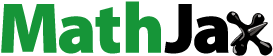
ABSTRACT
To investigate responses difference of soil potential nitrification rates (PNR) to heavy metals and antibiotics, batch toxicity experiments of Cu and sulfadiazine (SDZ) were conducted in four typical soils which were collected from different locations of China. Toxic differences of Cu and SDZ to soil PNR were evaluated based on their values of EC20 and EC50 calculated with logistic model. It was found that soil nitrification was a good indicator of Cu contamination in soils except for paddy soil. For SDZ, their ECX values were significantly higher than its concentration level (0.002 ~ 0.760 mg·kg-1) in soils. Thus, it was concluded that soil nitrification might be more sensitive to Cu than SDZ in field soil. The further Pearson correlation analysis indicated that the response of soil PNR to Cu and SDZ contamination were significantly related with soil PNR0 and pH.
KEYWORDS:
Nowadays, soil-heavy metal pollution has been a relatively serious problem in the world. Mining and sewage irrigation are often thought as the main reasons causing the increasing of heavy metals in agricultural soils. In fact, application of livestock feces in agricultural soils is also a key factor of heavy metals’ contamination in large scale; metals include some nutrient elements, copper, zinc, etc. [Citation1–Citation3]. As an essential trace element, Cu pollution, especially its soil pollution, was not payed enough attention in past. However, more attention is paid on the trace element copper pollution in soils with the great investigation of soil environment [Citation4–Citation6]. For example, Keiblinger et al. [Citation7] found a strong negative effect of Cu application on the total abundance of soil microbial biomass and the fungal biomass proxy ergosterol at relatively low concentrations.
Recently, antibiotics are widely used in treating many human and animal infectious diseases. But most of them cannot be metabolized in body, 80% of which is excreted into their excreta. Besides, the reclaimed water (derived from wastewater treatment plant) irrigation to the soil in agriculture is another potential antibiotics pollution source. Thus, lots of antibiotics are found in soils and become one kind of emerging pollutants. For example, in an agricultural field at Hannover, the tetracycline (TTC) level in the dried manure from the soil surface was 4 mg·kg−1, which exceeds the normal detected concentration of TTC in soil [0.27~1.01 mg·kg−1, Citation8], and might affect the soil organisms [Citation9]. Sulfonamides (SAs), one of the most useful antibiotics in animal farms, are often detected in soils. Previous investigations indicated that sulfonamides could affect the soil microbial activities, such as the enzyme activity, functional diversity and community structure. Toth et al. [Citation10] found that sulfonamides could have an inhibitory effect on soil nitrification periodically over the course of the study. In addition, China is one of the largest producers and consumers of antibiotics in the world, and sulfonamides are also widely used and released into the terrestrial environment. It was found that the mean residual level of sulfonamides in vegetable farms’ soils in four provinces of China was 2.61 μg·kg−1 [Citation11].
Nitrification is central process in global nitrogen cycle and this process mainly benefit from soil nitrogen-transforming microbes [Citation12, Citation13], which is considered to be easily influenced by environmental pollutants, such as heavy metals or antibiotics. So, soil nitrification is used to screen pollutants toxicity because of its sensitivity [Citation14]. Besides, relevant researches showed pH and other physical and chemical properties could also be an essential factor to affect soil nitrification [Citation15, Citation16]. Therefore, exploring the relationship between soil potential nitrification rates (PNR) and pollutants stress and environmental factors is a research strategy to evaluate the soil quality. However, most of researches are concentrated on Cd, Pb and other heavy metals that have serious pollution in soils; but Cu, a vital trace element for body, has not aroused attention as potential pollutants to date [Citation17–Citation19]. And the effect of antibiotic pollution on soil nitrification remain uncertain [Citation10, Citation20]. In this study, we address three issues in four soil systems of China: 1) the effect of Cu on soil PNR; 2) response of PNR to SDZ stress; 3) correlation between the soil PNR and environmental factors, which provide a reliable theoretical basis for the risk assessment of heavy metals and antibiotics pollution in the soil.
1. Materials and methods
1.1. Soil sampling
Soil samples were collected from 0 ~ 20 cm top soils (agricultural soil) of four different provinces, China. The physical and chemical properties of soils were determined in accordance to the traditional method after sieving with a 2 mm mesh (). Soil pH was determined by a pH meter (MT-8060, KADY, China) at the soil-to-water ratio of 1:2.5 and organic matter was determined with K2Cr2O7 oxidation method. Acetic ammonium saturation was used to determine the cation exchange capacity (CEC). The distribution of soil particle size was measured using a laser particle size analyzer (Rise 2008, Rise Ltd. Co., China). Cu in soil was determined using ICP-AES (Vista MPX, Varian, USA) after digestion with HCl, HNO3 and HF (8 mL, 3:9:4, v1/v2/v3). A standard reference material and a reagent blank were used for quality control. Our measured contents were in a good agreement with the certified values and recoveries ranged from 77 to 98%. Soil SDZ was firstly extracted with 1% formic acid-methanol (7:3 v1/v2) and then determined by high-performance liquid chromatography (HPLC 1100, Agilent, USA) after filtering with a 0.45 μm membranes. Similarity, blank and recovery tests were used to control assurance and quality (QA/QB). In addition, we did in triplicates to confirm the precision of the analysis method. The precision and bias were less than 10% in all analyses.
Table 1. Soil physical and chemical properties.
1.2. Soil treatments
Soil samples were incubated under 25 ± 2°C for soil microbial recovery. During the incubation, soil moisture was kept at 50 ± 5% maximum water field holding capacity (WHC) by adding deionized water periodically. After 14 days’ incubation, pre-cultured soil was spread with the stock solution of CuSO4 or SDZ to reach a final soil concentration of 100 ~ 1500 mg·kg−1 dry soil for Cu and 0.5 ~ 10 mg·kg−1 dry soil for SDZ, respectively. Detailed contractions of SDZ and Cu in each treatment were listed in . Subsequently, the soils were thoroughly mixed by hand and incubated for 7 days of exposure to Cu and SDZ. Here, EC20 and EC50 (effective concentration causing a 20% or 50% reduction in the PNR) could evaluate the toxicity of pollutants in soils and provide the basic for the risk assessment of soil environment quality. Thus, PNR was measured and ECx values for pollutant was determined based on PNR. Besides, three replicates were made for each concentration level and the soil without CuSO4 and SDZ was treated as control.
Table 2. Spiked and measured concentrations of Cu and SDZ.
1.3. Methods for determination and analysis of PNR
The PNR of the soil sample was measured using the ISO method described by Rusk et al. [Citation21], with slight modification. A subsample preincubated was collected from each microcosm for the first extraction. Another subsample was treated with 0.044 M (NH4)2SO4 to reach 100 mg N·kg−1, followed by another 14 days incubation at 25 ± 2°C in the dark, which water content was adjusted to 75% WHC. At the end of the incubation, the NO3−-N was extracted from the soil according to the introduction of a Nitrate nitrogen test kit (Cominbio Biotechnology Co. Ltd, Suzhou, China) and determined at 410 nm using a microplate reader (Infinite Pro 200, Tecan, Switzerland). PNR defined as the yield of nitrate per gram of dry soil per day, was calculated according to Equation (1).
Where:
PNR was the potential nitrification rate (NO3–N mg·kg−1·d−1); ω(NO3–N)1 and ω(NO3–N)2 was the content of NO3–N at 0 day and 14 days of the incubation after adding (NH4)2SO4, respectively, (mg·kg−1); X was the incubation period (days).
ECx was the Cu or SDZ effective concentration that inhibited the PNR to 20 or 50% compared to the control. In this study, the ECx was obtained through dose-response curve fitted by log-logistic distribution (Equation (2)).
Where:
y was soil PNR;A1 was the minimum value of PNR in all treatments; the value of A2 was the PNR of control; x was the concentration of Cu or SDZ when PNR was y (mg·kg−1);p was the slope parameter.
1.4. Data analysis and calculations
All parameters used in the analysis were three-times parallel mean with standard deviation. One-way ANOVA was applied to determine whether the PNR with different soils had significant difference. Pearson correlations analysis was conducted to evaluate the relationships between PNR with physicochemical parameters and pollutant concentrations (SPSS 17.0). Besides, experimental data and figures were completed with EXCEL 2010 and Oringin 8.5, respectively.
2. Results
2.1. Initial potential nitrification rate (PNR0) of soils
The PNR0 of four soils were shown in . Different lowercase letters expressed significant differences among PNR0 values of the selected soils. The results showed that four tested soils had different PNR0, with the lowest value (4.636 NO3–N mg·kg−1·d−1) in paddy soil and the highest in desert soil (6.344 NO3–N mg·kg−1·d−1). Only paddy soil and desert soil showing the significant difference (p < 0.05), while the PNR0 of brown soil (5.790 NO3–N mg·kg−1·d−1) and black soil (5.846 NO3–N mg·kg−1·d−1) had no significant difference.
2.2. Responses of PNR to Cu stress
To research the effects of Cu on soil PNR, series of acute toxicity tests were performed. As showed in , in 100 and 300 mg·kg−1 Cu contaminated paddy soil, the PNR values were 6.328 and 5.628 NO3–N mg·kg−1·d−1, respectively, which was slightly stimulated compared to its PNR0. However, the obvious inhibition of soil PNR was found in the remaining three soils (–)). Moreover, with the increase of Cu concentrations, the PNR values showed a significant decrease in all the tested soils, and the lowest PNR was found in soil samples treated with 1500 mg·kg−1 Cu addition, which was 2.317, 2.483, −0.570 and 2.478 NO3–N mg·kg−1·d−1 for paddy soil, desert soil, brown soil and black soil, respectively.
The Logistic model (Equation (2)) was used to analyze the relationship between PNR and Cu addition, and its effective concentration (ECx) was calculated by the dose-response curve. As shown in , the relationship between soil PNR and Cu concentrations could be fitted well with the log-logistic model, and brown soil was the best fitted one (R2 = 0.972, p < 0.01), followed by desert soil (R2 = 0.942, p < 0.01), black soil (R2 = 0.817, p < 0.05) and paddy soil (R2 = 0.808, p < 0.05). The corresponding Cu concentration in soil at which the PNR was reduced by 20% (Cu EC20) and 50% (Cu EC50) ranged from 156.63 mg·kg−1 (desert soil) to 1110.07 mg·kg−1 (paddy soil) and 418.73 mg·kg−1 (brown soil) to 1490.21 mg·kg−1 (paddy soil), respectively ().
Table 3. EC20 and EC50 values in different soils.
2.3. Effect of SDZ on soil PNR
As shown in , the PNR of desert soil and brown soil decreased gradually with the increasing concentrations of SDZ. The lowest PNR was detected in treated samples with 10 mg·kg−1 SDZ addition, which was 3.470 and 3.024 NO3–N mg·kg−1·d−1 for desert soil and brown soil, respectively. However, the PNR of remaining two soils did not show an obvious decrease. In 0.5, 1 and 3 mg·kg−1 SDZ contaminated paddy soil, the PNR values were 5.680, 5.923 and 5.185 NO3–N mg·kg−1·d−1, respectively, which was slightly stimulated compared to its PNR0, but its PNR recovered to the level of control with 5 and 10 mg·kg−1 SDZ addition. For the black soil, its PNR fluctuated continuously at PNR0 for all concentrations, without an obvious change. Here, what needed to be explained was paddy soil and black soil did not participate in the Logistic model fitting because their PNR were not inhibited by exogenous SDZ.
Similarly, the Logistic model (Equation (2)) was used to fit the data for dose-response curves and derive ECx values. For the paddy soil and black soil, their dose-response curves and ECx values could not be determined because inhibition relative to the control was not observed in experimental concentrations of SDZ. In contrast, the dose-response curves were fitted well in the desert soil (R2 = 0.960, p < 0.05) and brown soil (R2 = 0.982, p < 0.05). Besides, EC20 and EC50 value in the desert soil was 6.87 and 10.08 mg·kg−1, while the brown soil was 4.14 and 7.66 mg·kg−1, respectively, which was significantly higher than its concentration levels reported in the reference literature ().
3. Discussion
The different effect of Cu and SDZ on the PNR of four typical soils, China, was investigated in this study. The results indicated that the PNR values of four tested soils decreased gradually with the increase of Cu, which fitted well with the Logistic model. This result was consistent with that of Smolders et al. [Citation22]. In addition, brown soil had the lowest EC50 (Cu) value, which suggested nitrification microbes in this soil were more sensitive to Cu stress than the others [Citation23, Citation24]. For SDZ stress, four tested soils PNR responses were not consistent. This could be related to the difference in soil properties [Citation25]. Besides, it was worth noting that a good dose-response curve of SDZ and soil PNR was only found for desert and brown soil, which indicated the nitrifying microorganisms of paddy and black soil were not sensitive to SDZ contamination. It might be related to the previous contamination, as in the original black soil the concentration of SDZ was up to 0.58 mg·kg−1 (). Ding and He [Citation26] found that the microorganisms could adapt to even high concentrations of antibiotics through various adaptive mechanisms in chronically polluted environment.
To investigate the relationship among selected properties, we analyzed the correlation between the PNR0, ECX and initial concentrations of elements. As shown, soil PNR0 and EC50 (Cu) were both significantly affected by the soil pH (p < 0.05), indicating the initial PNR and toxic effect of the element were dependent on soil pH [Citation22]. In addition, EC20 (Cu) of soil PNR had a significant negative relationship with its PNR0, which suggested soil toxic threshold of Cu could be attributed to the soil initial potential nitrification rate [Citation18]. So, pH was the dominated factor that controlled the soil nitrification, which was consistent with previous researches. Stemarie and Pare [Citation15] investigated the effects of soil pH on net nitrification in forest floors across a wide range of boreal forest stands (soil pH 4.83~6.23) in a laboratory incubation experiment and found that increased pH promoted soil net nitrification. Similarity, the low pH was also regarded as an essential factor for the low nitrification rate in Scots pine forest soils [Citation27]. It could be related to the ammonia oxidizing microorganisms, the dominating microorganisms in soil nitrification, they were sensitive for pH [Citation28–Citation30]. Nicol et al. found the gene copies and transcripts of the amoA gene decreased and increased with increasing soil pH (4.9~7.5) for ammonia oxidizing microorganisms (AOA and AOB), respectively. However, there was not significant correlation between CEC, OM, Clay, Cu and SDZ, which illustrated these indicators did not influence soil nitrification significantly. And previous investigations shown different sampling time (such as different seasons) could lead to different responses for soil nitrification [Citation31]. Accordingly, future studies about soils nitrification response in other sampling time were warranted.
Table 4. Pearson’s correlation (r values) among the selected properties.
In this study, the EC20 (Cu) of soil PNR was adjacent to its risk screening value of agricultural land according to the national soil quality standard (GB15618-2018) (), which was belong to the light contamination for arable soil [Citation32]. It proved that soil nitrification was a good indicator to soils Cu contamination. However, SDZ was not list in the national soil quality standard (GB15618-2018), but the previous investigation revealed that SDZ concentrations in soil were mostly at the level of ‘μg·kg−1’ in arable soils () [Citation11, Citation33–Citation35]. Moreover, the toxic endpoint of SDZ to soil PNR was kept at the level of ‘mg·kg−1’, which EC20 was over than 4 mg·kg−1 for the tested soils in this study. It suggested that soil PNR might not be sensitive to soil SDZ contamination, which could not be used to screening SDZ contamination of the arable soils.
Overall, this study analyzed the responses difference of soil PNR to Cu and SDZ as well as the relationship with environmental factors. However, it was just performed under single stress. The combined pollution and response mechanism of PNR were not involved. Therefore, for the next study, the response mechanism of soil nitrifying microorganisms under Cu and SDZ combined pollution was necessary.
4. Conclusion
In this study, the ecological toxicity of Cu and SDZ to soil environment was explored from the point of soil nitrification. And, we obtained two essential conclusions: 1) Cu could decrease all tested soil PNR significantly, while SDZ inhibited PNR in desert and brown soil. Thus, soil PNR was a good indicator to assess soil Cu contamination, but not for SDZ; 2) the toxic effects of Cu and SDZ on soil PNR were both mainly controlled by the soil properties, which was very meaningful for the application of soil PNR in the ecological risk assessment of soil contamination.
Acknowledgments
The study was supported by the National Natural Science Foundation of China (NSFC) (No. 41671322; 41877122) and SDUT & Zibo City Integration Development Project (NO. 2016ZBXC102).
Disclosure statement
No potential conflict of interest was reported by the authors.
Additional information
Funding
References
- Kalyvas G, Gasparatos D, Papassiopi N, et al. Topsoil pollution as ecological footprint of historical mining activities in Greece. Land Degrad Dev. 2018; 29:2025–2035.
- Gatta G, Gagliardi A, Disciglio G, et al. Irrigation with treated municipal wastewater on artichoke crop: assessment of soil and yield heavy metal content and human risk. Water. 2018;03:255.
- Liu WH, Zhao JZ, Ouyang ZY, et al. Impacts of sewage irrigation on heavy metal distribution and contamination in Beijing, China. Environ Int. 2005;31:805–812.
- Dala-Paula BM, Custódio FB, Knupp EAN, et al. Cadmium, copper and lead levels in different cultivars of lettuce and soil from urban agriculture. Environ Pollut. 2018;242:383–389.
- Zhang P, Qin C, Hong X, et al. Risk assessment and source analysis of soil heavy metal pollution from lower reaches of Yellow River irrigation in China. SciTotal Environ. 2018;633:1136–1147.
- Barkett MO, Akün E. Heavy metal contents of contaminated soils and ecological risk assessment in abandoned copper mine harbor in Yedidalga, Northern Cyprus. Environ Earth Sci. 2018;77:378.
- Keiblinger KM, Schneider M, Gorfer M, et al. Assessment of Cu applications in two contrasting soils-effects on soil microbial activity and the fungal community structure. Ecotoxicology. 2018;27:217–233.
- Jiang WQ, Gao J, Cheng Z, et al. The effect of antibiotics on the persistence of herbicides in soil under the combined pollution. Chemosphere. 2018;204:303–309.
- Hamscher G, Sczesny S, Höper H, et al. Determination of persistent tetracycline residues in soil fertilized with liquid manure by high-performance liquid chromatography with electrospray ionization tandem mass spectrometry. Anal Chem. 2002;74:1509.
- Toth JD, Feng Y, Dou Z. Veterinary antibiotics at environmentally relevant concentrations inhibit soil iron reduction and nitrification. Soil Biol Biochem. 2011;43:2470–2472.
- Wei RC, He T, Zhang SX, et al. Occurrence of seventeen veterinary antibiotics and resistant bacterias in manure-fertilized vegetable farm soil in four provinces of China. Chemosphere. 2019;215:234–240.
- Kucharski J, Wyrwał A, Boros E, et al. Nitrification process as an indicator of soil contamination with heavy metals. Ecol Chem Eng. 2009;16:953–961.
- Dong L, Córdova-Kreylos AL, Yang J, et al. Humic acids buffer the effects of urea on soil ammonia oxidizers and potential nitrification. Soil Biol Biochem. 2009;41:1612–1621.
- Khan M, Scullion J. Effect of soil on microbial responses to metal contamination. Environ Pollut. 2000;110:115.
- Stemarie C, Paré D. Soil, pH and N availability effects on net nitrification in the forest floors of a range of boreal forest stands. Soil Biol Biochem. 1999;31:1579–1589.
- Mørkved PT, Dörsch P, Bakken LR. The N2O product ratio of nitrification and its dependence on long-term changes in soil pH. Soil Biol Biochem. 2007;39:2048–2057.
- Baghaie AH, Aghili F. Health risk assessment of Pb and Cd in soil, wheat, and barley in Shazand County, central of Iran. J Environ Health Sci Eng. 2019;5:1–11.
- Liu AJ, Fang DM, Wang C, et al. Recovery of soil nitrification after long-term zinc exposure and its co-tolerance to Cu in different soils. Environ Sci Pollut Res Int. 2015;22:314–319.
- Divisekara T, Navaratne AN, Abeysekara A S K. Impact of a commercial glyphosate formulation on adsorption of Cd(II) and Pb(II) ions on paddy soil. Chemosphere. 2018;198:334–341.
- Kotzerke A, Fulle M, Sharma S, et al. Alterations in total microbial activity and nitrification rates in soil due to amoxicillin-spiked pig manure. J Plant Nutr Soil Sci. 2011;174:56–64.
- Rusk JA, Hamon RE, Stevens DP, et al. Adaptation of soil biological nitrification to heavy metals. Environ Sci Technol. 2004;38:3092–3097.
- Smolders E, Brans K, Coppens F, et al. Potential nitrification rate as a tool for screening toxicity in metal-contaminated soils. Environ Toxicol Chem. 2001;20:2469–2474.
- Rooney CP, Zhao FJ, McGrath SP. Phytotoxicity of nickel in a range of European soils: influence of soil properties, Ni solubility and speciation. Environ Pollut. 2007;145:596–605.
- Smolders E, Buekers J, Oliver I, et al. Soil properties affecting toxicity of zinc to soil microbial properties in laboratory-spiked and field-contaminated soils. Environ Toxicol Chem. 2004;23:2633.
- Ueda MU, Kachina P, Marod D, et al. Soil properties and gross nitrogen dynamics in old growth and secondary forest in four types of tropical forest in Thailand. For Ecol Manage. 2017;398:130–139.
- Ding C, He J. Effect of antibiotics in the environment on microbial populations. Appl Microbiol Biotechnol. 2010;87:925–941.
- Nugroho RA, Röling WF, Laverman AM, et al. Low nitrification rates in acid Scots pine forest soils are due to pH-related factors. Microb Ecol. 2007;53:89–97.
- Cheng Y, Wang J, Mary B, et al. Soil pH has contrasting effects on gross and net nitrogen mineralizations in adjacent forest and grassland soils in central Alberta, Canada. Soil Biol Biochem. 2013;57:848–857.
- Persson T, Wirén A. Nitrogen mineralization and potential nitrification at different depths in acid forest soils. Nutr Uptake Cycling For Ecosyst. 1995;168/169:55–65.
- Nicol GW, Leininger S, Schleper C, et al. The influence of soil pH on the diversity, abundance and transcriptional activity of ammonia oxidizing archaea and bacteria. Environ Microbiol. 2008;10:2966–2968.
- Zhang Y, Ding H, Hu XX, et al. Nitrification and denitrification Activities and N2O emission of orchard soils cultivated for different periods of time. Agric Sci Technol. 2012;13:843–848.
- MEP of China (Ministry of Environmental Protection of China), National soil pollution survey bulletin, 2014.
- Michelini L, Reichel R, Werner W, et al. Sulfadiazine uptake and effects on Salix fragilis L. and Zea mays L. plants. Water Air Soil Pollut. 2012;223:5243–5257.
- An J, Chen HW, Wei SH, et al. Antibiotic contamination in animal manure, soil, and sewage sludge in Shenyang, northeast China. Environ Earth Sci. 2015;74:5077–5086.
- Baran W, Adamek E, Ziemiańska J, et al. Effects of the presence of sulfonamides in the environment and their influence on human health. J Hazard Mater. 2011;196:1–15.