ABSTRACT
The consumption of commercial bottled water worldwide has increased surprisingly. To ensure that its consumption has no harmful effects on human health, research must be carried out with living organisms. The growth of Nicotiana tabacum plants was analyzed after being irrigated with tap and commercial bottled water. Plants irrigated with commercial bottled water had leaves with chlorosis, smaller number of leaves with a shorter length of stem and leaf, compared to plants irrigated with tap water, and root, stem and leaf showed an increase of reactive oxygen species production with a significant decrease of chlorophylls. Chemical analysis of commercial bottled water showed a low ion concentration, and an acidic pH value (5.5) which is below the minimum of Mexican NOM-127-SSA1-2000 pH value standard (6.5–8.5). The growth, chlorophyll concentration and antioxidant capacity of plants irrigated with bottled water are negatively affected compared to those irrigated with tap water.
Graphical abstract

Introduction
There is an increase in commercial bottled water consumption worldwide and Mexico is one of the leading consumer countries [Citation1]. In Mexico, introduction of commercial bottled water historically was due to drinking water scarcity, to meet urgent needs during natural disasters and to provide it to the population of cities in desert areas [Citation2] as well as the presence of pathogenic microorganisms in drinking water [Citation3,Citation4].
The high consumption of commercial bottled water has led to research of the following three essential aspects. i) The mineral and organic composition, also radioactivity and microbiological contaminants, because they can have serious effects on human health [Citation5]. In the case of bottled water in Mexico, residual organochlorine pesticides, alpha radioactivity and presence of pathogen microorganisms such as total coliforms were found, all of those surpassed the limits for drinking water [Citation6,Citation7,Citation4]. ii) The water disinfection method, because derived compounds can react with dissolved organic ions in water, such as halogen compounds or nitrosamines which have been reported in drinking water [Citation8,Citation9]. Finally, iii) the type of plastic in which water is bottled has received special interest, because the polycarbonate plastics and resins employed for its elaboration [Citation10] may be able to liberate different elements and organic compounds to food or water [Citation11], which can affect the human endocrine system [Citation12]. For example, antimony (Sb) and phthalates were found in commercial bottled water of Monterrey City, Mexico, their presence is derived from polyethylene terephthalate (PET) plastic used for bottled water, both are considered toxic and hazardous elements in the environment [Citation13,Citation14].
In most of those research works, the objective is to determine whether quality of water intended for human consumption complies with established standards in the legislation of each country, to ensure that there is no risk to human health. However, direct evaluation of effects of prolonged consumption of commercial bottled water in human is scarce, probably because the effects on health are variable, such as the length of time in which first health problems appear, which may vary from months to years among people. Therefore, the use of living organisms, such as plants or animals is preferred in the study of diseases or therapies, due to the responsiveness or evolution to changes in its environment [Citation15]. Plants are widely used to monitor environmental pollution and, among plants used for this purpose, tobacco plants proved to be useful [Citation16]. Recently it has been demonstrated that gene expression and specific activity of enzymes of central metabolism for example NADP-malic oxidoreductase of N. tabacum are sensitive to diverse abiotic stressors, such as the changes of salinity, temperature, pH and drought, among others [Citation17,Citation18].
There are three facts that show the need for research on the adverse effects of human consumption of bottled water. Firstly, the research of drinking water quality to evaluate the risks on the health of Mexicans has been focused only on the analysis of mineral and organic components, radioactivity and biological contaminants. Secondly, there are few studies conducted to assess the effects of drinking commercial bottled water in live organisms. Finally, there is a high consumption of commercial bottled water in Mexico. To evaluate the possible health effects of drinking commercial water one could start by investigating the adverse effects on animal or plants models. Plants are adequate models because they have relative short life cycles and have been used to investigate effects of contaminants. In this work the growth of Nicotiana tabacum plants irrigated with commercial bottled water for 55 days was evaluated and compared with that of plants irrigated with tap water by measuring morphological and biochemical parameters.
Material and methods
Plants were grown according to Citation19. Seed germination and growth of Nicotiana tabacum were done in the botanical garden of Facultad de Estudios Superiores Iztacala, U.N.A.M. Before germination, seeds of Nicotiana tabacum plants received a chemical scarification treatment with a NaClO (4%) and were placed in a sieve with 10 μm mesh light, then washed with sterile distilled water. Later seeds were placed in sterile soil (filtered on a sieve with 3 mm mesh light) and incubated under standard growth conditions at 25°C, 35% relative humidity and with 12 hrs light and dark cycle. After three weeks, the seedlings were transplanted to sterile soil (filtered on a sieve with 1 cm mesh light). Two irrigation groups of five plants each were formed: i) tap water from Alcaldía Miguel Hidalgo, Mexico City, and ii) commercial bottled water for human consumption. Plants grew for 55 days with irrigation every third day with a volume of 10 mL. At the end of growth period, the length and width of leaf and length of the stem were measured. A sample of root, stem and leaf was taken from each plant, and placed in 1.5 mL Eppendorf tubes, flash frozen in liquid nitrogen and stored at −70°C.
Nicotiana tabacum total cell extracts
To prepare cell extracts, 100 mg samples were taken from each root, stem and leaf, and placed in 1.5 mL Eppendorf tubes, 300 μL of 10 mmol/L phosphate buffer (PBS 1X) and 3 μL of 100 mmol/L phenylmethylsulfonil fluoride (PMSF; Sigma-Aldrich; St. Louis Missouri, USA) were added, and samples homogenized with a teflon pistil at 4°C. Then, tubes were centrifuged at 16,000 x g, 5 min at 4°C. Supernatant was transferred to a new Eppendorf tube and incubated at 4°C. Protein concentration of cell extracts was determined by UV spectrophotometry and a standard bovine serum albumin protein curve (BSA; Sigma-Aldrich; Merck KGaA, Darmstadt, Germany).
Reactive oxygen species (ROS) production in Nicotiana tabacum
ROS levels in each of the three plant tissues analyzed (root, stem and leaf) were determined using the method of Citation20, doing three of each one of the five plants for each tissue of each of two experimental groups, i.e., 90 lectures in total. This method is based on reaction of hydrogen peroxide with the compound 2ʹ7’-dichlorodihydrofluorescein diacetate (DCFH2-DA; Sigma-Aldrich; St. Louis Missouri, USA), which produced 2ʹ7’-dichlorofluorescein with fluorescence emission. The reactions were done in a 96-well plate, to each well, 20 μL of cell extract, 225 μL of PBS 1X and 5 μL of DCFH2-DA (500 μmol/L) were added. Emission readings were taken every 20 min for 60 min in a fluorescence spectrophotometer (BioTek) at 485 nm excitation and 520 nm. The maximum value of fluorescence obtained in each time series was used as index of the production of ROS per mg of total protein.
Chlorophyll quantification
Chlorophylls were measured by the method of Citation21. First, 100 mg of leaf was taken from each plant, placed in a 1.5 mL Eppendorf tube, then 100 μL of acetone (80%) was added and the mixture was macerated with a teflon micro pistil. Then the macerated leaf was transferred to a 15 mL Falcon tube and diluted with 2 mL of acetone (80%), stirred in vortex and centrifuged at 500 x g for 10 min. In a quartz cell, 1 mL of extract was added and chlorophylls were determined in a spectrophotometer at 645 and 663 nm. Absorbance data obtained in each wavelength were used to calculate the chlorophyll concentration in mg/mL.
Antioxidant capacity of Nicotiana tabacum
Total antioxidant capacity in N. tabacum leaves was determined by a commercial system (No. 709,001, Cayman Chemical, Ann Arbor Michigan, USA). The system is based on an oxidation reaction, in which the enzyme metmyoglobin and H2O2 produce the oxidation of 2,2′-Azino-bis (3-ethylbenzothiazoline-6-sulfonic acid) diammonium salt (ABTS) and produces a color change, but antioxidants in the cell extracts prevent ABTS oxidation. In this work, instead of using the enzyme metmyoglobin, peroxidase (PeproTech, Rocky Hill New Jersey, USA) was used. Antioxidant capacity of cell extracts was determined by a standard TROLOX curve, this compound is an analogue of tocopherol or vitamin E. Oxidation reactions were carried out in a 96-well plate, 10 μL of cell extract (volume adjusted to protein concentration of 1.5 μg/μL), 10 μL of peroxidase, 40 μL of hydrogen peroxide and ABTS were added to each well. The plate was read in a spectrophotometer at 405 nm. The antioxidant capacity was expressed in equivalents of molar TROLOX concentration.
Water sampling and preparation
Bottled drinking water from a commercial brand was purchased from simple randomly selected retail shops in Alcaldía Miguel Hidalgo of Mexico City (n = 3), and tap water was collected in a home faucet in the same area (n = 3). At the time of purchase of the water bottles, it was verified that the expiration date was at least six months in the future. Protocols to collect, preserve and transport the tap and bottled water samples were performed following procedures described in Citation22. Immediately after collection, all the samples were transported to Laboratorio de Química Análitica of the Instituto de Geofísica, U.N.A.M. (laboratory specialized in water analysis) and kept in refrigeration until its analysis.
Chemical water analysis
Chemical composition of tap and commercial bottled water was determined by triplicate, in the Laboratorio de Química Análitica of the Instituto de Geofísica, U.N.A.M. The analysis included pH determination, conductivity (μs/cm), sodium, potassium, calcium and magnesium cations and carbonate, sulphate, chlorine, boron and nitrate anions (mg/L). This specialized laboratory carries out all chemical analyses according to standard procedures established by the American Public Health Association [Citation23] and described in Citation24.
Quantification of Na+ and K+ in Nicotiana tabacum
Determination of Na+ and K+ was done as in a previous work [Citation19]. A sample of 200 mg from root, stem and leaf of five plants was dehydrated in a drying oven at 80°C for 48 hrs. Then, the samples were weighed and placed in 15 mL glass test tubes and 10 mL of milli Q water was added. The tubes were incubated in a boiling water bath for 1 hour, after cooling to room temperature, they were centrifuged at 1800 x g for 10 min. Supernatant was transferred to a 15 mL falcon tube. Ion determination was made with 1 mL of supernatant in a flame photometer (Zeiss PF5). As a standard, a solution of 1 mmol/L NaCl and 1 mmol/L KCl (Sigma-Aldrich) was used. From readings, concentration of sodium and potassium expressed as mol/L was obtained.
Statistical analysis
The average and standard error of the mean (SEM) of each determination was obtained. Statistical significance of differences between the two groups of irrigation was tested by the Student-t test.
Results
Morphological parameters of N. tabacum with irrigation of tap and drinking bottled water
The average size of the leaves of plants was significantly different (). Leaf length of plants irrigated with commercial bottled water was shorter compared to leaf of plants irrigated with tap water (length 7.1 cm vs. 10.5 cm), also stem length was significantly different (7.8 cm vs. 12.3 cm). The average number of leaves was higher in the plants irrigated with tap water (10.6 vs. 8.0). In addition, chlorosis was observed in the leaves of plants growing with commercial bottled water ().
Table 1. Morphological measures of stem and leaf of plants irrigated with tap and commercial bottled water
Table 2. Basic chemical composition of water used for irrigation of Nicotiana tabacum.
ROS production and antioxidant capacity in cellular extracts of Nicotiana tabacum
To determine whether the chlorosis observed in leaves of plants grown with commercial bottled water correlates with the production of free radicals, oxygen reactive species were measured in all plants. In plants irrigated with commercial bottled water, ROS levels increased over time in all plant structures () this was particularly significant in the stem ()).
Figure 2. ROS fluorescence time series of cellular extracts of root, stem and leaf from plants irrigated with tap and commercial bottled water. Cellular extracts were loaded with 10 µM DHFC-DA and fluorescence (excitation at 485 nm and emission at 520 nm) was recorded every 20 min for 60 min. Differences in fluorescence were clearer at 60 min: a) ROS fluorescence data in cellular extracts of root, b) stem, and c) leaf. Each fluorescence data is the average of three lectures, and it was adjusted to total protein concentration. Each line represents the data of one plant
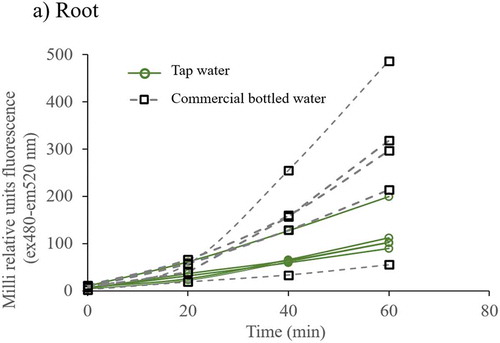
Figure 3. ROS, antioxidant capacity and chlorophylls levels in Nicotiana tabacum. a) Reactive oxygen species in root, stem, and leaf of plants irrigated with tap and commercial bottled water; b) antioxidant capacity in leaf; c) A, B and total chlorophyll in leaves (n = 5 for each group). Error bars are SEM. * P < 0.05
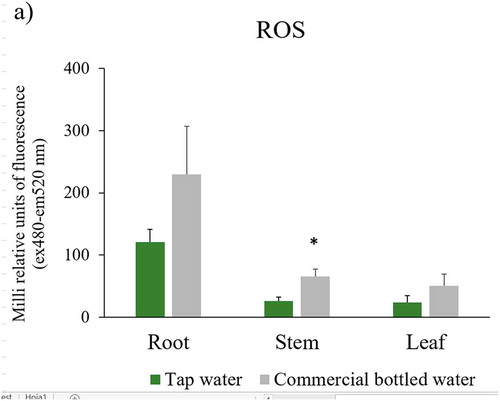
Regarding the antioxidant capacity, leaves of plants irrigated with commercial bottled water exhibited a reduction of antioxidant systems compared to plants irrigated with tap water ()).
Quantification of A, B and total chlorophyll
To corroborate whether leaves of plants showed a change in proportions of chlorophylls, their concentration was measured. Leaves of plants irrigated with commercial bottled water had a lower A, B, and total chlorophyll concentration compared to concentration of plants irrigated with tap water. Differences were statistically significant for A and total chlorophyll ()).
Na+ and K+ soluble ions in Nicotiana tabacum
To explore possible causes of chlorosis, concentration of sodium and potassium ions in root, stem and leaf were quantified. Na+ concentration showed a significant increase in root and leaf of plants with commercial bottled water irrigation ()), while highest concentration of sodium appeared in the stem of plants irrigated with tap water.
Figure 4. Na+ and K+ concentration in Nicotiana tabacum after being irrigated with tap and commercial bottled water. Quantification of a) sodium, and b) potassium in root, stem, and leaf of the plants of each irrigation group: tap water (n = 5) and commercial bottled water (n = 5). Error bars are SEM. ** P < 0.01, *** P < 0.001
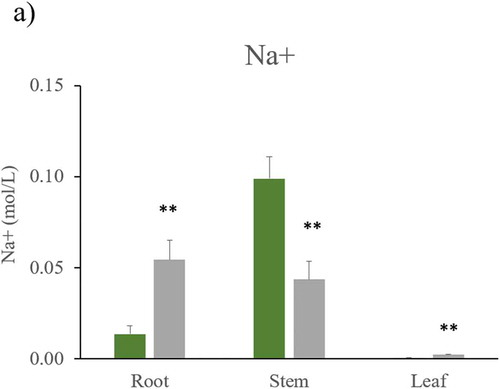
Plants irrigated with commercial bottled water had the highest K+ concentration ()). This difference was significant in root and leaf.
Chemical water analysis
The basic composition of ions in the two kinds of water was determined. shows that ions content in commercial bottled water decreased significantly in comparison with those in tap water. In addition, an acidic pH value (5.5) was observed in the commercial bottled water.
Discussion
In the present work, changes in growth of Nicotiana tabacum were observed after irrigation with tap and commercial bottled water. Plants irrigated with commercial bottled water were significantly smaller and presented yellowing of leaves, known as chlorosis, this condition is caused by lack of chlorophyll. Concentration of chlorophyll in leaves was measured to corroborate its low levels. As expected, in plants irrigated with commercial bottled water, A, B and total chlorophyll content were lower. Chlorophyll content in different leaf stages of tobacco plants was one of the main senescence biomarkers [Citation25]. Therefore, the presence of chlorosis in young leaves of N. tabacum irrigated with commercial bottled water is indicating a serious problem in the physiology of the leaves that leads to their senescence.
It was demonstrated that under diverse stress conditions, such as drought, flooding, chilling, increased salinity, etc., chlorophyll is degraded and this can lead to chlorosis in leaves [Citation26] with subsequent loss of leaves. However, this fact is just the tip of the iceberg, meaning chlorosis is the consequence of stress, then the next step was to investigate the cellular alteration that leads to chlorophyll degradation, considering that in different stress conditions a common factor is the overproduction of reactive oxygen (ROS) and nitrogen (NOS) species [Citation27,Citation28] with the depletion of antioxidant capacity, resulting in damage of biomolecules [Citation29,Citation30]. In this work ROS was measured by reaction with 2ʹ7’-dichlorodihydrofluorescein diacetate (DCFH-DA), regularly used to measure H2O2 [Citation31,Citation32] and the three tissue types analyzed of plants irrigated with commercial bottled water had a high level of ROS. The antioxidant capacity in the cell extracts of leaves of these plants was measured, and a decrease was observed.
In general, the antioxidant capacity is evaluated by the reaction of commercial enzyme metmyoglobin and H2O2 that oxidized ABTS, which is prevented by the antioxidants present in the cell extracts. However, in this work, peroxidase and H2O2 were used, the ABTS oxidation also occurred and green radical ABTS+ was produced. However, if in the cellular extracts catalases enzymes are present, the peroxide is transformed to water and oxygen, decreasing the formation of ABTS+ [Citation33]; therefore, a low absorbance value corresponds to a higher concentration of TROLOX, i.e., higher antioxidant capacity. Measuring the antioxidant capacity using peroxidase enzyme is also indicative of hydrogen peroxide formation, and in leaf extracts of plants irrigated with tap water, a higher concentration of TROLOX was observed, meaning antioxidant enzymes are degrading H2O2. In contrast, the leaf extracts of plants irrigated with commercial bottled water have a lower concentration of TROLOX and higher levels of ROS, this probably corresponds to damage of antioxidant enzymes which degrades superoxide and hydrogen peroxide.
To verify the presence of hydrogen peroxide and catalases in cellular extracts, we propose to carry out subsequent studies to measure such hydrogen peroxide by the radical scavenging activity of an iron chelator, for example caffeic acid [Citation34], which acts as a hydrogen peroxide scavenger by donating hydrogen atoms to reduce the hydrogen peroxide to water. And to determine the presence of catalase enzymes in plants, by measuring the antioxidant capacity with and without an inhibitor of catalase enzymes such as 3-amino-1,2,4 Triazole, which was used in adipocytes to impair the antioxidant defence system by increasing the concentration of H2O2 [Citation35].
Normally, plants have efficient mechanisms to scavenge ROS [Citation36], if antioxidant molecules and enzyme of plants decrease, and reactive oxygen species increase, an imbalance between ROS production and antioxidant capacity occurs, and an oxidative stress condition is established. The overproduction of ROS is potentially dangerous to proteins and lipids in the chloroplast. Therefore, it is considered that irrigation with commercial bottled water leads to a severe oxidative stress condition with an increase of reactive oxygen species linked to chlorosis observed in leaves.
In view of the oxidative stress observed, it was decided to examine the composition of the soil and water. Regarding the quality of the soil, in a previous work we reported ion composition of soil as suitable for the growth of Nicotiana tabacum [Citation19] due to the high content of organic matter (18%), neutral value of pH, adequate concentration of nutrients, such as total nitrogen (0.39%), assimilable phosphorus (2.93 ppm) and exchangeable potassium (294 mol/kg). In relation to chemical composition of tap and bottled water, in this work, bottled water was found to have a deficiency of sodium, potassium, magnesium and sulfate ions and had an acidic pH value (), these water characteristics could lead to two situations of alteration of the physiology of the plant. First, ions are essential nutrients for cellular metabolism and the physiology of organisms, for example in plants, potassium activates about 70 enzymes of metabolism [Citation37], and it is a part of the electrochemical gradient across the membrane, which maintains cell turgor and serves as an energy source to stimulate the transport of sucrose into the phloem. On the contrary, its deficiency in the soil or nutrient media solution reduces plant growth and leads to leaf senescence [Citation38,Citation39]. In this work, it was found that commercial bottled water had a K+ concentration of 0.28 mg/mL which corresponds to 0.007 mM, this is lower than the concentration reported in media with severe K+ deficiency (0.01 mM or 0.03 mM), which is employed to induce leaf senescence [Citation38].
It is clear that low levels of potassium in commercial bottled water can be a key factor in the chlorosis of leaves, as well as the low concentration of potassium observed in the stem of the plants irrigated with commercial bottled water. In the case of magnesium, this cation is a key component of chlorophyll molecules participating in light harvesting. Initial studies reported that Mg+2 deficiency triggers leaf chlorosis; recently a mechanism was proposed, in which ROS is produced via photooxidation, due to an accumulation of carbohydrates and impairment of CO2 fixation, which in turn may accelerate the photosynthetic electron transfer to O2, and potentiates ROS production [Citation40,Citation41]. Secondly, the acidic pH of water could change the characteristics of soil and in consequence change diverse functions in plants. At the beginning of irrigation with commercial bottled water, it is likely that composition of the soil buffers the acidic pH of the water as well as inside the cells, as it has been demonstrated that the cytoplasm pH of plants in normal condition is 7.5, i.e., slightly alkaline [Citation42,Citation43]. However, after prolonged irrigation with acidic pH water, it is very likely that soil pH balance is altered with effects in plant physiology. It has already been proved that short exposure to acidic pH of soil or growth medium can modify root water permeability, for example, the activity of aquaporin proteins specialized in root water transport can be reduced and consequently the nutrient and water transport from root to leaf is modified [Citation44,Citation45]. Instead, prolonged exposure to acidic pH can reduce plant growth, and this may be the case of plants irrigated with commercial bottled water. Both mineral deficiency and acid pH of water could be the cause of increased ROS production, reduction of chlorophylls and consequently smaller plant size observed in the plants irrigated with commercial bottled water.
In further studies, we propose to examine at different stages of plant growth the concentration of oxidative stress markers such as malondialdehyde and 3-Nitrotyrosine, which result from lipid peroxidation and tyrosine oxidation. To examine the accumulation of compounds derived from hydrocarbons, the ROS formation by dithiothreitol assay would be employed [Citation46]. In addition, the expression of NADP-malic oxidoreductase could be quantified as sensor of changes of soil pH.
The commercial bottled water analyzed in this work did not comply with the Mexican regulation NOM-127-SSA1-2000, which establishes a standard water pH value of 6.5–8.5. It is considered that if the adverse effects observed on the physiology of plants irrigated with commercial bottled water are extrapolated to human continuous consumption of water, it may have serious health implications. The first clearest effect would be the alteration of acid-base homeostasis, which could lead to low-grade metabolic acidosis, characterized by decreased blood pH (<7.35). This has been associated with the type of diet, due to the acid load it produces. This condition has been associated with an increased risk of development of cardiovascular diseases, insulin resistance, high blood pressure, and changes in bone mineral density [Citation47,Citation48]. Because the production of ROS is a common factor in all organisms with aerobic metabolism, we consider the ROS overproduction observed in plants irrigated with commercial bottled water as an important element that must be extrapolated to the effects on human health. In normal condition, ROS has a beneficial effect, it functions as secondary messenger in diverse cellular process, for example in plants it has an effect on the closing of stoma [Citation49,Citation50]. On the contrary, an excess of ROS induces oxidative damages under several environmental stress conditions. In humans, the overproduction of ROS is a key element in the theory of aging, even more so it is a marker of the pathology and progression of different chronic and degenerative diseases, such as diabetes, chronic obstructive pulmonary disease, osteoarthritis and some kinds of cancer [Citation51–54]. Therefore, it could be hypothesized that a prolonged intake of acidic water results in increased ROS production with deleterious effects on human health.
Conclusions
In this work we find that irrigation of Nicotiana tabacum with commercial bottled water leads to reduction of plant size and chlorosis of leaves. The study plants had a significant increase of reactive oxygen species and a decrease of A, B and total chlorophylls, and their leaves had a lower antioxidant capacity. Taken together, these factors could cause the altered physiology observed in the plants irrigated with commercial bottled water. Extrapolating these effects to human health, such as the loss of acid-base balance and the overproduction of ROS, drinking bottled water could accelerate the development, pathology and progression of several chronic diseases.
Highlights
Irrigation with bottled water for human consumption reduces the growth and chlorophylls concentration and increases ROS in Nicotiana tabacum.
Acidic pH value of bottled water is key to understanding the observed phenotype of N. tabacum irrigated with bottled water.
Extrapolating the effects of acidic pH of bottled water observed in plants, it could alter blood acid-base homeostasis in humans.
Acknowledgments
The authors thank to: Biologist Marcial García Pineda for facilitating the germination and growth of the plants in the botanical garden greenhouse of the Facultad de Estudios Superiores Iztacala, U.N.A.M.; PhD Yadira Ledesma Soto of UBIMED for her technical assistance, both from the Facultad de Estudios Superiores Iztacala, U.N.A.M.; Art Designer Daniel Díaz Íñiguez for his collaboration in graphic design of the graphical abstract; and to two anonymous reviewers whose comments helped to improve this manuscript.
Disclosure statement
The authors declare no competing interests.
Data availability statement
The data that support the findings of this study are available from the corresponding author upon reasonable request.
- Espinosa-García AC, Díaz-Ávalos C, González-Villarreal FJ, et al. Drinking water quality in a Mexico City university community: perception and preferences. Ecohealth. 2015;12(1):88–97.
- Regnier A, Gurian P, Mena KD. Drinking water intake and source patterns within a US-Mexico border population. Int J Environ Health Res. 2015;25(1):21–32.
- Travis PB, Goodman KJ, O'RourkeKM, et al. The association of drinking water quality and sewage disposal with Helicobacter pylori incidence in infants: the potential role of water-borne transmission. J Water Health. 2010;8(1):192–203.
- Cerna-Cortes JF, Cortes-Cueto AL, Villegas-Martínez D, et al. Bacteriological quality of bottled water obtained from Mexico City small water purification plants: incidence and identification of potentially pathogenic nontuberculous mycobacteria species. Int J Food Microbiol. 2019;306:108260.
- Chidya RCG, Singano L, Chitedze I, et al. Standards compliance and health implications of bottled water in Malawi. Int J Environ Res Public Health. 2019;16(6):951.
- Dávila Rangel JI, López del Rio H, Mireles García F, et al. Radioactivity in bottled waters sold in Mexico. Appl Radiat Isot. 2002;56(6):931–936.
- Díaz G, Ortiz R, Schettino B, et al. Organochlorine pesticides residues in bottled drinking water from Mexico City. Bull Environ Contam Toxicol. 2009;82(6):701–704.
- Cidu R, Frau F, Tore P. Drinking water quality: comparing inorganic components in bottled water and Italian tap water. J Food Compost Anal. 2011;24(2):184–193.
- Padhye LP, Kim J-H, Huang C-H. Oxidation of dithiocarbamates to yield N-nitrosamines by water disinfection oxidants. Water Res. 2013;47(2):725–736.
- Kang JH, Kondo F, Katayama Y. Human exposure to bisphenol A. Toxicology. 2006;226(2–3):79–89.
- Li X, Ying G-G,Su H-C, et al. Simultaneous determination and assessment of 4-nonylphenol, bisphenol A and triclosan in tap water, bottled water and baby bottles. Environ Int. 2010;36(6):557–562.
- Amraoui W, Adjabi N, Bououza F, et al. Modulatory role of selenium, and vitamin E, natural antioxidants, against bisphenol A-induced oxidative stress in wistar albinos rats. Toxicol Res. 2018;34(3):231–239.
- Chapa-Martínez CA, Hinojosa-Reyes L, Hernández-Ramírez A, et al. An evaluation of the migration of antimony from polyethylene terephthalate (PET) plastic used for bottled drinking water. Sci Total Environ. 2016;565:511–518.
- Salazar-Beltrán D, Hinojosa-Reyes L, Ruiz-Ruiz E, et al. Determination of phthalates in bottled water by automated on-line solid phase extraction coupled to liquid chromatography with UV detection. Talanta. 2017;168:291–297.
- Houdebine LM. Use of transgenic animals to improve human health and animal production. Reproduct Domest Anim. 2005;40(4):269–281.
- Kovalchuk I, Kovalchuk O. Transgenic plants as sensors of environmental pollution genotoxicity. Sensors (Basel). 2008;8(3):1539–1558.
- Müller GL, Drincovich MF, Andreo CS, et al. Nicotiana tabacum NADP-malic enzyme: cloning, characterization and analysis of biological role. Plant Cell Physiol. 2008;49(3):469–480.
- Doubnerová Hýsková V, Miedzińska L, Dobrá J, et al. Phosphoenolpyruvate carboxylase, NADP-malic enzyme, and pyruvate, phosphate dikinase are involved in the acclimation of Nicotiana tabacum L. to drought stress. J Plant Physiol. 2014;171(5):19–25.
- Calderón-Torres M, López-Estrada E, Romero-Maldonado A, et al. The heterologous expression of the Debaryomyces hansenii DhARO4 gene in Nicotiana tabacum improves growth yield, even after inhibition by saline stress. Revista Mexicana de Ingeniería Química. 2019;18(2):729–736.
- Hempel SL, Buettner GR, O’Malley YQ, et al. Dihydrofluorescein diacetate is superior for detecting intracellular oxidants: comparison with 2ʹ,7ʹ-dichlorodihydrofluorescein diacetate, 5(and 6) ‐carboxy‐2ʹ,7ʹ‐dichlorodihydro fluorescein diacetate, and dihydrorhodamine 123. Free Radic Biol Med. 1999;27(1–2):146–159.
- Porra RJ, Thompson WA, Kriedemann PE. Determination of accurate extinction coefficients and simultaneous equations for assaying chlorophylls a and b extracted with four different solvents: verification of the concentration of chlorophyll standards by atomic absorption spectroscopy. Biochimica et Biophysica Acta. 1989;975(3):384–394.
- Sigala I, Caballero M, Correa-Metrio A, et al. Basic limnology of 30 continental waterbodies of the Transmexican volcanic belt across climatic and environmental gradients. Boletín de la Sociedad Geológica Mexicana. 2017;69(2):313–370.
- APHA (American Public Health Association) AWWA (American Water Works Association), and WPCF (Water Pollution Control Federation). Standard methods for the examination of water and wastewater. Maryland: American Public Health Association; 2005.
- Armienta MA, Vilaclara G, De la Cruz- Reyna S, et al. Water chemistry of lakes related to active and inactive Mexican volcanoes. J Volcanol Geotherm Res. 2008;178(2):249–258.
- Tercé‐Laforgue T, Mäck G, Hirel B. New insights towards the function of glutamate dehydrogenase revealed during source‐sink transition of tobacco (Nicotiana tabacum) plants grown under different nitrogen regimes. Physiol Plant. 2004;120(2):220–228.
- Vergeiner C, Banala S, Kräutler B. Chlorophyll breakdown in senescent banana leaves: catabolism reprogrammed for biosynthesis of persistent blue fluorescent tetrapyrroles. Chem. 2013;19(37):12294–12305.
- Muneer S, Kim TH, Choi BC, et al. Effect of CO, NOx and SO2 on ROS production, photosynthesis and ascorbate-glutathione pathway to induce Fragarixannasa as a hyperaccumulator. Redox Biol. 2014;2:91–98.
- Xie X, He Z, Chen N, et al. The roles of environmental factors in regulation of oxidative stress in plant. Biomed Res Int. 2019;9732325.
- Kim Y, Mun B-G, Khan AL, et al. Regulation of reactive oxygen and nitrogen species by salicylic acid in rice plants under salinity stress conditions. PloS ONE. 2018;13(3):e0192650.
- Zhou R, Yu X, Zhao T, et al. Physiological analysis and transcriptome sequencing reveal the effects of combined cold and drought on tomato leaf. BMC Plant Biol. 2019;19(1):377.
- Takanashi T, Ogura Y, Taguchi H, et al. Fluorophotometric quantitation of oxidative stress in the retina in vivo. Invest Opthalmol Vis Sci. 1997;38(13):2721–2728.
- Griendling KK, Touyz RM, Zweier JL, et al. Measurement of reactive oxygen species, reactive nitrogen species, and redox-dependent signaling in the cardiovascular system. A scientific statement from the American heart association. Circ Res. 2016;119(5):e39–e75.
- Nguyen LT, Yang K-L. Combined cross-linked enzyme aggregates of horseradish peroxidase and glucose oxidase for catalyzing cascade chemical reactions. Enzyme Microb Technol. 2017;100:52–59.
- Adjimani JP, Asare P. Antioxidant and free radical scavenging activity of iron chelators. Toxicol Rep. 2015;2:721–728.
- Ruiz-Ojeda FJ, Gomez-Llorente C, María Aguilera C, et al. Impact of 3-amino-1,2,4-Triazole (3-AT)-derived increase in hydrogen peroxide levels on inflammation and metabolism in human differentiated adipocytes. PloS ONE. 2016;11(3):e0152550.
- Latowski D, Kuczyńska P, Strzałka K. Xanthophyll cycle – a mechanism protecting plants against oxidative stress. Redox Rep. 2011;16(2):78–90.
- Shabala S. Signalling by potassium: another second messenger to add to the list? J Exp Bot. 2017;68(15):4003–4007.
- Wang Y, Li B, Du M, et al. Mechanism of phytohormone involvement in feedback regulation of cotton leaf senescence induced by potassium deficiency. J Exp Bot. 2012;63(16):5887–5901.
- Hu W, Di Q, Wang Z, et al. Grafting alleviates potassium stress and improves growth in tobacco. BMC Plant Biol. 2019;19(1):130.
- Cakmak I, Kirkby EA. Role of magnesium in carbon partitioning and alleviating photooxidative damage. Physiol Plant. 2008;133(4):692–704.
- Peng YY, Liao LL, Liu S, et al. Magnesium deficiency triggers SGR-mediated chlorophyll degradation for magnesium remobilization. Plant Physiol. 2019;181(1):262–275.
- Mimura T, Shindo C, Kato M, et al. Regulation of cytoplasmic pH under extreme acid conditions in suspension cultured cells of Catharanthus roseus: a possible role of inorganic phosphate. Plant Cell Physiology. 2000;41(4):424–431.
- Shen J, Zeng Y, Zhuang X, et al. Organelle pH in the Arabidopsis endomembrane system. Mol Plant. 2013;6(5):1419–1437.
- Javot H, Maurel C. The role of aquaporins in root water uptake. Ann Bot. 2002;90(3):301–313.
- Kapilan R, Vaziri M, Zwiazek JJ. Regulation of aquaporins in plants under stress. Biol Res. 2018;51(1):4.
- Li N, Sioutas C, Cho A, et al. Ultrafine particulate pollutants induce oxidative stress and mitochondrial damage. Environ Health Perspect. 2003;111(4):455–460.
- Rebholz CM, Surapaneni A, Levey AS, et al. The serum metabolome identifies biomarkers of dietary acid load in 2 studies of adults with chronic kidney disease. J Nutr. 2019;149(4):578–585.
- Osuna-Padilla IA, Leal-Escobar G, Garza-García CA, et al. Carga ácida de la dieta; mecanismos y evidencia de sus repercusiones en la salud. Nefrología. 2019;39(4):343–354.
- Leshem Y, Levine A. Zooming into sub-organellar localization of reactive oxygen species in guard cell chloroplasts during abscisic acid and methyl jasmonate treatments. Plant Signal Behav. 2013;8(10):10.4161/psb.25689.
- Li J, Li Y, Yin Z, et al. OsASR5 enhances drought tolerance through a stomatal closure pathway associated with ABA and H2O2 signalling in rice. Plant Biotechnol J. 2017;15(2):183–196.
- Jiang QW, Kaili D, Freeman J, et al. Diabetes inhibits corneal epithelial cell migration and tight junction formation in mice and human via increasing ROS and impairing Akt signaling. Acta Pharmacol Sin. 2019;40(9):1205–1211.
- Marushchak M, Maksiv K, Krynytska I, et al. The severity of oxidative stress in comorbid chronic obstructive pulmonary disease (COPD) and hypertension: does it depend on ACE and AGT gene polymorphisms? J Med Life. 2019;12(4):426–434.
- Arra M, Swarnkar G, Ke K, et al. LDHA-mediated ROS generation in chondrocytes is a potential therapeutic target for osteoarthritis. Nat Commun. 2020;11(1):3427.
- Grasso D, Zampieri LX, Capelôa T, et al. Mitochondria in cancer. Cell Stress. 2020;4(6):114–146.