ABSTRACT
The focus was to assess the effects of biochar on Cd uptake by various vegetables and its downward movement in saline-alkali soil and carried out in polluted pots experiment. The results showed that the biomass of vegetables increased significantly by 16.9% to 519.9% while the Cd concentration in green pepper and eggplant fruits was decreased by 6.8% to 11.5% and 15.1% to 15.4% with the biochar application. The Cd in contaminated soil profile was transferred from topsoil (0-6 cm) to subsoil (6-12 cm) that was observed as 14.30 ± 4.17 mg kg-1. The concentrations of Cd in soil with different fractions like exchangeable, reducible and oxidizable and the Cd uptake by vegetables were reduced. The release of residual Cd was decreased by 0.3 to 20.1% over time with biochar application (0-12 cm). The biochar was effective in mitigating the soil Cd pollution by preventing the vertical Cd leaching.
KEYWORDS:
1. Introduction
With the boost in the development of economy and anthropogenic activities (such as industry, agriculture, mining, etc.), the serious pollution hazards are rapidly posing threats on the environment [Citation1–3].The human activities contributed to maximum soil pollution than natural factors, and heavy metals were one of the most common pollutants in a soil environment [Citation4–6]. At present, the water-soluble fraction changes and migration of heavy metals to deeper soil layers have a greater effect on the plant uptake of metals [Citation7]. Many conventional remediation techniques, such as replacement, electro-kinetics, soil flushing, and phytoremediation, have been developed to reduce the bioavailability and mobility of Cd in agricultural soils [Citation8,Citation9]. Zhang et al [Citation3] found that −13.86% of grain production was affected due to the heavy metal pollution in farmland soil as a result of mining, smelting, industry, sewage irrigation, urban development, and fertilizer application releasing certain amounts of heavy metals into soil. The bioavailability of different forms of copper (Cu) and zinc (Zn) was decreased in Chinese cabbage with the application of 10% (w: w) calcium-based bentonite in the soil [Citation10].The different heavy metals fractions in the soil are transformed into stabilized forms after natural leaching [Citation11], thus minimizing the risk of rainfall water to increase shallow groundwater heavy metals pollution (As, Cd, Cu, and Pb) [Citation12].
Biochar is a carbonaceous material that can be produced from different biowastes such as agricultural crop straw, wood chips, sewage sludge and etc. using the pyrolysis technique [Citation13–15].The biochar derived from crop straw contains valuable macronutrients (especially N and P) and some metal ions (e.g., Ca2+ and Mg2+), which play an important role in improving the soil fertility and plant growth [Citation16]. In addition, using various biochars as a soil amendment has a great potential for stabilizing the Cd and hence improving the soil properties [Citation17–19]. The sodium carboxymethyl cellulose stabilized the biochar-supported iron phosphate nanoparticle (10%, w: v). It could effectively immobilize Cd up to 81.3% after 28 days of remediation. The bio-accessibility of Cd, based on the physiological-based extraction test, was reduced by 80.0%, and its uptake by the below-ground and above-ground parts of cabbage and mustard was decreased by 44.8% and 70.2%, respectively, thereby promoting cabbage and mustard growth after the remediation [Citation20]. The 1–3% (w: w) biochar reduced porewater Cd in rice rhizosphere, resulting in 49%–68% and 26%–49% reduction in the root and grain Cd uptake in rice, respectively [Citation21]. Using a wheat straw biochar at 40 t ha−1greatly reduced the rice grain Cd content by 20%-90%, and ensured a safe Cd level (<0.4 mg kg−1)in rice grain from Cd-contaminated rice fields (total Cd 20 mg kg−1).This could be explained by a reduction in the extractable Cd pool in the biochar treated soil and was closely correlated to the rise in soil pH [Citation22]. The biochar, derived from the heartwood of Areca catechu, was highly efficient to sorb Cd and bind it with hydroxyl, carboxyl, amide and amine groups [Citation23].The application of biochar as a soil amendment is also beneficial in improving the physical and chemical properties of soil (such as pH, SOM, soil microstructure etc), which influence the immobilization of heavy metals [Citation24]. Moreover, the poultry manure compost (12%, w:w) significantly increased soil pH and soil dissolved organic matter that may form complex with Cd (50 mg Cd kg−1 soil), thereby transforming 47.8%–69.8% of soluble/exchangeable Cd to the organic-bound fraction, and consequently decreasing Cd uptake of pakchoi by 56.2%–62.5% as compared to unamended soil [Citation25].The wheat straw waste (Hordeum vulgare L.) also improved the qualities of heavy metal contaminated soils and increased the microbial counts and soil enzyme activities in rhizosphere of vetiver grass (Chrysopogon zizanioides L.).This also enhanced the proportion of HOAc-extractable Cd and soil microbial ecology; the multiple biochemical materials significantly enhanced the Cd content and bioaccumulation factor (BCF) of grass by about 412.4% and 403.4%, respectively than that of control [Citation26].
Saline alkali soils are widely distributed in the world, which can cause drought stress to crops. The saline-alkaline soil (pH: 7.64) showed significant increase in available Cd in soil solution of about 34.1%–49.7%, soil extractable Cd of 32.0%–51.6% and wheat root Cd concentration of 24.5%–40.2% and decreased activities of antioxidative enzymes of wheat root compared with control treatment (no alkaline- saline soil) [Citation27]. Therefore, it is necessary to restore saline-alkaline soil to mitigate contamination and degradation. The biochar application can revitalize the degraded soils such as saline-alkali soil by improving the soil nutrients availability, soil water retention, and the growth of the plant [Citation28, Citation29–30]. Drake et al [Citation29] found that Acacia pycnantha-biochar (5 Mg ha−1) increased Eucalyptus Viminalis height, and decreased the concentration of Mn (Manganese), N (Nitrogen) and S (Sulphur) in plant in the highly saline-s0ic soil (EC: 49.4 dS m−1, Exchangeable Sodium Percentage: 45.1%).
The effect of various biochars on Cd immobilization and uptake by plants has been widely studied, but few studies have focused on the migration of different Cd fractions with low molecular weight organic pollution in saline-alkaline soils. Moreover, the remediation potential of biochar in saline soils polluted with both heavy metals and organic pollutants is still to be studied. Therefore, it was hypothesized that the Cd kinetics and movement are influenced by biochar application in soil contaminated with low molecular weight organic pollution/matter, and biochar would reduce the Cd transfer from bottom to top in the vegetables in saline-alkaline soil. Based on this hypothesis, combined with outdoor pilot-scale experiments, the effects of biochar on the vertical movement of Cd under natural leaching conditions were studied, which could also provide a theoretical basis for promoting biochar use as a soil amendment for remediation of polluted soils.
2. Materials and methods
2.1. Point distribution and sampling
The soil was collected at 0 to 20 cm depth from a field in Yancheng (33°24ʹ47.70”N, 120°17ʹ43.85”E) Jiangsu Province, China. The soil was air-dried and stones, as well as plant litter, were removed, and then the soil was passed through 5 mm sieve and prepared for the experiment. The heavy metal contaminated soil was created by placing 500 g air-dried soil into a 2 L glass beaker and mixing it with 250 mL stock [1.2 g Cd, Cd(NO3)2 · 4H2O, 99%, Sinopharm Chemical Reagent Co. Ltd, China]. Similarly, another 500g soil was added with 6 g 2,4,6-trichlorophenol (2,4,6-TCP, 98%, Sigma-Aldrich, USA) in 250 mL solution, and thoroughly mixed to create organic polluted soil. Each wooden box with dimensions i.e. length × width × height = 1 × 2 × 1 m was filled with the soil which was uniformly mixed with biochar at levels of 0% (no biochar application)and 5% (w/w).The soil bulk density was similar to that in the field (~1.41 g cm−3). The contaminated soils were air-dried and passed through a 2 mm sieve, before placing the mon the top of the soil in each box. Then the soil pollution concentrations were about 20 mg Cd kg−1 and 100 mg 2,4,6-TCP kg−1 for whole soil in each box. After synthetically preparing the polluted soils, the vegetables were planted in it under the natural condition. According to the depth of profile soil, the 0–6, 6–12, 12–18, 18–24, 24–30 cm soils were defined as S1, S2, S3, S4, S5, respectively.
The green pepper (Capsicum annuum L., Sujiao No. 5), eggplant (Solanum melongena L., green jade), and Chinese cabbage (Brassica chinensis L.) were planted in a different season. When the vegetables were matured, the soil and vegetables samples were collected and pretreated. At the same time, the profile soil was collected and divided into five equal parts every 6 cm, such as 0–6 cm, 6–12 cm, 12–18 cm, 18–24 cm and 24–30 cm using a core sampler (Eijkelkamp, Netherlands). Then the soil samples were air-dried, ground to pass through a 2-mm sieve, and stored for subsequent analyses, such as the basic soil properties. Some of the 2-mm sieved air-dried soil was further ground to pass through a 0.15-mm sieve for measurements of different Cd fractions and 2,4,6-TCPconcentration. The basic soil properties were analyzed following the procedures described by Lu [Citation31].
Biochar was produced through the anoxic pyrolysis of wheat straw at 450°C in a continuous process using a vertical kiln composed of refractory bricks at the Sanli New Energy Company, Henan Province, China. The basic biochar properties were determined following the methods recommended by the International Biochar Initiative [Citation32], and are listed in .
Table 1. Basic saline-alkali soil and biochar properties
2.2. Experiment design and samples analysis
In this experiment, six treatments were designed and represented as Cd pollution (PZ1), 2,4,6-trichlorophenol (2,4,6-TCP) pollution (PZ2), Cd and 2,4,6-TCPcombined pollution (PZ3), Cd pollution and biochar (PZ4), 2,4,6-TCP and biochar (PZ5), Cd and 2,4,6-TCPcombined pollution and biochar (PZ6).
The heavy metal and organic pollutants were added in pots on April 10th, 2018. The green pepper and eggplant were planted on July 23rd and Chinese cabbage on October 7th in the year 2018. When the plants were matured enough to be harvested, the samples of plants were collected and washed with tap water, then rinsed with purified water thrice. Each plant sample was separated into roots, shoots, and fruit (except Chinese cabbage). Every part was placed in an air convection oven at 105°C for 30 min and further dried to constant weight at 60°C for another 48 h [Citation31]. The dried samples were crushed, mixed, and homogenized, then stored in air-tight polyethylene bags prior to chemical analysis.
The ground fine plant sample weighing 0.50 g was added in 100 mL beaker containing 10 mL HNO3:HClO4 (4:1, v/v) acid mixture and kept overnight for primary digestion. Then the mixture was put in a sand bath at 100–150°C for 30 min, and the temperature was raised (200–250°C) for another 30 min. When smoke began to rise in the beaker, the beaker was shaken frequently with hands to prevent samples becoming black. Where it was necessary, some amount of acid mixture was further added until the solution in the beaker became colorless and then transparent. After cooling, the digested solution was washed into 25 mL volumetric flask with double deionized water. The solution was filtered with filter paper and stored in refrigerator for further analysis.
The Cd fractions of soil samples were extracted according to the European Community Bureau of Reference (BCR) method, the brief process is shown in .
Table 2. The modified BCR sequential extraction
After the end of sequential extraction, the extract was separated from the solid residue by centrifugation using a centrifuge instrument (Eppendorf 5417R, Germany). The residual Cd/Pb from last step (such as step 1, 2 and 3) in the centrifuge tube was prepared for the next step. The separated solution was transferred to a 25 mL volumetric flask and then filtered. The heavy metals (Cd, Pb) contents in solutions were detected by flame atomic absorption spectrometry (FAAS, Zeenit 700p, Jena, Germany). The quality control of soil analysis for heavy metals was carried out by using national standard substances and their recovery rates were 95.6%-104.7% (Pb) and 96.8%-105.0% (Cd).
The 10 g soil was transferred to a 150 mL Erlenmeyer flask, 40 mL of n-hexane and propanone (chromatographically pure, v:v = 1:1) were added, and then the slurry was kept overnight and sonicated for 01hours. The soil slurries were then centrifuged (3500 rpm) for 10 minutes and the supernatant was filtered through a 0.45-μm nylon membrane. The solution was then analyzed for 2,4,6-TCP by gas chromatography (GC, Agilent 7890B) with an flame ionization detector (FID) detector equipped with a reverse-phase column, 60 m × 0.32 mm×0.25 μm. Helium was the carrier gas (1.5 mL min-1), and nitrogen was the make-up gas (60 mL min−1). The pressure was set at 13.4 Psi. The temperatures of the injector and detector were kept at 260°C and 320°C, respectively. The oven temperature was programmed from 60 to 170°C (2 min hold) at a rate of 25°C per min to 250°C (26 min hold). The injection volume was 1.0 μL splitless. All determinations were performed in triplicate [Citation33].
2.3. Statistical analysis
All data were expressed as means with plus or minus one standard deviation. Differences between the treatments were examined using a Student’s t-test in a two-way analysis of variance (ANOVA) and considered significant when p < 0.05. All statistical analyses were carried out using SPSS, version 22.0 (SPSS Institute, USA) and Origin 8.0 (Origin Lab, USA).
3 Results and discussion
3.1. Plant biomass and Cd uptake in two seasons
The biomass and Cd content of plants in the first season are shown in . The PZ4, PZ5, and PZ6 biochar treatments showed that the biomass of green pepper increased by 79.3%, 16.9%, and 28.2%, respectively compared with PZ1, PZ2, and PZ3. While in the PZ4, PZ5, and PZ6 treatments, the eggplant biomass increased by 519.9%, 42.2%, and 26.7%, respectively compared with PZ1, PZ2, and PZ3. The addition of biochar increased the biomass of green pepper and eggplant, especially in the soil polluted with heavy metals. The Cd content in green pepper and eggplant fruits were increased by 6.8%-11.5% and 15.1%-15.4%, respectively with biochar application. In the Chinese cabbage season, the biomass of Chinese cabbage in PZ4 was decreased by 54.0% compared with PZ1, but the biomass was increased by 484.3% and 289.1% in PZ5 and PZ6, respectively compared with PZ2 and PZ3 (). The vegetable species showed different trends in the uptake of the heavy metals in this experiment. The effects of biochar on improving soil properties were greater than that of Cd contamination on the biomass. The biochar improved the soil nutrition status and increased plant yield in the field experiment, similar results were also found in the other research studies [Citation27,Citation34]. Chen et al [Citation35] also reported that biochar application reduced rice grain Cd contents as much as 61% and 57% by using two low Cd accumulator rice cultivars in 0.9 mg kg−1 of Cd contaminated soil. A contradictory result showed that soil Cd contamination has led to a serious reduction of the crop yield [Citation3].
Table 3. Biomass and Cd uptake of green pepper, eggplant and Chinese cabbage
The Cd concentration in the vegetables is shown in . The Cd absorbed by green pepper stems was about 50% Cd of the whole plant, indicating that the green pepper has a greater ability to sorb the Cd. The Cd concentration of eggplant roots, leaves and fruits was uniform with the root concentration relatively higher than other parts. The Chinese cabbage leaves were the main pool to store the Cd, which was depended on the properties of the plant. The concentration of Cd in roots in Chinese cabbage was decreased by 1.6%-1.8% with biochar application compared with control (). The biochar decreased the bioavailable Cd and also promoted plant growth. Increasing the amount of biochar in the surface soil not only increased the aboveground biomass of Chinese cabbage but also enhanced the absorption capacity of Cd by Chinese cabbage. Therefore, the effect of biochar in improving the growth of Chinese cabbage with Cd absorption is stronger than that of biochar fixation [Citation36].
3.2. Effects of biochar on soil pH and soil organic matter
The soil pH and soil organic matter (SOM) changes were listed in . Compared with no biochar application, the surface soil pH of treatments PZ4 and PZ6 was increased by 0.02–0.58 unit, while the soil pH of PZ5 was not changed significantly. The pH of the soil with PZ4 and PZ6 treatments was increased by 0.07–0.35 at S2 (6–12 cm), while the pH of PZ5 was decreased by 0–0.14 unit ()). The topsoil pH was decreased by 0.02–0.56 units during the Chinese cabbage growth compared with the green pepper season in the same treatment, while the subsoil pH was decreased by 0.02–0.35 unit ()). The soil properties (such as pH, organic matter, etc.) played an important role in the contaminated soil mechanisms [Citation37]. The biochar has higher pH and cation-exchange capacity [Citation38] and contains more alkaline substances, such as carbonate and oxides, which increased alkaline ion exchange and soil pH [Citation39].
Figure 2. Changes of soil pH and soil organic matter in the profile during the two seasons (a: soil pH in green pepper season; b: soil pH in Chinese cabbage season; c: SOM in green pepper season; d: SOM in Chinese cabbage season)
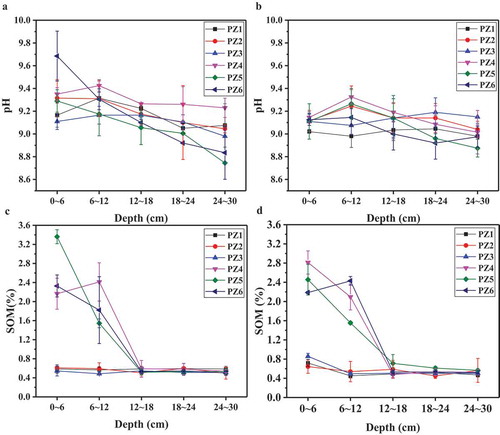
With the application of biochar in soil, the soil organic matter (SOM) content was increased by 1.0%-2.8% (). Without the biochar application, the SOM content in surface soil in the Chinese cabbage season was 1.1–1.6 times higher compared with the green pepper season under the same treatment. The SOM was mainly in the surface soil and subsoil, which was attributed to the S1 application of biochar. The surface SOM was usually higher than the subsoil because the soil was mixed with biochar and promoted plant growth. Owing to the special physicochemical properties, biochar provides abundant organic carbon which is a good source to assemble soil particles [Citation40]. When the biochar was applied to the contaminated soil, biochar could alter soil physicochemical properties (such as to enhance soil carbon sequestration and soil fertility) and immobilize soil contaminants [Citation41,Citation42]. The forms of heavy metals in soils were also subject to changes as result of alteration in pH, redox potential, soil organic matter and cation exchange capacity [Citation43,Citation44]. Moreover, by improving the soil properties, the application of biochar can significantly promote plant growth environment and increase plant biomass [Citation45]. Different plants have different abilities to uptake Cd, and the ability of plants to absorb cadmium may change with the change of soil physical and chemical properties [Citation46].
3.3. Effect of biochar on Cd vertical migration
The total amount of Cd in the soil under different treatments varies with different depth of the soil profile, as shown in . With the passage of time, the Cd contamination in the topsoil moved downward to the S2 (6–12 cm) in green pepper season, reaching 9.0–19.0 mg kg−1 ()). The Cd concentration in S1 (0–6 cm) decreased by 33.8%-38.4% in Chinese cabbage season under PZ1, PZ3 and PZ6 treatments compared with green pepper season, while the Cd content of biochar treated soil (PZ4) increased by 1.6% ()). This may be attributed to the low biomass of Chinese cabbage in PZ4 compared with others. Cadmium content in PZ1, PZ4, and PZ6 S2 (6–12 cm) in Chinese cabbage season was 26.8% – 62.8% lower than that in green pepper season, while the content of Cd in PZ3 increased by 51.2% compared with green pepper season. The uptake of Cd with PZ3 treatment was the lowest in Chinese cabbage (), and the Cd showed downward movement to S2 (6–12 cm). The Cd leaching was attributed to the rainfall, irrigation, and low molecular weight organic matter and several other factors.
Figure 3. Soil Cd Concentration Change with different depth (a: green pepper season; b: cabbage season)
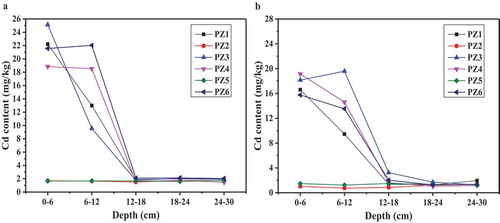
The biochar application and Cd pollution promoted the 2,4,6-TCP degradation in the soil by 53.8% (PZ3), 54.2%(PZ5) and ~100% (PZ6) compared with PZ2 (Table S1).In the open-air environment, the wheat straw biochar application in Cd polluted soil effectively increased the degradation ratio of 2,4,6-TCP in the soil. In the cabbage season, the 2,4,6-TCP concentration was under the detection limit, which was probably due to the complexed environmental factors. The 5% (w: w) pine chip biochar decreased Pb bioavailability and reduced the earthworms and grasses uptake, which has a positive relationship with organochlorine pesticide degradation in the pot experiment [Citation47]. Cui et al. [Citation48] also found that the 5% (w: w) wheat straw biocharreduced 2,4,6-TCP downward transport and promoted the degradation, which is correlated with improving soil water and allowed 2,4,6-TCP to be more easily transported to biochar particles.
3.4. Fractions changes of Cd during vertical migration
The Cd fractions changes and transfer in green pepper and eggplant are shown in ). The reducible and oxidizable Cd concentration of S1 treated with biochar (PZ4) was increased by 8.4% and 2.7%while the exchangeable and residual fractions Cd concentrations were decreased by 7.0% and 4.1%, respectively compared with no biochar application (PZ1) ()). The results showed that an increase in soil pH leads to the conversion of Cd into more immobile form (such as insoluble precipitation compound etc.) [Citation39,Citation49]. The properties of biochar (the pH, functional groups, the micropore structure) generally have greater effects on the Cd2+ hydrolysis and the formation of organic and carbonates bonds [Citation50]. In a previous study, Cui et al. [Citation51] investigated different soil Cd fractions using a sequential extraction procedure, followed by definitive Cd sorption analysis via XAS. They found that increasing pH played a significant role in Cd bioavailability reduction likely due to precipitation reactions with 15% (w: w) biochar application. Compared with PZ3, the reducible, oxidizable and residue fractions of Cd were decreased by 3.8%, 1.3% and 0.2% with PZ6 treatment, but the ratio of exchangeable fraction was increased by 5.2%. The exchangeable, reducible and oxidizable Cd at S2 (6–12 cm) were increased by 14.9%, 15.0% and 4.8% in PZ4 compared with PZ1 (), but the residue fraction was decreased by 34.7%. Compared with PZ1, the exchangeable, reducible and oxidable forms of Cd were increased by 9.2%, 0.9%, and 1.9% respectively at subsoil compared with PZ6, while the relative proportion of residual Cd was decreased by 12.0% (). The proportion of exchangeable and carbonate-bound Cd fractions in surface contaminated soil was more than 90%, while that of residual Cd fraction was less than 10%, suggesting that biochar application immobilized the most of Cd in contaminated soil. Guo et al [Citation52]. showed that the hydrated lime (11.15 t ha−1) mainly increased soil carbonates bound Cd fractions in a range from 16.8% to 28.3%, and hydroxyapatite (22.25 t ha−1) increased Fe/Mn oxides Cd fractions in a range from 31.4% to 42.1% in the Cd contaminated soils (Cd 1.47 mg kg−1).
Figure 4. Cd Fraction Distribution in Soil Profiles PZ1, PZ3, PZ4 and PZ6 in Two Seasons [a (PZ1), b(PZ3), c(PZ4) and d(PZ6) in green pepper season; e (PZ1), f(PZ3), g(PZ4) and h(PZ6) in Chinese cabbage season] (R1: Exchangeable Cd, R2: Reducible Cd,R3: Oxidizable Cd, R4: Residual Cd)
![Figure 4. Cd Fraction Distribution in Soil Profiles PZ1, PZ3, PZ4 and PZ6 in Two Seasons [a (PZ1), b(PZ3), c(PZ4) and d(PZ6) in green pepper season; e (PZ1), f(PZ3), g(PZ4) and h(PZ6) in Chinese cabbage season] (R1: Exchangeable Cd, R2: Reducible Cd,R3: Oxidizable Cd, R4: Residual Cd)](/cms/asset/2bd87b24-7659-4939-90a2-cbe99297278f/tcsb_a_1714487_f0004_b.gif)
The Cd fractions changes were shown in the cabbage season (). Without biochar amendment (PZ1), the reducible and oxidized Cd concentrations were increased by 6.3% and 4.2% compared with PZ4 (), while exchangeable and residual fractions Cd were relatively decreased by 10.2% and 0.2%, respectively. In the presence of biochar, Cd was likely initially to form mineral precipitates and was converted to organically bound phases over time [Citation51]. Compared with PZ3, the proportion of exchangeable and oxidizable Cd fractions was decreased by 2.2% and 0.9%, while the proportion of reducible state and residue fractions Cd was increased by 2.3% and 0.7% in PZ6. The ratios of exchangeable, reducible and oxidizable Cd at subsoil were increased by 0.7%, 7.0% and 7.6% in PZ4 compared with PZ1 (), but the residual Cd fraction was decreased by 15.3%. The treatment PZ6 showed that the exchangeable Cd fraction was increased by 6.3% at subsoil (), while the reducible, oxidable and residual fractions Cd were decreased by 4.1%, 1.6%, and 0.7% compared with PZ1.
In the case of the green pepper season, the residual Cd fraction of S1 (0–6 cm) was decreased by 0.3–4.2% in all treatments. The residual Cd fraction of S2 (6–12 cm) was decreased by 0.04–20.1% (), but the residual Cd fractions under PZ4 and PZ6 treatment were lower than PZ1 and PZ3 in Chinese cabbage season. In the 0–12 cm soil layer of PZ1 (), the proportion of exchangeable and reducible Cd in Chinese cabbage season was increased by 1.4–10.1% in green pepper season, while that in PZ4 and PZ6 treated with biochar decreased by 1.8–8.2% (). The ratio of residual Cd was decreased with biochar application, which indicated that the residual Cd in soil was released in a long time, while the biochar could effectively reduce the releasing of residual Cd in soil. Qiao et al. [Citation20] found that the Chinese herb medicine residue biochar immobilized 81.3% Cd in contaminated soil following28 days of incubation, with shifting from exchangeable (i.e., bioavailable) to residual and organically bound phases (i.e., less bioavailable). In this study, the ratio of oxidizable Cd was increased by 0.9–2.8% in soil (0–12 cm) in all treatments compared with no biochar application, indicating that the proportion of Cd oxidizable was higher with longer time. Cui et al. [Citation51] reported that when biochar was added, the Ca, Mg and K found on the adsorption sites of biochar were dissociated and replaced by heavy metals, such as Cd and Pb in granular wood-derived biochar
The ratio of each Cd fraction was improved with biochar application. During two seasons, the biochar treatments in PZ3 and PZ6 resulted in decreased exchangeable Cd fraction with increasing soil depth, while the ratio of reducible Cd was increased by 4.9%-17.7% in the topsoil and subsoil, suggesting that biochar application tends to reduce the release of residual Cd in Chinese cabbage season (). Xiao et al. [Citation53] found that maize straw biochar reduced DTPA extractable Cd by 6% to 27% and changed Cd fractions and their bioavailability in an alkaline soil contaminated with Cd (11.8 mg Cd kg−1).
Table 4. Percentage of soil Cd fraction in PZ3 and PZ6 Profiles (%)
3.5. Principal component analysis (PCA)
The PCA was used to evaluate the relationships among all variables, and possible sources of these elements analyzed. Two principal components PC1, PC2and PC3 were extracted whose eigenvalues were higher than 1. The PC1, PC2and explained 52.3%,30.0% and 10.7% of the variation respectively, the cumulative variance contribution of PC1, PC2and PC3was92.97%. From , it can be seen that the PC1 mainly includes exchangeable, reducible, and oxidizable Cd, along with Pb and Cd uptake by plants, their respective eigenvalues are 0.172, 0.169, 0.168, 0.111, 0.168, and 0.141 which indicate that they are from similar sources. The PC1 eigenvalues of SOM, pH and biochar were – 0.074, – 0.098 and – 0.035, and the main components of PC2 were 0.260, 0.219 and 0.296, which are medium loads with similar sources. The 2,4,6-TCP eigenvalues of PC1 and PC2 were −0.107 and −0.181, and the PC3 was 0.472. The factors of PC3 mainly included pH and 2,4,6-TCP. The eigenvalues of Pb and Cd uptake by eggplant were 0.333, 0.421, 0.626 and 0.168, indicating who came from similar sources. The 2,4,6-TCP had interaction with Cd absorption and soil pH of eggplant. However, the degradation of 2,4,6-TCP was relatively fast in green pepper season. The soil pH was significantly negatively correlated with the available Cd content (such as exchangeable Cd), whereas the available Cd content in soils was significantly positively correlated with the Cd contents in the vegetables. Zhan et al. [Citation54] also found similar results and reported that the rice straw biochar (2.25 kg m−2) improved the soil properties and decreased the Cd bioavailability. Moreover, they observed a strong significant negative correlation between the soil pH and available Cd content in soils, which in turn have a strong significant positive correlation with the Cd content in maize plants and grains.
There was a significant correlation between SOM and pH (p ≤ 0.01), while the correlations among exchangeable, reducible, oxidizable Cd and Cd uptake by plants were also high (data are not shown). The biochar application improved the interactions among the soil properties, Cd fractions and Cd concentration in soil and vegetables, which results in a reduction of the Cd hazardous environmental risk [Citation51].
4. Conclusion
The biochar effectively increased the biomass of vegetables under different pollution levels and reduced the proportion of exchangeable and reducible Cd by 6.8%-11.5% and 15.1%-15.4% in green pepper and eggplant fruits, respectively. The content of Cd was decreased by about 1.6% in Chinese cabbage root with the biochar application. The ratio of residual Cd in the treatment without biochar was decreased by 12.0–20.0% in Chinese cabbage season compared with green pepper season, while the biochar addition decreased residual Cd by 0.1–0.7%. The biochar was effective in reducing the soil residual Cd release in the long-term. Moreover, the biochar application accelerated the degradation of 2,4,6-TCP in soil, which was almost completely degraded in the natural environment before moving down to the subsoil.
The biochar prevented Cd transformation from surface soil to subsoil and protected the underground water from Cd pollution. The fractions of Cd and its uptake in plants were highly correlated with the soil properties, which have a greater contribution to Cd immobilization.
Supplemental Material
Download MS Word (16.4 KB)Acknowledgments
This work was supported by the National Natural Science Foundations of China (21677119, 41501339), Postgraduate Research & Practice Innovation Program of Jiangsu Province (SJCX17-0687) and Jiangsu Province Science Foundation for Youths under a grant number of BK20170475. The chemical properties, FTIR, TGA, SEM-EDS, and XPS were performed in the Analytical and Testing Center of Yancheng Institute of Technology.
Disclosure statement
No potential conflict of interest was reported by the authors.
Supplementary material
Supplemental data for this article can be accessed here.
- Cui L, Pan G, Li L, et al. Continuous immobilization of cadmium and lead in biochar amended contaminated paddy soil: A five-year field experiment. Ecol Eng. 2016;93:1–8.
- Wei B, Yang L. A review of heavy metal contaminations in urban soils, urban road dusts and agricultural soils from China. Microchem J. 2010;94(2):99–107.
- Zhang X, Zhong T, Liu L, et al. Impact of soil heavy metal pollution on food safety in China. Plos One. 2015;10(8):e0135182.
- Fellet G, Marmiroli M, Marchiol L. Elements uptake by metal accumulator species grown on mine tailings amended with three types of biochar. SciTotal Environ. 2014;468–469:598–608.
- Waqas M, Khan S, Qing H, et al. The effects of sewage sludge and sewage sludge biochar on PAHs and potentially toxic element bioaccumulation in Cucumis sativa L. Chemosphere. 2014;105(3):53–61.
- Yong SO, Usman ARA, Lee SS, et al. Effects of rapeseed residue on lead and cadmium availability and uptake by rice plants in heavy metal contaminated paddy soil. Chemosphere. 2011;85(4):677–682.
- Cui L, Pan G, Li L, et al. The reduction of wheat Cd uptake in contaminated soil via biochar amendment: a two-year field experiment. Bioresources. 2012;7(4):5666–5676.
- Cui L, Chen T, Cheng D, et al. Spatial distribution of total halogenated organic compounds (TX), adsorbable organic halogens (AOX), and heavy metals in wetland soil irrigated with pulp and paper wastewater. Chem Speciation Bioavailability. 2017;29(1):15–24.
- Tang X, Li Q, Wu M, et al. Review of remediation practices regarding cadmium-enriched farmland soil with particular reference to China. J Environ Manage. 2016;181:646–662.
- Zhao J, Wang Q, Ren X, et al. Effect of Ca-bentonite on Cu and Zn forms in compost and soil, and their absorption by Chinese cabbage. Environ Sci. 2018;39(4):1926–1933.
- Shangguan Y, Qin X, Zhao D. Migration and transformation of heavy metals in soils by lysimeter study with field condition. Res Environ Sci. 2015;28(7):1015–1024.
- Yang J, Chen T, Lei M. Risk of heavy metal pollution in soil and crops by reclaimed water irrigation in Beijing. J Nat Resour. 2011;(2):209–217.
- Ahmad M, Rajapaksha AU, Lim JE, et al. Biochar as a sorbent for contaminant management in soil and water: A review. Chemosphere. 2014;99(3):19–33.
- Fan Q, Sun J, Chu L, et al. Effects of chemical oxidation on surface oxygen-containing functional groups and adsorption behavior of biochar. Chemosphere. 2018b;207:33–40.
- Lehmann J. A handful of carbon. Nature. 2007;447(7141):143–144.
- He H, Tam NFY, Yao A, et al. Growth and Cd uptake by rice (Oryza sativa) in acidic and Cd-contaminated paddy soils amended with steel slag. Chemosphere. 2017;189:247-254.
- Fan Q, Cui L, Quan G, et al. Effects of wet oxidation process on biochar surface in acid and alkaline soil environments. Materials. 2018a;11(12).
- Suksabye P, Pimthong A, Dhurakit P, et al. Effect of biochars and microorganisms on cadmium accumulation in rice grains grown in Cd-contaminated soil. Environ Sci Pollut Res. 2016;23(2):962–973.
- Yin D, Wang X, Chen C, et al. Varying effect of biochar on Cd, Pb and As mobility in a multi-metal contaminated paddy soil. Chemosphere. 2016;152:196–206.
- Qiao Y, Wu J, Xu Y, et al. Remediation of cadmium in soil by biochar-supported iron phosphate nanoparticles. Ecol Eng. 2017;106:515–522.
- Yin D, Wang X, Peng B, et al. Effect of biochar and Fe-biochar on Cd and As mobility and transfer in soil-rice system. Chemosphere. 2017;186:S0045653517311773.
- Bian R, Chen D, Liu X, et al. Biochar soil amendment as a solution to prevent Cd-tainted rice from China: results from a cross-site field experiment. Ecol Eng. 2013;58(Complete):378–383.
- Chakravarty P, Sarma NS, Sarma HP. Biosorption of cadmium(II) from aqueous solution using heartwood powder of Areca catechu. Chem Eng J. 2010;162(3):949–955.
- Mohamed I, Ahamadou B, Li M, et al. Fractionation of copper and cadmium and their binding with soil organic matter in a contaminated soil amended with organic materials. J Soils Sediments. 2010;10(6):973–982.
- Chen HS, Huang QY, Liu LN, et al. Poultry manure compost alleviates the phytotoxicity of soil cadmium: influence on growth of Pakchoi (Brassica chinensis L.). Pedosphere. 2010;20(1):63–70.
- Wu B, Wang Z, Zhao Y, et al. The performance of biochar-microbe multiple biochemical material on bioremediation and soil micro-ecology in the cadmium aged soil. SciTotal Environ. 2019;686:719–728.
- Wang M, Chen S, Chen L, et al. Responses of soil microbial communities and their network interactions to saline-alkaline stress in Cd-contaminated soils. Environ Pollut. 2019;252:1609–1621.
- Yuan P, Wang J, Pan Y, et al. Review of biochar for the management of contaminated soil: preparation, application and prospect. SciTotal Environ. 2019;659:473–490.
- Gu C, Bai Y. Heavy metal leaching and plant uptake in mudflat soils amended with sewage sludge. Environ Sci Pollut Res. 2018;25(31):31031–31039.
- Drake J, Cavagnaro TR, Cunningham SC, et al. Does biochar improve establishment of tree seedlings in saline sodic soils. Land Degrad Dev. 2016;27(1):52–59.
- Lu RK, Zhu HZ, He PA, et al. Methods of inorganicpollutants analysis. In: Soil and agro-chemical analysismethods. Beijing: Agricultural Science and Technology Press; 2000. p. 205–266.
- Initiative IB 2012. Standardized product definition and product testing guidelines for biochar that is used in soil. IBI biochar standards.
- Zhang Z, Hong H, Zhou JL, et al. Fate and assessment of persistent organic pollutants in water and sediment from Minjiang River Estuary, southeast China. Chemosphere. 2003;52(9):1423–1430.
- Kaudal BB, Aponte C, Brodie G. Biochar from biosolids microwaved-pyrolysis: characteristics and potential for use as growing media amendment. J Anal Appl Pyrolysis. 2018;130:181–189.
- Chen D, Guo H, Li R, et al. Low uptake affinity cultivars with biochar to tackle Cd-tainted rice — A field study over four rice seasons in Hunan, China. SciTotal Environ. 2016;541:1489–1498.
- Wang L, Lin H, Dong Y, et al. Isolation of vanadium-resistance endophytic bacterium PRE01 from Pteris vittata in stone coal smelting district and characterization for potential use in phytoremediation. J Hazard Mater. 2017;341:1–9.
- Leibold MA, McPeek MA. Coexistence of the niche and neutral perspectives in community ecology. Ecology. 2006;87(6):1399–1410.
- Spokas KA, Novak JM, Stewart CE, et al. Qualitative analysis of volatile organic compounds on biochar. Chemosphere. 2011;85(5):869–882.
- Novak JM, Busscher WJ, Laird DL, et al. Impact of biochar amendment on fertility of a southeastern coastal plain soil. Soil Sci. 2009;174(2):105–112.
- Anderson CR, Condron LM, Clough TJ, et al. Biochar induced soil microbial community change: implications for biogeochemical cycling of carbon, nitrogen and phosphorus. Pedobiologia. 2011;54(5–6):0–320.
- Chen B, Zhou D, Zhu L. Transitional adsorption and partition of nonpolar and polar aromatic contaminants by biochars of pine needles with different pyrolytic temperatures. Environ Sci Technol. 2008;42(14):5137–5143.
- Jeffery S, Bezemer TM, Cornelissen G, et al. The way forward in biochar research: targeting trade-offs between the potential wins. Global Change Biol Bioenergy. 2015;7(1):1–13.
- Abd-Alla MH, Morsy FM, El-Enany A-WE, et al. Isolation and characterization of a heavy-metal-resistant isolate of Rhizobium leguminosarum bv. viciae potentially applicable for biosorption of Cd2+ and Co2+. Int Biodeterior Biodegrad. 2012;67(2):48–55.
- Deng Z, Zhang R, Shi Y, et al. Characterization of Cd-, Pb-, Zn-resistant endophytic sp. MXSF31 from metal accumulating and its potential in promoting the growth of rape in metal-contaminated soils. Environ Sci Pollut Res Int. 2014;21(3):2346–2357.
- Wu Y, Chen B. Effect of fulvic acid coating on biochar surface structure and sorption properties towards 4-chlorophenol. Sci Total Environ. 2019;691:595–604.
- Rodríguez-Vila A, Forján R, Guedes RS, et al. Changes on the phytoavailability of nutrients in a mine soil reclaimed with compost and biochar. Water Air Soil Pollut. 2016;227(12):453.
- Centofanti T, Chaney RL, Beyer WN, et al. Assessment of trace element accumulation by earthworms in an orchard soil remediation study using soil amendments. Water Air Soil Pollut. 2016;227(9):350.
- Cui L, Yin C, Chen T, et al. Biochar immobilizes and degrades 2,4,6-Trichlorophenol in soils. Environ Toxicol Chem. 2019b;38(6):1364–1371.
- Vivas A, Barea JM, Azcón R. Interactive effect of Brevibacillus brevis and Glomus mosseae, both isolated from Cd contaminated soil, on plant growth, physiological mycorrhizal fungal characteristics and soil enzymatic activities in Cd polluted soil. Environ Pollut. 2005;134(2):257–266.
- Chen H, Yang X, Wang P, et al. Dietary cadmium intake from rice and vegetables and potential health risk: A case study in Xiangtan, southern China. SciTotal Environ. 2018;639:271–277.
- Cui L, Noerpel MR, Scheckel KG, et al. Wheat straw biochar reduces environmental cadmium bioavailability. Environ Int. 2019a;126:69–75.
- Guo F, Ding C, Zhou Z, et al. Effects of combined amendments on crop yield and cadmium uptake in two cadmium contaminated soils under rice-wheat rotation. Ecotoxicol Environ Saf. 2017;148:303–310.
- Xiao R, Wang P, Mi S, et al. Effects of crop straw and its derived biochar on the mobility and bioavailability in Cd and Zn in two smelter-contaminated alkaline soils. Ecotoxicol Environ Saf. 2019;181:155–163.
- Zhan F, Zeng W, Yuan X, et al. Field experiment on the effects of sepiolite and biochar on the remediation of Cd- and Pb-polluted farmlands around a Pb-Zn mine in Yunnan Province, China. Environ Sci Pollut Res Int. 2019;26(8):7743–7751.