ABSTRACT
There are many literatures on the importance of bioresources from microalgae, but this review shows how microalgae are able to respond to the stress induced by microplastics (MP) and how the process leads to the production of bioresources. The study of these bioresources is significant because they are harnessed by man as energy and pharmaceutical tools. The extent of MP in the aquatic environment, microalgae stress induced by MP, the response of microalgae to stress induced by MP and bioresource production in microalgae due to stress induced by MP are described. The study shows that the presence of MP in the environment can induce nutrients and environmental stress, which enhances lipid accumulation and the production of other useful biotechnology resources. The study also shows that microalgae tend to produce resources that can respond to the stress induced by MP, which leads to a high removal efficiency of MP in aquatic ecosystems. The study also shows that the production of bioresources in the form of antioxidants, which are either enzymatic or non-enzymatic antioxidants, is the basic reason why microalgae are able to remove MP. A number of studies have shown the effect of MP on microalgae, while others have shown the efficiency of microalgae in the removal of MP from aquatic systems. But scanty literature exists showing how MP induces stress on microalgae. More studies are needed to show the mechanism and the concentration of bioresources produced by microalgae under the stress of MP.
1. Introduction
The poor method of plastic management in developing countries such as Nigeria is worrisome due to the risk associated with these pollutants on the proper functioning of the aquatic ecosystem [Citation1]. Several studies have been documented on the effects and management of larger plastics, but scanty literature exists on the fate, effects and management of MP, which is a product of larger plastics [Citation2]. MP are types of emerging contaminants that are worrisome due to the effect they exert on aquatic flora and fauna [Citation3,Citation4]. MP are pollutants that are less than 5 mm in length [Citation5,Citation6]. They are the bye-products of cosmetics, pharmaceutics, clothing, and industrial processes [Citation7,Citation8]. These pollutants persist in the aquatic environment, leading to bioaccumulation across the trophic level [Citation9]. MP are substances whose environmental and health effects have yet to be determined due to a lack of knowledge about the interactions and toxicological effects of the contaminants [Citation10]. The types of MP include antibiotics, surfactants, pesticides, pharmaceuticals, personal care products, effluents and nanomaterial [Citation11]. The limited information on the environmental fate of MP is responsible for the lack of management technology and the inability of regulatory agencies to develop proper regulation [Citation12].
Microalgae are microscopic, unicellular plant-like organisms that range in size from a few to a hundred micrometres [Citation13]. They are referred to as the most important tool in the aquatic ecosystem because they are the primary producers in the aquatic ecosystem [Citation14]. Microalgae can form biofilms with MP, leading to the formation of extracellular polymeric substances (EPS) [Citation15,Citation16]. This is detrimental to the balance of the aquatic ecosystem due to the dependence of aquatic fauna on microalgae [Citation17]. MP has been shown to cause blockage of the digestive tract, alteration of feeding habits, disruption of feeding urge, growth retardation, and reproduction inefficiency in aquatic fauna [Citation18,Citation19].
Microalgae are able to prevent the negative effect of MP on aquatic organisms by responding to any effect [Citation20]. The response tends to counteract the effect of MP on the microalgae, thereby preventing the accumulation of MP on the surface of microalgae and associated substrate [Citation21]. This response also led to the production of bioresources that are harnessed by man as energy and pharmaceutical bioresources [Citation22]. There are many literatures on the importance of bioresources from microalgae, but this review is the first to show how microalgae are able to respond to the stress induced by MP and how the process leads to the production of bioresources.
2. Extent of MP pollutants in aquatic environment
The increasing generation of plastic waste is due to the increase in the production of plastic and the manufacturing of plastic related materials. Citation23,reported that about 275 million metric tons of plastic was produced in 2010 by coastal countries. Citation24,reported the production of about 6300 million metric tons of plastic worldwide [Citation24]. A small proportion of the plastic produced is recycled while a larger proportion is released into landfills and aquatic bodies [Citation23]. An MP is produced when plastic material breaks down into pieces ranging from 5 millimetres to 100 nanometres [Citation25]. MP can also originate from other sources, including paint, domestic, sewage and other wastewaters [Citation26]. The two sources of MP are primary and secondary sources of MP, Primary Mp are MP that are produce from product such as personal care products, hand cleaners facial cleaner and tooth paste [Citation27]. This is because majority of these compounds contain PP (polypropylene), PE (polyethylene) and PS (polystyrene) [Citation28]. Secondary MP is MP resulting from the breakage of larger plastic pollutants [Citation29]. These pollutants are originated from land-based sources, which result from plastic littering and improper management of landfill sites [Citation30]. Citation31,reported that the larger amount of MP in the aquatic environment is as a result of secondary sources [Citation31]. This study stressed that the majority of MP in the aquatic environment is due to the breakdown of polymers such as LDPE (low density polyethylene), HDPE (high-density polyethylene), PP and nylon. This breakage is due to photo-oxidation assisted degradation caused by UV radiation and microorganism assisted oxidation [Citation32]. Weathering and mechanical forces including wave action and abrasion with sand also play a significant role in the breakage of plastic into MP [Citation33]. MP is heterogeneous pollutants due to their difference in size, shape, density and chemical composition because they are product of different plastic substance [Citation34]. Studies have shown that MP tends to accumulate in aquatic biota and substrate, leading to unbalance in the aquatic environment because of poor knowledge of the extent of MP in the aquatic ecosystem [Citation26]. The route of entry of MP into the aquatic environment is as a result of the discharge of domestic and industrial wastewater [Citation35,Citation36]. MP in leachates can also enter the aquatic environment from landfill as a result of runoff into the nearby aquatic environment [Citation37]. Recent studies by Citation38,on the distribution of MP in freshwater show variation in the concentration of MP among different freshwater sources, including stormwater, wetland, lake, river, estuarine, stream, glacier, and snow after analysis of 109 studies. The study reported a higher concentration of MP in the glacier and snow with a n value equal to 878. The high concentration of MP in glaciers and snow over the rest of the freshwater source is due to the net accumulation of MP during snow deposition due to the narrow chance of being washed off. Whereas, MP in the other sources of freshwater can be easily washed off by runoff even after accumulation [Citation38].
MP is persistence in the water column and sediment of the aquatic environment, as reported by Citation39. The persistence nature of MP is the reason why Ellen Macarthur Foundation predicted that the amount of MP will exceed the total number of fish in the aquatic environment [Citation6]. The aquatic sediment is referred to as the long-time sink for MP due to the deposition and accumulation of MP in sediments [Citation39]. A recent study by Citation40,demonstrated that the deposition and accumulation of MP in aquatic sediment tends to vary with depth, with sediment in the upper layer having more MP than sediment in the deeper layer. This study shows that recent sediment has higher MP than older sediment [Citation40]. A critical review by Citation41,gives details on the methods and concentration of MP detected by various researchers in different water sources [Citation41]. MP is toxic to aquatic organism and also acts as a substrate for the aggregation of toxic pollutants [Citation42]. This toxicity is as a result of the ingestion of MP which bioaccumulate and biomagnify across the food chain and food web. The bioaccumulation and biomagnification across the trophic level is due to the variation of MP in shape and density. This diversification of MP is the reason why organisms at every stage of the trophic level tend to ingest and accumulate MP [Citation43].
3. Microalgae stress induced by MP
MP tends to accumulate in aquatic biota due to its slow degradation rate [Citation44]. The accumulation of MP is detrimental to microalgae and makes microalgae the sole responsibility of MP flow across the trophic level [Citation45,Citation46]. MP also tends to negatively affect microalgae, leading to oxidative stress. The stress induced by MP to microalgae includes nutrient induced stress, environmental induced stress and oxidative induced stress [Citation47]. MP exposure to microalgae and its effects based on many effect criteria has been well summarized by Citation48.
MP induces nutrient stress on microalgae due to its ability to accumulate and magnify in the aquatic ecosystem. The accumulated MP stimulates the formation of biofilm, with the MP serving as the substrate [Citation49]. This biofilm increases the use of nutrients present in the aquatic ecosystem, leading to nutrient starvation [Citation48]. The state of nutrient starvation is referred to as nutrient stress because the process leads to the production of a high amount of reactive oxygen species (ROS) [Citation50,Citation51]. The advantage of nutrient stress is that the microalgae accumulate more lipid and also produce more bioresources in order to counteract the effect of ROS [Citation52]. MP is able to accumulate and transport harmful pollutants in the aquatic environment [Citation9]. This phenomenon hinders the penetration of sunlight, causes oxygen depletion and changes in the temperature of the water [Citation53]. This environmental factor alteration leads to stress on the microalgae, increased lipid accumulation, and an increase in bioresource production [Citation54]. The high production of bioresources is intended to cancel out any effect of ROS caused by oxidative stress caused by changes in environmental factors () [Citation55]. MP accumulation also alters the salinity and pH of the aquatic environment. The ability of microalgae to withstand the stress induced by salinity varies from species to species because the stress tends to interfere with the process of photosynthesis [Citation56]. The optimal pH for the growth of microalgae ranges from 8.2–8.7 and is also dependent on the species involved [Citation57].
4. Response of microalgae to stress induce by MP
Microalgae are the most important component of the aquatic environment due to their role as producers in the food chain and food web [Citation58]. The increased level of MP in the aquatic ecosystem is alarming because of its negative impact on microalgae which induce stress [Citation59]. This stress leads to the alteration of the morphology and physiology of the microalgae [Citation60]. In order to counteract the effect induced by MP, microalgae develop various response mechanisms which lead to the development of useful bioresources [Citation22]. Microalgae are able to respond to the stress induced by MP pollutants via morphological changes [Citation61]. Microalgae forms biofilm with MP and other toxic pollutants acting as a substrate, this biofilm tend to limit the amount of sunlight that penetrates into the aquatic ecosystem and also increases the utilization of nutrients present in the system [Citation62,Citation63]. The limiting of sunlight penetration leads to oxidative stress, while the increased utilization of nutrients leads to nutrient starvation in the system [Citation64]. Microalgae are able to counteract any effect that may result from stress caused by the limitation in sunlight penetration by increasing the amount of cellular chlorophyll production [Citation65]. Although, the limitation of sunlight leads to a reduction in microalgae growth and volume, the higher cellular chlorophyll produced by the microalgae annuls any effect caused by the stress by the utilization of the available sunlight energy present in the system [Citation66]. Nutrient starvation also causes stress in the microalgae, which affects biomass production, but microalgae are able to counteract the effect by the production and accumulation of more lipids in the microalgae cell [Citation67]. The aggregation of toxic pollutants by MP also increases the pH and salinity of the water, thereby contributing to the environmental stress induced on the microalgae [Citation68]. Both the environmental and nutrient stress induced by MP on microalgae lead to oxidative stress which involves increases in the amount of ROS produced by the microalgae [Citation69]. The increase in ROS produced by the microalgae is the reason why the growth and biomass production of the microalgae is retarded [Citation70,Citation71]. This effect is due to the ability of ROS to damage the deoxyribonucleic acid (DNA) of the nucleus, chloroplast and mitochondria [Citation72]. The damage to these organelles, which are the vital parts of the microalgae, causes mutation and genetic instability [Citation73]. Microalgae are able to annul any effects associated with over production of ROS by an antioxidant and non-antioxidant response mechanism () [Citation74,Citation75]. A detailed review on how microalgae are able to respond to over production of ROS can be found in the study of Citation21.
Figure 2. Response mechanisms of microalgae to stress induce by MP (a) MP stress induce (b) Enzymatic antioxidant response mechanism c non-enzymatic antioxidant mechanism (d) mechanism of radical scavenging by ascorbic acid [Citation76,Citation109].
![Figure 2. Response mechanisms of microalgae to stress induce by MP (a) MP stress induce (b) Enzymatic antioxidant response mechanism c non-enzymatic antioxidant mechanism (d) mechanism of radical scavenging by ascorbic acid [Citation76,Citation109].](/cms/asset/60a592de-6bce-4e79-baab-f5ef5f0b3c2e/tcsb_a_2033138_f0002_oc.jpg)
5. Mp induce bioresources production in microalgae
A number of studies have shown the strategies that can be used to enhance the production of bioresources by microalgae [Citation77]. The available studies have placed emphasis on the manipulation of conditions to cause nutrient and environmental stress [Citation78,Citation79]. The current study shows that the presence of MP in the environment can also induce nutrients and environmental stress which enhances lipid accumulation and production of other useful biotechnology resources [Citation8,Citation80]. MP can either cause a negative effect on the microalgae biomass concentration or have no inhibitory effect on the morphology of microalgae [Citation81]. The production of bioresources in the form of antioxidants, which are either enzymatic or non-enzymatic antioxidants is the basic reason why microalgae are able to remove MP from water, Although there is scanty literature showing bioresources production by microalgae under MP stress as presented in [Citation82]. The ability of microalgae to degrade MP is dependent on the production of a high amount of ROS, which stimulates the release of an enzyme that is meant to scavenge the excess ROS produced [Citation83,Citation84]. The enzymatic and non-enzymatic antioxidants that are produced in high amounts to counteract the effects induced by MP include polyphenol, carotenoid, proline, ascorbic acid, tocopherol, superoxide dismutase (SOD), peroxidase (POD), catalase (CAT), and glutathione reductase (GR) [Citation85]. This antioxidants are produce by microalgae in response to the stress induce by MP [Citation86]. These antioxidants are biological compounds that help the microalgae under the stress of ROS overproduction by quenching ROS and inhibiting lipid peroxidation [Citation87,Citation88]. This is why microalgae lipid accumulation increases under environmental and nutrient stress induced by MP [Citation89].
Table 1. Microplastics induce bioresources production in microalgae
Polyphenols are referred to as chemical compounds that contain hydroxylated aromatic rings [Citation90]. They are classified based on the number of units representing phenol in the compound [Citation91]. They are the most abundant secondary metabolites derived from microalgae due to their strong antioxidant properties [Citation92]. They are the most important biotechnological tools that are useful in medicine as anticarcinogenic, antimutagenic, and anti-inflammatory compounds [Citation93]. These compounds are produced by microalgae under stressful conditions to counteract the oxidative stress caused by nutrient and environmental factors [Citation94]. This study predicted that prolonged exposure of microalgae to MP tends to increase the production of polyphenol compounds because, in the presence of MP, the production of ROS increases [Citation95]. This prompts the need for the antioxidant system in order to annul any effects caused by the exposure [Citation96]. Although there is scanty literature showing the effect of MP on microalgae polyphenol production, a study by Citation97,depicts the variation in phenolic compound production as a function of the species of microalgae grown in industrial wastewater [Citation97]. This is an indication that the ability of microalgae to produce polyphenol compounds is dependent on the source of stress, the species of microalgae involved, and the extent of MP in the environment.
Another important non-enzymatic biomolecule produced by microalgae under the stress of MP is proline, tocopherol, and ascorbic acid, which are important biotechnological tools used in medicine [Citation98,Citation99]. Proline, for instance, is a vital biomolecule in the synthesis of protein due to its unique properties as a secondary amine [Citation100]. Although proline is a type of non-essential amino acid due to the ability of the human body to produce it, proline has been linked to the ability to heal the skin of people who do not produce or store much proline. Deep study into the use of proline has linked L-proline to osmoprotection, making it a vital pharmaceutical and biotechnological tool [Citation101,Citation102]. The ability of microalgae to tolerate the stress induced by Mp is also due to the presence of proline [Citation103,Citation104]. This stress induced by MP causes the increased production of proline by microalgae in order for the microalgae to counteract the oxidative effect resulting from the higher level of ROS produced by the microalgae [Citation105] (). Proline is utilized as a supplement for growth medium, especially in tissue culture experiments, due to its ability to prevent plant stress and increase plant yield [Citation106]. This shows that the production of proline by microalgae during stress induced by MP prevents oxidative stress on the growth and yield of microalgae biomass [Citation107]. Tocopherol and ascorbic acid are the most important medical and pharmaceutical tools derived from microalgae under stressful conditions due to the presence of vitamins in both antioxidants [Citation108]. Both antioxidants tend to deliver hydrogen ions in order to quench the activities of free radicals [Citation109]. The ability of tocopherol and ascorbic acid to donate hydrogen atoms to ROS is due to the weak bond in the antioxidants, which is about 10% weaker than the bond of polyphenols () [Citation110]. The ability of microalgae under stressful conditions to successfully annul the effect of ROS could also be linked to their ability to produce tocopherol and ascorbic acid [Citation111].
Figure 3. Microalgae increase in proline, ascorbic acid and tocopherol in response to stress induce by MP.
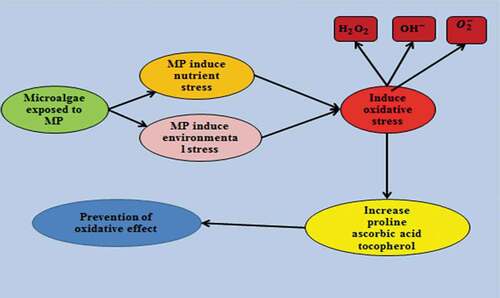
Enzymatic antioxidants are another important bioresource produced by microalgae under stressful conditions. These molecules are produced in response to stress induced by MP due to their ability to annul any effect of ROS produced by the microalgae [Citation109]. Enzymatic antioxidants tend to transform ROS and its metabolic products into harmless compounds. They tend to prevent the peroxidation of microalgae lipids and maintain the structure and function of the microalgae cell membrane. The process and mechanisms of how microalgae enzymatic antioxidants scavenge ROS have been summarized in a review by Citation21. The current review focuses on the economic importance of the enzymatic antioxidants produced by microalgae under the stress induced by MP. SOD is a medically important enzymatic antioxidant due to its therapeutic properties to annul physiological and pathological conditions such as cancer, inflammatory diseases, cystic fibrosis, ischemia, aging, rheumatoid arthritis, neurodegenerative diseases, and diabetes. Although SOD shows some limitations in clinical application, as such, its applications are limited to non-drug application in humans [Citation112,Citation113]. A detailed review on the role of SOD as a therapeutic agent is summarized in the work of Citation113. SOD is also an important industrial tool which was introduced to the market in 1990. Although the application of SOD in animals is drug application, but vice versa in humans [Citation114]. A detailed review conducted by Citation114,shows the industrial application of SOD. POD, CAT, and GR are enzymatic antioxidants that act on H2O2 and convert it into harmless water and oxygen. POD and CAT are widely utilized in industrial wastewater treatment and are also important tools used as part of preservatives [Citation115]. GR, on the other hand, is useful and has found important application in the field of medical biotechnology [Citation116].
6. Conclusion
The current study shows that MP tends to induce nutrient and environmental stress on microalgae. This stress increases the rate of ROS production, leading to oxidative stress on the microalgae. Microalgae tend to respond to the stress induced by MP by accumulation of lipids and production of bioresources, which are important biotechnological and medical tools. These bioresources are produced by microalgae in order to counteract any effects resulting from the exposure of the microalgae to MP. A number of studies have shown the effect of MP on microalgae, but scanty literature exists showing how MP induces stress on microalgae. There is also scanty literature showing how the stress induced by MP causes the production of some bioresources such as tocopherol, polyphenols, proline, and ascorbic acid. More studies are needed to fill in this gap and also show how the concentration of these bioresources tends to vary under the stress of different MP.
Disclosure statement
The authors declare that they have no known competing financial interests or personal relationships that could have appeared to influence the work reported in this paper.
Data availability statements
Data sharing not applicable to this article as no datasets were generated or analysed during the current study.
References
- Ferronato N, Torretta V. Waste mismanagement in developing countries: a review of global issues. Int J Environ Res Public Health. 2019;16(6):1060.
- Prata JC, Silva ALP, Da Costa JP, et al. Solutions and integrated strategies for the control and mitigation of plastic and microplastic pollution. Int J Environ Res Public Health. 2019c;16(13):2411.
- Gomes IB, Maillard J-Y, Simões LC, et al. Emerging contaminants affect the microbiome of water systems—strategies for their mitigation. Npj Clean Water. 2020;3(1):39.
- Patel M, Kumar R, Kishor K, et al. Pharmaceuticals of emerging concern in aquatic systems: chemistry, occurrence, effects, and removal methods. Chem Rev. 2019;119(6):3510–3673.
- Dey TK, Uddin ME, Jamal M. Detection and removal of microplastics in wastewater: evolution and impact. Environ Sci Pollut Res. 2021;28(14):16925–16947.
- Picó Y, Barceló D. Analysis and prevention of microplastics pollution in water: current perspectives and future directions. ACS Omega. 2019;4(4):6709–6719.
- Guerranti C, Martellini T, Perra G, et al. Microplastics in cosmetics: environmental issues and needs for global bans. Environ Toxicol Pharmacol. 2019;68:75–79.
- Liu G, Jiang R, You J, et al. microplastic impacts on microalgae growth: effects of size and humic acid. Environ Sci Technol. 2020;54(3):1782–1789.
- Miller ME, Hamann M, Kroon FJ. Bioaccumulation and biomagnification of microplastics in marine organisms: a review and meta-analysis of current data. PloS One. 2020;15(10):e0240792–e0240792.
- Naidoo RK, Simpson ZF, Oosthuizen JR, et al. Nutrient exchange of carbon and nitrogen promotes the formation of stable mutualisms between chlorella sorokiniana and Saccharomyces cerevisiae under engineered synthetic growth conditions. 2019;10.
- Freyria FS, Geobaldo F, Bonelli B. Nanomaterials for the abatement of pharmaceuticals and personal care products from wastewater. Appl Sci. 2018;8.
- Luo Y, Guo W, Ngo HH, et al. A review on the occurrence of micropollutants in the aquatic environment and their fate and removal during wastewater treatment. SciTotal Environ. 2014;473-474:619–641.
- Ugya AY, Hasan DUB, Ari HA, et al. Natural freshwater microalgae biofilm as a tool for the clean-up of water resulting from mining activities. All Life. 2020a;13(1):644–657.
- Omar WMW. Perspectives on the use of algae as biological indicators for monitoring and protecting aquatic environments, with special reference to malaysian freshwater ecosystems. Trop Life Sci Res. 2010;21(2):51–67.
- Decho AW, Gutierrez T. Microbial Extracellular Polymeric Substances (EPSs) in ocean systems. Front Microbiol. 2017;8:922.
- Ugya YA, Hasan DUB, Tahir SM, et al. Microalgae biofilm cultured in nutrient-rich water as a tool for the phycoremediation of petroleum-contaminated water. Int J Phytoremediation. 2021c;1–9.
- Fanesi A, Paule A, Bernard O, et al. The architecture of monospecific microalgae biofilms. Microorganisms. 2019;7(9):352.
- Setälä O, Norkko J, Lehtiniemi M. Feeding type affects microplastic ingestion in a coastal invertebrate community. Mar Pollut Bull. 2016;102(1):95–101.
- Ugya AY, Ajibade FO, Hua X. The efficiency of microalgae biofilm in the phycoremediation of water from river Kaduna. J Environ Manage. 2021a;295:113109.
- Issac MN, Kandasubramanian B. Effect of microplastics in water and aquatic systems. Environ Sci Pollut Res. 2021;28(16):19544–19562.
- Ugya AY, Imam TS, Li A, et al. Antioxidant response mechanism of freshwater microalgae species to reactive oxygen species production: a mini review. Chem Ecol. 2020b;36(2):174–193.
- Ugya AY, Ari HA, Hua X. Microalgae biofilm formation and antioxidant responses to stress induce by Lemna minor L., Chlorella vulgaris, and Aphanizomenon flos-aquae. Ecotoxicol Environ Saf. 2021b;221:112468.
- Aragaw TA. Microplastic pollution in African countries’ water systems: a review on findings, applied methods, characteristics, impacts, and managements. SN Appl Sci. 2021;3(6):629.
- Geyer R, Jambeck JR, Law KL. Production, use, and fate of all plastics ever made. Sci Adv. 2017;3(7):e1700782.
- Jiang B, Kauffman AE, Li L, et al. Health impacts of environmental contamination of micro- and nanoplastics: a review. Environ Health Prev Med. 2020;25(1):29.
- Barrett J, Chase Z, Zhang J, et al. Microplastic pollution in deep-sea sediments from the great Australian bight. 2020;7.
- Zitko V, Hanlon M. Another source of pollution by plastics: skin cleaners with plastic scrubbers. Mar Pollut Bull. 1991;22(1):41–42.
- Napper IE, Bakir A, Rowland SJ, et al. Characterisation, quantity and sorptive properties of microplastics extracted from cosmetics. Mar Pollut Bull. 2015;99(1–2):178–185.
- D’Angelo S, Meccariello R. Microplastics: a threat for male fertility. Int J Environ Res Public Health. 2021;18(5):2392.
- Sobhani Z, Lei Y, Tang Y, et al. Microplastics generated when opening plastic packaging. Sci Rep. 2020;10(1):4841.
- An L, Liu Q, Deng Y, et al. Microplastics in terrestrial environments: emerging contaminants and major challenges. In: He D, Luo Y, editors. Sources of microplastic in the environment. Cham: Springer International Publishing; 2020. p. 143–159.
- Yousif E, Haddad R. Photodegradation and photostabilization of polymers, especially polystyrene: review. SpringerPlus. 2013;2(1):398.
- Thompson RC, Olsen Y, Mitchell RP, et al. Lost at sea: where is all the plastic? Science. 2004;304(5672):838.
- Duis K, Coors A. Microplastics in the aquatic and terrestrial environment: sources (with a specific focus on personal care products), fate and effects. Environ Sci Eur. 2016;28(1):2.
- Bashir I, Lone FA, Bhat RA, et al. Concerns and threats of contamination on aquatic ecosystems. In: Bioremediation and biotechnology: sustainable approaches to pollution degradation. 2020. p. 1–26.
- Hubeny J, Harnisz M, Korzeniewska E, et al. Industrialization as a source of heavy metals and antibiotics which can enhance the antibiotic resistance in wastewater, sewage sludge and river water. PLOS ONE. 2021;16(6):e0252691.
- Silva ALP, Prata JC, Duarte AC, et al. Microplastics in landfill leachates: the need for reconnaissance studies and remediation technologies. Case Stud Chem Environ Eng. 2021;3:100072.
- Koutnik VS, Leonard J, Alkidim S, et al. Distribution of microplastics in soil and freshwater environments: global analysis and framework for transport modeling. Environ Pollut. 2021;274:116552.
- Fan Y, Zheng K, Zhu Z, et al. Distribution, sedimentary record, and persistence of microplastics in the pearl river catchment, China. Environ Pollut. 2019;251:862–870.
- Willis KA, Eriksen R, Wilcox C, et al. Microplastic distribution at different sediment depths in an urban estuary. 2017;4.
- Koelmans AA, Mohamed Nor NH, Hermsen E, et al. Microplastics in freshwaters and drinking water: critical review and assessment of data quality. Water Res. 2019;155:410–422.
- Avio CG, Gorbi S, Milan M, et al. Pollutants bioavailability and toxicological risk from microplastics to marine mussels. Environ Pollut. 2015;198:211–222.
- de Sá LC, Oliveira M, Ribeiro F, et al. Studies of the effects of microplastics on aquatic organisms: what do we know and where should we focus our efforts in the future? SciTotal Environ. 2018;645:1029–1039.
- Chamas A, Moon H, Zheng J, et al. degradation rates of plastics in the environment. ACS Sustain Chem Eng. 2020;8(9):3494–3511.
- Rummel CD, Jahnke A, Gorokhova E, et al. Impacts of biofilm formation on the fate and potential effects of microplastic in the aquatic environment. Environ Sci Technol Lett. 2017;4(7):258–267.
- Wang T, Hu M, Xu G, et al. Microplastic accumulation via trophic transfer: can a predatory crab counter the adverse effects of microplastics by body defence? SciTotal Environ. 2020;754:142099.
- Sun X-M, Ren L-J, Zhao Q-Y, et al. Microalgae for the production of lipid and carotenoids: a review with focus on stress regulation and adaptation. Biotechnol Biofuels. 2018;11(1):272.
- Prata JC, Da Costa JP, Lopes I, et al. Effects of microplastics on microalgae populations: a critical review. SciTotal Environ. 2019b;665:400–405.
- Dang H, Lovell CR. Microbial surface colonization and biofilm development in marine environments. Microbiol Mol Biol Rev. 2015;80(1):91–138.
- Gray DA, Dugar G, Gamba P, et al. Extreme slow growth as alternative strategy to survive deep starvation in bacteria. Nat Commun. 2019;10(1):890.
- Liu Z, Ren Z, Zhang J, et al. Role of ROS and nutritional antioxidants in human diseases. 2018;9.
- Mondal M, Goswami S, Ghosh A, et al. Production of biodiesel from microalgae through biological carbon capture: a review. 3 Biotech. 2017;7(2):99.
- Phaniendra A, Jestadi DB, Periyasamy L. Free radicals: properties, sources, targets, and their implication in various diseases. Ind J Clin Biochem: IJCB. 2015;30(1):11–26.
- Minhas AK, Hodgson P, Barrow CJ, et al. a review on the assessment of stress conditions for simultaneous production of microalgal lipids and carotenoids. Front Microbiol. 2016;7:546.
- Valavanidis A, Vlahogianni T, Dassenakis M, et al. Molecular biomarkers of oxidative stress in aquatic organisms in relation to toxic environmental pollutants. Ecotoxicol Environ Saf. 2006;64(2):178–189.
- Cheng D, He Q. Assessment of environmental stresses for enhanced microalgal biofuel production – an overview. 2014;2.
- Hannon M, Gimpel J, Tran M, et al. Biofuels from algae: challenges and potential. Biofuels. 2010;1(5):763–784.
- Khan MI, Shin JH, Kim JD. The promising future of microalgae: current status, challenges, and optimization of a sustainable and renewable industry for biofuels, feed, and other products. Microb Cell Fact. 2018;17(1):36.
- Mohamed ZA. Polysaccharides as a protective response against microcystin-induced oxidative stress in Chlorella vulgaris and Scenedesmus quadricauda and their possible significance in the aquatic ecosystem. Ecotoxicology. 2008;17(6):504.
- Ahmad S, Kothari R, Shankarayan R, et al. Temperature dependent morphological changes on algal growth and cell surface with dairy industry wastewater: an experimental investigation. 3 Biotech. 2020;10(1):24.
- Shetty P, Gitau MM, Maróti G. salinity stress responses and adaptation mechanisms in eukaryotic green microalgae. Cells. 2019;8.
- Davey ME, O’Toole GA. Microbial biofilms: from ecology to molecular genetics. Microbiol Mol Biol Rev. 2000;64(4):847–867.
- Yin W, Wang Y, Liu L, et al. Biofilms: the microbial “protective clothing” in extreme environments. Int J Mol Sci. 2019;20(14):3423.
- Tu C, Chen T, Zhou Q, et al. Biofilm formation and its influences on the properties of microplastics as affected by exposure time and depth in the seawater. SciTotal Environ. 2020;734:139237.
- Sharma PK, Saharia M, Srivstava R, et al. Tailoring microalgae for efficient biofuel production. 2018;5.
- Levasseur W, Perré P, Pozzobon V. A review of high value-added molecules production by microalgae in light of the classification. Biotechnol Adv. 2020;41:107545.
- Alishah Aratboni H, Rafiei N, Garcia-Granados R, et al. Biomass and lipid induction strategies in microalgae for biofuel production and other applications. Microb Cell Fact. 2019;18(1):178.
- Cavicchioli R, Ripple WJ, Timmis KN, et al. Scientists’ warning to humanity: microorganisms and climate change. Nature Rev Microbiol. 2019;17(9):569–586.
- Kim KH, Choi IS, Kim HM, et al. Bioethanol production from the nutrient stress-induced microalga Chlorella vulgaris by enzymatic hydrolysis and immobilized yeast fermentation. Bioresour Technol. 2014;153:47–54.
- Prata J, Da Costa J, Lopes I, et al. Effects of microplastics on microalgae populations: a critical review. SciTotal Environ. 2019a;665.
- Singh R, Paliwal C, Nesamma AA, et al. Nutrient deprivation mobilizes the production of unique tocopherols as a stress-promoting response in a new indigenous isolate monoraphidium sp. British Journal of Biomedical Science. 2020;77(1):7.
- Rezayian M, Niknam V, Ebrahimzadeh H. Oxidative damage and antioxidative system in algae. Toxicol Rep. 2019;6:1309–1313.
- Doudican NA, Song B, Shadel GS, et al. Oxidative DNA damage causes mitochondrial genomic instability in Saccharomyces cerevisiae. Mol Cell Biol. 2005;25(12):5196–5204.
- Cirulis JT, Scott JA, Ross GM. Management of oxidative stress by microalgae. Can J Physiol Pharmacol. 2013;91(1):15–21.
- Sansone C, Brunet C. Promises and challenges of microalgal antioxidant production. Antioxidants (Basel). 2019;8:199.
- Fukai T, Ushio-Fukai M. Superoxide dismutases: role in redox signaling, vascular function, and diseases. Antioxid Redox Signal. 2011;15(6):1583–1606.
- Zhu LD, Li ZH, Hiltunen E. Strategies for lipid production improvement in microalgae as a biodiesel feedstock. Biomed Res Int. 2016;2016:8792548.
- Chu W-L. Strategies to enhance production of microalgal biomass and lipids for biofuel feedstock. Euro J Phycol. 2017;52(4):419–437.
- Rawat I, Ranjith Kumar R, Mutanda T, et al. Dual role of microalgae: phycoremediation of domestic wastewater and biomass production for sustainable biofuels production. Appl Energy. 2011;88(10):3411–3424.
- Lagarde F, Olivier O, Zanella M, et al. Microplastic interactions with freshwater microalgae: hetero-aggregation and changes in plastic density appear strongly dependent on polymer type. Environ Pollut. 2016;215:331–339.
- Nguyen HT, Yoon Y, Ngo HH, et al. The application of microalgae in removing organic micropollutants in wastewater. Crit Rev Environ Sci Technol. 2021;51(12):1187–1220.
- Khalid M. Nanotechnology and chemical engineering as a tool to bioprocess microalgae for its applications in therapeutics and bioresource management. Crit Rev Biotechnol. 2020;40(1):46–63.
- Jeong C-B, Kang H-M, Lee M-C, et al. Adverse effects of microplastics and oxidative stress-induced MAPK/Nrf2 pathway-mediated defense mechanisms in the marine copepod Paracyclopina nana. Sci Rep. 2017;7(1):41323.
- Ugya AY, Hua X, Agamuthu P, et al. Molecular approach to uncover the function of bacteria in petrochemical refining wastewater: a mini review. Appl Ecol Environ Res. 2019;17(2):3645–3665.
- Meitha K, Pramesti Y, Suhandono S. Reactive oxygen species and antioxidants in postharvest vegetables and fruits. Int J Food Sci. 2020;2020:8817778.
- Roy U, Nielsen B, Milledge J. Antioxidant production in dunaliella. Appl Sci. 2021;11(9):3959.
- Guo Y, Ma W, Li J, et al. Effects of microplastics on growth, phenanthrene stress, and lipid accumulation in a diatom. Phaeodactyl Tricornut Environ Poll. 2020;257:113628.
- Mallick N, Mohn FH. Reactive oxygen species: response of algal cells. J Plant Physiol. 2000;157(2):183–193.
- Cole M, Coppock R, Lindeque PK, et al. Effects of nylon microplastic on feeding, lipid accumulation, and moulting in a coldwater copepod. Environ Sci Technol. 2019;53(12):7075–7082.
- Pandey KB, Rizvi SI. Plant polyphenols as dietary antioxidants in human health and disease. Oxid Med Cell Longev. 2009;2(5):270–278.
- Tsao R. Chemistry and biochemistry of dietary polyphenols. Nutrients. 2010;2(12):1231–1246.
- Sathasivam R, Radhakrishnan R, Hashem A, et al. Microalgae metabolites: a rich source for food and medicine. Saudi J Biol Sci. 2019;26(4):709–722.
- de Morais MG, Vaz BDS, de Morais EG, et al. Biologically active metabolites synthesized by microalgae. Biomed Res Int. 2015;2015:835761.
- Rico M, González AG, Santana-Casiano M, et al. Production of primary and secondary metabolites using algae. In: Tripathi BN, Kumar D, editors. Prospects and challenges in algal biotechnology. Springer Singapore Singapore; 2017. p. 311–326.
- Jerez-Martel I, García-Poza S, Rodríguez-Martel G, et al. Phenolic profile and antioxidant activity of crude extracts from microalgae and cyanobacteria strains. J Food Qual. 2017;2017:2924508.
- Ameri M, Baron-Sola A, Khavari-Nejad RA, et al. Aluminium triggers oxidative stress and antioxidant response in the microalgae Scenedesmus sp. J Plant Physiol. 2020;246-247:153114.
- Safafar H, Van Wagenen J, Møller P, et al. Carotenoids, phenolic compounds and tocopherols contribute to the antioxidative properties of some microalgae species grown on industrial wastewater. Mar Drugs. 2015;13.
- Del Mondo A, Smerilli A, Sané E, et al. Challenging microalgal vitamins for human health. Microb Cell Fact. 2020;19(1):201.
- Galasso C, Gentile A, Orefice I, et al. Microalgal derivatives as potential nutraceutical and food supplements for human health: a focus on cancer prevention and interception. Nutrients. 2019;11(6):1226.
- Kavi Kishor PB, Hima Kumari P, Sunita MSL, et al. Role of proline in cell wall synthesis and plant development and its implications in plant ontogeny. Front Plant Sci. 2015;6:544.
- Doron L, Segal NA, Shapira M. Transgene expression in microalgae—from tools to applications. 2016;7.
- Mutanda T, Naidoo D, Bwapwa JK, et al. Biotechnological applications of microalgal oleaginous compounds: current trends on microalgal bioprocessing of products. 2020;8.
- Siripornadulsil S, Traina S, Verma DPS, et al. Molecular mechanisms of proline-mediated tolerance to toxic heavy metals in transgenic microalgae. Plant Cell. 2002;14(11):2837–2847.
- Zhang LP, Mehta SK, Liu ZP, et al. Copper-induced proline synthesis is associated with nitric oxide generation in Chlamydomonas reinhardtii. Plant Cell Physiol. 2008;49(3):411–419.
- Cunha C, Faria M, Nogueira N, et al. Marine vs freshwater microalgae exopolymers as biosolutions to microplastics pollution. Environ Pollut. 2019;249:372–380.
- Pazuki A, Asghari J, Sohani MM, et al. Effects of some organic nitrogen sources and antibiotics on callus growth of indica rice cultivars. J Plant Nutrit. 2015;38(8):1231–1240.
- Zhang C, Chen X, Wang J, et al. Toxic effects of microplastic on marine microalgae skeletonema costatum: interactions between microplastic and algae. Environ Pollut. 2017;220:1282–1288.
- Schagen SK, Zampeli VA, Makrantonaki E, et al. Discovering the link between nutrition and skin aging. Dermatoendocrinol. 2012;4(3):298–307.
- Nimse SB, Pal D. Free radicals, natural antioxidants, and their reaction mechanisms. RSC Adv. 2015;5(35):27986–28006.
- Kurutas EB. The importance of antioxidants which play the role in cellular response against oxidative/nitrosative stress: current state. Nutr J. 2016;15(1):71.
- Apak R, Özyürek M, Güçlü K, et al. Antioxidant activity/capacity measurement. 1. classification, physicochemical principles, mechanisms, and Electron Transfer (ET)-based assays. J Agric Food Chem. 2016;64(5):997–1027.
- Ugya AY. The efficiency and antioxidant response of microalgae biofilm in the phycoremediation of wastewater resulting from tannery, textile, and dyeing activities. Int Aqua Res. 2021;13:289–300.
- Younus H. Therapeutic potentials of superoxide dismutase. Int J Health Sci (Qassim). 2018;12(3):88–93.
- Bafana A, Dutt S, Kumar S, et al. Superoxide dismutase: an industrial perspective. Crit Rev Biotechnol. 2011;31(1):65–76.
- Raveendran S, Parameswaran B, Ummalyma SB, et al. Applications of microbial enzymes in food industry. Food Technol Biotechnol. 2018;56(1):16–30.
- Reiter J, Pick A, Wiemann L, et al. A novel natural nadh and nadph dependent glutathione reductase as tool in biotechnological applications. SciMedCentral. 2014;2:1028.
- Li S, Wang P, Zhang C, et al. Influence of polystyrene microplastics on the growth, photosynthetic efficiency and aggregation of freshwater microalgae Chlamydomonas reinhardtii. SciTotal Environ. 2020;714:136767.
- Regalado C, García-Almendárez BE, Duarte-Vázquez MA. Biotechnological applications of peroxidases. Phytochem Rev. 2004;3(1–2):243–256.
- Hammond BR Jr., Renzi LM. Carotenoids. advances in nutrition (Bethesda, Md.). 2013;4:474–476.
- Solymosi K, Mysliwa-Kurdziel B. Chlorophylls and their derivatives used in food industry and medicine. Mini Rev Med Chem. 2017;17(13):1194–1222.
- Song C, Liu Z, Wang C, et al. Different interaction performance between microplastics and microalgae: the bio-elimination potential of Chlorella sp. L38 and Phaeodactylum tricornutum MASCC-0025. SciTotal Environ. 2020;723:138146.
- Chen Q, Li Y, Li B. Is color a matter of concern during microplastic exposure to Scenedesmus obliquus and Daphnia magna? J Hazard Mater. 2020;383:121224.
- Ansari FA, Ratha SK, Renuka N, et al. Effect of microplastics on growth and biochemical composition of microalga Acutodesmus obliquus. Algal Res. 2021;56:102296.
- Khoubnasab Jafari M, Ansarin K, Jouyban A. Comments on “use of malondialdehyde as a biomarker for assesing oxidative stress in different disease pathologies: a review”. Iran J Public Health. 2015;44(5):714–715.
- Yang W, Gao X, Wu Y, et al. The combined toxicity influence of microplastics and nonylphenol on microalgae Chlorella pyrenoidosa. Ecotoxicol Environ Saf. 2020;195:110484.
- Hadiyanto H, Khoironi A, Dianratri I, et al. Interactions between polyethylene and polypropylene microplastics and Spirulina sp. microalgae in aquatic systems. Heliyon. 2021;7(8):e07676.
- Jiang L, Wang Y, Yin Q, et al. Phycocyanin: a potential drug for cancer treatment. J Cancer. 2017;8(17):3416–3429.