ABSTRACT
The coprecipitation method was used to produce magnetic cotton straw biochar (MCS). Elemental analysis, FTIR, XRD, SEM, BET and magnetization curves were used to characterize MCS and CS. The results show that the MCS had excellent magnetic separation properties. When the pH value was higher than 5, the pH of the aniline solution had little effect on the adsorption capacity of MCS and CS. However, with increasing temperature, the adsorption capacity increased significantly. The maximum adsorption capacities of MCS and CS for aniline were 38.67 mg/g and 42.57 mg/g, respectively. The adsorption process of aniline was spontaneous, endothermic, and involved monolayer sorption and chemical adsorption. The adsorption capacity was due to the presence in magnetic biochar of phenolic hydroxyls and H-bond donors that adsorbed aniline. Therefore, the application of MCS presents good prospects for the removal of toxic and refractory aniline from water.
1. Introduction
China produced 432.8 million tons of coke in 2018 [Citation1]. Coking wastewater contains many organic compounds that are difficult to biodegrade, including phenols, anilines, quinolines and inorganic ammonia, and is one of the largest sources of industrial wastewater in China [Citation2]. After the coking sewage is treated, the main toxic components in the coking sewage are pollutants with low concentrations but high toxicity, such as benzenes, polycyclic aromatic hydrocarbons, chloramine and aniline [Citation3]. Aniline causes methemoglobinemia, damages the liver and kidneys and is easily absorbed by human skin. Its toxicity is classified as highly toxic [Citation4]. The National First-degree Wastewater Discharge Standard of aniline according to the National Standard of the People’s Republic of China for integrated wastewater discharge standard (GB 8978–1996) is 1.0 mg/L, so it is necessary to discharge sewage after remove aniline from water.
The current methods for removing aniline from water include electrochemical technology [Citation5], nanofiltration [Citation6], reverse osmosis [Citation7] and adsorption [Citation8]. Electrochemical technology has the advantages of a small reactor size, high removal rate and ease of handling, but other toxic substances that are difficult to degrade are produced during the reaction [Citation9]. Nanofiltration and reverse osmosis can lead to membrane fouling, which can decrease the filtration flux and affect the treatment effect. The adsorption operation is simple, low cost and will not produce toxic substances; therefore, it is worth further studying aniline removal from water by adsorption.
Adsorption materials include fiber [Citation8], activated carbon [Citation10] and biochar [Citation11]. In recent years, biochar research has developed rapidly. Biochar mostly uses agricultural wastes as raw materials, such as rice straw [Citation12], bamboo [Citation13], fir [Citation14], and reed [Citation15]. It is prepared by pyrolysis under oxygen-limited conditions. Biochar can effectively remove organic pollutants from wastewater [Citation16,Citation17]. China is a traditional agricultural country with abundant agricultural waste resources. However, most straw is discarded. For example, more than 40 million tons of cotton stalks are produced every year [Citation18] in China, but most of the cotton stalks are burned as waste or discarded. Therefore, the discarded cotton stalks can be used as a raw material to produce biochar. Cotton stalk biochar has a low cost and can be used to protect the water environment when used for adsorbing pollutants and can further protect the environment. However, in adsorption methods, some adsorbents have a small adsorbing capacity or are difficult to separate from the solution. Some adsorbents have poor adsorption stability and may be desorbed, resulting in secondary pollution and other problems.
Compared with traditional biochar, the magnetic biochar (MBC) adsorbent is easily recovered from the reaction system under the action of a low-intensity external magnetic field. Additionally, magnetic separation requires less energy and operating costs than conventional filtration, centrifugation, or gravity separation [Citation19]. In the past decade, three modification methods were developed by previous researchers to synthesize magnetic biochar, including direct pyrolysis of biomass/metal salt precursors, liquid-phase coprecipitation of iron salt/ferrite, and reduction of ferrite by borohydride [Citation20]. Magnetic biochar has been used to adsorb organic compounds such as crystal violet [Citation21], tetracycline [Citation22], sulfamethoxazole [Citation23], and aniline [Citation24]. The magnetic biochar-adsorbed aniline was made from Douglas fir fired at 900 °C, impregnated with FeCl3 and pyrolyzed at 600 °C. Despite its high adsorption capacity (when the initial concentration of aniline was 50 mg/L, 0.05 g of biochar was added to a 25 mL aniline solution, and more than 90% of aniline was removed in the first 5 min), it is complicated and expensive to make. Therefore, it is necessary to prepare magnetic biochar for the adsorption of aniline that is easy to make and inexpensive.
In this study, (1) magnetic biochar derived from cotton stalks is prepared by loading Fe3O4 onto the surface of pristine biochar. (2) The removal effect of aniline from solution by the magnetic biochar was studied, and the separation properties of magnetic biochar were also examined. (3) The factors affecting the adsorption of aniline by magnetic biochar were analyzed, and the adsorption mechanism was discussed.
2. Materials and methods
2.1 Biochar and magnetic biochar production
Cotton stalks were obtained from a local farm (Wuhan, China). Three parallel experiments were conducted for all the tests, and the error of the parallel experiments was controlled within 5%. The cotton stalks were pyrolyzed at 300 °C, 450 °C, and 600 °C for 3 h under oxygen-restricted conditions [Citation25] and then sieved to 100 meshes or less to prepare the biochar CS (cotton stalk). One gram of CS was added to a 200 ml solution containing 0.5 g of FeCl3 · 6H2O and 1 g of FeSO4 · 7H2O (the molar ratio was 1:2) [Citation26]. The pH of the solution was adjusted to 11 and then filtered [Citation27]. The solid was dried overnight in an oven at 60 °C [Citation28], and sieved to 100 meshes or less to prepare the magnetic cotton stalk biochar (MCS).
2.2 Characterization
Particle distribution was measured by a laser particle analyzer (Bettersize2000E, Better, China). A zeta potential analyzer was used to measure the surface charge (Zetasizer Nano ZS, Malvern, UK). The element analysis was measured by an element analyzer (Vario EL cube, Elementar, Germany), and the content of iron was measured with an ICP-OES (Optima 8300, PerkinElmer, USA). The surface area was determined according to the N2 adsorption–desorption curve (TriStar II 3020, Micromeritics, USA). The surface morphology of the biochar was examined with a scanning electron microscope (SEM, JEM-6700 F, Hitachi Limited, Japan). The changes in functional groups on the surface of the biochar were measured by Fourier transform infrared spectroscopy (FTIR, NICOLET 5700, Thermo Electron Corporation, USA). The crystalline minerals in the biochar samples were investigated by X-ray diffraction (XRD, PANalytical, X’Pert Pro, Netherlands). The magnetic properties of the magnetic biochar were characterized by a magnetic hysteresis loop drawn by a vibration sample magnetometer (PPMS-9 T, Quantum Design, USA).
2.3 Batch adsorption
Batch adsorption was carried out in a mechanical shaker at 25 °C and 170 rpm. Biochar (0.1 g) was added to 50 ml of aniline solution (50 mg/L) and shaken for 24 h. The mixture was filtered through a 0.22 μm filter [Citation25]. The concentration of aniline in the filtered liquid was quantified using high-performance liquid chromatography (HPLC, Agilent 1100, USA) with a C18 column (150 mm*4.6 mm*5 μm). A mixture of methanol (25%), 0.2% NH4AC and 0.1% NH3·H2O buffer solution (75%) was used as the mobile phase with a flow rate of 1 mL·min−1 [Citation29].
Adsorption kinetics were determined by adding 0.1 g of biochar into 50 ml of aniline (50 mg/L) and then shaking the solution in a mechanical shaker (170 rpm) at 25 °C for 0.5, 1, 2, 5, 10, 15, 20, 24 and 48 h. Isotherms were determined by adding 0.1 g of biochar into 50 ml of aniline (30 ~ 600 mg/L) and shaking for 24 h. The remaining steps were the same as the adsorption experiments.
The influence of environmental factors was studied (). The shaking speeds were all 170 rpm with a reaction time of 24 h and initial aniline concentrations of 50 mg/L. The pH was adjusted by NaOH or HCl solutions (0.01 mmol/L, 1 mmol/L, and 100 mmol/L).
Table 1. Experiment of influencing factors
The MCS which reached the adsorption equilibrium was separated from the solution system by magnet and the concentration of aniline in the solution was measured. The isolated MCS was desorpted with 20 mL of HCl (0.1 mol/L) for 2 h, then the MCS was washed with deionized water to neutral and dried at 70 °C. The treated MCS was used for the aniline adsorption test, the experimental conditions was the same as the adsorption kinetics for 24 h. The adsorption-desorption process was repeated for 5 times to study the recycling performance of the MCS.
Data processing and mathematical models were applied in this study (supplemental material).
3. Results and discussion
3.1 Characteristics of MCS and CS
The elemental composition of MCS and CS is shown in . After modification, the content of Fe increased from 0.052% to 18.82%, indicating that the magnetic biochar was loaded with a large amount of iron. The XRD patterns () and ()) of MCS and CS show that the intensity and crystal structure of XRD diffraction peaks changed significantly. There were main peaks in the MCS diffraction pattern (2θ = 35.71°, 43.41°, 57.39°, and 62.6°). These peaks correspond to the four indexed planes (311), (400), (511), and (440) of maghemite. They are characteristic peaks of Fe3O4 (magnetite) [Citation30], indicating that the main crystalline phase in MCS is magnetite and that Fe3O4 was successfully loaded onto MCS [Citation31].
Table 2. Physicochemical characteristics of MCS and CS
The FTIR spectra of MCS and CS before and after aniline adsorption are shown in . It can be seen that MCS and CS have similar peak shapes at most wave numbers. MCS and CS had characteristic peaks of aniline at 3355 cm−1 and 3430 cm−1 after the adsorption of aniline. MCS shows a distinct characteristic Fe-O stretching vibration peak at approximately 587 cm−1 before and after adsorption [Citation32] but not on the surface of CS. The characteristic Fe-O stretching vibration peak was due to the Fe-O bond in Fe3O4 [Citation33]. This indicates that Fe3O4 was successfully loaded onto MCS. The FTIR results were consistent with the XRD and elemental analysis results, indicating that MCS was magnetized.
The hysteresis curve of MCS is shown in ). It can be seen from the hysteresis curve that the saturation magnetization intensity of the MCS sample is 15.03 emu/g, which is superparamagnetic at room temperature, without coercive force and remanence. The MCS particles can be easily separated from the solution by using permanent magnets, as shown in ). The above analysis shows that the prepared MCS has good magnetic properties [Citation15].
Figure 3. (a) Magnetization curve of MCS at room temperature. (b) The photo of the MCS adsorbed on the magnet
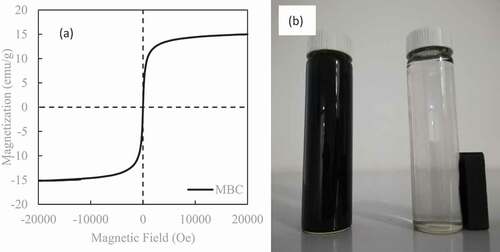
shows the SEM spectrum before and after CS magnetization. It can be seen from that the CS surface is relatively smooth, and it has some pore structure, but the pore structure is underdeveloped. The MCS and CS are quite different in terms of surface micromorphology. The Fe3O4 particles exhibit a spherical-like shape with a uniform particle-size distribution ranging from 60 to 100 nm [Citation34]. The surface of MCS contained small spherical particles, which indicates that Fe3O4 is supported on the surface of CS. MCS had a higher BET surface area (118 m2/g) than CS (). This may be due to the additional surface area provided by Fe3O4 fixed on the surface of the biochar. The pore volume and the average pore size of the two biochars were similar. Therefore, the adsorption effect of MCS and CS on aniline shows little difference.
Table 3. The specific surface area and pore structure of different biochars
3.2 Adsorption performance
Biochar yield is 40.06% at 300 °C, 33.7% at 450 °C and 24.8% at 600 °C. As the pyrolysis temperature increased, the adsorption ability of MCS and CS increased significantly as shown in , but the biochar yield decline rapidly. This is due to the devolatilization of fiber in the cotton stalk at the time of heating, with the increase in temperature the volatiles increases. Previous studies have shown that with the increase in firing temperature, the BET surface area of biochar increases, so the adsorption ability of MCS and CS increased [Citation35]. However, when the temperature is greater than 800 °C, the specific surface area decreases, which may be related to shrinkage, and the narrowing of micropores leads to pore closure. Considering the yield problem, 600 °C was selected as the optimal pyrolysis temperature [Citation35].
shows the kinetics of aniline adsorption. The kinetics of MCS and CS on aniline adsorption were similar when the initial concentration of aniline was 50 mg/L. Aniline is quickly adsorbed by the adsorbent in the first 5 h. With time, the adsorption capacity of MCS and CS on aniline was gradually balanced. The adsorption capacity of CS for aniline is greater than that of MCS after 48 hours of adsorption.
Figure 6. Adsorption kinetics of aniline adsorption on (a) MCS and (b) CS, adsorption isotherm curves for aniline adsorption on (c) MCS and (d) CS
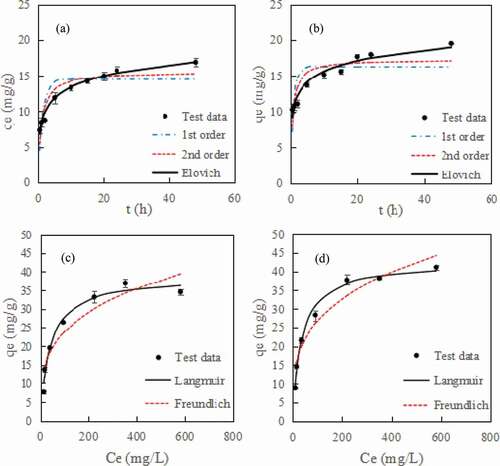
According to , the correlation coefficient (R2) obtained by fitting the Elovich kinetic equation is greater than 0.953. Therefore, the Elovich kinetic equation described well the kinetic process of aniline adsorption. The Elovich kinetic model mainly describes the adsorption process of adsorbate on the surface of heterogeneous materials. The equation is an empirical equation describing a process involving a series of reaction mechanisms, such as solute diffusion in the solution phase or at the interface, surface activation and deactivation [Citation36].
Table 4. Kinetic parameters for aniline adsorption on MCS and CS
The adsorption isotherm curves for aniline adsorption on MCS and CS shown in ). The most suitable model was the Langmuir model, with correlation coefficients of 0.976 and 0.988, and the Freundlich correlation coefficients were 0.885 and 0.936 (). The maximum adsorption capacity of MCS for aniline was 38.672 mg/g and that of CS for aniline was 42.570 mg/g. The Langmuir isotherm model based on a high correlation coefficient shows that aniline removal was monolayer sorption shows that MCS and CS have a higher specific surface area; therefore, they have a considerable adsorption capacity for aniline [Citation37]. The comparison on the adsorption capacity for aniline of the developed MCS with the state of the art shows in . The adsorption capacity of MCS was not as high as other adsorbents, but the production method of MCS was simple, and MCS can be easily separated from the solution after use. Magnetic biochar can be recovered more efficiently, faster and with less energy consumption than other adsorbents because it does not require more time-consuming filtration or centrifugation, and filtration methods can lead to screen clogging or adsorbent loss.
Table 5. Isotherm parameters for aniline adsorption on MCS and CS
Table 6. Comparison of aniline adsorption capacities on MCS versus other adsorbents
3.3 Effects of pH, adsorption temperature and dosage of adsorbent
As shown in , when the pH value was less than 5, the adsorption capacity of MCS and CS increased gradually with decreasing pH value. When the pH value was greater than 5, the pH of the aniline solution did not substantially affect the adsorption capacity of MCS and CS. This is because the pKa value of aniline is 4.6; aniline exists mainly as cationic forms when the pH value is below 4.6 and mainly exists in a neutral form when the pH value is 5–11 [Citation38]. The zeta potentials of MCS and CS were −23.6 mV and −43.5 mV, respectively. When the pH value was less than 4.6, aniline in the form of cations increased with decreasing pH and was more easily adsorbed by the biochar. The zeta potential of CS was lower, and the adsorption capacity of CS was thus larger than that of MCS. Strong acidic media can affect the stability of Fe3O4. This will free up more sites for aniline adsorption [Citation39]. As can be seen from the , the ability of MCS to adsorb aniline increases rapidly when the pH value was less than 3.
Aniline can be combined with hydrogen (H) on the surface. Biochar contains phenolic hydroxyls and has the functions of ketone and aryl oxide. The surface sites of phenols are H-bond donors of aniline. The aniline nitrogen lone pair is a hydrogen bond acceptor that interacts through π-π electron-donor-acceptor [Citation24] ().
Figure 8. The reaction equations of adsorption dominated by hydrogen bond formed between aniline molecule and carboxylic or phenolic group
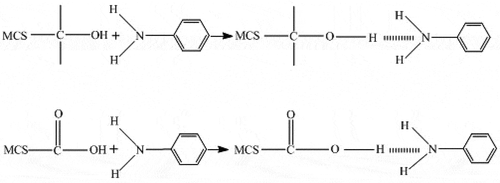
As shown in , when the initial concentration of aniline was 50 mg/L, the dose of MCS was 4 g/L, and the removal rate of aniline reached 78%. When the dosages of MCS and CS were greater than 10 g/L, aniline was completely absorbed, and the removal rate of aniline reached 100%. However, from economic and environmental viewpoints, 4 g/L was selected herein as the optimum BC loading.
As shown in , temperature greatly affects aniline adsorption. With the increase in temperature from 15 °C to 45 °C, the adsorption capacity of CS increased from 13.11 mg/g to 19.57 mg/g and that of MCS increased from 10.75 mg/g to 16.77 mg/g. Adsorption of aniline is an endothermic process [Citation40].
As shown in , the negative ΔG° values (−14.023 to −18.875 KJ/mol) indicate that the adsorption of aniline on the biochar is spontaneous [Citation41]. The positive values of ΔH° (2.508–3.130 kJ/mol) indicate that all adsorption processes of aniline are endothermic. Furthermore, the positive value of ΔS° (0.015–0.017 kJ/mol) reveals an increase in the randomness of aniline at the solid–liquid interface [Citation42]. A higher temperature can improve the solubility of aniline and the active point on the biochar surface. The increase in temperature is favorable for the adsorption of aniline.
Table 7. Thermodynamic parameters for the adsorption of aniline adsorption on MCS and CS
3.4 Recycling performance of MCS
The cecycling performance of MCS after aniline adsorption shown in . The adsorption capacity of MCS for aniline after regeneration decline slightly, but it still maintains 80.2% of the initial adsorption capacity after five adsorption-desorption cycles. Therefore, MCS is easy to reuse and can reduce the cost. With the increase in the times of adsorption-desorption cycles, the decrease in the specific surface area, total pore volume and the number of surface functional groups of MCS cause the decrease in the adsorption sites.
4. Conclusion
In this paper, the coprecipitation method was used to prepare magnetic biochar (MCS) as an adsorbent to remove aniline from water. The prepared MCS showed high magnetic strength, and its saturation magnetic susceptibility was 15.03 emu/g. The adsorption process of aniline was spontaneous, endothermic, and involved monolayer sorption and chemical adsorption. The magnetic biochar showed a good adsorption effect in solutions with different pH values. When the initial concentration of aniline solution was 50 mg/L and the dosage of MCS was 4 g/L, the removal rate of aniline was 78%. The maximum adsorption capacity of MCS and CS for aniline was 38.672 mg/g and 42.570 mg/g. But MCS can be easily separated from the solution after use. Magnetic biochar can be recovered more efficiently, faster and with less energy consumption than biochar because it does not require more time-consuming filtration or centrifugation, and filtration methods can lead to screen clogging or adsorbent loss. Therefore, MCS presents better application prospects for removing toxic and refractory aniline from water.
Acknowledgments
This work was partially supported by the Knowledge Innovation Project (Natural Science Foundation) of Hubei Province (No. 2019CFB458), Engineering Research Center of Urban Disasters Prevention and Fire Rescue Technology of Hubei Province and the Wuhan Water Engineering & Technology Co. Ltd.
Disclosure statement
No potential conflict of interest was reported by the author(s).
Correction Statement
This article has been republished with minor changes. These changes do not impact the academic content of the article.
Additional information
Funding
References
- Cui P. Analysis of coke industry economic operation and coke market development tendency in China.China coal. 2019;045:5–9.
- Qing Wei QS. Study on the treatment of simulated coking wastewater by O3 and O3/Fenton processes in a rotating packed bed.Chem React Eng Techno. 2015;5:93386–93393.
- Kaiyuan H, Chaohai W. Correlation analysis of coking wastewater pollution index. Environ Chem. 2015;34:1661–1670.
- Orge CA, Faria JL, Pereira MFR. Photocatalytic ozonation of aniline with TiO2-carbon composite materials. J Environ Manage. 2017;195:208–215.
- Xiaoliang L, Hao X. Electrochemical oxidation of aniline by a novel Ti/TiOxHy/Sb-SnO2 electrode. Chin J Catal. 2016;37(11):1860–1870.
- Hidalgo AM, Leon G, Gomez M, et al. Polyamide nanofiltration membranes to remove aniline in aqueous solutions. Environ Technol. 2014;35(9):1175–1181.
- Gómez JL, León G, Hidalgo AM, et al. Application of reverse osmosis to remove aniline from wastewater. Desalination. 2009;245(1–3):687–693.
- Satilmis B, Uyar T. Removal of aniline from air and water by polymers of intrinsic microporosity (PIM-1) electrospun ultrafine fibers. J Colloid Interface Sci. 2018;516:317–324.
- Karthikeyan S, Viswanathan K, Boopathy R, et al. Three dimensional electro catalytic oxidation of aniline by boron doped mesoporous activated carbon. J Ind Eng Chem. 2015;21:942–950.
- Itoi H, Maki S, Ninomiya T, et al. Electrochemical polymerization of pyrene and aniline exclusively inside the pores of activated carbon for high-performance asymmetric electrochemical capacitors. Nanoscale. 2018;10(20):9760–9772.
- Braida WJ, Pignatello JJ, Lu Y, et al. Sorption hysteresis of benzene in charcoal particles. Environ Sci Technol. 2003;37(2):409–417.
- Li H, Mahyoub SAA, Liao W, et al. Effect of pyrolysis temperature on characteristics and aromatic contaminants adsorption behavior of magnetic biochar derived from pyrolysis oil distillation residue. Bioresour Technol. 2017;223:20–26.
- Dong CD, Chen CW, Hung CM. Synthesis of magnetic biochar from bamboo biomass to activate persulfate for the removal of polycyclic aromatic hydrocarbons in marine sediments. Bioresour Technol. 2017;245:188–195.
- Dai SJ, Zhao YC, Niu DJ, et al. Preparation and reactivation of magnetic biochar by molten salt method: relevant performance for chlorine-containing pesticides abatement. J Air Waste Manag Assoc. 2018;69:1–13.
- Zhao H, Lang Y. Adsorption behaviors and mechanisms of florfenicol by magnetic functionalized biochar and reed biochar. J Taiwan Inst Chem Eng. 2018;88:152–160.
- Ahmed MB, Zhou JL, Ngo HH, et al. Insight into biochar properties and its cost analysis. Biomass Bioenergy. 2016;84:76–86.
- Ahmed MB, Zhou JL, Ngo HH, et al. Adsorptive removal of antibiotics from water and wastewater: progress and challenges. Sci Total Environ. 2015;532:112–126.
- Du SK, Zhu X, Wang H, et al. High pressure assist-alkali pretreatment of cotton stalk and physiochemical characterization of biomass. Bioresour Technol. 2013;148:494–500.
- Ma Y, Wang Q, Sun X, et al. A novel magnetic biochar from spent shiitake substrate: characterization and analysis of pyrolysis process. Biomass Convers Biorefin. 2014;5(4):339–346.
- Hu X, Xu J, Wu M, et al. Effects of biomass pre-pyrolysis and pyrolysis temperature on magnetic biochar properties. J Anal Appl Pyrolysis. 2017;127:196–202.
- Sun P, Hui C, Azim Khan R, et al. Efficient removal of crystal violet using Fe3O4-coated biochar: the role of the Fe3O4 nanoparticles and modeling study their adsorption behavior. Sci Rep. 2015;5(1):12638.
- Yaoyu Z, Yangzhou H, Yangzhuo X, et al. Analyses of tetracycline adsorption on alkali-acid modified magnetic biochar: site energy distribution consideration. SciTotal Environ. 2019;650:2260–2266.
- Reguyal F, Sarmah AK. Adsorption of sulfamethoxazole by magnetic biochar: effects of pH, ionic strength, natural organic matter and 17α-ethinylestradiol. SciTotal Environ. 2018;628–629: 722–730.
- Bombuwala Dewage N, Liyanage AS, Smith Q, et al. Fast aniline and nitrobenzene remediation from water on magnetized and nonmagnetized Douglas fir biochar. Chemosphere. 2019;225:943–953.
- Kong X, Liu Y, Pi J, et al. Low-cost magnetic herbal biochar: characterization and application for antibiotic removal. Environ Sci Pollut Res. 2017;24(7):6679–6687.
- Oladipo AA, Ifebajo AO. Highly efficient magnetic chicken bone biochar for removal of tetracycline and fluorescent dye from wastewater: two-stage adsorber analysis. J Environ Manage. 2018;209:9–16.
- Fengfeng M, Baowei Z. Adsorption characteristics of p-nitrophenol removal by magnetic biochar. China Environ Sci. 2019;39:170–178.
- Hao Z, Wang C, Yan Z, et al. Magnetic particles modification of coconut shell-derived activated carbon and biochar for effective removal of phenol from water. Chemosphere. 2018;211:962–969.
- Jiang Y, Yang K, Shang Y, et al. Response and recovery of aerobic granular sludge to pH shock for simultaneous removal of aniline and nitrogen. Chemosphere. 2019;221:366–374.
- Dong C-D, Chen C-W, Tsai M-L, et al. Degradation of 4-nonylphenol in marine sediments by persulfate over magnetically modified biochars. Bioresour Technol. 2019;281:143-8.
- Pi Z, Li X, Wang D, et al. Persulfate activation by oxidation biochar supported magnetite particles for tetracycline removal: Performance and degradation pathway. Journal of Cleaner Production. 2019;235:1103-15.
- Yuan S-J, Dai X-H. Facile synthesis of sewage sludge-derived mesoporous material as an efficient and stable heterogeneous catalyst for photo-Fenton reaction. Appl Catal B Environ. 2014;154–155:252–258.
- Dong X, He L, Hu H, et al. Removal of 17β-estradiol by using highly adsorptive magnetic biochar nanoparticles from aqueous solution. Chem Eng J. 2018;352:371–379.
- Dong CD, Chiu-Wen C, Chang-Mao H. Persulfate activation with rice husk-based magnetic biochar for degrading PAEs in marine sediments. Environ Sci Pollut Res. 2019;26(33):33781–33790.
- Mubarak NM, Sahu JN, Abdullah EC, et al. Plam oil empty fruit bunch based magnetic biochar composite comparison for synthesis by microwave-assisted and conventional heating. J Anal Appl Pyrolysis. 2016;120:521–528.
- Hu X, Long L, Gong T, et al. Enhanced alginate-based microsphere with the pore-forming agent for efficient removal of Cu(II). Chemosphere. 2020;240:124860.
- Yan J, Xue Y, Long L, et al. Adsorptive removal of As(V) by crawfish shell biochar: batch and column tests. Environ Sci Pollut Res. 2018;25(34):34674–34683.
- Wu Y, Guo J, Han Y, et al. Insights into the mechanism of persulfate activated by rice straw biochar for the degradation of aniline. Chemosphere. 2018;200:373–379.
- Reguyal F, Sarmah AK. Site energy distribution analysis and influence of Fe3O4 nanoparticles on sulfamethoxazole sorption in aqueous solution by magnetic pine sawdust biochar. Environ Pollut. 2017;233:510.
- Yang K, Yang J, Jiang Y, et al. Correlations and adsorption mechanisms of aromatic compounds on a high heat temperature treated bamboo biochar. Environ Pollut. 2016;210:57–64.
- Ai T, Jiang X, Liu Q, et al. Daptomycin adsorption on magnetic ultra-fine wood-based biochars from water: kinetics, isotherms, and mechanism studies. Bioresour Technol. 2018;273:8–15.
- Heo J, Yoon Y, Lee G, et al. Enhanced adsorption of bisphenol A and sulfamethoxazole by a novel magnetic CuZnFe2O4–biochar composite. Bioresour Technol. 2019;281:179–187.
- Villacanas F, Pereira MF, Orfao JJ, et al. Adsorption of simple aromatic compounds on activated carbons. J Colloid Interface Sci. 2006;293(1):128–136.
- Wu G-Q, Zhang X, Hui H, et al. Adsorptive removal of aniline from aqueous solution by oxygen plasma irradiated bamboo based activated carbon. Chem Eng J. 2012;185–186:201–210.
- Gao D-W, Hu Q, Pan H, et al. High-capacity adsorption of aniline using surface modification of lignocellulose-biomass jute fibers. Bioresour Technol. 2015;193:507–512.
- Roshan B, Kadirvelu K, Kumar NS. Investigation of aniline adsorption onto spherical carbon: optimization using response surface methodology. J Eng Res Appl. 2013;3:943–952.
- Kakavandi B, Jonidi Jafari A, Rezaei Kalantary R, et al. Simultaneous adsorption of lead and aniline onto magnetically recoverable carbon: optimization, modeling and mechanism. J Chem Technol Biot. 2016;91(12):3000–3010.