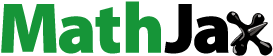
ABSTRACT
With the climate warming leading to thawing permafrost, the trace metal releasing from the permafrost is of increasing importance. The source area of the Yellow river (SAYR) in Tibet Plateau, underlain by permafrost which provides important source water, was investigated. One hundred and eleven water samples across SAYR were collected in April 2014, when permafrost thawing began and the precipitation season had not arrived yet. It was found that the arsenic concentration level was relatively higher than other pristine surface water in the world, but similar to other source regions on the Tibetan Plateau, showing SAYR is pristine and can serve as a reference region for determining the human influence on permafrost-affected landscapes. The positive correlation of arsenic with sulfate suggested that the arsenic was predominantly derived from the weathering process of arsenic-rich sulfides. Climate warming enhanced thawing depth of permafrost may accelerate the release and mobilization of arsenic to the surface water.
1. Introduction
Permafrost thawing not only impacts the hydrological cycle but also impact the water quality [Citation1]. The element stored in the frozen soil can be released with the thawing process [Citation2,Citation3]. Schuster et al. [Citation3] estimated the Northern Hemisphere permafrost soils contained nearly twice as much Mercury as all other soils, the ocean and atmosphere combined. On the other hand, the increased permafrost thawing depth extends the water residence time and further enhances the enrichment of the elements in the water [Citation1,Citation4]. Research done in permafrost areas in the Austrian–Italian Alps [Citation5] and in the Colorado Front Range [Citation6] has shown that thawing permafrost alters water chemistry. The climate change is accelerating the permafrost thawing process [Citation7]. It also impacts the aquatic ecosystem health and the drinking water sources [Citation8].
Arsenic is an important environment- and health-concerned element owing to its known chronic and epidemic toxicity. It is ubiquity, widely distributed in the surface water, groundwater, soil, rocks and atmosphere [Citation9]. High arsenic concentration in drinking water can cause serious health problems, such as melanosis, hyperkeratosis, restrictive lung disease, gangrene, hypertension, cancers and peripheral vascular disease [Citation10]. Currently, elevated concentrations of arsenic have been found in many areas of the world, making this element a significant pollution source of water [Citation11–Citation14]. Literature showed that the river basin in Tibet Plateau has a relatively high concentration of arsenic [Citation15–Citation17]. Since Tibet Plateau is the source of several important large rivers, an increase of the arsenic might have a vital impact on the downstream ecosystem health. Investigating the arsenic level is necessary in the Tibet Plateau area.
The source area of the Yellow River (SAYR) located in the northeast of the Qinghai-Tibetan Plateau underlined with permafrost, which is one of its main water recharge sources. SAYR is an important study area for investigating arsenic release to the surface water in the permafrost area. It is suffering from extensive thawing during the last two decades due to the changing climate. Since 0.12 billion people live in the Yellow River Basin, the water quality of SAYR has remarkable importance. The source, distribution and concentration level of arsenic in the surface water in SAYR are vital to estimate the ecological risk on the whole Yellow River basin.
This study characterized background level of the arsenic in permafrost-affected surface water of the SAYR and its potential sources, representing a remote region far from evident anthropogenic arsenic sources; in addition, investigated if the accelerating permafrost thawing due to the climate change might increase the release of arsenic in the permafrost area.
2. Materials and methods
2.1. Study area
The SAYR (33°56′–35°31′N and 95°55′–98°41′E, elevation 4100–5442 meters above sea level (m asl)) is located on the northeastern Qinghai-Tibet Plateau and occupies the Yellow River valley above Duoshixia (34°46′25.15″N, 98°20′59.03″E; 4222 m asl), with a total catchment area of approximately 2.5 × 104 km2 [Citation18] (). It is bordered by the Bayan Har Mountains in the south, by the Buqing Mountains in the north and by the Geshigeya Mountains in the west (). The topography includes hills, valleys, lakes, rivers and high plains. Most of the lakes discharge freshwater to rivers, such as the GalalaCo, Xingxinghai, Xingxiuhai lake clusters and the two largest lakes – the Ngoring Lake (34°46′–35°05′N, 97°32′–98°54′E, 4266–4267 m asl) and the Gyaring Lake (34°49′–35°01′N,97°03′–97°27′E, 4289–4290 m asl). They modulate river runoffs and the local microclimate [Citation8].
Due to the high elevation, typically 4200–5000 m asl, although no glaciers are represented in the SAYR area, the permafrost is widely distributed. Discontinuous permafrost distributed at 4300–4400 m asl, while continuous permafrost above 4400 m asl. The permafrost in SAYR is sensitive to climate change because of the delicate thermal balance and the strong monsoon impact [Citation8]. The mean annual air temperature in these areas is lower than −4°C and the mean annual precipitation ranges from 300 to 400 mm. Both the temperature and precipitation decrease gradually from southeast to northwest. More than 80% of the total annual precipitation occurs from May to September. Seasonal temperature variations are rather small. With a long cold season and a short warm season, freezing temperatures can occur in any time. Rather, limited variation in temperature, changes in precipitation rate, relatively high evaporation, frequent strong winds and strong solar radiation are the main climate characteristic of SAYR area, which is good for rock weathering process. This region is sparsely populated, with a population density range from 0.5 to 2 persons per square kilometer [Citation19], where the human impact could be neglected.
2.2. Method
In April 2014, marking the start of Spring and permafrost thawing season in the region, water samples (n = 111) were collected across the whole SAYR. The sampling sites were primarily determined by the accessibility, with an effort to cover most of the branches of river systems and the large lakes (). In addition, the high altitude is accompanied by a low oxygen level in the air, making the sampling work difficulty. During sampling, most of the lakes and river branches above 4000 m asl were covered by ice, indicating the starting of the thawing season. In this study, the arsenic concentration in the spring water samples was used to represent the arsenic level in the groundwater.
The GPS coordinates of the samples were recorded by a handled GPS device (Gamin e Trex Vista C). Temperature was measured with a probe. Water samples were collected in two 100 ml polyethylene bottles separately in each sampling location, one of which was acidified with HCl (trace metal grade) in order to make the pH value smaller than 2 for arsenic testing. The other was kept original for anion analysis. Water samples were kept in cold condition (4°C) until analysis.
Before analysis, water samples were filtered through 0.22-μm nucleopore polycarbonate filters. Arsenic and iron concentrations were measured by ICP-MS with a detection limit of 0.01 ppb at the Analysis and Testing Center of Lanzhou university in China. Anions including phosphate, nitrate and sulfate were analyzed by ion chromatography (IC, Dionex DX5000) in Nanjing Institute of Geography and Limnology, Chinese Academy of Sciences.
3 Results and discussion
3.1 Arsenic in SAYR
3.1.1 Arsenic concentration distribution
Arsenic concentrations in the river water varied over the range of 4.3–77.1 μg/L (n = 76), with a mean value of 25.2 μg/L (). Concentrations at the low end of the range (4.3 μg/L) have been found in a river beside Bayan Har mountain (97.79°E,34.19°N), whereas the high end of the range (77.1 μg/L) has been found in a small permafrost-charged closed river basin called Shuangchagou (97.32°E,34.65°N) (). In lake water, arsenic concentrations were in a wider range of 9.3–131.0 μg/L (n = 35), with a mean value of 39.6 μg/L, higher than that in the river water. The lowest concentration of arsenic in lake water also appeared beside Bayan Har mountain (97.79°E, 34.19°N), and the highest concentration of arsenic in lake water was found in Gyaring lake (97.50°E, 35.00°N) (). Low-lying closed area often accompanied with high concentrations of arsenic (Elena and Smedley 2008), such as Shuangchagou and Gyaring lake in this study.
Table 1. Summary of arsenic in the source region of Yellow river.
The arsenic concentration in SAYR was similar to other parts of the river source region on the Tibetan Plateau () but relatively higher than other pristine source water in the world (). In Tibet river and lake systems, average arsenic concentration was 15.9 μg/L in Yarlung Tsangbo, 8.5 μg/L in Lhasa river, 59.34 μg/L in Shiquan river [Citation15] and 52.2 μg/L in Tuotuo river [Citation17], which were consistent with the arsenic level in SAYR. In other pristine areas of the world, except for some area influenced by geothermal, the arsenic level was sort of high, varied between 85 and 153 μg/L, like in Owens River, CA, USA [Citation20]. In the Po river of Italy, where anthropogenic inputs of arsenic were low, the average concentration of arsenic was 1.3 μg/L [Citation21]. In upstream of Garonne, Dordogne and Isle rivers, France, average arsenic concentration was only 2.14, 1.05 and 2.82 μg/L, respectively, [Citation22]. They are all much lower than the arsenic concentration in SAYR.
Table 2. Levels of arsenic in Tibet river and lake systems.
Table 3. Arsenic levels in other river systems.
3.1.2 Arsenic output
The total output of arsenic from the SAYR was represented by the amount from the Huangheyan hydrological station () in this study. Dissolved arsenic flux outlet from SAYR was calculated by
in which C represented the mean concentration of the arsenic measured around the hydrological station (μg/L), Q was the total runoff in April (m3) and J represented the flux of arsenic flow into the river (kg). Approximately 903.4 kg of dissolved arsenic in April was released into the Yellow River from SAYR, impacting the ecosystem downstream. The annual flux of arsenic from the English Channel toward the North Sea was 4442 tons [Citation23]. The fluxes of dissolved arsenic of the Gomati River ranged from 400 to 1500 kg each month [Citation24]. In Scheldt estuary, the annual output to the sea at around 26.3 × 103 kg year −1 [Citation25]. In Seine Estuary, mean arsenic export to the English Channel was estimated at 33.2 ± 6 tons year−1 [Citation26]. Compared to these estuary areas, influenced by industrial origin or the transport and diagenesis of marine sediments, arsenic output from SAYR was not that high but still will impact the ecosystem in the whole Yellow River basin.
3.2 Factors controlling arsenic distribution in SAYR
3.2.1 Arsenic enrichment in natural water
The source of arsenic can be divided into natural and anthropogenic, with more than 99% of the total As in the environment related to natural sources, including volcanism, weathering, hydrothermal and microbiological activities [Citation27]. Metal-(hydr)oxides and arsenic-rich sulfides are the most common natural arsenic forms [Citation28,Citation29]. Arsenic can be released to water phase via reductive dissolution of metal-(hydr)oxides, desorption from metal-(hydr)oxides, or oxidative dissolution of As-sulfides minerals [Citation30].
The high arsenic concentration in natural water in Tibet Plateau might be associated with a higher arsenic concentration in soil and the geothermal spring. The soil samples in SAYR, the arsenic concentrations range from 3.3 to 90.2 mg/kg within the depth of 1 m soil profile [Citation31]. The soil in Tibet has a considerably high background arsenic concentration, compared with the commonly cited crustal background value of 10 mg/kg [Citation32]. The arsenic enrichment in soil probably results from the weathering process of ophiolite melange and meta-sedimentary sequences containing shale, phyllite and schist that may be rich in arsenic [Citation32]. In addition, geothermal spring distributed in Tibet Plateau contained a high concentration of arsenic [Citation17], suggesting the bedrock of the geothermal might be arsenic sources too.
Chemically speaking, high arsenic concentration in SAYR possibly was mainly related to the dissolution of arsenic-rich sulfides or arsenic-bearing Fe-oxides since no significant correlation between iron and arsenic was observed in the river and lake water samples (). Arsenic and sulfate concentration had a significant positive correlation (p-value < 0.05) (), suggesting that in earth crust of SAYR area, arsenic might be co-precipitated with sulfides in sediment rocks [Citation33,Citation34].
Besides the high concentration in the soil and geothermal spring, the relatively high arsenic concentration compared with other pristine surface water systems might be due to the specified climate condition, which is beneficial for the enrichment of the arsenic in the water. SAYR was located in arid-semiarid region [Citation35]. The mean annual air temperature is lower than −4 °C, and the annual precipitation varies between 300 and 400 mm [Citation8]. The cold and arid climate condition can accelerate the weathering process and further the decomposition rate of bedrock and biogeochemical cycle, promoting the arsenic migrate and enrichment process. Reducing conditions can facilitate arsenic transport by allowing it to stay in solution or by liberating arsenic adsorbed to surfaces from geologic sources, such as permafrost sediments and bedrocks [Citation28,Citation30].
3.2.2 Permafrost and groundwater
The SAYR is wholly with permafrost [Citation8]. Since the 1970s, climate warming has resulted in the degradation of frozen ground, making the ground temperature rising; the thaw depth increasing, and permafrost becoming thinner [Citation8]. These all increase the residence time of the permafrost soil water, enhancing leaching and mobilization of the trace element like arsenic from frozen soil to surface waters in the permafrost zone [Citation36]. From 30-year stream water chemistry data set, Todd et al. [Citation37] attributed the increasing concentration of contaminant to the melting of permafrost and falling water table.
Groundwater was another important arsenic source in the surface water due to the low precipitation and temperature and high evaporation rate [Citation35]. The typical arsenic concentration in the groundwater is much higher in the pristine source area [Citation30]. From the spring water samples collected in this study (), the arsenic concentrations were much higher than river water samples.
3.2.3 Different arsenic formation mechanism in lake and river water
The arsenic level in lake water was higher than that in the river water. For the two largest lakes – the Gyaring and Ngoring lakes () – it might be due to that the two lakes were drainage outlet of over 70% of the SAYR area. They accumulated much of the arsenic in the lakes.
The different physical and chemical condition in the lake and river systems can also impact the arsenic release process from immobile status, making the arsenic concentration in the lake different from that in the river. Organic matter [Citation38,Citation39] and clay minerals content [Citation40] can enhance the mobility of the arsenic. They are richer in the lake sediment than in the river bedrock; hence, arsenic level is higher in lake water. In addition, since phosphate and arsenic have similar chemical properties, phosphate can compete with arsenic anions for adsorption sites [Citation41], therefore influence the mobility of arsenic. With sufficient phosphate in the water, organisms usually intake phosphate instead of arsenic for their metabolic activity, inducing arsenic enrichment in phosphate-rich lakes [Citation42]. High concentration of phosphate in the lake water of SAYR () helped the mobilization of dissolved the arsenic, increased the mobilized arsenic concentration. On the contrary, the phosphate concentrations in the river water were close to zero (), resulting in the lower arsenic concentration in river water.
4. Conclusions
The overall concentration of arsenic in SAYR surface water varied over the range of 4.3–77.1 μg/L in the river water, with 25.2 μg/L in average, while varied over the range 9.3–131.0 μg/L in lake water, with 39.6 μg/L in average. Generally speaking, concentrations of arsenic in lake water were higher than that in river water. The Arsenic in SAYR mainly came from the weathering process of arsenic-rich sulfides. Because the permafrost thawing process can extend the residence time of water in the soil, with the help of high clay content, accelerating permafrost thawing due to the climate change can increase the release of arsenic in the permafrost area. The arsenic concentration in SAYR was similar to that reported for other pristine regions on Tibet Plateau. It could be concluded that SAYR is pristine and can serve as a reference region for determining the human influence on permafrost-affected landscapes or comparing similar pristine areas in the permafrost-affected region related to arsenic.
Disclosure statement
No potential conflict of interest was reported by the authors.
Additional information
Funding
References
- Vonk JE, Tank SE, Bowden WB, et al. Reviews and syntheses: effects of permafrost thaw on Arctic aquatic ecosystems. Biogeosciences. 2015;12(23):7129–7167.
- Schuur EAG, Vogel JG, Crummer KG, et al. The effect of permafrost thaw on old carbon release and net carbon exchange from tundra. Nature. 2009;459(7246):556–559.
- Schuster PF, Schaefer KM, Aiken GR, et al. Permafrost stores a globally significant amount of mercury. Geophys Res Lett. 2018;45(3):1463–1471.
- Romanovsky VE, Drozdov DS, Oberman NG, et al. Thermal state of permafrost in Russia. Permafrost Periglacial Processes. 2010;21(2):136–155.
- Krainer K, Bressan D, Dietre B, et al. A 10,300-year-old permafrost core from the active rock glacier Lazaun, southern Ötztal Alps (South Tyrol, northern Italy). Quat Res. 2017;83(2):324–335.
- Williams MW, Knauf M, Caine N, et al. Geochemistry and source waters of rock glacier outflow, Colorado Front Range. Permafrost Periglacial Processes. 2006;17(1):13–33.
- O’Donnell JA, Jorgenson MT, Harden JW, et al. The effects of permafrost thaw on soil hydrologic, thermal, and carbon dynamics in an Alaskan Peatland. Ecosystems. 2012;15(2):213–229.
- Jin HJ, He R, Cheng G, et al. Changes in frozen ground in the source area of the yellow river on the Qinghai-Tibet Plateau, China, and their eco-environmental impacts. Environ Res Lett. 2009;4(4):045206.
- He J, Charlet L. A review of arsenic presence in China drinking water. J Hydrol. 2013;492:79–88.
- Huang L, Wu H, van der Kuijp TJ. The health effects of exposure to arsenic-contaminated drinking water: a review by global geographical distribution. Int J Environ Health Res. 2015;25(4):432–452.
- Xie Z, Sun Z, Zhang H, et al. Contamination assessment of arsenic and heavy metals in a typical abandoned estuary wetland-a case study of the yellow river delta natural reserve. Environ Monit Assess. 2014;186(11):7211–7232.
- Mandal BK, Suzuki KT. Arsenic round the world: a review. Talanta. 2002;58(1):201–235.
- Hossain MF. Arsenic contamination in Bangladesh - an overview. Agric Ecosyst Environ. 2006;113(1–4):1–16.
- Thakur JK, Thakur RK, Ramanathan AL, et al. Arsenic contamination of groundwater in Nepal-an overview. Water. 2011;3(1):1–20.
- Zhang Y, Sillanpää M, Li C, et al. River water quality across the Himalayan regions: elemental concentrations in headwaters of Yarlung Tsangbo, Indus and Ganges River. Environ Earth Sci. 2015;73(8):4151–4163.
- Tian L, Li Y, Yao T. Preliminary study on the arsenic concentration in surface water on the Tibetan Plateau. J Glaciol Geocryology. 2002;24(1):98–101.
- Li C, Kang S, Chen P, et al. Geothermal spring causes arsenic contamination in river waters of the southern Tibetan Plateau, China. Environ Earth Sci. 2014;71(9):4143–4148.
- Luo D, HuiJun J, Marchenko S, et al. Distribution and changes of active layer thickness (ALT) and soil temperature (TTOP) in the source area of the Yellow River using the GIPL model. Sci China-Earth Sci. 2014;57(8):1834–1845.
- Hu G, Jin H, Dong Z, et al. Driving forces of aeolian desertification in the source region of the Yellow River: 1975–2005. Environ Earth Sci. 2013;70(7):3245–3254.
- Wilkie JA, Hering JG. Rapid oxidation of geothermal arsenic(III) in streamwaters of the eastern Sierra Nevada. Environ Sci Technol. 1998;32(5):657–662.
- Pettine M, Mastroianni D, Camusso M, et al. Distribution of As, Cr and V species in the Po-Adriatic mixing area, (Italy). Mar Chem. 1997;58(3–4):335–349.
- Masson M, Schäfer J, Blanc G, et al. Seasonal variations and annual fluxes of arsenic in the Garonne, Dordogne and Isle Rivers, France. SciTotal Environ. 2007;373(1):196–207.
- Michel Pierre AB, Joelle N, Jane S. Evaluation of dissolved and particulate arsenic flux in the Dover strait (fluxmanche program). Oceanolog Acta. 1993;16(5–6):585–591.
- Jigyasu DK, Kuvar R, Singh S, et al. Seasonal variations and flux of arsenic in Gomati River, Ganga Alluvial Plain, Northern India. In: Raju NJ, Gossel W, Ramanathan AL, et al., editors, Management of water, energy and bio-resources in the era of climate change: emerging issues and challenges. Cham: Springer International Publishing; 2015. p. 85–96.
- De Gieter M, Elskens M, Baeyens W. Fluxes and major transport routes of arsenic in the scheldt estuary. Mar Chem. 2005;95(1–2):15–30.
- Michel P, Chiffoleau JF, Averty B, et al. High resolution profiles for arsenic in the seine estuary. seasonal variations and net fluxes to the English channel. Cont Shelf Res. 1999;19(15–16):2041–2061.
- Singh R, Singh S, Parihar P, et al. Arsenic contamination, consequences and remediation techniques: A review. Ecotoxicol Environ Saf. 2015;112:247–270.
- Ferguson JF, Gavis J. A review of the arsenic cycle in natural waters. Water Res. 1972;6(11):1259–1274.
- Maest AS, Pasilis SP, Miler LG, et al. Redox geochemistry of arsenic and iron in Mono Lake, California, USA. In: Kharaka YK, Maest AS, editors. Water-rock interaction, Vols 1 and 2: vol 1: low Temperature Environments; Vol 2: moderate and high temperate environments. Rotterdam, Netherlands: AA Balkema; 1992p. 507–511.
- Smedley PL, Kinniburgh DG. A review of the source, behaviour and distribution of arsenic in natural waters. Appl Geochem. 2002;17(5):517–568.
- Yu C, Sun Y, Yu Z, et al. Arsenic release mechanism with seasonal permafrost freeze-thaw process. J Contam Hydrol. Submitted.
- Li S, Wang M, Yang Q, et al. Enrichment of arsenic in surface water, stream sediments and soils in Tibet. J Geochem Explor. 2013;135:104–116.
- Rowland Helen AL, Omoregie EO, Millot R, et al. Geochemistry and arsenic behaviour in groundwater resources of the Pannonian Basin (Hungary and Romania). Appl Geochem. 2011;26:1–17.
- Mandal SK, Dey M, Ganguly D, et al. Biogeochemical controls of arsenic occurrence and mobility in the Indian Sundarban mangrove ecosystem. Mar Pollut Bull. 2009;58(5):652–657.
- Matsuoka N, Ikeda A, Sueyoshi T, et al. Permafrost and hydrology in the source area of the Yellow River. Bull Geol Surv Jpn. 2009;60(1–2):39–57.
- Antcibor I, Eschenbach A, Zubrzycki S, et al. Trace metal distribution in pristine permafrost-affected soils of the Lena River delta and its hinterland, northern Siberia, Russia. Biogeosciences. 2014;11(1):1–15.
- Todd AS, Manning AH, Verplanck PL, et al. Climate-change-driven deterioration of water quality in a mineralized watershed. Environ Sci Technol. 2012;46(17):9324–9332.
- McArthur JM, Banerjee DM, Hudson-Edwards KA, et al. Natural organic matter in sedimentary basins and its relation to arsenic in anoxic ground water: the example of West Bengal and its worldwide implications. Appl Geochem. 2004;19(8):1255–1293.
- Wang S, Mulligan CN. Effect of natural organic matter on arsenic release from soils and sediments into groundwater. Environ Geochem Health. 2006;28(3):197–214.
- Root TL, Gotkowitz MB, Bahr JM, et al. Arsenic geochemistry and hydrostratigraphy in Midwestern U.S. Glacial deposits. Ground Water. 2010;48(6):903–912.
- Guan X, Dong H, Ma J, et al. Removal of arsenic from water: effects of competing anions on As(III) removal in KMnO4-Fe(II) process. Water Res. 2009;43(15):3891–3899.
- Hasegawa H, Rahman MA, Matsuda T, et al. Effect of eutrophication on the distribution of arsenic species in eutrophic and mesotrophic lakes. SciTotal Environ. 2009;407(4):1418–1425.