ABSTRACT
Nervous system diseases, especially degenerative diseases of the central nervous system (CNS), seriously endanger human health, and the injured adult neurons are difficult to self-renew. Therefore, cell replacement therapy with good application prospects has become a hot spot in biomedical research. Human embryonic stem cells (hESCs) are promising sources of cells with unlimited proliferation ability and multiple differentiation potential. The purpose of this study was to use these characteristics of hESCs to obtain a large number of differentiated and stable neural stem cells (NSCs) and provide potential tissue engineering materials for the treatment of neurological diseases. Cultured on low adhesion plate for 4 days in vitro, the hESCs can differentiate into suspended embryoid bodies (EBs). After further culture of EBs for 4 days, high-purity Nestin-positive NSCs with typical rosette structure can be induced, and these cells can further differentiate into a variety of mature nerve cells. Meanwhile, hESCs could form in vivo typical teratomas that contained rosette structures. Our results indicated that hESCs had the ability to differentiate into rosette NSCs both in vitro and in vivo, and the bioactive rosettes are of great significance to understanding the mechanism of neural development and studying the treatment of neurodegenerative diseases.
GRAPHICAL ABSTRACT
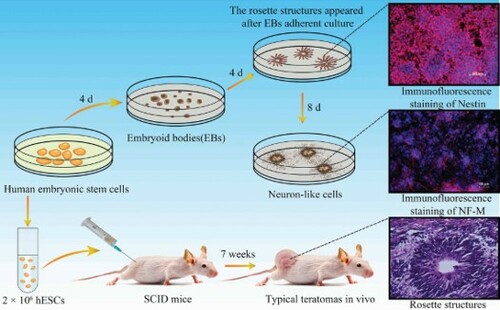
Introduction
The nervous system had a limited proliferative capacity, so it is difficult for the injured adult neurons to self-renew, which seriously hampered the prognosis of patients with diseases of the nervous system (Rodemer and Selzer Citation2019; Veneruso et al. Citation2019; Wang XJ et al. Citation2019). Fortunately, nerve cells that are usually difficult to obtain but of great value to disease treatment can be obtained by the directional differentiation of human embryonic stem cells (hESCs), which provided a powerful tool for disease models, drug screening and regenerative medicine (Karagiannis et al. Citation2019; Kojima et al. Citation2019; Xia et al. Citation2019). Currently, hESCs were one of the most promising cell sources amenable for commercialization of cell therapy products, but there has always been an controversy. The direct application or modification of hESCs is still in debate, some rules and regulation restrict the use of the cells for therapeutic and research purposes. However, the use and engineery of tissues resulting from hESCs differentiation is possible (Gill et al. Citation2016; Shroff et al. Citation2017).
Neural stem cells (NSCs) can self-update and differentiate into different types of nerve cells to maintain the integrity of the neurological function (Cummings et al. Citation2005). Studies have shown that NSCs grafts can promote the recovery of nerve function in rats with spinal cord injury by producing new cells and nerve growth factors (Gazdic M et al. Citation2017; Zarei-Kheirabadi et al. Citation2020). hESCs-NSCs overcome the problem of fewer sources of NSCs, and the differentiated cells have good uniformity. Our experiments were conducted to observe the hESCs differentiated into rosette NSCs in vitro and in vivo and to accurately evaluate the differentiation efficiency.
Materials and methods
Ethical statement
All the severe combined immunodeficient (SCID) mice were obtained from the Laboratory Animal Center of Shanghai, Academy of Science, China, and all experimental procedures were conducted in accordance with Chinese legislation on animal protection and approved by the Ethics Committee of Jiangsu University.
Human embryonic stem cells culture
hESCs line (SHhES2) was donated by M.D Jin Ying, School of Medicine, Shanghai Jiao Tong University (Li et al. Citation2010). The hESCs were initially maintained on KM3 feeders with a daily change of a medium that consisted of 80% knockout Dulbecco's Modified Eagle's Medium (DMEM), 20% knockout serum replacement, 1 mM L-glutamine, 1% nonessential amino acid (NEAA), 4 ng/mL basic fibroblast growth factor (bFGF) (all from Gibco, USA) and 0.1 mM β-mercaptoethanol (Sigma, USA). The colonies of hESCs were passaged every 4 days (Hu et al. Citation2012).
Differentiation cultures of hESCs in vitro
The undifferentiated hESCs colonies were dissociated by collagenase IV (Sigma, USA) with repeated pipetting to obtain small clumps, which were transferred to ultra-low attachment 6-well plates (Corning, USA). The embryoid bodies (EBs) were grown in medium consisted of 80% knockout DMEM, 20% knockout serum replacement, 1 mM L-glutamine, 1% NEAA and 0.1 mM β-mercaptoethanol. The cells were mixed gently before putted into the incubator to prevent cell aggregation. The medium was changed every other day. EBs were cultured for 4 days in suspension cultures. Then EBs were adherent cultured for additional 4 and 12 days in 96-well plates coated with 0.1% gelatin in neural induction medium containing DMEM/F12 (Hyclone, USA), 2% B27, 20 ng/mL bFGF, 20 ng/mL EGF, 1% NEAA (all from Gibco, USA). Every other day the medium was changed. When proceeded western blotting, cells were cultured in 6-well plates.
Teratoma formation in vivo
The potential of hESCs to form derivatives of ectoderm layers was examined in teratomas. Approximately 2 × 106 hESCs were harvested and re-suspended in a mixture of phosphate buffered saline (PBS; pH 7.3). The cell mixtures were subcutaneously injected into the rear leg of 4-weeks old SCID mice. About 7 weeks after injection, teratomas were dissected from the mice and fixed with 4% paraformaldehyde overnight. Tumors were embedded in paraffin and histologically examined after hematoxylin and eosin (HE) staining. Tumors were not detected in the control group that inoculated KM3 feeder cells (2 × 106) into the same sites.
Immunofluorescence
The cells were fixed with 4% paraformaldehyde for 20 min at room temperature, permeabilized with 0.3% Triton X-100 for 8 min and then blocked in 5% bovine serum albumin (BSA) in PBS for 20 min. After each step cells were washed with PBS for 5 min. The primary antibodies Nestin and medium-molecular-weight neurofilament (NF-M) (1:300, SAB, USA) were diluted with antibody diluent in proportion, then incubated overnight at 4°C. The next day was incubated with secondary antibodies Anti-rabbit IgG-Cy3 (1:100, Beijing ComWin Biotech, China) for 1 h at 37°C. Nuclei was counterstained with 1 µg/mL 4′,6-diamidino-2-phenylindole (DAPI, Sigma, USA) for 10 min at room temperature. Images were captured using a fluorescent microscope (Carl Zeiss Jena, Germany).
Western blotting analysis
Total protein of the adherent cells was extracted and 100 µg protein was subjected to 12% SDS-PAGE and subsequently electroblotted onto PVDF membranes. Dilute antibody to different concentrations with antibody diluent, then incubated overnight at 4°C with primary antibodies against NF-M (1: 600, ProteintechGroup, USA) and β-actin (1: 2000, ProteintechGroup, USA) respectively. Then incubated with species-specific horseradish peroxidase-conjugated secondary antibodies (Santa Cruz, USA) at a concentration of 1:3000. Finally, the immunoblots were visualized using ECL western blotting detection reagents (GE Healthcare, Buckinghamshire, UK). The protein density of each band was detected using a Quantity One Software for semi-quantitative evaluation.
RNA isolation and qRT-PCR
The cells at different stages of differentiation were collected separately. Total RNA was extracted from cells using TRIzol (Thermofisher, USA) and the purity and integrity of total RNA were evaluated by OD260/280. The RNA was reverse transcribed into cDNA using Olig(dT) as the primer according to the manufacturer's instructions by using the reverse transcription kit (Transgen Biotech, China), the resulting cDNA was used for qRT-PCR. Primers were: NF-M F: 5′-AAGTGAGGAGAAAGTGGTGGTG-3′; R: 5′TTTACTATGGCGTGTGAAGTGA-3′. GAPDH F: 5′- ATCAAGTGGGGCGATGCTG-3′; R: 5′ACCCATGACGAACATGGGG-3′. qRT-PCR was performed in three steps and the results were analyzed using CFX96 fluorescence qPCR instrument (Bio-Rad Laboratories, Inc. USA).
Statistical analysis
GraphPad Prism 5 statistical software was used for statistical processing. The experiment was repeated three times, and the data was expressed in mean ± SD. One-way-ANOVA method was used to compare data between single factor treatment groups. P<0.05 considered the difference to be statistically significant.
Result
Rosette structures in vitro
As shown in Figure A, the protocol was used for the generation of hESCs-NSCs and neuron-like cells. The hESCs cultured on KM3 feeder showed a uniform circular or elliptical shape with a high nuclear-cytoplasmic ratio, the hESCs arranged closely and the boundaries were clear (Figure B). Dissociated hESCs were seeded in ultra-low adhesion 6-well plates, cells gathered into small clumps growing in suspension and a dense spherical structure with good refraction were appeared on the 4th day. The three-dimensional spheres, which formed by the aggregation of hESCs in a suspension environment, were called EBs (Figure C). EBs were adherent culture in the specified medium for 4 days, the small, elongated cells in the center had generated rosette structures resembling the early neural tube, which often seen in the vicinity of adherent clumps. The cells were clustered distribution and very easily to distinguish from surrounding cells (Figure D). EBs expressed the neural marker Nestin and can be mutually transformed with the adherent cells (Figure (E-G)). The Nestin was also positive for these adherent cells in the rosette structures (Figure (H-J)).
Figure 1. Neural differentiation of EBs in vitro. (A) Schematic for neural differentiation; (B) hESCs cultured on KM3 feeder; (C) EBs after suspending culture; (D) The rosette structures appeared after EBs adherent culture, the white arrows indicated rosette structures; (E-G) Immunofluorescence staining of Nestin in EBs; (H-J) Immunofluorescence staining of Nestin in rosette structures; (B-J: Scale bars = 100 µm).
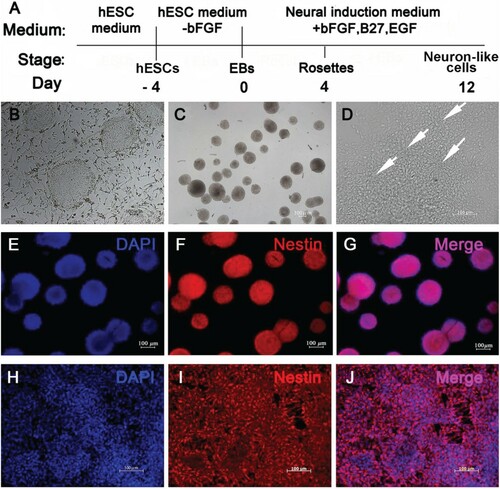
Rosette structures in vivo
The hESCs produced typical immature teratomas in vivo after injection into SCID mice (Figure A). The teratomas contained representative ectoderm tissues such as neural epithelia forming rosette structures (Figure (B-D)).
Figure 2. Formation of neural rosette structures at 7 weeks after hESCs injection into SCID mice. (A) Typical teratomas in vivo; (B) HE staining of the teratomas; (C) The white arrows indicated rosette structures; (D) The white arrows indicated rosette structure; (B: Scale bar = 200 µm; C: Scale bar = 100 µm; D: Scale bar = 50 µm).
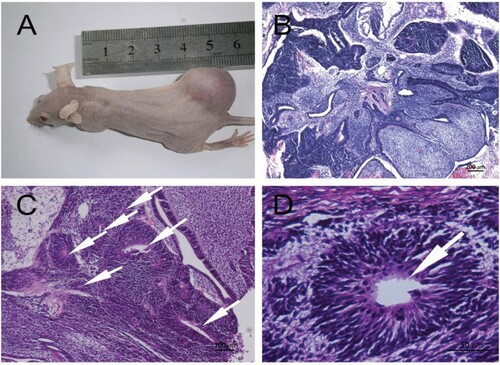
Neural differentiate of EBs in vitro
As time went on, cells moved out from the mass became much more and longer. For 12 days, a large number of cells grew out of the clumps, giving a starburst appearance. Cells spreaded out like a spider's web under a light microscope, the cell body was plump and had obvious three-dimensional structure. Most cells have two protrusions and a small portion of cells have more than one protuberance, arranging each other into an orderly bundle of fibers, we called these cells neuron-like cells (Figure A). Frequently, at the bottom of these cells we also could see a small number of irregular cells. On the 12th day, the gene and protein expression level of neuron-like cells was significantly higher than the other groups (Figure (B-C), P < 0.05). Immunofluorescence staining of the differentiated culture revealed that filamentous cells in the outgrowth areas expressed neuronal marker NF-M after cultured for 12 days (Figure (D-F)).
Figure 3. Neural differentiation of EBs in vitro for 12 days. (A) Neuron-like cells after EBs adherent culture; (B)The gene expression levels of NF-M; (C) The protein expression of NF-M; (D-F) Immunofluorescence staining of NF-M in neuron-like cells; (A, D-F: Scale bars = 100 µm; B, C: *P < 0.05, neuron-like cells compared with hESCs and EBs; β-actin: internal reference).
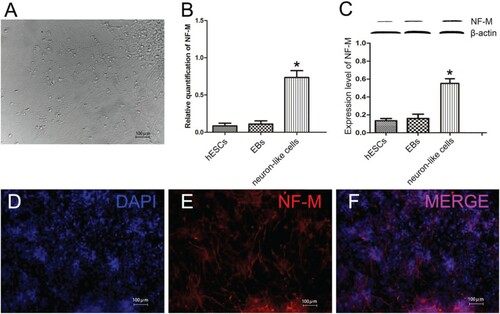
Discussion
Since the injured nerve could not regenerate, studies on nerve cells replacement had been increasingly growing in recent years (Deng et al. Citation2019). As a cell source of regenerative medicine, hESCs have great potential for clinical application. However, since hESCs are a kind of pluripotent cell that could not determine the direction of differentiation after transplantation, they will produce many other miscellaneous cells while obtaining the required cells (Khan et al. Citation2018). The discovery of clinical application potential of NSCs and neuron has greatly promoted the development of modern neuroscience. Therefore, the study of the differentiation of hESCs into NSCs and neuron cells is of great theoretical and practical significance for the repair of nerve injury (de Boni et al. Citation2018).
There are three main methods to induce differentiation of hESCs into NSCs in vitro. In this experiment, the EBs suspension culture method was used. This method of suspension re-attachment greatly preserves the ability of hESCs to differentiate naturally, which helps explore the specific mechanism during the induction process. At present, many differentiation systems are established based on the EBs differentiation system, such as hematopoietic stem cells, NSCs, cardiomyocytes, etc., the purpose is to simulate the embryonic development process in vivo. The cells in the three embryonic germ layers of EBs support each other, providing a microenvironment for differentiation and growth.
On the first day the adherent rate of EBs could only reach 53.33% with no firm adherent, which may be related to serum-free culture (Lee M et al. Citation2016). On the second day, the cells around the adherent clump periphery expanded outward in the form of monolayer, while the central cells gradually accumulated thickened and showed multi-layer growth. The neuroepithelial rosette structures that expressed neural marker Nestin were formed in the thickened region on the 4th day. Nestin expressed in the cytoplasm was also known as neuroepithelial stem cell protein, for Nestin expression was an important reference value for the identification of NSCs (Wang J et al. Citation2018; Wilhelmsson et al. Citation2019).
The neural rosette is a key morphological change in neural development, including cell polarization, neuronal differentiation gradient and interkinetic nuclear migration (INM) (Banda et al. Citation2015). NSCs were wrapped in the rosette to balance proliferation and differentiation. As potential transplanted sources in vivo, the rosette NSCs have the ability to differentiate into neurons and glial cells, have low immunogenicity, and can secrete a variety of neurotrophic factors, so they are widely used in scientific research and clinical trials, such as lateral sclerosis, Parkinson's disease, brain tumors and other diseases (Zhang et al. Citation2016; Hribkova et al. Citation2018).
hESCs can differentiate into embryonic precursors of all corresponding adult tissues in vitro and in vivo. Further research revealed that after injecting hESCs into SCID mice, hESCs-derived teratomas formed in vivo. Teratomas comprise all three germ layer-derived cell types demonstrating the multi-directional differentiation potential of hESCs. However, the risk of teratoma formation by residual undifferentiated cells after in vivo transplantation is the major obstacle to the clinical application of these cells (Lee AS et al. Citation2017).
Neural phenotype is mainly derived from the ectoderm, and perhaps the most promising phenotype to obtain from hESCs. Microarray data analysis showed that brain-derived NSCs and hESC-derived NSCs expressed different gene groups. Among them, hESC-derived NSCs preferred to express the markers of pre-neuroectodermal layer, which was thought to mimic the pattern of neurogenesis in vivo to a certain extent (Lian et al. Citation2007; Pan et al. Citation2017).
After 12 days of induction, we could see two circumstances happened in the adherent EBs: part of EBs were radially centered by adherent clumps with plump cell bodies and strong dimensional sense, the processes of cells showed long filamentous shape and the adjacent cell processes were weaved into a spider web, and these cells had a typical neuron morphology; another part of EBs unfolded, so only a small amount of strong third dimension and short protrusion neuron-like cells could be seen at the surface of EBs under the light microscope. However, we found that a large amount of typical morphology neurons could be seen at the bottom of cells after immunofluorescence staining. Along with the prolongation of induction, the expression level of NF-M protein also gradually increased. NF-M belonged to Type IV of the intermediate silk protein family, which had been widely used as neuron cell marker in the central and peripheral nervous system (Liu et al. Citation2019; Tang et al. Citation2019). Therefore, we conformed that we successfully induced rosette NSCs and neuron-like cells from hESCs in vitro.
In summary, hESCs (SHhES2) could differentiate into rosette NSCs in vitro and in vivo, and further into neuron-like cells after induction. They were the most promising potential source of NSCs and provided a rich and stable cell support for nerve regeneration.
Acknowledgments
We appreciate the stem cell bank, Chinese Academy of Sciences under a Materials Transfer Agreement for providing us hESCs lines (SHhES2). This work was supported by the National Natural Science Foundation of China (grant number 81571221), a project funded by the Priority Academic Program Development of Jiangsu Higher Education Institutions (PAPD, 4013000024/002) and Qing Lan Project of Jiangsu Province.
Disclosure statement
No potential conflict of interest was reported by the author(s).
Data availability statement
Data and materials supporting the results or analysis presented in the paper are available free of charge.
Additional information
Funding
References
- Banda E, McKinsey A, Germain N, Carter J, Anderson NC, Grabel L. 2015. Cell polarity and neurogenesis in embryonic stem cell-derived neural rosettes. Stem Cells Dev. 24(8):1022–1033.
- Cummings B, Uchida N, Tamaki S, Salazar D, Hooshmand M, Summers R, Gage FH, Anderson AJ. 2005. Human neural stem cells differentiate and promote locomotor recovery in spinal cord-injured mice. Proc Natl Acad Sci U S A. 102(39):14069–14074.
- de Boni L, Gasparoni G, Haubenreich C, Tierling S, Schmitt I, Peitz M, Koch P, Walter J, Wullner U, Brustle O. 2018. DNA methylation alterations in iPSC- and hESC-derived neurons: potential implications for neurological disease modeling. Clin Epigenetics. 10:13.
- Deng W, Shao F, He Q, Wang Q, Shi W, Yu Q, Cao X, Feng C, Bi S, Chen J, et al. 2019. EMSCs build an all-in-one niche via cell-cell lipid raft assembly for promoted neuronal but suppressed astroglial differentiation of neural stem cells. Adv Mater (Deerfield Beach, Fla). 31(10):e1806861.
- Gazdic M, Volarevic V, Arsenijevic A, Erceg S, Moreno-Manzano V, Arsenijevic N, Stojkovic M. 2017. Stem cells and labeling for spinal cord injury. Int J Mol Sci. 18(1):6.
- Gill KP, Hung SS, Sharov A, Lo CY, Needham K, Lidgerwood GE, Jackson S, Crombie DE, Nayagam BA, Cook AL, et al. 2016. Enriched retinal ganglion cells derived from human embryonic stem cells. Sci Rep. 6:30552.
- Hribkova H, Grabiec M, Klemova D, Slaninova I, Sun YM. 2018. Calcium signaling mediates five types of cell morphological changes to form neural rosettes. J Cell Sci. 131(3):jcs206896.
- Hu J, Hu S, Ma Q, Wang X, Zhou Z, Zhang W, Sun X, Zhu W, Qian H, Xu W. 2012. Immortalized mouse fetal liver stromal cells support growth and maintenance of human embryonic stem cells. Oncol Rep. 28(4):1385–1391.
- Karagiannis P, Takahashi K, Saito M, Yoshida Y, Okita K, Watanabe A, Inoue H, Yamashita JK, Todani M, Nakagawa M, et al. 2019. Induced pluripotent stem cells and their use in human models of disease and development. Physiol Rev. 99(1):79–114.
- Khan FA, Almohazey D, Alomari M, Almofty SA. 2018. Isolation, culture, and functional characterization of human embryonic stem cells: current trends and challenges. Stem Cells Int. 2018:1429351.
- Kojima K, Miyoshi H, Nagoshi N, Kohyama J, Itakura G, Kawabata S, Ozaki M, Iida T, Sugai K, Ito S, et al. 2019. Selective ablation of tumorigenic cells following human induced pluripotent stem cell-derived neural stem/progenitor cell transplantation in spinal cord injury. Stem Cells Transl Med. 8(3):260–270.
- Lee M, Kim Y, Ryu JH, Kim K, Han YM, Lee H. 2016. Long-term, feeder-free maintenance of human embryonic stem cells by mussel-inspired adhesive heparin and collagen type I. Acta Biomater. 32:138–148.
- Lee AS, Tang C, Hong WX, Park S, Bazalova-Carter M, Nelson G, Sanchez-Freire V, Bakerman I, Zhang W, Neofytou E, et al. 2017. Brief Report: External beam radiation therapy for the treatment of human pluripotent stem cell-derived teratomas. Stem Cells. 35(8):1994–2000.
- Li C, Yang Y, Lu X, Sun Y, Gu J, Feng Y, Jin Y. 2010. Efficient derivation of Chinese human embryonic stem cell lines from frozen embryos. In Vitro Cell Dev Biol Anim. 46(3-4):186–191.
- Lian Q, Lye E, Suan Yeo K, Khia Way Tan E, Salto-Tellez M, Liu TM, Palanisamy N, El Oakley RM, Lee EH, Lim B, et al. 2007. Derivation of clinically compliant MSCs from CD105+, CD24- differentiated human ESCs. Stem Cells. 25(2):425–436.
- Liu H, Xia J, Wang T, Li W, Song Y, Tan G. 2019. Differentiation of human glioblastoma U87 cells into cholinergic neuron. Neurosci Lett. 704:1–7.
- Pan M, Wang X, Chen Y, Cao S, Wen J, Wu G, Li Y, Li L, Qian C, Qin Z, et al. 2017. Tissue engineering with peripheral blood-derived mesenchymal stem cells promotes the regeneration of injured peripheral nerves. Exp Neurol. 292:92–101.
- Rodemer W, Selzer ME. 2019. Role of axon resealing in retrograde neuronal death and regeneration after spinal cord injury. Neural Regen Res. 14(3):399–404.
- Shroff G, Dhanda Titus J, Shroff R. 2017. A review of the emerging potential therapy for neurological disorders: human embryonic stem cell therapy. Am J Stem Cells. 6(1):1–12.
- Tang C, Wang M, Wang P, Wang L, Wu Q, Guo W. 2019. Neural stem cells behave as a functional niche for the maturation of newborn neurons through the secretion of PTN. Neuron. 101(1):32–44.e6.
- Veneruso V, Rossi F, Villella A, Bena A, Forloni G, Veglianese P. 2019. Stem cell paracrine effect and delivery strategies for spinal cord injury regeneration. J Controlled Release. 300:141–153.
- Wang J, Huang Y, Cai J, Ke Q, Xiao J, Huang W, Li H, Qiu Y, Wang Y, Zhang B, et al. 2018. A Nestin-Cyclin-dependent kinase 5-dynamin-related protein 1 axis regulates neural stem/progenitor cell stemness via a metabolic shift. Stem Cells. 36(4):589–601.
- Wang XJ, Peng CH, Zhang S, Xu XL, Shu GF, Qi J, Zhu YF, Xu DM, Kang XQ, Lu KJ, et al. 2019. Polysialic-acid-based micelles promote neural regeneration in spinal cord injury therapy. Nano Lett. 19(2):829–838.
- Wilhelmsson U, Lebkuechner I, Leke R, Marasek P, Yang X, Antfolk D, Chen M, Mohseni P, Lasic E, Bobnar ST, et al. 2019. Nestin regulates neurogenesis in mice through notch signaling from astrocytes to neural stem cells. Cerebral Cortex (New York, NY: 1991). 29(10):4050–4066.
- Xia B, Chen G, Zou Y, Yang L, Pan J, Lv Y. 2019. Low-intensity pulsed ultrasound combination with induced pluripotent stem cells-derived neural crest stem cells and growth differentiation factor 5 promotes sciatic nerve regeneration and functional recovery. J Tissue Eng Regen Med. 13(4):625–636.
- Zarei-Kheirabadi M, Sadrosadat H, Mohammadshirazi A, Jaberi R, Sorouri F, Khayyatan F, Kiani S. 2020. Human embryonic stem cell-derived neural stem cells encapsulated in hyaluronic acid promotes regeneration in a contusion spinal cord injured rat. Int J Biol Macromol. 148:1118–1129. eng.
- Zhang D, Mai Q, Li T, Huang J, Ding C, Jia M, Zhou C, Xu Y. 2016. Comparison of a xeno-free and serum-free culture system for human embryonic stem cells with conventional culture systems. Stem Cell Res Ther. 7(1):101.