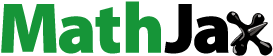
ABSTRACT
The concentrations of six heavy metals were determined in the sediments of the Changli ecological monitoring area, northwest of the Bohai Bay. The spatial distribution of the metals was discussed, and the ecological risk was evaluated using the potential ecological risk index (PERI) and secondary phase enrichment factor (KSPEF). The content ranges of Cu, Pb, Cd, Cr, Ni, and Zn were 1.38–25.78, 11.13–21.96, 0.12–0.54, 15.44–120.60, 0.48–57.11, and 44.21–156.38 mg·kg−1, respectively. Based on the results of the geochemical speciation analysis, the residual phase was the main form for all five metals (Cu, Pb, Cd, Cr, and Zn). Compared to the assessment results of KSPEF, PERI is more suitable for the pollution evaluation of heavy metals. The results indicated that all the sediments pose a moderate to considerable level of risk, except site S14 (low risk), and Cd was found to be the main contributor of the ecological risk due to heavy metals.
1. Introduction
In recent years, with rapid industrial development, the quantity of heavy metals discharged into marine systems, has increased continuously. Heavy metals are highly toxic, degradation-resistant, and are easily absorbed and accumulated in aquatic organisms. Heavy metal contamination in sediments have been a concern because of its persistence and ecological risks [Citation1–3]. The chemical properties of heavy metals in sediments, determined by geochemical speciation, significantly influence their environmental behavior and toxicity [Citation4]. Therefore, the toxicity and ecological risks of heavy metals are measured by geochemical speciation [Citation5].
The potential ecological index [PERI), proposed by Hakanson [Citation6], has been widely used in the evaluation of heavy metal pollution in sediments because of the consideration of metal content and biological toxicity factors [Citation7,Citation8]. However, the metal content was used without considering chemical speciation, resulting in an overestimated risk level. The secondary phase enrichment factor (KSPEF), which is based on geochemical speciation, can better reflect the chemical activity and bioavailability of heavy metals in sediments [Citation9].
The Changli ecological monitoring area, located in the northwest of the Bohai Sea, is a typical offshore area. It is the most active zone of the three interfaces of land, sea, and atmosphere, with unique ecological value and great resource potential [Citation10,Citation11]. The physicochemical parameters of estuarine coastal waters change drastically under the influence of land-sea interactions, making it a typical ecologically sensitive area. With population growth and economic development, many heavy metals are discharged into the coastal waters through river migration and atmospheric deposition. The content and distribution of heavy metals in the sediments of the coastal waters of the Bohai Sea have been reported in many studies [Citation12–16]. However, only a few reports are available in the literature on geochemical speciation and comprehensive ecological risk assessment of the sediments of this area.
The objectives of the present study are as follows: (i) to investigate the pollution status of six heavy metals (Cu, Pb, Cd, Cr, Ni, and Zn) and their spatial distribution; (ii) to analyze the relationship between heavy metal content and physicochemical characteristics of sediments; and (iii) to use PERI and KSPEF methods to evaluate the ecological risks based on the metal contents.
2. Materials and methods
2.1. Sampling
The study area was located in the Changli Gold Coast National Nature Reserve of Hebei Province (39°25 – 39°40 N, 119°11–119°37 E) as shown in the map (). Irregular semi-diurnal tides are the dominant tidal type in the study area. The estuary in Changli named Luanhe, Xinkai, and Dapuhe, respectively. This area has unique economic value and great resources potential because it has the most active areas of interactions between the land, sea and atmosphere. Further, the Changli industry in farm raised aquatic products was worth 629.2 million CNY in 2009, accounting for more than half of that of Hebei Province [Citation17–19].
2.2. Sampling and chemical analysis
In May 2015, a clam sampler was used to collect surface sediment samples (0–10 cm) from 19 different sites (S1–S19) (). Each sediment sample was placed in a polythene wrapper and transported to the laboratory at −20°C for further analysis.
Air-dried sediment samples were used to determine the granularity and organic matter (OM). The granularity of the samples was measured using a laser granularity analyzer (Mastersizer 3000, Malvern, UK). Before analysis, 30% H2O2 and 10% HCl solutions were used to remove organic matter and carbonates from all sediment samples. Granularity can be categorized into three size groups: clay (< 4 μm), silt (4–63 μm), and sand (63–2000 μm) [Citation20,Citation21]. For OM, the air-dried sediment samples were oxidized with potassium bichromate, followed by titration with an aqueous ferrous solution [Citation22]. The results were expressed as a percentage of the total dry mass.
The samples were dried to a constant mass at 60°C, ground in a ball mill, and filtered through a 63 μm mesh sieve. The heavy metal content of the sediments was determined [Citation23,Citation24]. Approximately 0.15 g samples were digested using a mixed solution (HNO3 + 30% H2O2 + HF, v:v:v = 15:7:5) at 180°C for 24 h in an electric heat drum wind drying oven (DHG-9075A, Shanghai Yiheng Scientific Instruments Co., Ltd. China), and 4 mL boric acid saturated solution was added to the digestion system and digested at 180°C for another 12 h. The total contents in a certain volume solution for Pb, Zn, Cd, Cr, Ni, and Cu were determined using a quadrupole inductively coupled plasma mass spectrometry (ICP-MS) (X Series 2, Thermo Fisher Scientific, USA). All metal contents were expressed in milligrams per kilogram of dry mass.
Meanwhile, certified sediment reference material (GSD9 and GBW07309, provided by the National Center for Geological Experiment and Testing, China Geological Survey) was measured using the same method for quality assurance. The obtained recoveries (92–106%) for various heavy metals indicated that the results were reasonable and credible.
The contents of four speciation of metals (i.e. acid-soluble, reducible, oxidizable, and residual fractions) in the sediment samples were measured using the modified Bureau Communautaire de Référence (BCR) sequential extraction procedure [Citation25]. The content of each metal species was determined using ICP-MS, as mentioned above. The recoveries of various heavy metals (i.e. the ratio of the sum of the four fractions to the total content) were 88.87–125.04% for Pb, 87.40–126.61% for Zn, 84.43–124.43% for Cr, 87.40–130.33% for Ni, and 70.28–130.68% for Cu. Moreover, the results of the reagent blanks indicate that there was no contamination during analysis.
2.3. Metal assessment in sediment
2.3.1. Potential ecological risk index
PERI is one of the most extensively used methods for evaluating heavy metal pollution and associated ecological risks [Citation9,Citation26–29]. The PERI was calculated using EquationEquation (1)(1)
(1) .
where and
are the measured contents of metal i in the sediments and their corresponding reference background values (mg·kg−1),
is the biological toxicity factor of metal i (),
is the potential ecological risk factor of metal i. In this study, the reference background values of heavy metals in the sediments of the Bohai Sea were used as described by Zhao and Yan [Citation30], ().
Table 1. The background values and the biological toxicity factors of six metals [Citation6,Citation30,Citation31]
2.3.2. Secondary phase enrichment factor, KSPEF
Ecological risk assessment of heavy metals based on geochemical speciation can better reflect the chemical activity and bioavailability of heavy metals in sediments [Citation9]. To highlight the importance of the metal content in bioavailable fractions (i.e. F1 + F2 + F3), the secondary phase enrichment factor (KSPEF) proposed by Huo et al. Citation32 was used to evaluate sediment pollution [Citation33]. The metal source and the influence of the bioavailability of heavy metals were considered in this method. KSPEF was calculated according to EquationEquation (2)(2)
(2) :
where and
are the sum of the contents of fractions 1–3 (F1 + F2 + F3) and the residual phase (F4) extracted using the modified BCR sequential extraction procedure for a given metal in a sediment sample, respectively;
and
are the sum of the contents of fractions 1–3 and the residual phase for a given element in an uncontaminated reference sediment sample. The
ratio for the metal content in S13 was chosen as the reference value in this study because there was no significant anthropogenic source of toxic metals at this station.
2.4. Statistical analysis
All data for the sediments were determined in triplicate. Pearson’s correlation analysis was used to analyze the relationship between metal-to-metal, metal-to-OM, and metal-to-grain size composition in the sediments. Statistical analyses were performed using SPSS 18.0. Correlations were considered significant at P < 0.05.
3. Results and discussion
3.1. The range and distribution of heavy metals in sediments
The ranges of the contents (mg·kg−1) in sediment samples were 1.38–25.78 for Cu, 11.13–21.96 for Pb, 0.12–0.54 for Cd, 15.44–120.60 for Cr, 0.48–57.11 for Ni, and 44.21–156.38 for Zn, respectively (). As for the average contents (mg·kg−1), Zn had the highest value (85.80 ± 24.64), followed by Cr (69.07 ± 28.00), Ni (23.15 ± 15.07), Pb (17.95 ± 4.03), Cu (16.28 ± 8.99), and Cd (0.29 ± 0.13). According to the classification criteria proposed by the General Administration of Quality Supervision, Inspection, and Quarantine of the People’s Republic of China [Citation34], the metal content is divided into three levels. Compared to the limit values listed in , the Cr contents of all the sites met the standard requirements of Class II but exceeded that of Class I at seven sites (S1, S3, S6, S8, S11, S16, and S17). The contents of Cd at two sites (S9 and S17) and Zn at one site (S19) met the standard requirements of Class II, whereas the contents of Cu and Pb were lower than the limit value of Class I. However, the limit values of Ni were not covered in marine sediment quality [Citation34].
Table 2. General characteristics of sediments collected from the study area
Sediment quality guidelines (SQGs) are often used to assess the adverse biological risks in marine sediments [Citation28,Citation35]. Based on SQGs, the metal contents in sediments delineated by the values of threshold effect level (TEL) and probable effect level (PEL), were divided into three ranges (i.e. rare, occasional, and frequent adverse effects) [Citation36,Citation37]. All the metal contents were below the limit values of PEL; however, the contents of Cr (14 sites) and Ni (13 sites) exceeded the corresponding values of TEL, and Cu contents at approximately half of the sites were beyond the value of TEL (18.7 mg·kg−1) (). The Zn content at two sites (S12 and S19) exceeded 124 mg·kg−1 (TEL). Based on the analysis above, adverse effects on aquatic ecosystems may occur occasionally at the majority of sites, particularly due to Cr, Ni, Cu, and Zn.
Compared to other coastal areas, all the metal contents in the present study were at low to medium levels (). Pb contents were lower than those in Luanhe Estuary, Bohai Bay [Citation15] near the study area.
Table 3. The contents of heavy metals in the surface sediments in marine environments (mg·kg−1)
3.2. Correlations between metal-to-metal, metal-to-OM, and metal-to-grain size composition in sediments
Particle size distribution and OM content are important factors affecting the spatial distribution of metals [Citation42, Citation43]. Grain size analysis () indicated that the sedimentary particles primarily belonged to sand (> 63 μm). The average percentages of sand, silt, and clay were 76.66%, 15.98%, and 5.39%, respectively. With the exception of S5, the contents of silt (53.79%) and clay (26.93%) were higher than that of sand (16.83%). Higher percentages of sand and silt have also been described in other coastal regions around the Bohai Sea, for example, 67% and 20% in the near-shore area, northern Shandong Peninsula [Citation44], and 49.72% and 44.28% in estuarine surface sediments from Dongying City [Citation9].
Table 4. Pearson’s correlation matrix for metals and general characteristics in surface sediments from Changli ecological monitoring area, Bohai Sea
The OM content of the sediments in the area ranged from 0.06% to 2.48% (average 0.75 ± 0.78%), which exhibited the lowest and highest values at S19 and S5, respectively (). According to the classification method in [Citation45], the OM content of all stations was high (less than 5%). Significant negative correlations were observed between OM and sand percentage (r = – 0.624, P < 0.01), whereas OM was significantly positively correlated with both silt (r = 0.637, P < 0.01) and clay (r = 0.751, P < 0.01) (). There was a significant negative correlation between OM and sand percentage (r = − 0.624, P < 0.01), while there was a significant positive correlation between OM and silt (r = 0.637, P < 0.01) and clay (r = 0.751, P < 0.01) (). The highest OM content (2.48%) at S5 corresponded to the lowest clay percentage, indicating finer marine sediments with a higher OM content [Citation9].
It is widely believed that high levels of heavy metals are associated with OM because fine-grained particles provide additional adsorption points with larger specific surface areas in sediments [Citation9,Citation46]. In this study, Cu, Pb, and Ni were positively correlated with OM (P < 0.01). There was a positive or negative correlation between sand, silt, clay, and Ni (P < 0.05). The clay content was positively correlated with Cu (P < 0.05). There was no significant correlation between metals contents with OM, sand, silt, and clay in the Changli ecological monitoring area. The present study implies that special terrestrial pollution imports, maritime activities, and hydrodynamic conditions may be the most important factors depending on their spatial variation [Citation9,Citation47].
As shown in , there were no significant correlations between Zn and other elements (P > 0.05). The significant and positive correlations between Cu, Pb, Cd, Cr, and Ni () demonstrated a common origin (natural or man-made) [Citation47,Citation48] for these metals in the Bohai Sea.
3.3. Geochemical fractionation of heavy metals in the surface sediments
In the present study, speciation analysis of five metals (Cu, Pb, Cr, Ni, and Zn) was conducted because of the lower detection limit of the instrument for different phases of Cd. The results of the modified BCR sequential extraction are shown in . The main forms of all five metals were found to be in the residual fraction (F4), except for Cu at site S18 and Pb at sites S2, S3, and S5. For all sites, the average proportions of the F4 fraction were 62.27% for Cu, 56.36% for Pb, 71.62% for Cr, 69.89% for Ni, and 79.75% for Zn. The high proportion of Zn in the residual fraction suggests that there is a minor potential threat to biota due to its poor availability [Citation49].
Figure 2. % distributions of metal speciation in sediments from different sampling sites. F1: water/acid soluble fraction; F2: reducible fraction; F3: oxidizable fraction; F4: residual fraction.
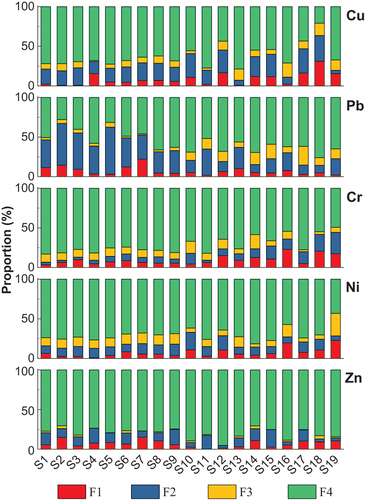
Among the non-residual fractions of metals, the mobility and availability, in descending order, are the acid-soluble, reducible, and oxidizable fractions [Citation50,Citation51]. However, the sum of the three fractions [i.e. F1 + F2 + F3) is considered the mobile fraction reported by Rauret [Citation25]. In this study, the average percentages of mobile metals in sediments, in descending order, were Pb (43.64%], Cu (37.73%), Ni (30.11%), Cr (28.38%), and Zn (20.25%). Higher proportions of the mobile fraction for Pb (30.64–71.98%) could pose a serious threat to aquatic systems. Approximate proportions of Pb in the non-residual fractions were also reported in the sediments of Jiaozhou Bay, China, with a range of 36.45–57.29% [Citation52].
Pb existed mainly in the forms of residue (F4) and bound to Fe-Mn oxides [F2). This was consistent with the results mentioned in Morillo’s and Cuong’s research [Citation53,Citation54], which indicated that Fe and Mn hydrous oxides could effectively scavenge Pb in the sediments.
3.4. The calculation results of two approaches
The results of and PERI based on these methods are listed in . According to the classification method mentioned in , all metals except Cd presented a low risk at each station, with an
value of less than 40. Cd at three sites (S9, S16, and S17] posed a high risk with
values of 176.26, 160.12, and 180.61, respectively. Cd showed moderate or considerable risk at all sites. These results imply that the higher contents of Cd at S9, S16, and S17 contributed to the higher PERI values at the three sites (194.55 for S9, 176.22 for S16, and 199.19 for S17), suggesting considerable ecological risk.
Table 5. Calculated and PERI values of heavy metals in surface sediments from Bohai Sea
Table 6. The evaluating indicator of the potential ecological risk [Citation6]
The secondary phase enrichment factor can reveal more information on identifying the most common contaminants and pollution degree [Citation33]. The KSPEF values of the studied metals are shown in . The results indicated a significant difference in the distribution among the different metals and stations. The maximum and minimum values were observed at sites S18 (for Cu, 14.0) and S12 (for Zn, 0.3), respectively. The average values of KSPEF for metals were in the following order: Cu (no enrichment–severe enrichment) > Cr (no enrichment–moderately severe enrichment) > Ni (no enrichment–moderately severe enrichment) > Zn (no enrichment–minor enrichment) > Pb (no enrichment–moderate enrichment).
3.5. The comparison of the PERI and KSPEF results
Each method has its own limitations. Therefore, it is particularly important to use comprehensive methods to evaluate heavy metal pollution in the sediments in the study area. Overall, the evaluation results based on PERI values were more serious than those based on KSPEF values. Among all stations, S14 posed the lowest potential ecological risk, with the minimum PERI value of 46.20, whereas it presented moderate enrichment because of the KSPEF value of 3.0 Cu. As for the secondary phase enrichment factor, the selection of ratio was difficult. Choosing different reference points yields different results; however, this method can reflect the enrichment of the extractable phase of heavy metals in the sediments in the study area. The content of Cu at S18 was low (4.7 mg·kg−1), while the proportion of mobile phase (F1 + F2 + F3) accounted for 79.2% of the total contents. Therefore, this did not mean that the Cu content could have a harmful effect on the organisms at S18, with a KSPEF value of 14.0 Cu.
Evaluation methods based on the total content and chemical speciation are important for assessing the ecological risk posed by polluted sediments [Citation55,Citation56]. It is necessary to combine the chemical and bioavailability analyses of metals to improve the existing risk assessment methods. PERI considers the different concentrations of heavy metals in sediments, their biotoxicity, and the spatial heterogeneity of background values. This could reflect the biological risk of heavy metals to the environment, but it does not consider the impact of heavy metals on organisms living in sediment or overlying water [Citation57–60]. KSPEF is a risk assessment method based on the morphology of heavy metals, which considers the importance of the fugitive form of heavy metals in terms of bioavailability; however, it does not consider the absolute amounts of heavy metals, and bioavailability is not equivalent to biotoxicity [Citation33,Citation61,Citation62]. Therefore, when evaluating the risk of heavy metal pollution in sediments, it is essential to consider the influence of the elemental content and morphological distribution of heavy metals. A comprehensive consideration of the heavy metal species, total concentration, biotoxicity, bioavailability, and other parameters will enable a more objective and scientific assessment of the environmental risk of heavy metals in sediments.
4. Conclusions
In the study area, the contents of all six metals met the Class I or Class II criteria described in the Chinese Marine Sediment Quality (GB 18668–2002). Adverse effects on aquatic systems may occur occasionally because the contents of Cr, Ni, Cu, and Zn exceed the limit values of the corresponding TELs of the SQGs. The sedimentary particles were mainly sand, and the OM of the sediments was at lower levels. The Cu, Pb, and Ni contents were significantly correlated with OM. The main form of all the five metals was the residual phase (F4). Based on the calculated PERI values, most stations exhibited moderate to considerable risk, except for site S14. As for the results of KSPEF, the extractable phase of Cu in the sediments was the most enriched.
Disclosure statement
No potential conflict of interest was reported by the author(s).
Additional information
Funding
References
- Arisekar U, Shakila RJ, Shalini R, et al. Distribution and ecological risk assessment of heavy metals using geochemical normalization factors in the aquatic sediments. Chemosphere. 2022;294:133708.
- Ma T, Sheng Y, Meng Y, et al. Multistage remediation of heavy metal contaminated river sediments in a mining region based on particle size. Chemosphere. 2019;225:83–92.
- Zhuang M, Sanganyado E, Li P, et al. Distribution of microbial communities in metal-contaminated nearshore sediment from Eastern Guangdong, China. Environ Pollut. 2019;250:482–492.
- Stamatis N, Kamidis N, Pigada P, et al. Quality indicators and possible ecological risks of heavy metals in the sediments of three semi-closed East Mediterranean Gulfs. Toxics. 2019;7(2):30.
- Cai S, Zhou S, Cheng J, et al. Heavy metals speciation and distribution of microbial communities in sediments from the abandoned Mo-Ni polymetallic mines, southwest of China. Environ Sci Pollut Res. 2022;29:1–15.
- Hakanson L. An ecological risk index for aquatic pollution control. A sedimentological approach. Water Res. 1980;14(8):975–1001.
- Ntakirutimana T, Du G, Guo JS, et al. Pollution and potential ecological risk assessment of heavy metals in a lake. Polish J Environ Stud. 2013;22(4):1129–1134.
- Zhang Z, Lu Y, Li H, et al. Assessment of heavy metal contamination, distribution and source identification in the sediments from the Zijiang River, China. SciTotal Environ. 2018;645:235–243.
- Liu Q, Wang F, Meng F, et al. Assessment of metal contamination in estuarine surface sediments from Dongying City, China: use of a modified ecological risk index. Mar Pollut Bull. 2018;126:293–303.
- Manbohi A, Mehdinia A, Rahnama R, et al. Microplastic pollution in inshore and offshore surface waters of the southern Caspian Sea. Chemosphere. 2021;281:130896.
- Werner BT. Complexity in natural landform patterns. Science. 1999;284(5411):102–104.
- Ding X, Ye S, Yuan H, et al. Spatial distribution and ecological risk assessment of heavy metals in coastal surface sediments in the Hebei Province offshore area, Bohai Sea, China. Mar Pollut Bull. 2018;131:655–661.
- Lin M, Zhang Y, Xue C, et al. Distribution pattern of heavy metals in the surface sediments of the area of circum-Bohai Bay and ecological environment assessment. Mar Geol Quat Geol. 2013;33(6):41–46. (in Chinese).
- Liu H, Du D, Ma Z, et al. Distribution pattern and contamination assessment of heavy metals in surface sediments of Beidaihe near-shore area. Mar Geol Front. 2015;31(7):47–51. (in Chinese).
- Liu J, Yin P, Chen B, et al. Distribution and contamination assessment of heavy metals in surface sediments of the Luanhe River Estuary, northwest of the Bohai Sea. Mar Pollut Bull. 2016;109(1):633–639.
- Zhang L, Qin Y, Zheng B, et al. Distribution and pollution assessment of heavy metals in sediments from typical areas in the Bohai Sea. Acta Scientiae Circumstantiae. 2011;31(8):1676–1684. (in Chinese).
- Li H, Gao X, Gu Y, et al. Comprehensive large-scale investigation and assessment of trace metal in the coastal sediments of Bohai Sea. Mar Pollut Bull. 2018;129(1):126–134.
- Su Y, Liu J, Han X, et al. The effects of mariculture on the marine environment [J]. Jiangsu Agric Sci. 2012;40(3):306–309. (in Chinese).
- Tian Y, Jiang Y, Liu Q, et al. Using a water quality index to assess the water quality of the upper and middle streams of the Luanhe River, northern China. SciTotal Environ. 2019;667:142–151.
- Kiani-Harchegani M, Sadeghi SH, Asadi H. Comparing grain size distribution of sediment and original soil under raindrop detachment and raindrop-induced and flow transport mechanism. Hydrol Sci J. 2018;63(2):312–323.
- Wentworth CK. A scale of grade and class terms for clastic sediments. J Geol. 1922;30(5):377–392.
- Onjefu SA, Shaningwa F, Lusilao J, et al. Assessment of heavy metals pollution in sediment at the Omaruru River basin in Erongo region, Namibia. Environ Pollutants Bioavailability. 2020;32(1):187–193.
- Ryss IG. The chemistry of fluorine and its compounds. State Publishing House for Scientific; 1956. Technical, and Chemical Literature, Moscow (English translation U.S. Atomic Energy Commission translation Series AEC-tr-3927).
- Wu S, Zhao YH, Feng X, et al. Application of inductively coupled plasma mass spectrometry for total metal determination in silicon-containing solid samples using the microwave-assisted nitric acid–hydrofluoric acid–hydrogen peroxide–boric acid digestion system. J Anal At Spectrom. 1996;11(4):287–296.
- Rauret G, Lopez-Sanchez JF, Sahuquillo A, et al. Improvement of the BCR three step sequential extraction procedure prior to the certification of new sediment and soil reference materials. J Environ Monit. 1999;1(1):57–61.
- Liu S, Pan G, Zhang Y, et al. Risk assessment of soil heavy metals associated with land use variations in the riparian zones of a typical urban river gradient. Ecotoxicol Environ Saf. 2019;181:435–444.
- Ranjani M, Veerasingam S, Venkatachalapathy R, et al. Assessment of potential ecological risk of microplastics in the coastal sediments of India: a meta-analysis. Mar Pollut Bull. 2021;163:111969.
- Tian K, Wu Q, Liu P, et al. Ecological risk assessment of heavy metals in sediments and water from the coastal areas of the Bohai Sea and the Yellow Sea. Environ Int. 2020;136:105512.
- Yang Q, Hu G, Yu R, et al. Distribution, fractionation, and contamination assessment of heavy metals in offshore surface sediments from western Xiamen Bay, China. Acta Geochim. 2016;35(4):355–367.
- Zhao Y, Yan M. Geochemistry of sediments of the China shelf sea. Beijing, China: Science Press; 1994. (In Chinese).
- Xu Z, Ni S, Tuo X, et al. Calculation of heavy metals’ toxicity coefficient in the evaluation of potel ecological risk index. Environ Sci Technol. 2008;31(2):112–115. (In Chinese).
- Hou W, Fengru H, Jingsheng C, Zhenbang J. Comparative study of assessment method for river particulate heavy metal pollution. Scientia geographica sinica (Chinese). 1997;17(1):81–86. http://geoscien.neigae.ac.cn/EN/10.13249/j.cnki.sgs.1997.01.81.
- Sakan S, Popović A, Anđelković I, et al. Aquatic sediments pollution estimate using the metal fractionation, secondary phase enrichment factor calculation, and used statistical methods. Environ Geochem Health. 2016;38(3):855–867.
- AQSIQ (General Administration of Quality Supervision, Inspection and Quarantine of the People’s Republic of China). Marine sediment quality (GB18668-2002). Beijing (China): Standards Press of China; 2002. (In Chinese).
- Fakhradini SS, Moore F, Keshavarzi B, et al. Polycyclic aromatic hydrocarbons (PAHs) in water and sediment of Hoor Al-Azim wetland, Iran: a focus on source apportionment, environmental risk assessment, and sediment-water partitioning. Environ Monit Assess. 2019;191. DOI:10.1007/s10661-019-7360-0.
- Long ER, Macdonald DD, Smith SL, et al. Incidence of adverse biological effects within ranges of chemical concentrations in marine and estuarine sediments. Environ Manage. 1995;19(1):81–97.
- MacDonald DD, Carr RS, Calder FD, et al. Development and evaluation of sediment quality guidelines for Florida coastal waters. Ecotoxicology. 1996;5(4):253–278.
- Neyestani MR, Bastami KD, Esmaeilzadeh M, et al. Geochemical speciation and ecological risk assessment of selected metals in the surface sediments of the northern Persian Gulf. Mar Pollut Bull. 2016;109(1):603–611.
- El Zrelli R, Courjault-Radé P, Rabaoui L, et al. Heavy metal contamination and ecological risk assessment in the surface sediments of the coastal area surrounding the industrial complex of Gabes city, Gulf of Gabes, SE Tunisia. Mar Pollut Bull. 2015;101(2):922–929.
- Parra S, Bravo MA, Quiroz W, et al. Distribution and pollution assessment of trace elements in marine sediments in the Quintero Bay (Chile). Mar Pollut Bull. 2015;99(1–2):256–263.
- Sindern S, Tremöhlen M, Dsikowitzky L, et al. Heavy metals in river and coast sediments of the Jakarta Bay region (Indonesia)—geogenic versus anthropogenic sources. Mar Pollut Bull. 2016;110(2):624–633.
- Zhu Z, Xue J, Deng Y, et al. Trace metal contamination in surface sediments of intertidal zone from Qinhuangdao, China, revealed by geochemical and magnetic approaches: distribution, sources, and health risk assessment. Mar Pollut Bull. 2016;105(1):422–429.
- Wang Z, Lin K, Liu X. Distribution and pollution risk assessment of heavy metals in the surface sediment of the intertidal zones of the Yellow River Estuary, China. Mar Pollut Bull. 2022;174:113286.
- Xu G, Pei S, Liu J, et al. Surface sediment properties and heavy metal pollution assessment in the near-shore area, north Shandong Peninsula. Mar Pollut Bull. 2015;95(1):395–401.
- Marin V, Moreno M, Vassallo P, et al. Development of a multistep indicator-based approach (MIBA) for the assessment of environmental quality of harbours. ICES J Mar Sci. 2008;65(8):1436–1441.
- Fan W, Xu Z, Wang WX. Metal pollution in a contaminated bay: relationship between metal geochemical fractionation in sediments and accumulation in a polychaete. Environ Pollut. 2014;191:50–57.
- Agah H, Bastami KD, Fumani NS. Ecological risk, source and preliminary assessment of metals in the surface sediments of Chabahar Bay, Oman Sea. Mar Pollut Bull. 2016;107(1):383–388.
- Bastami KD, Taheri M, Bagheri H, et al. Response of sediment-dwelling annelida community in relation to geochemical parameters in the Gorgan Bay, Caspian Sea. Int J Environ Sci Technol. 2014;11(7):2025–2036.
- Davutluoglu OI, Seckın G, Kalat DG, et al. Speciation and ımplications of heavy metal content in surface sediments of Akyatan Lagoon–Turkey. Desalination. 2010;260(1–3):199–210.
- Liu X, Sheng Y, Liu Q, et al. Dissolved oxygen drives the environmental behavior of heavy metals in coastal sediments. Environ Monit Assess. 2022;194(4):1–12.
- Zimmerman AJ, Weindorf DC. Heavy metal and trace metal analysis in soil by sequential extraction: a review of procedures. Int J Anal Chem. 2010;2010:1–8.
- Lin Y, Meng F, Du Y, et al. Distribution, speciation, and ecological risk assessment of heavy metals in surface sediments of Jiaozhou Bay, China. Hum Ecol Risk Assess. 2016;22(5):1253–1267.
- Morillo J, Usero J, Gracia I. Heavy metal distribution in marine sediments from the southwest coast of Spain. Chemosphere. 2004;55(3):431–442.
- Cuong DT, Obbard JP. Metal speciation in coastal marine sediments from Singapore using a modified BCR-sequential extraction procedure. Appl Geochem. 2006;21(8):1335–1346.
- Amiard JC, Geffard A, Amiard-Triquet C, et al. Relationship between the lability of sediment-bound metals (Cd, Cu, Zn) and their bioaccumulation in benthic invertebrates. Estuar Coast Shelf Sci. 2007;72(3):511–521.
- Xie Q, Li H, Li Z, et al. Accumulation, chemical speciation and ecological risks of heavy metals on expanded polystyrene microplastics in seawater. Gondwana Res. 2022. In press. DOI:10.1016/j.gr.2022.01.017.
- Chen Y, Jiang X, Wang Y, et al. Spatial characteristics of heavy metal pollution and the potential ecological risk of a typical mining area: a case study in China. Process SafEnviron Prot. 2018;113:204–219.
- Feng Y, Bao Q, Yunpeng C, et al. Stochastic potential ecological risk model for heavy metal contamination in sediment. Ecol Indic. 2019;102:246–251.
- Soliman NF, Nasr SM, Okbah MA. Potential ecological risk of heavy metals in sediments from the Mediterranean coast, Egypt. J Environ Health Sci Eng. 2015;13(1):1–12.
- Williams JA, Antoine J. Evaluation of the elemental pollution status of Jamaican surface sediments using enrichment factor, geoaccumulation index, ecological risk and potential ecological risk index. Mar Pollut Bull. 2020;157:111288.
- Abrahim GMS, Parker RJ. Assessment of heavy metal enrichment factors and the degree of contamination in marine sediments from Tamaki Estuary, Auckland, New Zealand. Environ Monit Assess. 2008;136(1):227–238.
- He Z, Li F, Dominech S, et al. Heavy metals of surface sediments in the Changjiang (Yangtze River) Estuary: distribution, speciation and environmental risks. J Geochem Explor. 2019;198:18–28.