yellow cinnamon soil, manual loessial soil and aeolian sandy soil, which was at 60 min, 100 min and 120 min, respectively, and this was attributed to the different morphological features. The applicability of the Pseudo-first-order, Pseudo-second-order, Elovich models and intra-particle diffusion models has been simulated and statistically analyzed, which implied that the kinetics data was well fitted with the Pseudo-second-order model. That showed that adsorption of V(Ⅴ) onto soils were a complex dynamic adsorption process with a certain proportion of chemical and physical adsorption. The isothermal data was well fitted with Freundlich model, so the adsorption of V(Ⅴ) on soil was considered as a multilayer adsorption on a heterogeneous adsorbent surface and the maximum adsorption amount onto yellow cinnamon soil, manual loessial soil and aeolian sandy soil, and the maximum adsorption amount were 1124.6 mg kg−1, 854.4 mg kg−1 and 748.6 mg kg−1, respectively. The maximum adsorbing capacity of V(Ⅴ) on yellow cinnamon soil, manual loessial soil and aeolian sandy soil reached stable in the pH range from 4.0 to 8.0. The adsorption capacity decreased with the increase of ionic strength. The results revealed that adsorption of V(Ⅴ) in soil have great difference due to the soil mineralogy and physical–chemical properties, among which BET surface area, soil organic matter and composition of particulate matter played an important role in vanadium adsorption.
Acknowledgement
This research was partially supported by the National Key Research and Development Program [grants numbers 2017YFD0801500, 2018YFD0800400]; National Natural Science Foundation of China [grants numbers 41877018, 41771260]; Key research and development program of Shaanxi province [grants numbers 2017SF-377, 2018ZDXM-SF-030]; Industry University Research Application Cooperative Innovation Key Program of Yangling Agricultural Hi-tech Industries Demonstration Zone [grants numbers 2017CXY-10]; the Fundamental Research Funds for the Central Universities [grants numbers 2452017107]; and the Chinese Scholarship Council.
Disclosure statement
No potential conflict of interest was reported by the authors.
Additional information
Funding
References
- Almeida M, Filipe S, Humanes M, et al. Vanadium haloperoxidases from brown algae of the Laminariaceae family. Phytochemistry. 2001;57(5):633–642.
- Ceci A, Maggi O, Pinzari F, et al. Growth responses to and accumulation of vanadium in agricultural soil fungi. Appl Soil Ecol. 2012;58:1–11.
- Mukherjee B, Patra B, Mahapatra S, et al. Vanadium—an element of atypical biological significance. Toxicol Lett. 2004;150(2):135–143.
- Natsui H, Shibahara T, Yonezawa Y, et al. Effect of manganese and vanadium valences on microstructures and reliability of BaTiO3-based multi-layer ceramic capacitors. IEEE Trans Ultrason Ferroelectr Freq Control. 2012;59(9):1996.
- Mandiwana KL, Panichev N. Electrothermal atomic absorption spectrometric determination of vanadium(V) in soil after leaching with Na 2 CO 3. Anal Chim Acta. 2004;517(1):201–206.
- Gäbler HE, Glüh K, Bahr A, et al. Quantification of vanadium adsorption by German soils. J Geochem Explor. 2009;103(1):37–44.
- Namasivayam C, Sangeetha D. Removal and recovery of vanadium(V) by adsorption onto ZnCl2 activated carbon: kinetics and isotherms. Adsorption. 2006;12(2):103–117.
- Zhang L, Liu X, Wei X, et al. Preparation and characterization of chitosan-zirconium(IV) composite for adsorption of vanadium(V). Int J Biol Macromol. 2014;64(2):155.
- Liu X, Liang C, Du L, et al. Sorption-desorption behaviors of As in different burden brown soils. J Basic Sci Eng. 2011;19(2):222–230.
- Gupta SS, Bhattacharyya KG. Adsorption of Ni(II) on clays. J Colloid Interface Sci. 2006;295(1):21.
- Wiesmeier M, Lützow MV, Spörlein P, et al. Land use effects on organic carbon storage in soils of Bavaria: the importance of soil types. Soil Tillage Res. 2015;146:296–302.
- Xue T, Wang RQ, Zhang MM, et al. Adsorption and desorption of Mercury(II) in three forest soils in Shandong Province, China. Pedosphere. 2013;23(2):265–272.
- Luo X, Yu L, Wang C, et al. Sorption of vanadium (V) onto natural soil colloids under various solution pH and ionic strength conditions. Chemosphere. 2017;169:609–617.
- Zhu H, Xiao X, Guo Z, et al. Adsorption of vanadium (V) on natural kaolinite and montmorillonite: characteristics and mechanism. Appl Clay Sci. 2018;161:310–316.
- Dos Anjos VE, Rohwedder JR, Cadore S, et al. Montmorillonite and vermiculite as solid phases for the preconcentration of trace elements in natural waters: adsorption and desorption studies of As, Ba, Cu, Cd, Co, Cr, Mn, Ni, Pb, Sr, V, and Zn. Appl Clay Sci. 2014;99:289–296.
- Peacock CL, Sherman DM. Vanadium(V) adsorption onto goethite (α-FeOOH) at pH 1.5 to 12: a surface complexation model based on ab initio molecular geometries and EXAFS spectroscopy. Geochim Cosmochim Acta. 2004;68(8):1723–1733.
- Manohar DM, And BFN, Anirudhan TS. Removal of Vanadium(IV) from aqueous solutions by adsorption process with aluminum-pillared bentonite. Ind Eng Chem Res. 2005;44(17):6676–6684.
- Food and Agriculture Organization of the United Nations. World reference base for soil resources. Rome: Food and Agriculture Organization of the United Nations; 1998.(World soil resources reports vii), 88.
- Guedes RS, Melo LCA, Vergütz L, et al. Adsorption and desorption kinetics and phosphorus hysteresis in highly weathered soil by stirred flow chamber experiments. Soil Tillage Res. 2016;162:46–54.
- Wang Y, Chen Y, Xie H, et al. Lead adsorption and transport in loess-amended soil-bentonite cut-off wall. Eng Geol. 2016;215:69–80.
- Kumar AP, Reddy PR, Reddy VK. A rapid, simple, and sensitive spectrophotometric determination of traces of vanadium (V) in foodstuffs, alloy steels, and pharmaceutical, water, soil, and urine samples. Anal Lett. 2008;41(6):1022–1037.
- Lagergren S. Zur theorie der sogenannten adsorption gelöster stoffe, Kungliga Svenska Vetenskapsakademiens. Handlingar. 1898;24:1–39.
- Blanchard G, Maunaye M, Martin G. Removal of heavy metals from waters by means of natural zeolites. Water Res. 1984;18(12):1501–1507.
- Low MJD. Kinetics of chemisorption of gases on solids. Chem Rev. 1960;60(3):267–312.
- Weber WJ, Morriss JC. Kinetics of adsorption on carbon from solution. J Sanitary Eng Div ASCE. 1963;89(2):31–59.
- Langmuir I. The adsorption of gases on plane surfaces of glass, mica and platinum. Am Chem Soc. 1918;40(9):1361–1403.
- Freundlich HMF. Uber die adsorption in lasungen. J Phys Chem. 1906;57:385–370.
- Temkin MI, Pyzhev V. Kinetics of ammonia synthesis on promoted iron catalyst. Acta Physival Chem. 1940;12(3):327–356.
- Shi T, Jia S, Chen Y, et al. Adsorption of Pb(II), Cr(III), Cu(II), Cd(II) and Ni(II) onto a vanadium mine tailing from aqueous solution. J Hazard Mater. 2009;169(1–3):838.
- Ali RM, Hamad HA, Hussein MM, et al. Potential of using green adsorbent of heavy metal removal from aqueous solutions: adsorption kinetics, isotherm, thermodynamic, mechanism and economic analysis. Ecol Eng. 2016;91:317–332.
- Chandrasekaran A, Ravisankar R, Rajalakshmi A, et al. Assessment of natural radioactivity and function of minerals in soils of Yelagiri hills, Tamilnadu, India by gamma ray spectroscopic and Fourier Transform Infrared (FTIR) techniques with statistical approach. Spectrochim Acta A Mol Biomol Spectrosc. 2015;136(6007):1734–1744.
- Huang G, Chen Z, Wang J, et al. Adsorption of arsenite onto a soil irrigated by sewage. J Geochem Explor. 2013;132:164–172.
- Maiti A, Basu JK, De S. Experimental and kinetic modeling of As(V) and As(III) adsorption on treated laterite using synthetic and contaminated groundwater: effects of phosphate, silicate and carbonate ions. Chem Eng J. 2012;191(6):1–12.
- Zhu N, Yan T, Qiao J, et al. Adsorption of arsenic, phosphorus and chromium by bismuth impregnated biochar: adsorption mechanism and depleted adsorbent utilization. Chemosphere. 2016;164:32–40.
- Mohanty K, Das D, Biswas MN. Adsorption of phenol from aqueous solutions using activated carbons prepared from Tectona grandis sawdust by ZnCl2 activation. Chem Eng J. 2005;115(1):121–131.
- Gupta VK, Pathania D, Kothiyal NC, et al. Polyaniline zirconium (IV) silicophosphate nanocomposite for remediation of methylene blue dye from waste water. J Mol Liq. 2014;190:139–145.
- Zolgharnein J, Shahmoradi A. Adsorption of Cr(VI) onto elaeagnus tree leaves: statistical optimization, equilibrium modeling, and kinetic studies. J.Chem.Eng.Data. 2010;55(9):3428–3437.
- Roy A, Chakraborty S, Prasad Kundu S, et al. Adsorption of Anionic-Azo dye from aqueous solution by lignocellulose-biomass jute fiber: equilibrium, kinetics, and thermodynamics study. 2012;51:12095–12106.
- Boyd GE, Jr LSM, Adamson AW. The exchange adsorption of ions from aqueous solutions by organic zeolites. III. Performance of deep adsorbent beds under non-equilibrium conditions 1. J Am Chem Soc. 1947;69(11):2818.
- Rao RAK, Khan MA, Rehman F. Utilization of Fennel biomass (Foeniculum vulgari) a medicinal herb for the biosorption of Cd(II) from aqueous phase. Chem Eng J. 2010;156(1):106–113.
- Giles CH, MacEwan TH, Nakhwa SN, et al. Studies in adsorption. Part XI. A system of classification of solution adsorption isotherms, and its use in diagnosis of adsorption mechanisms and in measurement of specific surface areas of solids. J Chem Soc. 1960;3: 3973–3993.
- Mikkonen A, Tummavuori J. Retention of vanadium (V) by three Finnish mineral soils. Eur J Soil Sci. 2010;45(3):361–368.
- Kango S, Kumar R. Low-cost magnetic adsorbent for As(III) removal from water: adsorption kinetics and isotherms. Environ Monit Assess. 2016;188(1):60.
- Afsar MZ, Hoque S, Osman KT. A comparison of the Langmuir, Freundlich and Temkin equations to describe phosphate sorption characteristics of some representative soils of Bangladesh. Int J Soil Sci. 2012;7(3):91–99.
- Shafqat MN, Pierzynski GM. The Freundlich adsorption isotherm constants and prediction of phosphorus bioavailability as affected by different phosphorus sources in two Kansas soils. Chemosphere. 2014;99(3):72–80.
- Feng Q, Zhang Z, Chen Y, et al. Adsorption and desorption characteristics of Arsenic on soils: kinetics, equilibrium, and effect of Fe(OH)3 colloid, H2SiO3 colloid and phosphate. Procedia Environ Sci. 2013;18:26–36.
- Xiao XY, Yang M, Guo ZH, et al. Permissible value for vanadium in allitic udic ferrisols based on physiological responses of green Chinese cabbage and soil microbes. Biol Trace Elem Res. 2012;145(2):225–232.
- Ma J, Guo H, Lei M, et al. Blocking effect of colloids on arsenate adsorption during co-transport through saturated sand columns. Environ Pollut. 2016;213:638–647.
- Plaza I, Ontiveros-Ortega A, Calero J, et al. Implication of zeta potential and surface free energy in the description of agricultural soil quality: effect of different cations and humic acids on degraded soils. Soil Tillage Res. 2015;146(Part B):148–158.
- Ko I, Davis AP, Kim J-Y, et al. Effect of contact order on the adsorption of inorganic arsenic species onto hematite in the presence of humic acid. J Hazard Mater. 2007;1:53–60.
- Blackmore DPT, Ellis J, Riley PJ. Treatment of a vanadium-containing effluent by adsorption/coprecipitation with iron oxyhydroxide. Water Res. 1996;30(10):2512–2516.
- Fang J, Zhang K, Sun P, et al. Co-transport of Pb2+ and TiO2 nanoparticles in repacked homogeneous soil columns under saturation condition: effect of ionic strength and fulvic acid. SciTotal Environ. 2016;571:471–478.
- Wang T, Liu W, Xiong L, et al. Influence of pH, ionic strength and humic acid on competitive adsorption of Pb (II), Cd (II) and Cr (III) onto titanate nanotubes. Chem Eng J. 2013;215:366–374.
- Wang S, Gao B, Li Y, et al. Manganese oxide-modified biochars: preparation, characterization, and sorption of arsenate and lead. Bioresour Technol. 2015;181:13–17.
- van Santen RA, Tranca I, Hensen EJM. Theory of surface chemistry and reactivity of reducible oxides. CatalToday. 2015;244(SupplementC):63–84.