Abstract
The significance of dental materials in dentin-pulp complex tissue engineering is undeniable. The mechanical properties and bioactivity of mineral trioxide aggregate (MTA) make it a promising biomaterial for future stem cell-based endodontic therapies. There are numerous in vitro studies suggesting the low cytotoxicity of MTA towards various types of cells. Moreover, it has been shown that MTA can enhance mesenchymal stem cells' (MSCs) osteo/odontogenic ability. According to the preferred reporting items for systematic reviews and meta-analyses (PRISMA), a literature review was conducted in the Medline, PubMed, and Scopus databases. Among the identified records, the cytotoxicity and osteo/odontoblastic potential of MTA or its extract on stem cells were investigated. Previous studies have discovered the differentiation-inducing potential of MTA on MSCs, providing a background for dentin-pulp complex cell therapies using the MTA, however, animal trials are needed before moving into clinical trials. In conclusion, MTA can be a promising candidate dental biomaterial for futuristic stem cell-based endodontic therapies.
1. Introduction
Based on laboratory and clinical studies, mineral trioxide aggregate (MTA) overcame the drawbacks of many conventional substances, and it has been considered as a suitable surrogate in the clinical practice [Citation1–3]. Chemical assessments have shown that in contrast to Portland Cement, MTA contains smaller pieces and fewer harmful heavy metals [Citation4–7]. Hence, it has received much attention as an ideal dental material for various endodontic uses, including pulp capping, pulpotomy, root perforation treatment, and root canal filling [Citation1,Citation8,Citation9]. Several investigators have evaluated the physical properties of MTA, and although physical characteristics can be influenced by methods of placement and environmental conditions, MTA exhibits favorable sealing ability, solubility and compressive strength [Citation10–12]. MTA setting is initiated in the existence of moisture. In the course of hardening, a release of calcium hydroxide and a rise in pH could be detected. Bismuth was reported as another crucial component in this mixture whose solubility increases in acidic conditions, similar to inflammatory environments, and accordingly the precipitation rate of calcium hydroxide in hydrated MTA increases [Citation2,Citation7,Citation13]. Among various MTA types, several studies focused on gray (GMTA) and white (WMTA) forms of this material. The results revealed lower level of hydroxyapatite formation within hydrated WMTA. On the other hand, more silica, calcium, and phosphorus were present in WMTA [Citation13–15].
When in direct contact with phosphate-buffered saline (PBS), a layer resembling the hydroxyapatite structure was produced over MTA-filled root canal walls [Citation16]. Numerous in vivo and in vitro investigations confirmed good durability and sealing ability of the said layer. Thus, it could be concluded that MTA can develop an appropriate barrier as a filling material in endodontic procedures [Citation3,Citation6,Citation8,Citation16].
Contradictory results on the antimicrobial nature of MTA could be attributed to the type of MTA, the preparation method, and the species of examined microorganism. Some studies indicated that the antibacterial and antifungal effects of MTA were concentration and time-dependent [Citation17–19]. Although some authors believed that it provides a broad-spectrum toxicity against bacteria and fungi, others reported limited antifungal effect by GMTA and no antibacterial impact on strict anaerobes [Citation18,Citation20].
The purpose of this review is to determine what is currently known about the in vitro effects of MTA on MSCs, including its cytotoxicity, differentiation-inducing potential, and the signaling pathways through which these effects are mediated. We begin the review with an overview of the current state of knowledge of cytotoxicity of MTA towards MSCs derived from different sources. We continue with an overview of in vitro approaches recruited to establish MTA-conditioned media. We then present a summary of studies that have examined MTA’s effects on the expression of osteo/odontoblastic gene markers, mineralization potential of MSCs, and conclude with ideas for future research.
2. Material and methods
2.1. Inclusion criteria and search strategy
In July 2020 a literature review was conducted in the Medline, PubMed, and Scopus databases following the preferred reporting items for systematic reviews and meta-analyses (PRISMA) guidelines. The search keywords were: ‘mineral trioxide aggregate’, ‘stem cell’, and ‘oste/odontoblast differentiation’. Using the Boolean operators ‘AND/OR’ along with the addition of synonyms for the keywords, the final search strategy was: ((‘mineral trioxide aggregate’ OR ‘MTA’) AND (‘stem cell’) AND (‘differentiation’ OR ‘osteogenic’ OR ‘odontogenic’). The inclusion criteria were publications in English within the past 12 years (January 2008 to July 2020), investigating cytotoxicity of MTA and its effects on osteo/odontoblastic potential of MSCs. We excluded review articles, case reports, narratives, and online early published articles. Studies investigating the differentiation-inducing potential of MTA mixture with other root-filling materials, and studies that used solvents other than what was provided by the company were also excluded ().
2.2. Data extraction and outcomes
Three independent reviewers performed the literature search, title, and abstract scrutinization, and systemic full-text evaluation. Disagreements were resolved by discussion to reach a definitive consensus. Data from each study were extracted by reviewers using an abstraction form, and the following details were collected: Authors, publication year, study type, cell source, MTA preparation protocol, treatment period, differentiation and cytotoxicity assays, and outcomes.
3. Results
3.1. Cytotoxicity of MTA towards MSCs
When freshly prepared, due to its alkalinity, the cytotoxicity of MTA mixture is high [Citation15,Citation21]. However, Sarkar et al. in 2005 provided another important characteristic about MTA using EDAX to prove that the formed structure was similar to hydroxyapatite crystals, a major mineral element of dentin and enamel, and from these data, they concluded that MTA shows a noncytotoxic nature [Citation16]. A review by Camilleri and Piit Ford in 2006 highlighted greater noncytotoxic nature of MTA compared to traditional substances [Citation22]. Earlier studies focused on various cell types such as gingival and periodontal ligament derived fibroblasts, human osteosarcoma, odontoblasts, dental pulp cells, and bone marrow cells in direct contact with MTA or its extract[Citation23–27]. Recent studies have turned their attention to and concentrated on dentinogenesis and the differentiation-inducing potential of MTA on MSCs (). It has been shown that MTA is a nontoxic dental material for bone marrow-derived MSCs [Citation28–32,Citation52]. On the other hand, different MTA-based materials had toxic effects on tooth-resident stem cells in a time and concentration-dependent manner [Citation33,Citation34,Citation36,Citation38,Citation39]. The time allowed for establishing an MTA-conditioned medium is another crucial factor in these studies. In particular, since alkalinity decreases over time, longer extraction time may result in lower cytotoxicity [Citation13,Citation34,Citation36,Citation38–40].
Table 1. Cytotoxicity of MTA towards MSCs.
3.2. Differentiation-inducing potential of MTA
While cell and molecular signalings involved in the migration of dental pulp progenitor cells during natural reparative dentinogenesis are not fully understood, recent studies focused on strategies mimicking these natural phenomena [Citation53–57]. Similar to well-known hematopoietic stem cell transplantation procedure, it has faced many difficulties in developing regenerative approaches. These challenges include choosing the best cell source, means of differentiation and lineage potency, and the plasticity of the stem cells [Citation58,Citation59]. Well documented results of pulp capping with MTA suggested that it can provide a surface for adhesion of progenitor cells. Moreover, its low-level cytotoxicity, paracrine effects, and potential to induce expression of osteo/odontoblast gene markers in adult tissue-derived stem cells make MTA an applicable chemical for stem cell-based therapies in dentin-pulp regeneration [Citation60–62].
To elucidate the differentiation promoting potential of MTA, previous researchers recruited three main approaches. Treating cells with freshly prepared MTA-conditioned medium was suggested by Hakki et al. [Citation24]. According to this procedure, it was suggested that the bioactive ingredients of MTA are released after incubating the dried MTA in appropriate media for 7 days to produce MTA-conditioned media. The supernatant could be diluted with fresh media to establish different concentrations. Alternatively, cells can be cocultured with MTA discs of diverse sizes, placed in transwell inserts [Citation63]. The third method is to culture the cells in direct contact with MTA. Through this method, one can evaluate the role of MTA in cytodifferentiation, cell migration and adhesion. This shows how this method can be considered to be more comparable to in vivo conditions than other methods [Citation64,Citation65].
Evidence from transwell migration assay suggests that MTA can enhance the adhesion and migration of tooth-resident and bone marrow derived MSCs in a concentration-dependent manner [Citation29,Citation45,Citation66]. Additionally, under various conditions, osteo/odontogenic differentiation-inducing potentials of MTA have been reported (). Based on these studies, MTA can stimulate or enhance the expression of genes involved in upstream and downstream signaling pathways leading to mineralization and production of collagenous and non-collagenous proteins in extracellular matrix. This was confirmed by real-time PCR, Western blotting, and microscopic examinations.
Table 2. Differentiation-inducing potential of MTA.
In particular, osteo/odontogenic markers such as Osteocalcin (OCN), Osteopontin (OPN), Alkaline phosphatase (ALP), Bone sialoprotein (BSP), and Collagen I (COLI) were upregulated in direct contact with MTA or its eluent. The most crucial point to note is that several studies reported increased expression of Runt-related transcription factor 2 (RUNX2), Osterix (OSX), and DSPP. The transcription factor RUNX2 functions as the master regulator of mineralization-related genes at the early stages of mineralized tissue development directly or through RUNX2-related signaling pathways [Citation74]. Consequently, downstream regulators such as OSX continue to be expressed at later stages and stimulate differentiation into osteo/odontoblast-like cells and expression of bone or tooth-related genes, specially DSPP and OCN, which are involved in the nucleation phase of dentin calcification and late stages of bone development, respectively [Citation75–77].
To provide deeper insights into how MTA can promote osteo/odontoblast-like phenotype, signaling pathways that are responsible for hard tissue formation were evaluated in . Of the three parallel mechanisms of mitogen-activated protein kinases (MAPKs), i.e. P38, extracellular signal-regulated kinase (ERK1/2), and Jun N-terminal kinase (JNK1/2/3), ERK and P38 have been proved to have a definite role in osteo/odontoblast differentiation. Both routes induce RUNX2 phosphorylation, which increases its transcriptional activity [Citation78–82]. Moreover, P38 has another phosphorylation target, Distal-less homeobox 5 (DLX5), which in phosphorylated form, stimulates OSX expression [Citation83–85]. However, the role of JNK in the modulation of hard tissue development is contradictory. On one hand, it is reported that triggered JNK might induce phosphorylation on inhibitory sites of RUNX2, which decreases its transcriptional potential. Contrastingly, it is reported that the JNK pathway can activate osteo/odontogenesis by reducing SMAD6 binding to BMP receptor 1, and making the receptor available for SMAD1 binding [Citation86–88].
Figure 2. Involvement of major signaling pathways in osteo/odontogenesis effects of MTA on MSCs. IKK: IκB kinase, IκBα masks the nuclear localization signal (NLS) of NF-κB and inhibits its function, AP-1: Activator protein 1, question mark (?) means that the mechanism of action is unknown.
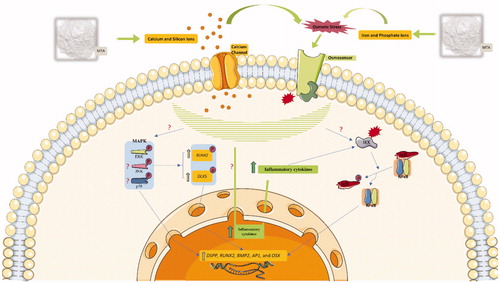
Several studies reported that calcium and silicon ions in the MTA-conditioned medium favor ERK and P38 activation by establishing osmotic stress and inward current of these ionic products through calcium channels. This is represented by the reduced osteo/odontogenic-inducing potential of MTA after application of calcium channel blocker and ERK/P38 inhibitors [Citation73,Citation89–91]. On the contrary, results from a few studies were not consistent with the stimulatory influence of MTA on the P38 pathway and suggested that MTA predominantly promotes osteo/odontogenesis through ERK alone or with JNK [Citation32,Citation38]. Furthermore, few studies indicated that the nuclear factor kappa B (NFκB) signaling pathway is intricately involved in osteo/odontogenesis acquired by treatment with MTA. Data from immunofluorescence and Western blot assays confirm that NFκB can be activated after MTA treatment. This might be attributable to the over expression of inflammatory cytokines in response to MTA treatment [Citation41,Citation43,Citation73,Citation92].
was drawn by modifying SMART Servier Medical Art illustrations (http://smart.servier.com/), provided by Les Laboratoires Servier, licensed under a Creative Common Attribution 3.0 Unported License.
4. Discussion
Numerous in/ex vivo and in vitro studies have illustrated extensive applications of MTA in endodontic treatments, specifically including revitalization procedure and stem cell-based dentin-pulp complex therapies. In this review, by organizing the literatures on the modulation of osteo/odontoblast-like differentiation of MSCs through introducing MTA, we came to this inevitable conclusion that various means of MTA treatment can promote mineralization and expression/overexpression of osteo/odontogenic markers in MSCs. However, future studies should continue to minimize experimental shortcomings. For example, they should focus on developing quantitative methodologies and performing experiments with a larger sample size to confirm these conclusions.
In vitro evaluations revealed the involvement of major signaling pathways in the effects of MTA on osteo/odontogenesis of MSCs. Unfortunately, these results had some limitations, including limited number of studies and inconsistency of experimental designs. Different types of cell sources and means of MTA treatment have been employed, making it difficult to determine a fundamental mechanism of action. It is worth mentioning that several studies reported cross-talk among different levels of ERK/P38 routes of MAPK and NFκB pathways. Moreover, the activity of these pathways can vary in different organs and cells [Citation93–95]. Accordingly, it is hard to tell whether MTA can trigger simultaneous activation of these routes separately, or it plays a significant role in the stimulation of one of them, which can lead to cross-activation of the other pathways. Future studies should consider these cross-talks and the activity of signaling pathways in different MSCs. Once determined, according to the short- and long-lasting effects of MTA on the specific type of MSCs, a particular protocol including the appropriate MSC’s source and concentration of MTA, can be developed for dentin-pulp complex regeneration when a dental material is involved.
Since the dentin-pulp complex structure could be significantly affected in extensive endodontic lesions, the presence of dental materials in the futuristic stem cell-based endodontic therapies is inevitable. In conclusion MTA could be an appropriate option for this purpose in that not only it exhibits noncytotoxic properties, but also it can induce or upregulate the differentiation of MSCs towards osteo/odontoblast-like cells. However, further studies are necessary to establish to what extent MTA contributes to the regulation of MSCs differentiation.
Acknowledgments
The authors thank Mr. Anthony Iovene (University of New Haven) for comments on the manuscript.
Disclosure statement
No potential conflict of interest was reported by the author(s).
References
- Apaydin ES, Shabahang S, Torabinejad M. Hard-tissue healing after application of fresh or set mta as root-end-filling material. J Endod. 2004;30(1):21–24.
- Chng HK, Islam I, Yap AUJ, et al. Properties of a new root-end filling material. J Endod. 2005;31(9):665–668.
- Asgary S. Mineral trioxide aggregate and evidence-based practice. In: Josette Camilleri (Ed.). Mineral trioxide aggregate in dentistry. Berlin, Heidelberg: Springer Berlin Heidelberg; 2014. p. 173–199.
- Chang S-W, Yoo H-M, Park DS, et al. Ingredients and cytotoxicity of MTA and 3 kinds of Portland cements. J Korean Acad Conserv Dent. 2008;33(4):369.
- Siboni F, Taddei P, Prati C, et al. Properties of NeoMTA Plus and MTA Plus cements for endodontics. Int Endod J. 2017;50:e83–e94.
- Gandolfi MG, Taddei P, Tinti A, et al. Apatite-forming ability (bioactivity) of ProRoot MTA. Int Endod J. 2010;43(10):917–929.
- Ber BS, Hatton JF, Stewart GP. Chemical modification of ProRoot MTA to improve handling characteristics and decrease setting time. J Endod. 2007;33(10):1231–1234.
- Braga Xavier C, Weismann R, Gerhardt de Oliveira M, et al. Root-end filling materials: apical microleakage and marginal adaptation. J. Endod. 2005;31(7):539–542.
- Camilleri J, Gandolfi MG, Siboni F, et al. Dynamic sealing ability of MTA root canal sealer. Int Endod J. 2011;44(1):9–20.
- Islam I, Kheng Chng H, Jin Yap AU. Comparison of the Physical and Mechanical Properties of MTA and Portland Cement. J Endod. 2006;32(3):193–197.
- Gurgel-Filho ED, Leite FM, Lima J. B d, et al. Comparative evaluation of push-out bond strength of a MTA-based root canal sealer. Braz J Oral Sci. 2014;13(2):114–117.
- Flores-Ledesma A, Barceló Santana F, Bucio L, et al. Bioactive materials improve some physical properties of a MTA-like cement. Mater Sci Eng C Mater Biol Appl. 2017;71:150–155.
- Li Q, Coleman NJ. The hydration chemistry of ProRoot MTA. Dent Mater J. 2015;34(4):458–465.
- Ha WN, Shakibaie F, Kahler B, et al. Deconvolution of the particle size distribution of ProRoot MTA and MTA Angelus. Acta Biomater Odontol Scand. 2016;2(1):7–11.
- Chang S-W. Chemical characteristics of mineral trioxide aggregate and its hydration reaction. Restor Dent Endod. 2012;37(4):188–193.
- Sarkar NK, Caicedo R, Ritwik P, et al. Physicochemical basis of the biologic properties of mineral trioxide aggregate. J Endod. 2005;31(2):97–100.
- Lovato KF, Sedgley CM. Antibacterial activity of endosequence root repair material and ProRoot MTA against clinical isolates of enterococcus faecalis. J. Endod. 2011;37(11):1542–1546.
- Jafari F, Jafari S, Samadi Kafil H, et al. Antifungal activity of two root canal sealers against different strains of candida. Iran Endod J. 2017;12(1):98–102.
- Alsalleeh F, Chung N, Stephenson L. Antifungal activity of endosequence root repair material and mineral trioxide aggregate. J Endod. 2014;40(11):1815–1819.
- Ribeiro CS, Scelza MFZ, Hirata Júnior R, et al. The antimicrobial activity of gray-colored mineral trioxide aggregate (GMTA) and white-colored MTA (WMTA) under aerobic and anaerobic conditions. Oral Surg Oral Med Oral Pathol Oral Radiol Endod. 2010;109(6):e109–e112.
- AlAnezi AZ, Zhu Q, Wang Y-H, et al. Effect of selected accelerants on setting time and biocompatibility of mineral trioxide aggregate (MTA). Oral Surg Oral Med Oral Pathol Oral Radiol Endod. 2011;111(1):122–127.
- Camilleri J, Pitt Ford TR. Mineral trioxide aggregate: a review of the constituents and biological properties of the material. Int Endod J. 2006;39(10):747–754.
- Ding S-J, Kao C-T, Chen C-L, et al. Evaluation of human osteosarcoma cell line genotoxicity effects of mineral trixoide aggregate and calcium silicate cements. J Endod. 2010;36(7):1158–1162.
- Hakki SS, Bozkurt SB, Hakki EE, et al. Effects of mineral trioxide aggregate on cell survival, gene expression associated with mineralized tissues, and biomineralization of cementoblasts. J Endod. 2009;35(4):513–519.
- Chen C-L, Huang T-H, Ding S-J, et al. Comparison of calcium and silicate cement and mineral trioxide aggregate biologic effects and bone markers expression in MG63 cells. J Endod. 2009;35(5):682–685.
- Willershausen I, Wolf T, Kasaj A, et al. Influence of a bioceramic root end material and mineral trioxide aggregates on fibroblasts and osteoblasts. Arch. Oral Biol. 2013;58(9):1232–1237.
- Yan P, Yuan Z, Jiang H, et al. Effect of bioaggregate on differentiation of human periodontal ligament fibroblasts. Int Endod J. 2010;43(12):1116–1121.
- Costa F, Sousa Gomes P, Fernandes MH. Osteogenic and angiogenic response to calcium silicate-based endodontic sealers. J Endod. 2016;42(1):113–119.
- D’Antò V, et al. Effect of mineral trioxide aggregate on mesenchymal stem cells. J Endod. 2010;36:1839–1843.
- Hengameh A, Reyhaneh D, Nima MM, et al. Effects of two bioactive materials on survival and osteoblastic differentiation of human mesenchymal stem cells. J Conserv Dent. 2014;17(4):349–353.
- Margunato S, Taşlı PN, Aydın S, et al. In vitro evaluation of ProRoot MTA, biodentine, and MM-MTA on human alveolar bone marrow stem cells in terms of biocompatibility and mineralization. J Endod. 2015;41(10):1646–1652.
- Wang Y, Li J, Song W, et al. Mineral trioxide aggregate upregulates odonto/osteogenic capacity of bone marrow stromal cells from craniofacial bones via JNK and ERK MAPK signalling pathways. Cell Prolif. 2014;47(3):241–248.
- Natu VP, Dubey N, Loke GCL, et al. Bioactivity, physical and chemical properties of MTA mixed with propylene glycol. J Appl Oral Sci. 2015;23(4):405–411.
- Kulan P, Karabiyik O, Kose GT, et al. Biocompatibility of accelerated mineral trioxide aggregate on stem cells derived from human dental pulp. J Endod. 2016;42(2):276–279.
- Widbiller M, Lindner SR, Buchalla W, et al. Three-dimensional culture of dental pulp stem cells in direct contact to tricalcium silicate cements. Clin Oral Investig. 2016;20(2):237–246.
- Niu L-n, Watson D, Thames K, et al. Effects of a discoloration-resistant calcium aluminosilicate cement on the viability and proliferation of undifferentiated human dental pulp stem cells. Sci Rep. 2015;5:17177.
- Bortoluzzi EA, Niu L-N, Palani CD, et al. Cytotoxicity and osteogenic potential of silicate calcium cements as potential protective materials for pulpal revascularization. Dent Mater. 2015;31(12):1510–1522.
- Zhao X, He W, Song Z, et al. Mineral trioxide aggregate promotes odontoblastic differentiation via mitogen-activated protein kinase pathway in human dental pulp stem cells. Mol Biol Rep. 2012;39(1):215–220.
- Jaberiansari Z, Naderi S, Tabatabaei FS. Cytotoxic effects of various mineral trioxide aggregate formulations, calcium-enriched mixture and a new cement on human pulp stem cells. Iran Endod J. 2014;9:271–276.
- Agrafioti A, Taraslia V, Chrepa V, et al. Interaction of dental pulp stem cells with Biodentine and MTA after exposure to different environments. J Appl Oral Sci. 2016;24(5):481–486.
- Wang Y, Yan M, Fan Z, et al. Mineral trioxide aggregate enhances the odonto/osteogenic capacity of stem cells from inflammatory dental pulps via NF-κB pathway. Oral Dis. 2014;20(7):650–658.
- Peters OA, Galicia J, Arias A, et al. Effects of two calcium silicate cements on cell viability, angiogenic growth factor release and related gene expression in stem cells from the apical papilla. Int Endod J. 2016;49(12):1132–1140.
- Yan M, Wu J, Yu Y, et al. Mineral trioxide aggregate promotes the odonto/osteogenic differentiation and dentinogenesis of stem cells from apical papilla via nuclear factor Kappa B signaling pathway. J Endod. 2014;40(5):640–647.
- Saberi EA, Karkehabadi H, Mollashahi NF. Cytotoxicity of various endodontic materials on stem cells of human apical papilla. Iran. Endod. J. 2016;11:17–22.
- Schneider R, Rex Holland G, Chiego D, et al. White mineral trioxide aggregate induces migration and proliferation of stem cells from the apical papilla. J. Endod. 2014;40(7):931–936.
- Rodríguez-Lozano FJ, García-Bernal D, Oñate-Sánchez RE, et al. Evaluation of cytocompatibility of calcium silicate-based endodontic sealers and their effects on the biological responses of mesenchymal dental stem cells. Int Endod J. 2017;50(1):67–76.
- Collado-González M, García-Bernal D, Oñate-Sánchez RE, et al. Biocompatibility of three new calcium silicate-based endodontic sealers on human periodontal ligament stem cells. Int Endod J. 2017;50(9):875–884.
- Collado-González M, García-Bernal D, Oñate-Sánchez RE, et al. Cytotoxicity and bioactivity of various pulpotomy materials on stem cells from human exfoliated primary teeth. Int Endod J. 2017;50:e19–e30.
- Chen I, Salhab I, Setzer FC, et al. A new calcium silicate-based bioceramic material promotes human osteo- and odontogenic stem cell proliferation and survival via the extracellular signal-regulated kinase signaling pathway. J Endod. 2016;42(3):480–486.
- Guven EP, Yalvac ME, Sahin F, et al. Effect of Dental Materials Calcium hydroxide-containing cement, mineral trioxide aggregate, and enamel matrix derivative on proliferation and differentiation of human tooth germ stem cells. J Endod. 2011;37(5):650–656.
- Lee B-N, Lee K-N, Koh J-T, et al. Effects of 3 endodontic bioactive cements on osteogenic differentiation in mesenchymal stem cells. J. Endod. 2014;40(8):1217–1222.
- Babaki D, Matin MM. Odontoblast-like cytodifferentiation of dental stem cells: a review. Iran Endod J. 2020;15:79–89.
- Babb RC, Chandrasekaran D, Zaugg LK, et al. A mouse model to study reparative dentinogenesis. Methods Mol Biol. 2019;1922:111–119.
- Vidovic-Zdrilic I, Vijaykumar A, Mina M. Activation of αSMA expressing perivascular cells during reactionary dentinogenesis. Int Endod J. 2019;52(1):68–76.
- Li X, Pedano MS, Camargo B, et al. Experimental tricalcium silicate cement induces reparative dentinogenesis. Dent Mater. 2018;34(9):1410–1423.
- Tran X, Salehi H, Truong M, et al. Reparative mineralized tissue characterization after direct pulp capping with calcium-silicate-based cements. Materials (Basel). 2019;12(13):2102.
- Njeh A, et al. Reactionary and reparative dentin formation after pulp capping: Hydrogel vs. Dycal. Evidence-Based Endod. 2016;1:3.
- Trounson A, McDonald C. Stem cell therapies in clinical trials: progress and challenges. Cell Stem Cell. 2015;17(1):11–22.
- Trounson A, DeWitt ND. Pluripotent stem cells progressing to the clinic. Nat Rev Mol Cell Biol. 2016;17(3):194–200.
- Giraud T, Jeanneau C, Rombouts C, et al. Pulp capping materials modulate the balance between inflammation and regeneration. Dent Mater. 2019;35(1):24–35.
- Mehrvarzfar P, Abbott PV, Mashhadiabbas F, et al. Clinical and histological responses of human dental pulp to MTA and combined MTA/treated dentin matrix in partial pulpotomy. Aust Endod J. 2018;44(1):46–53.
- Dubey N, Min K, Rosa V. Dental stem cells for pulp regeneration. In: B. Zavan, E. Bressan (Eds.). Dental stem cells for pulp regeneration. Cham: Humana Press; 2016. p. 147–163.
- Yasuda Y, Ogawa M, Arakawa T, et al. The effect of mineral trioxide aggregate on the mineralization ability of rat dental pulp cells: an in vitro study. J Endod. 2008;34(9):1057–1060.
- Koh ET, McDonald F, Pitt Ford TR, et al. Cellular response to mineral trioxide aggregate. J Endod. 1998;24(8):543–547.
- Paranjpe A, Smoot T, Zhang H, et al. Direct contact with mineral trioxide aggregate activates and differentiates human dental pulp cells. J Endod. 2011;37(12):1691–1695.
- Araújo LB, Cosme-Silva L, Fernandes AP, et al. Effects of mineral trioxide aggregate, BiodentineTM and calcium hydroxide on viability, proliferation, migration and differentiation of stem cells from human exfoliated deciduous teeth. J Appl Oral Sci. 2018;26:e20160629.
- Asgary S, Nazarian H, Khojasteh A, et al. Gene expression and cytokine release during odontogenic differentiation of human dental pulp stem cells induced by 2 endodontic biomaterials. J Endod. 2014;40(3):387–392.
- Niu L-N, Pei D-D, Morris M, et al. Mineralogenic characteristics of osteogenic lineage-committed human dental pulp stem cells following their exposure to a discoloration-free calcium aluminosilicate cement. Dent Mater. 2016;32(10):1235–1247.
- Hanafy AK, Shinaishin SF, Eldeen GN, et al. Nano hydroxyapatite & mineral trioxide aggregate efficiently promote odontogenic differentiation of dental pulp stem cells. Open Access Maced J Med Sci. 2018;6(9):1727–1731.
- Maher A, et al. The effect of commercially available endodontic cements and biomaterials on osteogenic differentiation of dental pulp pluripotent-like stem cells. Dent. J. 2018;6:48.
- Miller AA, Takimoto K, Wealleans J, et al. Effect of 3 bioceramic materials on stem cells of the apical papilla proliferation and differentiation using a dentin disk model. J Endod. 2018;44(4):599–603.
- Saberi EA, Farhad-Mollashahi N, Sargolzaei Aval F, et al. Proliferation, odontogenic/osteogenic differentiation, and cytokine production by human stem cells of the apical papilla induced by biomaterials: a comparative study. Clin Cosmet Investig Dent. 2019;11:181–193.
- Wang Y, Zhou Y, Jin L, et al. Mineral trioxide aggregate enhances the osteogenic capacity of periodontal ligament stem cells via NF-κB and MAPK signaling pathways. J Cell Physiol. 2018;233(3):2386–2397.
- Gaur T, Lengner CJ, Hovhannisyan H, et al. Canonical WNT signaling promotes osteogenesis by directly stimulating Runx2 gene expression. J Biol Chem. 2005;280(39):33132–33140.
- Chen S, Gluhak-Heinrich J, Wang YH, et al. Runx2, osx, and dspp in tooth development. J Dent Res. 2009;88(10):904–909.
- Komori T. Regulation of bone development and extracellular matrix protein genes by RUNX2. Cell Tissue Res. 2010;339(1):189–195.
- Baek W-Y, Lee M-A, Jung JW, et al. Positive regulation of adult bone formation by osteoblast-specific transcription factor osterix. J Bone Miner Res. 2009;24(6):1055–1065.
- Artigas N, Ureña C, Rodríguez-Carballo E, et al. Mitogen-activated protein kinase (MAPK)-regulated interactions between osterix and Runx2 are critical for the transcriptional osteogenic program. J Biol Chem. 2014;289(39):27105–27117.
- Greenblatt MB, Shim J-H, Glimcher LH. Mitogen-activated protein kinase pathways in osteoblasts. Annu Rev Cell Dev Biol. 2013;29:63–79.
- Sciandra M, Marino MT, Manara MC, et al. CD99 drives terminal differentiation of osteosarcoma cells by acting as a spatial regulator of ERK 1/2. J Bone Miner Res. 2014;29(5):1295–1309.
- Ge C, Xiao G, Jiang D, et al. Identification and functional characterization of ERK/MAPK phosphorylation sites in the Runx2 transcription factor. J Biol Chem. 2009;284(47):32533–32543.
- Xiao G, Jiang D, Thomas P, et al. MAPK pathways activate and phosphorylate the osteoblast-specific transcription factor, Cbfa1. J Biol Chem. 2000;275(6):4453–4459.
- Ulsamer A, Ortuño MJ, Ruiz S, et al. BMP-2 induces osterix expression through up-regulation of Dlx5 and its phosphorylation by p38. J Biol Chem. 2008;283(7):3816–3826.
- Ortuño MJ, Susperregui ARG, Artigas N, et al. Osterix induces Col1a1 gene expression through binding to Sp1 sites in the bone enhancer and proximal promoter regions. Bone. 2013;52(2):548–556.
- Ortuño MJ, Ruiz-Gaspà S, Rodríguez-Carballo E, et al. p38 regulates expression of osteoblast-specific genes by phosphorylation of osterix. J Biol Chem. 2010;285(42):31985–31994.
- Liu H, Liu Y, Viggeswarapu M, et al. Activation of c-Jun NH(2)-terminal kinase 1 increases cellular responsiveness to BMP-2 and decreases binding of inhibitory Smad6 to the type 1 BMP receptor. J Bone Miner Res. 2011;26(5):1122–1132.
- Huang YF, Lin JJ, Lin CH, et al. C-Jun N-terminal kinase 1 negatively regulates osteoblastic differentiation induced by BMP2 via phosphorylation of Runx2 at Ser104. J Bone Miner Res. 2012;27(5):1093–1105.
- Biver E, Thouverey C, Magne D, et al. Crosstalk between tyrosine kinase receptors, GSK3 and BMP2 signaling during osteoblastic differentiation of human mesenchymal stem cells. Mol Cell Endocrinol. 2014;382(1):120–130.
- Huang S-C, Wu B-C, Kao C-T, et al. Role of the p38 pathway in mineral trioxide aggregate-induced cell viability and angiogenesis-related proteins of dental pulp cell in vitro. Int Endod J. 2015;48(3):236–245.
- Woo S-M, Hwang Y-C, Lim H-S, et al. Effect of nifedipine on the differentiation of human dental pulp cells cultured with mineral trioxide aggregate. J Endod. 2013;39(6):801–805.
- Wu B-C, Kao C-T, Huang T-H, et al. Effect of verapamil, a calcium channel blocker, on the odontogenic activity of human dental pulp cells cultured with silicate-based materials. J Endod. 2014;40(8):1105–1111.
- Chen YW, Ho CC, Huang TH, et al. The ionic products from mineral trioxide aggregate-induced odontogenic differentiation of dental pulp cells via activation of the Wnt/β-catenin signaling pathway. J Endod. 2016;42(7):1062–1069.
- Luo K. Signaling cross talk between TGF-β/Smad and other signaling pathways. Cold Spring Harb Perspect Biol. 2017;9(1):a022137.
- Yang H, Guo Y, Wang D, et al. Effect of TAK1 on osteogenic differentiation of mesenchymal stem cells by regulating BMP-2 via Wnt/β-catenin and MAPK pathway. Organogenesis. 2018;14(1):36–45.
- Mao C-y, Wang Y-g, Zhang X, et al. Double-edged-sword effect of IL-1β on the osteogenesis of periodontal ligament stem cells via crosstalk between the NF-κB, MAPK and BMP/Smad signaling pathways. Cell Death Dis. 2016;7(7):e2296–e2296.