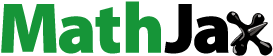
ABSTRACT
Based on the important effect of catalysts on the plasma-catalytic system, the CM/Z5 catalyst prepared by the incipient-wetness impregnation method is used for non-thermal plasma catalysis for toluene removal. The low-temperature plasma used is produced by dielectric barrier discharge. Toluene removal performance with single plasma is investigated by variation in experimental parameters. Results indicate that with the increase in discharge length, the selectivity of carbon dioxide increased. With the increase in toluene concentration and gas flow rate, carbon dioxide selectivity decreases gradually, while toluene conversion shows almost no difference. With the introduction of CM/Z5 catalyst into the back end of plasma, the toluene conversion rate and carbon dioxide selectivity could be significantly improved and ozone in the exhaust gas could be eliminated to meet emission standards at the same time. This work is meaningful in promoting the industrial application of plasma catalytic coupling technology to treat volatile organic compounds.
1. Introduction
Volatile organic compounds (VOCs) emitted from various industrial processes and human activities do great harm to both the environment and human health [Citation1]. For the abatement of low-concentration VOCs, traditional methods such as thermal combustion [Citation2], catalytic oxidation [Citation3,Citation4], adsorption and advanced oxidation [Citation5,Citation6] are not appropriate, because of their technical or economical disadvantages.
In the past few years, the non-thermal plasma (NTP) technique has been widely applied to the removal of dilute VOCs due to its unique properties, including fast response at atmospheric pressure and high electron energy within short residence time [Citation7–10]. However, NTP alone still confronted with some imperfection, such as low energy efficiency, poor CO2 selectivity and generation of undesirable by-products [Citation11,Citation12]. To overcome the limitations of NTP, many researchers have developed a new method through combining catalysis into the NTP system [Citation13,Citation14]. It has been proved that NTP coupled with catalysis can not only reduce the formation of by-products but also improve the removal efficiency [Citation15,Citation16]. This leads to the fact that plasma and catalysis take place simultaneously and interact with each other, corresponding to the ‘synergy effect’ [Citation17,Citation18]. Nevertheless, the drawbacks of plasma catalysis combined technology, such as incomplete elimination of O3 and low removal efficiency of aromatic hydrocarbons, inhibited its large-scale application.
In this work, the Cu-Mn/ZSM-5 catalyst was prepared by the successive impregnation method and combined in the post-plasma for the removal of a representative VOC (toluene). The optimal operating conditions for single plasma systems such as reactor structure parameters, discharge parameters and gas parameters that may affect toluene removal were obtained. To confirm the difference between the single plasma system and the plasma catalysis system, the toluene degradation performance of the two types of systems was investigated. The experimental results of toluene removal based on plasma-catalysis provide a guidence for the practical application of the coupling technology for the abatement of VOCs.
2. Experimental
2.1 Preparation of catalyst
The catalyst carrier is a cylinder-shaped ZSM-5 zeolite (Si/Al = 300, [diameter] = 2 mm, [length] = 10 mm, specific surface area is 325 m2/g), which was marked as Z5.
The catalyst was prepared by the successive impregnation method [Citation19,Citation20]. 40 g Z5 samples were placed in a beaker. 15.63 g of Mn(NO3)2 50 wt. % and 9.12 g of Cu(NO3)2 · 3H2O were mixed in distilled water to prepare a mixed aqueous nitrate solution. The Z5 samples were impregnated by the above mixed solution and sonicated for 30 min, then left at room temperature for 24 h. Finally, the sample was dried at 120°C for 12 h and calcined at 550°C for 4 h. The obtained catalyst sample was labeled as CM/Z5.
2.2 Experimental setup
The experimental system consisting of a gas supply system, dielectric barrier discharge (DBD) reactor system and the gas analysis system is shown in . Toluene vapor was carried by air flowing through a bubbler immersed in an ice-water mixture bath at 0°C. The DBD reactor (DBD-50, Nanjing Suman Plasma Technology Co., Ltd.), the core of the apparatus, was made of a quartz tube ([inner diameter] = 25 mm, [outer diameter] = 20 mm) and a dense wire mesh in close contact with its outer surface as a ground electrode, in the center is a corundum rod ([outer diameter] = 12 mm) connected to high voltage. A variable-frequency high-voltage AC power supply (CTP-2000 K, Nanjing Suman Plasma Technology Co., Ltd.) was applied between the two electrodes. The afterglow area of discharge is filled with CM/Z5 catalyst. The gas at the inlet and outlet of the reactor is analyzed online by gas chromatography GC 2060 equipped with a FID detector. The chromatographic column is HP-5 type capillary column ([column length] = 30 m, [inner diameter] = 0.32 mm, [inner coating thickness] = 0.25 µm). Chromatographic detection conditions are as follows: furnace temperature, 60°C; detector temperature, 300°C and injection port temperature, 100°C. The ozone concentration in the outlet tail gas was detected and analyzed by an ozone analyzer.
Figure 1. Schematic diagram of the experimental set-up. 1- Air cylinder 2- Mass flow meter 3- Ice-water mixture bath 4- Gas mixer 5- Oscilloscope 6- Plasma reactor (discharge zone) 7- Catalyst 8- Gas chromatography 9- Ozone analyzer.
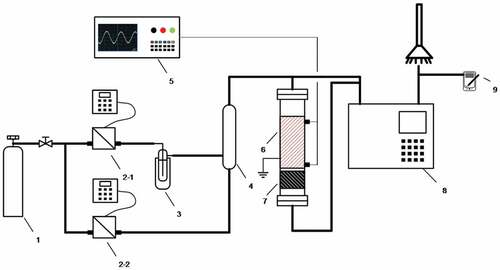
The efficiency of toluene removal (C7H8 conversion) and CO2 selectivity were calculated as follows:
Where Cin and Cout represent the inlet and outlet concentrations of toluene, CCO2 represents CO2 concentration in exhaust gas.
3. Results and discussion
3.1 Toluene degradation in NTP alone
3.1.1 Effect of valid discharge length
In order to investigate the effect of the valid discharge length of the DBD reactor on the degradation of toluene, the length of the wire mesh outside the quartz tube was changed from 6 cm to 15 cm. The reaction parameters were as follows: [C7H8 flow rate] = 250 mL/min; [C7H8 concentration] = 500 ppm; [frequency] = 9.6 kHz; [discharge voltage] = 11.9 KV. The correlations between the discharge lengths and toluene degradation efficiency were investigated, and the results are shown in . It can be seen that toluene conversions present almost no difference with the increase in discharge length from 6 cm to 15 cm. A high value of toluene conversion (> 90%) can be maintained. This result indicates that high toluene removal efficiency can be obtained without too long discharge length under this electrical parameter.
Figure 2. a). Effect of discharge length on toluene conversion; b). Effect of discharge length on CO2 selectivity; c). Effect of discharge length on ozone generation.
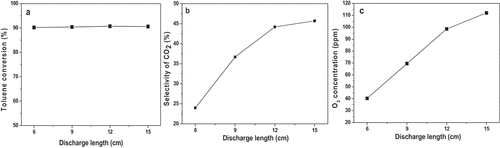
The effect of the valid discharge length on CO2 selectivity was also studied, and the results are presented in . It can be found that the discharge length has a significant influence on CO2 selectivity. As the discharge length increases from 6 cm to 15 cm, the CO2 selectivity grows from 24% to 45.7%. This may be due to the fact that as the valid discharge length increases, the residence time of gaseous toluene increases. It brings about the higher probability of toluene molecules colliding with active oxygen groups, which is beneficial for the deep oxidation of toluene. In addition, the effect of discharge length on the generation of O3 was inspected, and the results are displayed in . As can be seen, the efficiency of O3 generation shows almost the same variation regularity as CO2 selectivity with the growth of the discharge length. As the length of the discharge area increases (6→15 cm), the outlet O3 concentration gradually increases. It is for the reason that the increasing residence time of pollutants gives rise to the higher collision probability of O radicals, leading to an increase of the generation rate of O3.
In summary, the longer discharge length results in the increasing mineralization of toluene to a certain extent, but the balance between removal efficiency and economy should be considered. The discharge length is fixed at 12 cm in the following research sections.
3.1.2 Effect of discharge voltage
The reaction parameters were as follows: [C7H8 flow rate] = 250 mL/min; [C7H8 concentration] = 500 ppm; [frequency] = 9.6 kHz. The effect of the discharge voltage on C7H8 conversion was investigated. As shown in , toluene has not been converted when the voltage was low (< 6.8 kV). The blue light generated by the discharge could not be observed in the discharge area. In the case, the discharge has not started, which leads to no conversion of toluene. When the voltage increased to 8.4 kV, 26.6% of C7H8 conversion was obtained; when the voltage continuously increased to 11.9kV, the toluene conversion could reach a value of 90.7%; when the voltage continued to increase, the toluene conversion tended to be stable.
Figure 3. a). Effect of discharge voltage on toluene conversion; b). Effect of discharge voltage on CO2 selectivity; c). Effect of discharge voltage on ozone generation.
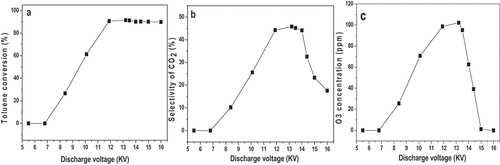
Under the same conditions, the effect of discharge voltage on CO2 selectivity was also explored, and the results are presented in . Different from the variation regularity of C7H8 conversion, after the CO2 selectivity increased to 44%, if the discharge voltage continuously increased (>14kV), the CO2 selectivity decreased. It can be explained that, with the increase in the voltage, electrons and active groups generated in the unit area increased. This resulted in a higher probability of collision with toluene molecules, and thus, the toluene conversion and CO2 selectivity grew. However, when the voltage was too high, dielectric breakdown would take place and no plasma could be formed. Even though the relatively high efficiency of toluene conversion could be maintained in plasma, the mineralization of toluene would be significantly reduced. A similar phenomenon has been reported in the literature [Citation21,Citation22].
The influence of the discharge voltage on the ozone generation is illustrated in . It can be found that with the increase of the voltage, the ozone concentration first increased and then decreased. The highest value of O3 concentration (98–102 ppm) can be attained with the discharge voltage of 11.5–13.5kV. When the voltage was lower than 11.5 kV, the O3 concentration increased with the increase in the voltage. The higher voltage leads to stronger discharge and more electrons produced, giving rise to the higher probability of collision with oxygen, which brings about an increase in the O3 concentration. When the voltage was too high (>13.5kV), the O3 concentration would decrease as the voltage increased. On the one hand, O3 will be decomposed by the bombardment of high-energy electrons with too high energy; on the other hand, with too high energy, the temperature in the discharge area will increase. The higher the temperature, the faster the O3 decomposition rate. During the process of this experiment, when the voltage was greater than 13.5 KV, the temperature in the tube could reach 150°C (measured using a FOTRIC 325C handheld thermal imager). At this temperature, ozone decomposed rapidly, leading to a decline of the ozone concentration.
3.1.3 Effect of applied frequency
To gain an insight into the influence of applied frequency, we explored the relationship between the frequency and the toluene conversion, and the results are shown in . The reaction parameters were as follows: [C7H8 flow rate] = 250 mL/min; [C7H8 concentration] = 500 ppm; [discharge voltage] = 11.9KV.
Figure 4. a). Effect of frequency on toluene conversion; b). Effect of frequency on CO2 selectivity; c). Effect of frequency on ozone generation.
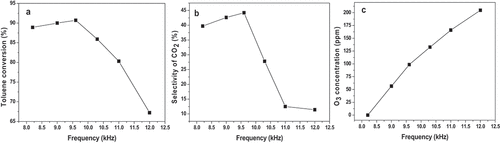
As can be seen from , the C7H8 conversion first rises and then decreases. When the applied frequency is 8.2 kHz, the value of C7H8 conversion reaches 88.9%; when the frequency is increased to 9.6 kHz, the value of C7H8 conversion increases to 90.7%. As the frequency is continuously increased to 12 kHz, the toluene conversion drops to 67.2%. Zhu et al. have reported a similar variation regularity of toluene conversion with applied frequency [Citation23].
The influence of applied frequency on CO2 selectivity is shown in . It can be seen that CO2 selectivity displays a similar variation trend as toluene conversion with the increase of frequency. As the frequency increases, the CO2 selectivity first increases and then decreases. The highest value of CO2 selectivity can be obtained as the frequency is increased to 9.6 kHz. This phenomenon can also be explained by the aforementioned resonance theory.
The influence of frequency on the generation of ozone is shown in . It is clear that with the increase of frequency (8.2 kHz→12 kHz), the O3 concentration gradually increases (0→204.6 ppm). This is due to the fact that the DBD reactor began to discharge with the increasing frequency. It has been generally accepted that the number of electrons can increase as applied frequency is increased. Hence, the probability of collision between the generated electrons and oxygen molecules increases, resulting in more active oxygen groups (O2−), which would be furtherly combined with oxygen to produce more ozone [Citation24].
3.1.4 Effect of feed concentration
The reaction parameters were as follows: [C7H8 flow rate] = 250 mL/min; [discharge voltage] = 11.9KV; [frequency] = 9.6 kHz. The feed concentration of toluene was changed to investigate the effect of toluene concentration on the degradation performance of toluene.
It can be seen from that as the feed concentration increases (70→2000 ppm), the C7H8 conversion decreases slightly, but the value of conversions can be maintained at a high level (around 90%). The result is due to the fact that once discharge parameters are determined, the number of active species produced by gas ionization is also determined. Under these circumstances, the increase in the initial concentration of toluene brings about a higher proportion of toluene molecules in the gas flow, which will inevitably lead to a lower probability of collisions between toluene molecules and the active groups. In contrast, CO2 selectivity changes obviously with the variation of the feed concentration of toluene. When the concentration is 70 ppm, the selectivity of CO2 after toluene conversion can reach 62.7%; the CO2 selectivity continuously decreases with the reduction of feed concentration. A very low CO2 selectivity of 11.6% is obtained when the concentration increases to 2000 ppm. It can be explained that the increasing proportion of toluene in the feed gas means a decrease in the proportion of carrier gas (air), leading to the reduction of the proportion of O2. Therefore, the amount of active oxygens formed by O2 in the gas flow decreases, which results in reducing the degree of toluene oxidation [Citation25]. These results suggest that the toluene concentration has a greater impact on the degree of mineralization. High-concentration toluene leads to the fact that more toluene is not completely converted into CO2, but to other intermediates (benzaldehyde, benzyl alcohol, benzyl formate, benzoic acid, etc.) [Citation26]. When the feed toluene concentration is low, the efficiency of conversion and mineralization of toluene enhances. All the results indicate that the plasma technique is suitable for the removal of low-concentration toluene with a relatively better treatment performance.
3.1.5 Effect of gas flow rate
The residence time of toluene molecules in the discharge area largely determines the possibility of collisions between toluene molecules and high-energy electrons, thereby affecting the degradation performance of toluene. When the volume of the discharge area through which the exhaust gas passes is constant, the gas flow rate determines the residence time of the exhaust gas components. Therefore, to explore the effect of residence time is actually to investigate the influence of the gas flow rate. The reaction parameters were as follows: [C7H8 concentration] = 500 ppm; [discharge voltage] = 11.9kV; [frequency] = 9.6 kHz. The effect of the toluene gas flow rate on the removal efficiency and CO2 selectivity is displayed in .
Making a rise of gas flow rate, the toluene conversion slightly decreases. The influence trend of waste gas flow on CO2 selectivity is basically the same as that of toluene conversion. When gas flow rate varies from 100 mL/min to 1500 mL/min, CO2 selectivity decreases from 47.5% to 12.5%. The results can be attributed to the fact that the growth of gas flow rate reduces the residence time of toluene molecules in the discharge region, leading to each toluene molecule sharing fewer high-energy electrons and active species [Citation26]. In a word, the reactants and intermediates cannot react sufficiently before leaving the discharge zone, giving rise to the reduction in the removal efficiency and CO2 selectivity.
3.1.6 Effect of water vapor content
In order to investigate the effect of the humidity on the performance of plasma for toluene removal, a water vapor generator (FD-PG, Friend Experimental Equipment, China) was used to generate some amount of water vapor, which was introduced into the system. The reaction parameters were controlled as follows: [C7H8 concentration] = 500 ppm; [C7H8 flow rate] = 250 mL/min; [discharge voltage] = 11.9KV; [frequency] = 9.6 kHz. Considering the fact that water vapor content had no obvious effect on toluene conversion efficiency, we directly studied its effect on CO2 selectivity, and the results are illustrated in .
From , it is clear that moisture content has a significant effect on the selectivity of CO2 in plasma degradation of toluene. When the humidity increases appropriately, the CO2 selectivity gradually increases. When the water vapor content of the exhaust gas was increased to about 8.4%, the CO2 selectivity reached the highest value (53.6%), and then the humidity of the exhaust gas continued to increase, and the CO2 selectivity began to decrease. Generally, more OH• radicals can be generated in the process of plasma by adding an appropriate amount of water. OH• groups work as active species, which have more oxidizing power than O•, and can improve toluene conversion [Citation24]. Conversely, if the humidity is too high, too many water molecules will absorb more electrons and reduce the number of high-energy electrons, resulting in the reduction of active species. For this reason, the degree of deep oxidation and mineralization of toluene will be reduced.
3.2 Plasma-catalytic destruction of toluene
3.2.1 Plasma-catalytic performance of toluene removal
On the basis of optimizing the structural parameters of the reactor (discharge length of 12 cm) and electrical parameters (discharge voltage of 11.9kV, frequency of 9.6 kHz), we introduced CM/Z5 catalyst in the post-plasma (at the back of the plasma) system to investigate the plasma-catalyst coupling. The effect of treating low-concentration toluene waste gas (70ppm, 1 L/min) is shown in . As a reference, the stability study for the single plasma treatment of low-concentration toluene was also implemented.
Figure 8. Performances of toluene removal in the plasma alone and plasma-catalysis system (Reaction conditions: Room temperature; Atmosphere pressure; [C7H8 concentration] = 70 ppm, [C7H8 flow rate] = 1 L/min, GHSV = 18,000 h−1).
![Figure 8. Performances of toluene removal in the plasma alone and plasma-catalysis system (Reaction conditions: Room temperature; Atmosphere pressure; [C7H8 concentration] = 70 ppm, [C7H8 flow rate] = 1 L/min, GHSV = 18,000 h−1).](/cms/asset/70a89c37-6813-4168-a81b-f138187131b5/tcsb_a_2065363_f0008_oc.jpg)
It can be seen from that under the optimal process parameters, the initial toluene conversion is about 88% in the single plasma treatment, the selectivity of CO2 is about 41%, and the reaction lasts for 18 hours and the treatment performance remains stable. It may due to the fact that the residence time of toluene is very short in the reactor of plasma alone. Although up to 88% of toluene can be converted, the randomness of collisions between high-energy electrons and pollutant molecules leads to the generation of intermediate products, and its degree of mineralization is low. Introducing the CM/Z5 catalyst at the back end of the plasma, the toluene can be completely converted in the initial stage (within 2 h). This is due to the fact that the catalyst has an adsorption effect and can completely adsorb toluene vapour in a short time. The toluene conversion began to decrease after 2 h and then remained stable (> 94%). In terms of CO2 selectivity, after the introduction of the CM/Z5 catalyst, the CO2 selectivity was significantly improved, from about 41% to about 70%. The above results indicate that the introduction of the catalyst can greatly improve the degradation performance of toluene in NTP system.
shows the variation regularity of the outlet ozone concentration with time after the treatment of toluene exhaust gas in the NTP alone and NTP-catalysis system. When using isolated plasma, the ozone concentration in the exhaust gas exceeds 110 ppm. When the catalyst is introduced, the ozone concentration in the exhaust gas drops to almost zero (meet the ‘Indoor Air Quality Standard’ GB/T 18883–2002), because of an excellent catalytic performance of O3 decomposition (described in Supplementary Material). In summary, the addition of the catalyst not only improves the toluene conversion efficiency and CO2 selectivity of plasma treatment but also solves the problem of secondary pollution caused by ozone generated in the tail gas.
3.2.2 Analysis of performance
Compared to single NTP, the combination of plasma with the CM/Z5 catalyst significantly promoted the toluene removal efficiency and CO2 selectivity. The obvious improvement primarily depends on the properties of the introduced catalyst. It has been reported that the specific surface areas of the catalysts are closely related to the number density of active sites on the catalyst surface for the adsorption of the pollutants and intermediates [Citation27]. CM/Z5 catalyst of large specific surface area of 310.4 m2/g (Table S1) can provide more toluene adsorption sites, which may prolong the residence time of toluene and intermediates and enhance the possibility of collisions between toluene and the chemically reactive species in the plasma-catalytic process [Citation27]. It is well known that ozone is an important oxidizing species formed in NTP and plays a key role in the process of degradation of toluene. When CM/Z5 catalyst was introduced, ozone was quickly consumed and decomposed into O2 and O• [Citation28]. The reaction rate constant of toluene reacting with O• is 4.75 × 108 times that of it reacting with O3, indicating that toluene molecules are more easily oxidized by O• than by O3 [Citation29]. Fortunately, the decomposition of ozone by CM/Z5 catalyst can form more O• in the plasma-catalytic system, which would contribute to toluene degradation efficiency and CO2 selectivity. Based on the above analysis, the NTP system equipped with CM/Z5 catalyst presents a good performance for toluene removal.
4 Conclusion
This work carried out a study on the removal of toluene in the single plasma and plasma-catalysis system, and the following conclusions were drawn:
(1) For a single plasma treatment of toluene, the change in the discharge zone length has almost no effect on the conversion rate of toluene, but the CO2 selectivity increases with the increase in the discharge length;
(2) When the discharge voltage is 11.9kV and the frequency is 9.6 kHz, plasma treatment of toluene can obtain the highest toluene conversion rate (90.7%) and CO2 selectivity (44%), but the concentration of ozone produced in the exhaust gas is relatively high (around 100 ppm);
(3) With the increase in toluene feed concentration and gas flow rate, the selectivity of CO2 in the toluene product of plasma treatment gradually decreases, but the toluene conversion rate does not change significantly;
(4) The proper water vapor content (8.4%) in the toluene waste gas is beneficial to the plasma degradation reaction of toluene and can increase the selectivity of CO2 in the product to 53.6%;
(5) The introduction of CM/Z5 catalyst at the back end of the plasma can significantly improve the performance of toluene removal. The toluene treatment reaction lasts for 18 hours, the toluene conversion rate is maintained above 94%, the CO2 selectivity is stabilized at about 70% and the exhaust gas can be guaranteed – meet ozone emission standards.
The performance of toluene after the coupling catalyst provides a reference for the practical application of the hybrid plasma catalyst technology to treat VOCs.
Availability of data and material
The datasets used or analyzed during the current study are available from the corresponding author on a reasonable request.
Supplemental Material
Download MS Word (418.5 KB)Acknowledgments
This work was supported by the Chenguang Program of Shanghai Education Development Foundation and Shanghai Municipal Education Commission (Project Number 18CGB12).
Disclosure statement
No potential conflict of interest was reported by the author(s).
Supplementary material
Supplemental data for this article can be accessed here
References
- Shen X, Zhao Y, Chen Z, et al. Heterogeneous reactions of volatile organic compounds in the atmosphere. Atmos Environ. 2013;68:297–314.
- Lu HF, Zhou Y, Han WF, et al. High thermal stability of ceria-based mixed oxide catalysts supported on ZrO 2 for toluene combustion. Catal Sci Technol. 2013;3(6):1480–1484.
- Li K, Tang X, Yi H, et al. Low-temperature catalytic oxidation of NO over Mn–Co–Ce–Ox catalyst. Chem Eng J. 2012;192:99–104.
- Qu Z, Gao K, Fu Q, et al. Low-temperature catalytic oxidation of toluene over nanocrystal-like Mn–Co oxides prepared by two-step hydrothermal method. Catal Commun. 2014;52:31–35.
- Einaga H, Teraoka Y, Ogata A. Catalytic oxidation of benzene by ozone over manganese oxides supported on USY zeolite. J Catal. 2013;305:227–237.
- Demeestere K, Dewulf J, Van Langenhove H. Heterogeneous photocatalysis as an advanced oxidation process for the abatement of chlorinated, monocyclic aromatic and sulfurous volatile organic compounds in air: state of the art. Crit Rev Environ Sci Technol. 2007;37(6):489–538.
- Chung WC, Mei DH, Tu X, et al. Removal of VOCs from gas streams via plasma and catalysis. Catalysis Rev. 2019;61(2):270–331.
- Czapka T, Maliszewska I, Olesiak-Bańska J. Influence of atmospheric pressure non-thermal plasma on inactivation of biofilm cells. Plasma Chem Plasma Process. 2018;38(6):1181–1197.
- Jiang N, Lu N, Shang K, et al. Innovative approach for benzene degradation using hybrid surface/packed-bed discharge plasmas. Environ Sci Technol. 2013;47(17):9898–9903.
- Yan X, Zhu T, Fan X, et al. Removal of p-chlorophenol in mist by DC Corona discharge plasma. Chem Eng J. 2014;245:41–46.
- Liang WJ, Lin MA, Jian LI. Removal of toluene by non-thermal plasma coupled with catalyst. J Beijing Uni Technol. 2014;40(2):161–166.
- Huang H, Ye D, Guan X. The simultaneous catalytic removal of VOCs and O3 in a post-plasma. CatalToday. 2008;139(1–2):43–48.
- Fan X, Zhu T, Sun Y, et al. The roles of various plasma species in the plasma and plasma-catalytic removal of low-concentration formaldehyde in air. J Hazard Mater. 2011;196:380–385.
- Li Y, Fan Z, Shi J, et al. Post plasma-catalysis for VOCs degradation over different phase structure MnO2 catalysts. Chem Eng J. 2014;241:251–258.
- Wu J, Huang Y, Xia Q, et al. Decomposition of toluene in a plasma catalysis system with NiO, MnO2, CeO2, Fe2O3, and CuO catalysts. Plasma Chem Plasma Process. 2013;33(6):1073–1082.
- Guo Y, Liao X, He J, et al. Effect of manganese oxide catalyst on the dielectric barrier discharge decomposition of toluene. CatalToday. 2010;153(3–4):176–183.
- Chen HL, Lee HM, Chen SH, et al. Removal of volatile organic compounds by single-stage and two-stage plasma catalysis systems: a review of the performance enhancement mechanisms, current status, and suitable applications. Environ Sci Technol. 2009;43(7):2216–2227.
- Chen J, Xie Z. Removal of H2S in a novel dielectric barrier discharge reactor with photocatalytic electrode and activated carbon fiber. J Hazard Mater. 2013;261:38–43.
- Qin CH, Huang XM, Dang XQ, et al. Toluene removal by sequential adsorption-plasma catalytic process: effects of Ag and Mn impregnation sequence on Ag-Mn/g-Al2O3. Chemosphere. 2016;162:125–130.
- Xu XX, Wang PT, Xu WC, et al. Plasma-catalysis of metal loaded SBA-15 for toluene removal: comparison of continuously introduced and adsorption-discharge plasma system. Chem Eng J. 2016;283:276–284.
- Shahna FG, Bahrami A, Alimohammadi I, et al. Chlorobenzene degradation by non-thermal plasma combined with EG-TiO2/ZnO as a photocatalyst: effect of photocatalyst on CO2 selectivity and byproducts reduction. J Hazard Mater. 2017;324:544–553.
- Li D, Li X, Bai M, et al. CO2 reforming of CH4 by atmospheric pressure glow discharge plasma: a high conversion ability. Int J Hydrogen Energy. 2009;34(1):308–313.
- Zhu T, Li J, Liang WJ, et al. experimental research on toluene decomposition with high frequency DBD. High Voltage Eng. 2009;2:350–363.
- Pan KL, Chen DL, Pan GT, et al. Removal of phenol from gas streams via combined plasma catalysis. J Ind Eng Chem. 2017;52:108–120.
- Chang MB, Lee HM. Abatement of perfluorocarbons with combined plasma catalysis in atmospheric-pressure environment. CatalToday. 2004;89(1–2):109–115.
- Wang BW, Chi CM, Xu M, et al. Plasma-catalytic removal of toluene over CeO2-MnOx catalysts in an atmosphere dielectric barrier discharge. Chem Eng J. 2017;322:679–692.
- Zhu XB, Gao X, Qin R, et al. Plasma-catalytic removal of formaldehyde over Cu–Ce catalysts in a dielectric barrier discharge reactor. Appl Catal B Environ. 2015;170:293–300.
- Xu XX, Wu JL, Xu WC, et al. High-efficiency non-thermal plasma-catalysis of cobalt incorporated mesoporous MCM-41 for toluene removal. CatalToday. 2017;281:527–533.
- Huang R, Lu MJ, Wang PT, et al. Enhancement of the non-thermal plasma-catalytic system with different zeolites for toluene removal. RSC Adv. 2015;5(88):72113–72120.