ABSTRACT
Copper-based nanopesticides hold significant promise for agricultural applications. However, a critical challenge that persists is the need for effective control over the release of Cu2+. To this end, this study systematically investigated the Cu2+ release characteristics of Cu-based NPs with varying morphologies, species, and coating agents. It is found that CuO NPs exhibited extremely low Cu2+ concentration, rendering them ineffective for plant disease control. Cu(OH)2 nanowires cannot continuously release Cu2+ due to its larger size and enhanced stability. Conversely, Cu(OH)2 NPs exhibited sustained Cu2+ release, albeit at a slower rate. Modification with surfactants could significantly affect the Cu2+ release behavior, particularly the coating of carboxymethyl starch, which notably promoted Cu2+ release from Cu(OH)2 NPs, achieving sustained release for 27 days. Furthermore, carboxymethyl starch-coated Cu(OH)2 particles could consistently release Cu2+ in the pH range of 5–7. This finding may offer the inspiration of long-lasting control of plant diseases.
1 Introduction
In recent years, the application of nanotechnology in agriculture has garnered significant attention, particularly in the development of nanopesticides [Citation1,Citation2]. Nanopesticides are pesticides made using nanomaterials, which offer higher activity and efficacy compared to traditional pesticides. The small size and unique surface properties of NPs enable better adsorption on plant surfaces, enhancing adhesion and persistence [Citation3]. Additionally, nanopesticides play an active role in crop nutrient management by improving the utilization and absorption of nutrients through nano-sized soil applications [Citation4]. This allows for controlled fertilizer release, reducing pollution and waste while improving crop yield and quality.
However, the development and application of nanopesticides still face challenges. Among them, Cu-based NPs have shown excellent antimicrobial and cost-effective properties [Citation5,Citation6]. Cu(OH)2 and CuO NPs (NPs) are the primary components of these pesticides [Citation7–9]. These small particles could gradually release Cu2+ which is a broad-spectrum antibacterial antibiotic [Citation10]. Therefore, Cu-based NPs has been commonly used to protect crops from bacteria induced diseases [Citation11]. Compared with traditional Cu2+ pesticide, Cu-based NPs require lower concentrations while exhibiting higher bactericidal activity, resulting in more efficient disease control [Citation12]. Cu-based NPs also demonstrate superior adhesion and penetration properties, allowing for better attachment to crop surfaces and entry into plant tissues [Citation3]. Furthermore, the release of Cu2+ is relative slow, providing long-term protection for corps.
The control of Cu2+ release from Cu-based NPs remains a challenge. On the one hand, the Cu2+ concentration cannot be too low as its lowest bactericidal concentration is 63.5 μg/L [Citation13]. On the other hand, the high Cu2+ concentration could also result in toxicity to plants as Cu2+ could replace Mg2+ in chlorophyll [Citation14]. Therefore, the concentration of Cu2+ should be controlled in an appropriate concentration range. From the cost and benefit perspective, controlling the Cu2+ releasing at an appropriate rate could reduce the number of pesticide sprays and thus reduce the labor costs. Thus, control of Cu2+ release from Cu-based NPs is of great significance. Nevertheless, the study of Cu2+ release from Cu-based nanopesticides is still scarce [Citation15,Citation16].
Modification of Cu-based NPs with organic surfactant may be a possible solution for Cu2+ release control. The control of active constituent release has been commonly studied in the field of drug delivery [Citation17]. For example, encapsulating the active ingredient in polymers or liposomes and controlling the pore structure on nanoparticle surfaces allows for gradual release, prolonging its action time [Citation18,Citation19]. Learning from this pathway, chemical modification of metal oxide nanopesticides through covalent bonding with a polymer or other compound was adopted to can control release rates [Citation20–22]. This method enables adjustable release rates and durations based on specific needs, while remaining controllable and cost-effective. Whereas, chemical modification methods often require the use of capping agents which may pose ecological risks [Citation23]. In addition, environmental factors such as temperature, pH might affect the Cu2+ release in their practical application. Given that, the critical environment factor such as pH should be taken into consideration when modification of Cu-based NPs [Citation24]. Nevertheless, research on the Cu2+ release control from Cu-based NPs nanopesticides remains limited, making it crucial to explore environmentally friendly release strategies for Cu-based NPs.
Herein, this study aims to systematically investigate the Cu2+ release from Cu-based NPs. To achieve the impact of particle component, morphology, and the species of modification surfactants, seven Cu-based NPs, i.e. CuO NPs, Cu(OH)2 NPs, Cu(OH)2 nanowires (NWs), and rhamnolipid (Rha), gelatin (Gel), carboxymethyl starch (CMS), and PEG (PEG) coated Cu(OH)2 NPs, were prepared by sintering method and coordination precipitation method. Their Cu2+ release behavior in 27 days were quantitatively monitored to screen for long-lasting and effective Cu-based NPs. Additionally, the influence of pH on Cu2+ release was also investigated, considering real application processes. This work may contribute novel insights for the green and controlled release of Cu-based NPs.
2 Materials and methods
2.1 Reagents and materials
High-purity anhydrous copper sulfate, ammonia (NH4OH, GR, 25–28%), sodium hydroxide (NaOH, AR, ≥96.0%), oxalic acid (AR, ≥99.8%), and ethanol (AR, ≥95%) were procured from Beijing Sinopharm Chemical Reagent Co. Ltd. Rhamnolipid (95%) was bought from Sigma-Aldrich Co. Ltd (Germany). Gelatin (from pigskin), sodium starch glycolate (AR) and PEG (AR) were sourced from J&K Scientific Co. Ltd. Ultra-pure water (18.2 MΩ) produced with a Milli-Q Gradient system (Millipore, Bedford, U.S.A.) was used throughout.
2.2 Synthesis of Cu-based NPs
Preparation of Cu(OH)2 NPs and Cu(OH)2 NWs. Cu-based NPs were prepared through coordination precipitation method based on previous study with moderate modification [Citation25]. The coordination precipitation method entailed adding ammonia to a CuSO4 solution to form intermediate Cu2(OH)22+ species, which further reacted with excess NH4OH to yield Cu2(NH3)42+ complexes. The gradual addition of NaOH then facilitated the release of Cu2+ from these complexes, leading to the formation of Cu(OH)2 NPs. The morphology of these NPs, influenced by the nucleation and growth rates within the liquid-phase reaction, was modulated by varying the concentrations and addition rates of NH4OH and NaOH. In this study, the morphology of Cu(OH)2 NPs was tailored by controlling the NaOH addition rate. The Cu(OH)2 NPs was synthesized by first mixing 30 mL of 3 mol/L ammonia solution into 0.05 mol/L CuSO4 solution, under continuous magnetic stirring for 20 minutes to yield flocculent copper precursors. Subsequently, 10 mL of 0.5 mol/L NaOH solution was introduced into the mixture at a rate of 10 mL/min, followed by an additional 20 min of stirring. The resulting solution was washed three times with pure water until a neutral pH was achieved, centrifuged at 4500 rpm, and the precipitate was dried in a blast oven to obtain the Cu(OH)2 NPs. The synthesis process of Cu(OH)2 NWs is identical to that of Cu(OH)2 NPs, except adjust the NaOH addition rate to 2 min/L.
Preparation of surfactant coated Cu(OH)2 NPs. The flocculent copper precursors solution was obtained through above described method. Then, surfactants including Rha, Gel, CMS, and PEG, were added into above solutions with final concentration of 1 mM. After incubation for 1 hours under stirring, 10 mL of 0.5 mol/L NaOH solution was added at a rate of 2 mL/min. Post-stirring, washing, centrifuging, and drying steps were identical to the Cu(OH)2 NPs preparation.
Preparation of CuO NPs. The precipitation roasting method used for creating CuO NPs involved reacting oxalic acid with Cu2+ ions to form copper oxalate precipitates, which were then converted into CuO NPs through high-temperature sintering. An ethanol/water (1:1, v:v) solution served as the solvent for preparing 0.10 mol/L solutions of CuSO4 solution and oxalic acid. The oxalic acid solution was added dropwise to the CuSO4 solution under magnetic stirring for 20 min. The precipitate obtained was copper oxalate, which was centrifuged, washed with ethanol 3–5 times, vacuum dried at 60°C for 3 h, and roasted at a ramp of 10°C/min to 270°C, held for 2 hours to yield black CuO NP powder.
2.3 Monitoring of Cu2+ release from Cu-based NPs
A 250 mg sample of the synthesized Cu-based NP powder was dispersed in 500 mL of deionized water and shaken at 150 rpm. Samples with volume of 10 mL were taken out at 6 h, 1, 2, 3, 6, 9, 14, 20, and 27 days. The samples were filtered using a 50 mL ultrafiltration tube (Millipore, Amicon Ultra), centrifuged at 7000 rpm for 15 min. The filtrate had 200 µL of 65% nitric acid solution added dropwise, mixed thoroughly, and analyzed for Cu2+ concentration using inductively coupled plasma mass spectrometry (ICP-MS). To evaluate the influence of pH on Cu2+ release, the pH of the water was adjusted to approximately 5, 6, and 7 using diluted NaOH and HNO3 solutions, and the aforementioned steps were replicated. To ensure the accuracy of Cu analysis, experimental blank group, which used pure water to substitute the Cu-based NP suspension was set, and Cu did not to be detected, indicating interference would not be introduced in this procedure.
2.4 Characterization
Particle morphology, composition, and surface functional groups were characterized using different analytical instruments. A Quanta FEG 250 scanning electron microscope (SEM) equipped with energy-dispersive X-ray spectroscopy (EDS) was utilized to examine particle morphology. The sample preparation involved dispersing a small quantity of Cu-based NPs in deionized water, ultrasonic dispersion, and then placing 25 µL of this dispersion onto a clean silicon wafer, which was subsequently vacuum dried for SEM analysis. Fourier transform infrared spectroscopy (FTIR, Thermo-Nicolet Nexus 6700) was employed to identify surface functional groups. The concentration of Cu2+ was quantified using an Agilent ICP-MS 8900.
3 Results and discussions
3.1 Preparation and characterization of Cu-based NPs
The experimental results are illustrated in , indicating that a rapid addition of NaOH solution (0.5 M, 10 mL/min) resulted in Cu(OH)2 NPs with a lamellar granular morphology, due to the orthorhombic crystal system of Cu(OH)2 favoring such a structure. These particles ranged in size from 47.0 to 232.7 nm and exhibited relative uniformity. Conversely, when the NaOH addition rate was reduced to 10 mL/min, the resulting Cu(OH)2 NWs with morphologies of rod-like or wire structures, with lengths spanning 174.5 to 1595.2 nm and diameters between 26.7 and 81.2 nm. The CuO NPs also adopted a lamellar morphology but with more defined edges and a broader size range of 100.4 to 690.2 nm, compared to the Cu(OH)2 NPs.
Figure 1. Characterization of copper pesticide NPs. SEM images of Cu(OH)2 NPs (a), Cu(OH)2 NWs (b), CuO NPs (c), rha-coated Cu(OH)2 NPs (d), gel-coated Cu(OH)2 NPs (e), CMS-coated Cu(OH)2 NPs (f), and PEG-coated Cu(OH)2 NPs (g), respectively.
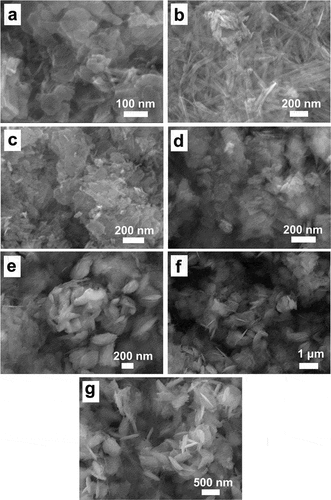
The role of surfactants in nanoparticle preparation, particularly in stabilizing particles and altering their morphology, prompted the use of Rha, Gel, CMS, and PEG in this study. The synthesis of surfactant-capped Cu(OH)2 particles followed a similar procedure to that of the Cu(OH)2 NPs, with the addition of surfactants after the formation of the flocculent Cu2(NH3)4SO4 precipitate, followed by thorough mixing and subsequent NaOH addition to induce precipitation. The findings, depicted in Figures d-g, reveal that Rha-coated Cu(OH)2 NPs maintained a lamellar structure akin to Cu(OH)2 NPs but were significantly larger, ranging from 51.7 to 628.4 nm. This increase in size may be attributed to the tighter binding of the surfactant to the particle surfaces, which elevates the nucleation potential energy, reduces the number of crystalline nuclei, and consequently enlarges the particle size. Gel and CMS-coated Cu(OH)2 NPs exhibited similar spike-like morphologies, with sizes of 138.2 to 409.8 nm and 418.6 to 1269.4 nm, respectively. When utilizing PEG as a surfactant, the Cu(OH)2 NPs predominantly appeared as thick flakes, sized between 181.0 and 979.0 nm, accompanied by occasional fine needle-like structures possibly arising from localized high concentrations during NaOH addition.
In addition, the particles were characterized using X-ray scattering spectroscopy and FTIR spectroscopy to analyze the presence of surfactants on their surfaces. The EDS results, presented in , indicated strong carbon signals for all surfactant-coated particles, which, when correlated with SEM imagery and Cu elemental distribution maps, suggested the presence of surfactants on the particle surfaces. Further characterization was conducted using FTIR spectroscopy, with findings shown in . All particles, except for the CuO NPs, exhibited pronounced peaks at 3572 cm−1 and 3314 cm−1, corresponding to the stretching vibrations of free O-H groups and hydrogen-bonded O-H groups in Cu(OH)2 particles, respectively. The surfactant-coated Cu(OH)2 particles, including those coated with Rha, Gel, and CMS, displayed broad peaks between 2500 and 3500 cm−1, aligning with the characteristic peaks of carboxylate O-H stretching vibrations. Notably, these peaks partially overlapped with the free O-H stretching vibration peaks of uncoated Cu(OH)2 particles. However, the O-H stretching vibration peaks of the surfactant-coated particles were more pronounced and sharper compared to those without carboxyl groups, such as PEG, indicating that the surfactants were successfully attached to the Cu(OH)2 particles using the modification method. Additionally, post-modification, the Cu(OH)2 particles exhibited a distinct and sharp peak at 1641 cm−1, likely due to the C=O stretching vibration and O-H bending vibration under hydrogen bonding. Therefore, the combined SEM and FTIR characterization results confirm the successful modification of Cu(OH)2 particles with different surfactants.
3.2 Monitoring of Cu2+ release from different Cu-based NPs
The sterilization effect of copper nanopesticides is primarily achieved through the release of Cu2+ ions, which are crucial for controlling plant diseases. Consequently, monitoring the release of Cu2+ is a vital aspect of evaluating nano-copper pesticides. According to existing research, the minimum bactericidal concentration of Cu2+ is 63.5 μg/L [Citation13], making it necessary for the released Cu2+ concentration to exceed this threshold. However, excessive Cu2+ can displace Mg2+ from chlorophyll, disrupting plant photosynthesis and causing phytotoxicity; thus, the Cu2+ concentration must be carefully controlled. Ideally, Cu2+ should be released in low doses and at a sustained rate to ensure bactericidal efficiency while extending its effectiveness for long-term disease control.
In this study, various Cu-based NPs (500 mg/L) were added to water, sampled at different time points, and separated via ultrafiltration to determine the concentration of free Cu2+ ions and obtain their release kinetic curves. The results, depicted in , showed that the Cu2+ concentration released from CuO NPs was approximately 25 μg/L, falling short of the bactericide requirement. The concentration of Cu2+ from Cu(OH)2 NWs was ~125 μg/L, but remained relatively constant over time, which may be attributed to the larger, more stable nature of the wire structure, hindering the release of Cu2+. The initial Cu2+ concentration in the Cu(OH)2 NPs group was approximately 108.6 μg/L, gradually increasing over time to reach 214.9 μg/L by day 27. Thus, granular Cu(OH)2 particles appear to be more suitable for use as nanopesticides due to their favorable release profile.
Figure 4. Cu2+ release kinetic profiles of different Cu-based NPs. (a) Cu2+ release profiles of Cu(OH)2 NPs, Cu(OH)2 NWs, and CuO NPs; (b) Cu2+ release profiles of rha, gel, CMS, and PEG-coated Cu(OH)2 NPs.
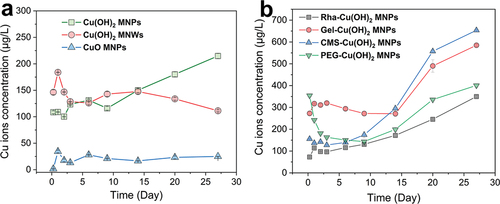
The Cu2+ release kinetics of Cu(OH)2 NPs modified with four different coating agents are presented in . The Rha-coated Cu(OH)2 NPs exhibited a steady and gradual increase in Cu2+ concentration, reaching 349.9 μg/L by day 27. In contrast, the Gel-coated Cu(OH)2 particles maintained a stable Cu2+ level for the first 15 days, suggesting minimal release during this period. Afterward, the concentration surged to 584.9 μg/L, which could be attributed to the initial strong encapsulation by Gel that prevented early release, followed by eventual dissolution and delayed release of Cu2+. The CMS-coated Cu(OH)2 NPs showed a continuous increase in Cu2+ concentration, with a modest rise during the first 3 days and then a consistent upward trend, culminating in 653.7 μg/L on day 27. The pattern of Cu2+ concentration change in PEG-coated Cu(OH)2 NPs group was notably distinct, characterized by an initial decrease, stabilization, and subsequent increase. While there is no definitive theory to explain this behavior, it is hypothesized that fine needle-like Cu(OH)2 NPs dissolved quickly upon water addition as particles with smaller sizes are more likely to release counterpart ions due to its high surface energy [Citation26]. This results in an initial high Cu2+ concentration of 354.6 μg/L. Subsequently, these ions may have been adsorbed by larger Cu(OH)2 particles, causing a reduction in free Cu2+ levels and establishing an equilibrium. Over time, the dissolution of PEG from the surfaces of the larger particles could have facilitated a gradual increase in Cu2+ concentration. Among the seven Cu-based NPs assessed, the CMS-coated Cu(OH)2 NPs demonstrated the highest initial Cu2+ concentration and sustained release capability, making them the most suitable candidate for copper nanopesticides.
3.3 Monitoring of Cu2+ release under different pH conditions
Given that the pH of the solvent for Cu(OH)2 NPs or rainwater can vary between 5.0 and 7.0 during practical applications, the study investigated the Cu2+ release kinetics of each Cu-based NPs across this pH range. The results, depicted in , showed that for all groups except the CuO NPs, the Cu2+ concentration at pH 5 was slightly higher than at pH 6 and pH 7, which both exhibited similar trends and concentrations. Notably, the Cu2+ concentration at the sixth hour was higher at lower pH values, likely due to smaller Cu(OH)2 particles being more sensitive to pH and dissolving more rapidly within the initial 6 h. In the Cu(OH)2 NWs, Rha-coated Cu(OH)2 NPs, CMS-coated Cu(OH)2 NPs, and PEG-coated Cu(OH)2 NPs groups, there was an observed decrease in Cu2+ concentration initially, presumably because larger particles adsorbed the released Cu2+, as previously discussed. Upon comparative analysis of the seven copper-based NPs, the carboxymethyl starch-coated Cu(OH)2 particles were found to release Cu2+ continuously, with a release concentration meeting sterilization requirements and demonstrating minimal pH sensitivity, thus offering broader applicability.
Figure 5. Cu2+ release monitoring of Cu-based NPs at different pH values. Characterization of copper pesticide NPs. Cu2+ release kinetic curves of Cu(OH)2 NPs (a), Cu(OH)2 NWs (b), CuO NPs (c), rha-coated Cu(OH)2 NPs (d), gel-coated Cu(OH)2 NPs (e), CMS-coated Cu(OH)2 NPs (f), and PEG-coated Cu(OH)2 NPs (g), respectively.
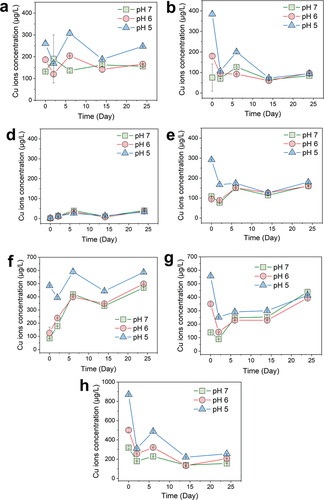
4 Conclusions
In this study, we conducted a comprehensive investigation into the release kinetics of Cu2+ from Cu-based NPs with varying shapes, compositions, and surfactant coatings. We also examined the impact of environmental pH levels on Cu2+ release. The findings revealed that CuO NPs exhibited minimal Cu2+ solubility in water, rendering them ineffective for plant disease control. The Cu(OH)2 NWs were found to be large and stable, but they struggled to release Cu2+ in a sustained manner. In contrast, granular Cu(OH)2 NPs were capable of a sustainable release, albeit at a slower rate. The application of surfactants notably influenced the release behavior of Cu2+. Among the surfactants tested, CMS coating significantly enhanced the release of Cu2+ from Cu(OH)2 NPs, sustaining the release over a period of 27 days. The release kinetics across different pH values was investigated, it was observed that CMS-coated Cu(OH)2 NPs could maintain a consistent release of Cu2+ and fulfill the bactericidal concentration requirements under real-world environmental pH conditions (pH 5–7). This suggests that such particles have the potential to provide long-term protection of plants from bacterial induced diseases, highlighting their applicability for agricultural use.
Acknowledgements
This work was kindly supported by the Yunnan (China) Province Enterprise Basic Research Application Basic Research Joint Project (202101BC070001-026, 202101BC070001-011), National Natural Science Foundation of China (22206181 and 22106028), and Scientific Research Funding of National Institute of Metrology of China (AKYCX2202).
Disclosure statement
No potential conflict of interest was reported by the author(s).
Additional information
Funding
References
- Chhipa H. Nanofertilizers and nanopesticides for agriculture. Environ Chem Lett. 2016;15(1):15–85. doi: 10.1007/s10311-016-0600-4
- Kookana RS, Boxall ABA, Reeves PT, et al. Nanopesticides: guiding principles for regulatory evaluation of environmental risks. J Agric Food Chem. 2014;62(19):4227–4240. doi: 10.1021/jf500232f
- Ding Y, Wang Q, Zhu G, et al. Application and perspectives of nanopesticides in agriculture. J Nanopart Res. 2023;25(8):159. doi: 10.1007/s11051-023-05811-5
- Prasad R, Bhattacharyya A, Nguyen QD. Nanotechnology in sustainable agriculture: recent developments, challenges, and perspectives. Front Microbiol. 2017;8:1014. doi: 10.3389/fmicb.2017.01014
- Bakshi M, Kumar A. Copper-based nanoparticles in the soil-plant environment: assessing their applications, interactions, fate and toxicity. Chemosphere. 2021;281:130940. doi: 10.1016/j.chemosphere.2021.130940
- Wang D, Saleh NB, Byro A, et al. Nano-enabled pesticides for sustainable agriculture and global food security. Nat Nanotech. 2022;17(4):347–360. doi: 10.1038/s41565-022-01082-8
- Tegenaw A, Tolaymat T, Al-Abed S, et al. Characterization and potential environmental implications of select cu-based fungicides and bactericides employed in U.S. markets. Environ Sci Technol. 2015;49(3):1294–1302. doi: 10.1021/es504326n
- Zhao S, Su X, Wang Y, et al. Copper oxide nanoparticles inhibited denitrifying enzymes and electron transport system activities to influence soil denitrification and N2O emission. Chemosphere. 2020;245:125394. doi: 10.1016/j.chemosphere.2019.125394
- Gomes DG, Sanada K, Pieretti JC, et al. Nanoencapsulation boosts the copper-induced defense responses of a susceptible coffea arabica cultivar against hemileia vastatrix. Antibiotics. 2023;12(2):249. doi: 10.3390/antibiotics12020249
- Portelinha J, Duay SS, Yu SI, et al. Antimicrobial peptides and copper(II) ions: novel therapeutic opportunities. Chem Rev. 2021;121(4):2648–2712. doi: 10.1021/acs.chemrev.0c00921
- Truong HT, Nguyen LCT, Quang Le L. Synthesis and antifungal activity of copper nanoparticles against fusarium oxysporum pathogen of plants. Mater Res Express. 2023;10(6):065001. doi: 10.1088/2053-1591/acdb34
- Kah M, Kookana RS, Gogos A, et al. A critical evaluation of nanopesticides and nanofertilizers against their conventional analogues. Nat Nanotechnol. 2018;13(8):677–684. doi: 10.1038/s41565-018-0131-1
- Gan Y, Wu Y, Pang S, et al. Comparison of antibacterial properties of silver ions,copper ions and zinc ions on common candida. J Xinxiang Medical University. 2023;40(1):24–28.
- Küpper H, Setlik I, Setliková E, et al. Copper-induced inhibition of photosynthesis: limiting steps of in vivo copper chlorophyll formation in scenedesmus quadricauda. Functional Plant Biol. 2003;30(12):1187–1196. doi: 10.1071/FP03129
- Liu W, Qin Y, Liu S, et al. Synthesis and antifungal activity of slow-release pyridinylcarbonyl chitooligosaccharide copper complexes. Carbohydr Polym. 2022;291:119663. doi: 10.1016/j.carbpol.2022.119663
- Vencalek BE, Laughton SN, Spielman-Sun E, et al. In situ measurement of CuO and Cu(OH)2 nanoparticle dissolution rates in quiescent freshwater mesocosms. Environ Sci Technol Lett. 2016;3(10):375–380. doi: 10.1021/acs.estlett.6b00252
- Mura S, Nicolas J, Couvreur P. Stimuli-responsive nanocarriers for drug delivery. Nature Mater. 2013;12(11):991–1003. doi: 10.1038/nmat3776
- Makadia HK, Siegel SJ. Poly Lactic-co-Glycolic Acid (PLGA) as biodegradable controlled drug delivery carrier. Polymers. 2011;3(3):1377–1397. doi: 10.3390/polym3031377
- Wu GH, Milkhailovsky A, Khant HA, et al. Remotely triggered liposome release by near-infrared light absorption via hollow gold nanoshells. J Am Chem Soc. 2008;130(26):8175–8177. doi: 10.1021/ja802656d
- Zhou J, Liu G, Guo Z, et al. Stimuli-responsive pesticide carriers based on porous nanomaterials: a review. Chem Eng J. 2023;455:140167. doi: 10.1016/j.cej.2022.140167
- Ma X, Zhu X, Mu Y, et al. Fabrication of polydopamine reduced CuO nanoparticle–alginate composite nanogels for management of pseudomonas synringae pv. tabaci in tobacco. Pest Manag Sci. 2022;79(3):1213–1224. doi: 10.1002/ps.7298
- Zhu X, Ma X, Gao C, et al. Fabrication of CuO nanoparticles composite ε-polylysine-alginate nanogel for high-efficiency management of alternaria alternate. Int J Biol macromol. 2022;223:1208–1222. doi: 10.1016/j.ijbiomac.2022.11.072
- Hering I, Eilebrecht E, Parnham MJ, et al. Evaluation of potential environmental toxicity of polymeric nanomaterials and surfactants. Environ Toxicol Pharmacol. 2020;76:103353. doi: 10.1016/j.etap.2020.103353
- Hortin JM, Anderson AJ, Britt DW, et al. Copper oxide nanoparticle dissolution at alkaline pH is controlled by dissolved organic matter: influence of soil-derived organic matter, wheat, bacteria, and nanoparticle coating. Environ Sci Nano. 2020;7(9):2618–2631. doi: 10.1039/D0EN00574F
- Luna IZ, Hilary LN, Chowdhury AMS, et al. Preparation and characterization of copper oxide nanoparticles synthesized via chemical precipitation method. OALib. 2015;2(3):1–8. doi: 10.4236/oalib.1101409
- Zhang W, Yao Y, Sullivan N, et al. Modeling the primary size effects of citrate-coated silver nanoparticles on their ion release kinetics. Environ Sci Technol. 2011;45(10):4422–4428. doi: 10.1021/es104205a