ABSTRACT
The effects of flavonoids, in the medical formulation Daflon 500 mg (DAF) in protection of the heart against ischemia/reperfusion are not completely understood. Heart function data from Langendorff perfused Wistar rat hearts (n = 8/group) were digitally computed, and the infarct size was evaluated using 2,3,5-triphenyl tetrazolium chloride (TTC) staining and cardiac enzyme levels. The total oxidants (TOS) and antioxidants (TAS) levels as well as pro- and anti-inflammatory cytokines levels were estimated by TOS and TAS assay kits and enzyme-linked immunosorbent assay (ELISA), respectively. The antioxidant enzymes, superoxide dismutase (SOD) and catalase (CAT) were evaluated using RANDOX kits. Longtime treatment of DAF for 2 or 4 weeks normalized cardiac hemodynamics, vascular dynamics, and heart contractility. A significant reduction in the infarct size and cardiac enzyme levels (P < .05) was produced by DAF treatment. Short time infusion of DAF two hours before inducing ischemia yielded similar results. DAF treatment significantly (P < .05) decreased TOS levels in the perfusion effluent and increased SOD levels in the cardiomyocytes. In conclusion, treatment with DAF prevents the deterioration of cardiac function and ischemic injury caused by oxidative stress and inflammatory cytokines. The protective effects of DAF are possibly due to its antioxidant and anti-inflammatory properties.
Background
Cardiovascular diseases (CVDs) are a major cause of morbidity and mortality, with ischemic heart disease (IHD) being the leading cause of death worldwide (Cassar et al. Citation2009; Benjamin et al. Citation2019). IHD eliminates or diminishes coronary perfusion, depriving myocytes of oxygen and essential nutrients (Canty and Suzuki Citation2012). Lack of oxygen negatively impacts the process of metabolism, resulting in reduced ATP availability, which in turn causes irreversible damage to the heart (Lim Citation2017). The death of cardiomyocytes produced by hypoxia is attributed to prolonged ischemia with or without reperfusion leading to myocardial infarction (MI) (Canty and Suzuki Citation2012). The MI size is recognized to increase the chances of arrhythmia, heart failure and worsen the prognosis of the IHD (Burns et al. Citation2002; Bellandi et al. Citation2004). Reperfusion by revascularization of the occluded blood vessel remains the standard method for the protection of cardiomyocytes against I/R injury. However, it contributes considerable injury to the heart (Gersh et al. Citation2005). This scenario necessitates the introduction of new therapies to assist reperfusion in order to provide a better outcome in the protection of the heart against ischemia/reperfusion (I/R) injury (Bolli et al. Citation2004).
The role of reactive oxygen species (ROS) and inflammatory cytokines and their interactions in I/R injury are not completely understood. ROS are believed to underlie many diseases including cardiovascular diseases (Sugamura and Keaney Citation2011). However, they are also essential molecules in many physiological functions (Palazzo et al. Citation2011). Although the role of oxidative stress in I/R injury and postconditioning protection is well documented (Suzuki Citation2009; Dare et al. Citation2015), the reported results are discordant. I/R causes an increased production of ROS from the mitochondria (Hausenloy and Yellon Citation2013) which oxidizes many proteins leading to their damage and loss of function (Burgoyne et al. Citation2013). Reperfusion is associated with increased ROS production and exacerbates cell death (Braunersreuther and Jaquet Citation2012). Redox signaling produces antioxidants which neutralize free radicals and contribute in protecting the heart during I/R (Pagliaro and Penna Citation2015). Antioxidants either neutralize ROS directly or activate pathways that protect the heart (Techapiesancharoenkij et al. Citation2015). Administration of antioxidants before or during reperfusion reduces the severity of I/R injury possibly by overcoming the detrimental effects of ROS (Banerjee et al. Citation2002). Hence, the excessive production of ROS caused by the reperfusion and the reduction in antioxidants possibly caused by comorbidities, such as diabetes mellitus, requires higher levels of antioxidants for their neutralization (Penna et al. Citation2009).
Inflammatory cytokines contribute to both health and disease (Dinarello Citation2000; Zhang et al. Citation2007). The inflammatory response during I/R injury results in overproduction of proinflammatory cytokines such as tumor necrosis factor-alpha (TNF-α), interleukin-1-beta (IL-1β) and IL-6. The excessive production of these cytokines may contribute to the worsening of I/R injury (Yu et al. Citation2010; Jong et al. Citation2016). Anti-inflammatory cytokines, on the other hand, play an essential role in counterbalancing the influence of proinflammatory cytokines (Dinarello Citation2000; Zhang et al. Citation2007). Anti-inflammatory medicines are known to significantly reduce the content of serum inflammatory factors, IL-1β, IL-6 and TNF-α in injury (Marquez Loza et al. Citation2017). Interleukin 10 (IL-10) an anti-inflammatory cytokine, showed a potent role in mitigating the inflammatory responses of TNF-α, IL-1β, and IL-6 (Zhang et al. Citation2007).
DAF is a micronized purified flavonoid fraction containing 90% diosmin and 10% flavonoids expressed as hesperidin (Sirlak et al. Citation2010). DAF has shown beneficial effects on CVD, however, its role in I/R is not clear (Lyseng-Williamson and Perry Citation2003; Boisseau Citation2007). DAF is a member of the flavonoid family and is known for its antioxidant activities (Kobo et al. Citation2014). In general, flavonoids have potent antioxidant and anti-inflammatory effects, which make them suitable for the treatment of several diseases (de Souza et al. Citation2016). DAF inhibits cellular oxygenase, enhances cellular antioxidants (Nijveldt et al. Citation2001) and it is a well-known drug for the treatment of varicose veins and venous ulcers (Raffetto Citation2016). It has also shown high efficiency in the treatment of leg edema, skin disorders, venous disease symptoms and helps in healing skin ulcers (Ramelet Citation2001). DAF is also prescribed for many vascular disease patients to provide protection against I/R injury. The potentials of DAF in the protection of the heart has yet to be studied and only a single clinical study has shown DAF to have beneficial effects in stabilizing heart function after cardiac operations (Sirlak et al. Citation2010). Therefore, in this study, the antioxidant Daflon (DAF) was used to investigate its possible protective role to the heart, its effect on oxidative stress and on the levels of inflammatory cytokines during I/R.
Materials and methods
Experimental animals
This study was approved by the Health Science Center, Kuwait University Animal Ethics Committee and the experiments were performed according to the animal care guidelines of Kuwait University and international guidelines. All animals were maintained under controlled temperature (21–24°C), 12 h light/dark cycle (7 am to 7 pm) and humidity (50%). Animals were housed in plastic cages (2 rats per cage), and food and water were available ad libitum.
Male Wistar rats (250–350 g) were anesthetized by an intramuscular injection of sodium pentobarbital (60 mg/kg) and anticoagulated with the intraperitoneal injection of heparin (1000 U/kg body weight). Animals were then sacrificed by cervical dislocation. The diaphragm was accessed by a transabdominal incision and cut carefully to expose the thoracic cavity. The thorax was opened by a bilateral incision along the lower margin of the chest from the last to the first ribs. The thoracic cage was folded back, and the heart was exposed and excised. To limit ischemic injury during the time between excision and the restoration of vascular perfusion, the heart was directly immersed in cold (4°C) Krebs-Hensleit (KH) solution (Khalaf and Babiker Citation2016).
Drugs and chemicals
Rats were randomly subdivided into six groups (n = 8) (Figure ). One group served as sham in which the hearts were cannulated and a thread was passed below the coronary artery with no additional treatment. An other group served as a control, in which the animals were subjected to I/R with no additional treatment. The rest of the rats were divided into two major groups. The first group received long-term treatment of 1 g DAF dissolved in distilled water (1 g/1 L) and was subdivided into two subgroups; one subgroup was subjected to DAF for 2 weeks and another subgroup for 4 weeks. The second group comprised DAF acute treatment subgroups, of which one subgroup was injected intravenously with 0.1 g/Kg DAF dissolved in normal saline 2 h before the sacrifice. The hearts of another subgroup of rats were perfused with 1 g of DAF dissolved in 1 L of KH solution beginning 5 min before the start of reperfusion and continued for 10 min during reperfusion. All doses where adopted from previous studies (Delbarre et al. Citation1995).
Figure 1. Schematic representation showing the experimental protocols used in the study. Hearts isolated from Wister rats were subjected to one of the following protocols (n = 8). (A) Sham, only canulation, stabilization and perfusion for 1 h. (B) Unprotected ischemia-reperfusion (control). (C) Hearts isolated from rats treated with DAF in drinking water for 4 weeks. (D) Hearts isolated from rats treated with DAF in drinking water for 2 weeks. (E) Hearts isolated from rats injected with intravenous DAF 2 h before sacrifice. (F) Hearts infused with DAF at the beginning of reperfusion.
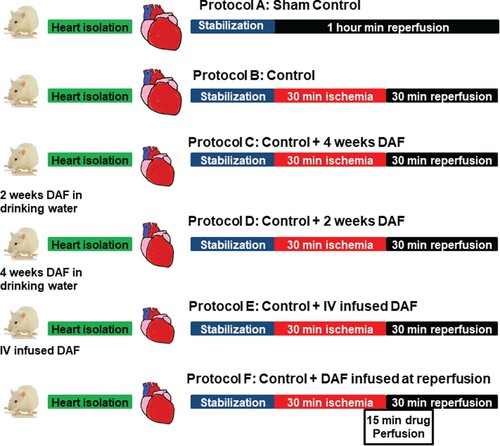
Experimental procedure
Heart cannulation and perfusion were performed using a modified Langendorff setup for the perfusion of rat heart as described before (Abwainy et al. Citation2016). Briefly, the isolated heart was put in cold KH buffer (4°C) and was immediately mounted and fixed to a small cannula and then perfused retrograde with freshly prepared KH buffer. The buffer was gassed with a mixture of O2 (95%) and CO2 (5%), the pH of which was 7.35–7.45, and the temperature of the perfusion buffer was maintained at 37°C, by circulation in a temperature controller using a water bath (RMS Lauda, Dr. R. Wobser GmbH & Co, Germany) and a Techno Circulator (Cole-Parmer Instrument Company, USA). The myocardial temperature was monitored by a needle thermistor probe (Thermalert TH-5, Physitemp, USA) inserted at the apex of the heart. The heart was allowed 10 min for acclimatization until the normal contractile function and the heart rhythm was restored. A water-filled latex balloon was placed and secured in the left ventricular (LV) cavity (Mulvihill and Foley Citation2002). The balloon was attached to a pressure transducer and a DC bridge amplifier (DC-BA) with a pressure module (DC-BA type 660, Hugo-Sachs Electronik, Germany) and interfaced to a personal computer for online monitoring of the hemodynamics (LV end-diastolic pressure (LVEDP)), the LV maximum developed pressure (DPmax) and LV contractility (+dP/dt or -dP/dt). The balloon was inflated using a microsyringe until a baseline LVEDP of 6 mmHg was reached. The DPmax, (mmHg) was derived from the online acquisition of the LV end-systolic pressure (LVESP) using the Max–Min module (Number MMM type 668, Hugo-Sachs Elektronik-Harvard Apparatus GmbH, Germany), which converts the output from a DC bridge amplifier to DPmax by subtracting LVEDP from the maximal LVESP.
The coronary vascular dynamics were evaluated by assessing the coronary flow (CF, mL/min) and coronary vascular resistance (CVR, mmHg/mL/min). The CF was continuously measured using an electromagnetic flow probe attached to the inflow of the aortic cannula and interfaced with a personal computer. Continuous monitoring of the CF in mL/min was verified manually by a timed collection of the coronary effluent. The CVR was computed once every 10 s along with the hemodynamics data by an online data acquisition program (Isoheart software V 1.524-S, Hugo-Sachs Electronik, Germany). The perfusion pressure (PP) was kept constant at 50 mmHg throughout the experimental procedure in all protocols. The PP was measured immediately downstream from the flow probe in a branch of the aortic cannula using a Statham pressure transducer (P23 Db). Constant PP was ensured using an electronic perfusion assembly (Module PPCM type 671, Hugo-Sachs Electronik, Germany).
The heart was then instrumented with pacing electrodes placed on the right atrial (RA) appendage. Regional ischemia was produced by passing a snare around the left coronary artery approximately 0.5 cm below the atrioventricular groove. A rigid plastic tube was positioned between the heart and the suture to ensure complete occlusion of the coronary vessel and to allow the re-opening of the occluded vessel without mechanical damage. All hearts were subjected to 30 min ischemia followed by 30 min reperfusion.
Sample collection and storage
Coronary effluent was collected manually at the apex of the heart from the coronary outflow in small tubes at the end of the reperfusion phase. The hearts were also collected at the end of reperfusion. All heart samples and coronary effluents were frozen in liquid nitrogen and stored at −80°C for further analysis.
Evaluation of infarct size by triphenyl tetrazolium chloride (TTC) staining
The infarct size was measured 24 h after the end of reperfusion (n = 4) using Scion ImageJ (ImageJ, Wayne Rasb and National Institute of Health, USA). At the end of the reperfusion, the heart was stored overnight at −20°C. The next day, each heart was sliced transversely into 4–6 pieces from apex to base. The slices were then incubated in 1% TTC solution in isotonic pH 7.40 phosphate buffer and then fixed in 4% formaldehyde for 24 h. Red-stained viable tissues were easily distinguished from the pale, non-stained necrotic infarcted tissue. The red and white areas of every slice were manually indicated on the image. The percentage of the infarcted area of the LV was calculated for every section of the heart (Babiker et al. Citation2019).
Assessment of heart injury via creatine kinase and lactate dehydrogenase levels
Cardiomyocyte injury was evaluated by quantifying creatine kinase (CK) and lactate dehydrogenase (LDH) levels in the coronary effluent at the end of the reperfusion period as described previously by Ferrera et al. (Citation2009).
Immunoblotting
Left ventricles (n = 4) were homogenized in ice-cold lysis buffer, and the homogenate was centrifuged at 4000 rpm for 10 min. The supernatant was collected, the protein content was measured, and the samples were aliquoted and stored at −80°C for further analysis. The expression of the protein in question was determined as described previously (Al-Herz and Babiker Citation2017). Equal loading was checked by stripping the membrane and reprobing with GAPDH antibodies. The total caspase-3 levels were determined using monoclonal antibodies (Cell Signaling Technology, Inc cat# 9662). Detection was performed using enhanced chemiluminescence after incubation with a suitable secondary antibody conjugated to horseradish peroxidase (ECL; Cell Signaling Technology). Densitometric analysis was performed using Quantity One software (BioRad).
Estimation of cytokines by enzyme-linked immunosorbent assay (ELISA)
The LV homogenate (n = 4) was used to evaluate the expression of the proinflammatory cytokines: TNF-α (cat# MBS175904), IL-1 (cat# MBS825017), and IL-6 (cat# MBS355410) and the anti-inflammatory cytokine, IL-10 (cat# MBS355232) using ELISA. The protein content was measured in the samples and the latter were diluted as suggested by the manufacturer’s assay protocols for the respective commercial kits (Biosource International, USA).
Estimation of total oxidant levels
The total oxidant levels (TOS) were quantified after DAF treatment before I/R procedure or at reperfusion using the TOS Status Assay Kit (Rel Assay Diagnostics Kit, cat# RL035) (Erel Citation2005). Snap-frozen heart tissue (n = 4) was homogenized in RIPA buffer as recommended by the TOS Kit manufacturer. RIPA buffer was prepared (using Tris, NaCl, NP-40, EDTA, Na-deoxycholate, 0.1% SDS, protease inhibitor (Sigma Aldrich, St. Louis, Missouri, cat# s8820)), benzamidine and phenylmethane sulfonyl fluoride or phenylmethylsulfonyl fluoride (PMSF). Na-deoxycholate, protease inhibitor, and PMSF were added on the day of the experiment. The homogenate was then centrifuged at 15,000g for 25 min at 4°C, and the supernatant was separated and stored at −80 °C. The samples were placed on ice and diluted 1:10 with deionized (DI) water. Next, 18.75 µl of DI water (blank), standard solution or homogenized samples were pipetted into the corresponding wells of a 96-well plate. Then, 125 µl of reagent 1 (R1) was added to all wells, and the initial absorbance was read at 530 nm after 30 s. Then, 6.25 µl of reagent (colored 2,2'-azino-bis(3-ethylbenzothiazoline-6-sulphonic acid (ABTS) radical solution)) was added to all wells and incubated for 10 min at room temperature, and the second absorbance was measured at 530 nm. The results were calculated according to the manufacturer’s guidelines by fitting the absorbance values into the equation provided with the kit.
Estimation of total antioxidant levels
The heart homogenate (n = 4) was also used to analyze the total antioxidant status (TAS) after DAF treatment, before I/R procedure or at reperfusion using a commercially available assay kit (Rel Assay Diagnostics kit, cat#RL032) (Erel Citation2004). Deionized water (7.5 µl; blank), standard solution or homogenized samples were pipetted into the corresponding wells of a 96-well plate. Then, 125 µl of reagent 1 (R1) was added to all wells, and the initial absorbance was read at 660 nm after 30 s. Next, 6.25 µl of reagent 2 (ABTS radical solution) was added to all wells and incubated for 10 min at room temperature, and the second absorbance was measured at 660 nm. The results were calculated according to the manufacturer’s guidelines by fitting the absorbance values into the equation provided with the kit.
Antioxidant enzymes status
The LV homogenate (n = 4) was used for the evaluation of antioxidant enzymes activity. Superoxide dismutase (SOD; EC.1.15.1.1) and Catalase (CAT; EC.1.11.1.6) activity was measured by UV spectrophotometer with RANDOX kits (Cat. No. SD 125; Randox Labs Ltd., Crumlin, UK) according to the manufacturer’s instructions.
Statistical analysis
The data were represented as the mean ± S. E. M. Two-way analysis of variance (ANOVA) was used to assess the significant differences between the means of different groups. The post hoc analysis, using the Tukey test, was conducted for further comparison. An unpaired two-sided ‘t’ test was used to analyze differences in infarct size between the groups. In all cases, P < .05 was considered statistically significant.
Results
Effects of ischemia on cardiac hemodynamics and vascular dynamics
In this study, the body and heart weights at the time of sample collection were not significantly different between the experimental groups. The average water intake was similar in all experimental groups (42.57 ± 1.4 mL/rat in control vs 41.45 ± 1.9 ml/rat in DAF treated animals). There were differences in the baseline values and the values measured at the end of reperfusion in the heart hemodynamics, contractility and coronary-vascular dynamics in sham animals, however, these difference did not reach significant levels. Regional ischemia resulted in a significant (P < .01) deterioration in LV, coronary vascular dynamics and LV contractility compared to sham hearts. Treatment with DAF for 4, 2 weeks or 2 h before the animal sacrifice protected the heart against I/R injury compared with the results for the respective ischemic periods and the controls. This protection, however, was not observed when DAF was infused immediately at reperfusion (Figure ). Treatment with DAF for 4, 2 weeks or 2 h before sacrifice resulted in a significant (P < .01) improvement in LV dynamics; DPmax, LVEDP and LV contractility (P < .05) and the vascular dynamics assessed by the CF (P < .05) and CVR (P < .05) compared with those of the respective ischemic periods and controls (Figure and Table ). DAF administration at the time of reperfusion after the local ischemia did not produce significant protection to the heart against I/R injury, neither did it improve the LV dynamics, contractility nor coronary vascular dynamics compared with the results for the respective ischemic periods and the control group (Figure and Table ). The recovery shown in all hearts in the cardiovascular parameters are comparable to sham group values (Figure and Table ).
Figure 2. Left ventricle function (DPmax and LVEDP) and coronary vascular dynamics (CF and CVR) during post-ischemic recovery after DAF treatment protocols (n = 8). The data were computed after 30 min reperfusion and expressed as mean ± SEM. DPmax: maximum developed pressure; LVEDP: left ventricular end-diastolic pressure; CF: coronary flow; CVR: coronary vascular resistance; Ctr, control; DAF 2 h, Daflon infusion 2 h before sacrifice; DAF 2W, Daflon administration for 2 weeks; DAF 4W, Daflon administration for 4 weeks; Rep + DAF, Daflon infusion at reperfusion. *P < .05 compared with respective controls, **P < .01 compared with sham and †P < .05 compared with the ischemic period.
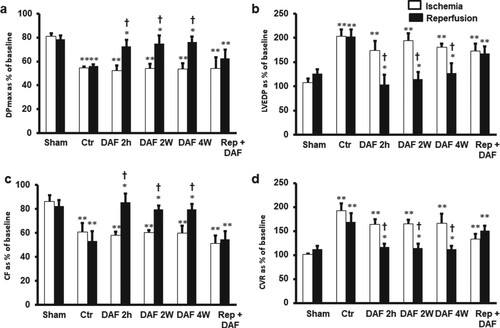
Table 1. Effects of ischemia/reperfusion and DAF treatment on heart contractility (+dP/dt and −dP/dt).
Variability of infarct size in the control and treatment groups and cardiomyocytes survival
In line with the hemodynamics data, the infarct size presented as a percentage area of the LV was significantly (P < .001) decreased by DAF exposure for 4, 2 weeks or 2 h before the sacrifice compared with the values for the respective controls (Figure ). There were no significant differences in the infarct size ratios between the groups that showed treatment-induced improvements (Figure ). Similar results were obtained when heart enzymes levels were measured (Table ). DAF administration for 4, 2 weeks or 2 h before sacrifice resulted in a marked decrease in myocyte apoptosis as shown by caspase-3 expression levels (P < .01) (Figure ). All the protective effects were concealed when DAF was infused at reperfusion (Figures and and Table ).
Figure 3. Infarct size after DAF treatment (n = 4). Top: representative 2,3,5-triphenyl-2H-tetrazolium chloride-stained heart slices for each treatment condition. Bottom: measured infarct size, normalized to the LV area, in isolated rat hearts at the end of reperfusion. Ctr, control; Rep, reperfusion; DAF 2 h, Daflon infusion 2 h before sacrifice; DAF 2W, Daflon administration for 2 weeks; DAF 4W, Daflon administration for 4 weeks. *P < .001 compared with respective controls.
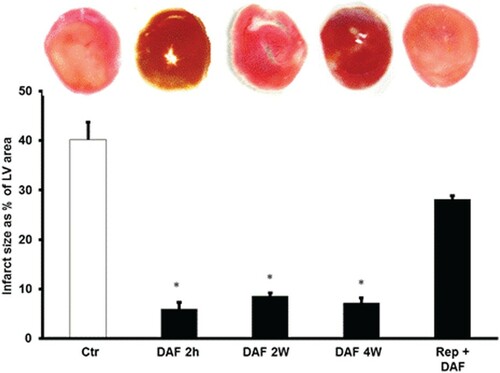
Figure 4. DAF effect on caspase-3 expression in the supernatants of homogenized left ventricles by immunoblotting (n = 4). (A) Western blot showing the expression of caspase-3 and (B) expression levels of caspase-3. Values are means ± SEM for 4 individual experiments. Ctr, control; DAF 2 h, Daflon infusion 2 h before sacrifice; DAF 2W, Daflon administration for 2 weeks; DAF 4W, Daflon administration for 4 weeks. *P < .05 compared with the respective controls.
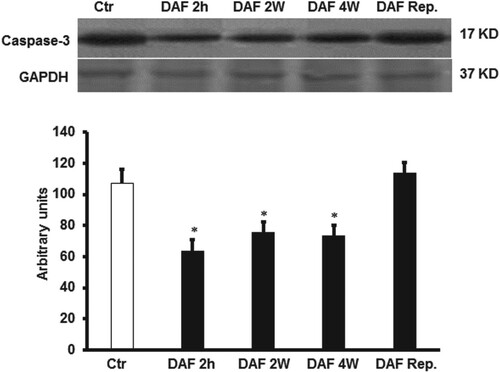
Table 2. Effects of ischemia/reperfusion and DAF treatment on cardiac enzymes.
Role of cytokines in DAF-mediated protection of the heart from ischemia/reperfusion injury
To study the role of cytokines on I/R injury and the potential protection provided by DAF, the levels of TNF-α, IL-1β, IL-6 and IL-10 were measured in the cardiomyocyte lysate by ELISA. DAF treatment for 4, 2 weeks or 2 h before sacrifice resulted in a remarkable decrease in the expression levels of TNF-α, IL-1β, and IL-6 (P < .01, P < .05 and P < .05 respectively) (Figure (A–C)) compared with that of the untreated controls.
Figure 5. Proinflammatory and anti-inflammatory cytokine levels in the cardiac muscle samples after DAF infusion for 2, 4 weeks, or 2 h before sacrifice or at reperfusion compared with those in the control group (n = 4). (A) DAF decreased the TNF-α protein levels, (B) decreased the IL-1 protein levels, (C) decreased the IL-6 protein levels and (D) had no effect on the anti-inflammatory cytokine IL-10 protein levels. Ctr, control; DAF 2 h, Daflon infusion 2 h before sacrifice; DAF 2W, Daflon administration for 2 weeks; DAF 4W, Daflon administration for 4 weeks. *P < .05 compared with the respective controls.
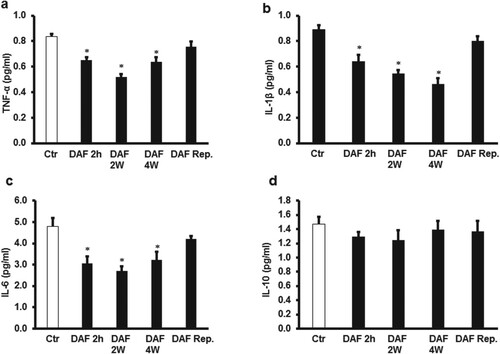
To evaluate the potential anti-inflammatory effects of DAF, the levels of IL-10 were measured using ELISA. Unexpectedly, the IL-10 protein levels were not affected by DAF treatment for 4, 2 weeks or 2 h before sacrifice or when infused at reperfusion (Figure (D)). DAF infusion at the time of reperfusion did not show a significant effect on the TNF-α, IL-1β, IL-6 or IL-10 levels (Figures (A–D)). There were no significant differences between TNF-α, IL-1β, IL-6 levels in the protective treatments (Figure ).
Oxidants and antioxidants status in DAF treatments
Interestingly, DAF treatment 4, 2 weeks or 2 h before the animal sacrifice and ischemic intervention resulted in a significant (P < .05) increase in TAS levels and a significant (P < .05) decrease in TOS levels (Figure (A,B)) compared to respective controls. Surprisingly, I/R procedure after the oral administration of DAF to the rats in drinking water for 4, 2 weeks or 2 h before the animal sacrifice extinguished the significant differences in the levels of TOS or TAS observed before the ischemic intervention in the cardiomyocytes lysate compared with those of untreated hearts (Figure (A,B)). However, the TAS levels in the effluent were below the detection level, TOS levels were significantly (P < .05) lowered by the administration of DAF to the rats in the drinking water for 4, 2 weeks or 2 h before the animal sacrifice compared to the respective controls. This decrease in TOS levels was not observed when DAF was infused at reperfusion (Figure (C)). There were no significant differences in the TOS levels between the protective treatments (Figure ).
Figure 6. Estimation of oxidative stress in rat hearts. (A) Total oxidant and (B) total antioxidant levels in cardiac muscle samples after DAF administration for 2, 4 weeks, or 2 h before the animal sacrifice at the end of treatment period and before ischemia-reperfusion procedure compared with the levels in the control group (n = 4). Ctr, control; DAF 2 h, Daflon infusion 2 h before sacrifice; DAF 2W, Daflon administration for 2 weeks; DAF 4W, Daflon administration for 4 weeks. *P<0.05 compared with the respective controls.
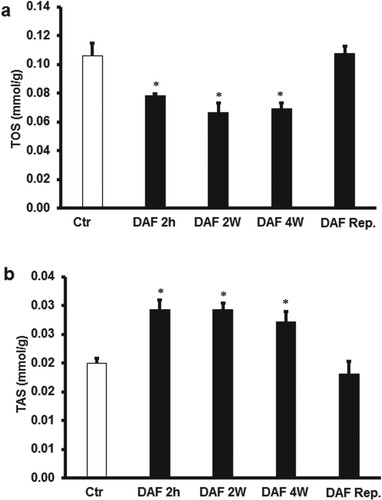
Figure 7. Estimation of oxidative stress in rat hearts. (A) Total oxidant and (B) total antioxidant levels in cardiac muscle samples after DAF administration for 2, 4 weeks, or 2 h before sacrifice or at reperfusion compared with the levels in the control group (n = 4). (C) Total oxidants levels in the effluent samples after DAF administration for 2, 4 weeks, or 2 h before sacrifice or at reperfusion compared with the levels in the control group. Ctr, control; DAF 2 h, Daflon infusion 2 h before sacrifice; DAF 2W, Daflon administration for 2 weeks; DAF 4W, Daflon administration for 4 weeks. *P < .05 compared with the respective controls.
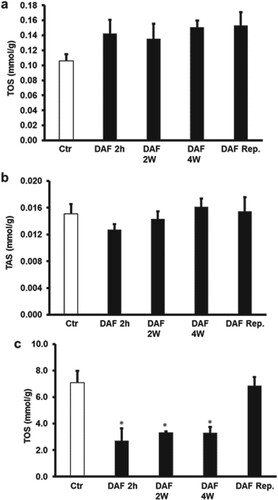
In order to confirm oxidants and antioxidant levels, the effect of DAF treatment on the antioxidant enzymes, SOD and CAT was studied. Ischemia/reperfusion decreases the cardiomyocytes SOD and CAT protein levels, which was significantly (P < .01) increased by DAF treatment for 4, 2 weeks or 2 h before sacrifice compared with the controls. This increase SOD and CAT levels was not observed when DAF was infused at reperfusion (Figure ).
Figure 8 . Estimation of oxidative stress in rat hearts using SOD and CAT levels. (A) SOD in cardiac muscle samples after DAF administration for 2, 4 weeks, or 2 h before sacrifice or reperfusion compared with the levels in the control group (n = 4). (B) CAT in cardiac muscle samples after DAF administration for 2, 4 weeks, or 2 h before sacrifice or at reperfusion ompared with the levels in the control group (n = 4). SOD, superoxide dismutase; CAT, catalase; Ctr, control; DAF 2 h, Daflon infusion 2 h before sacrifice; DAF 2W, Daflon administration or 2 weeks; DAF 4W, Daflon administration for 4 weeks. *P < .05 compared with the respective controls.
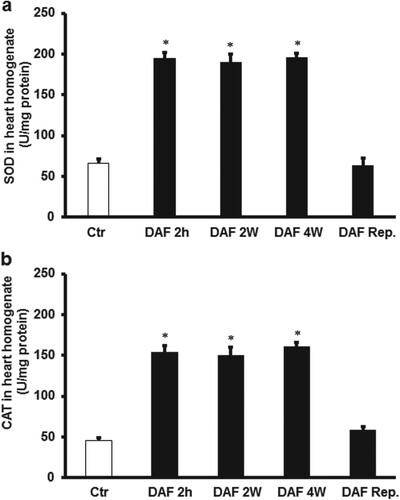
Discussion
Protection of the heart by DAF
This study demonstrates the protective effects of DAF on the heart when given before and after I/R injury. Longtime treatment of healthy hearts with DAF for 4, 2 weeks or 2 h before the animal sacrifice normalized the heart functions. This teatment also protected the I/R injury indicated by decreased infarct size, cardiac enzymes and caspase-3 levels. Surprisingly, the infusion of DAF at the start of reperfusion did not protect the heart against I/R injury (Figure ). Literature regarding the effect of DAF on I/R injury is scarce, however, pretreatment of patients with DAF 4 days before cardiac surgery procedure improved the cardiac functions and decreased the length of hospitalization (Sirlak et al. Citation2010). The identification of this protective effect reveals the potential effects of this medicine in the protection of the heart. Similarly, the results of this study are consistent with those of Sirlak and co-workers (Sirlak et al. Citation2010), although their study proved the stabilization or improvement of cardiac function in the cardiac surgery setting. Our study targeted the role of DAF in the improvement of heart function after I/R. The protection produced by DAF in the recent study seems to be by a pathway involving the blockade of proinflammatory cytokines which is supported by the findings of de Souza and colleagues (Citation2016). The neutralization of ROS which was shown previously by Nijveldt et al. (Citation2001) and increased expression of antioxidants enzymes proteins which is in line with the findings of Abdel-Rafei and his coworkers (Citation2017) are also crucial for this protection. It is noteworthy that DAF is prescribed for many vascular disease patients to provide patients protection against I/R injury.
Figure 9. Schematic representation showing the protective effects of DAF observed in this study (n = 8) for hemodynamics and (n = 4) for infarct size, antioxidants effects and anti-inflammatory. Ctr, control; Isch., ischemia; Rep., reperfusion; AF 2 h, Daflon infusion 2 h before sacrifice; DAF 2W, Daflon administration for 2 weeks; DAF 4W, Daflon administration for 4 weeks.
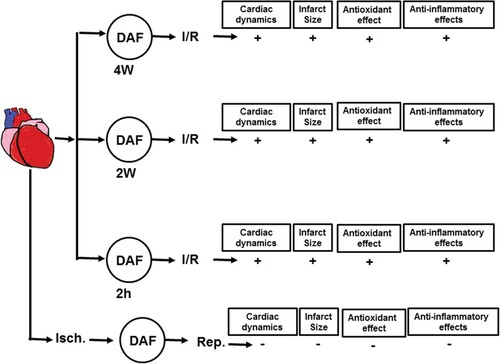
Possible pathways of DAF-mediated heart protection in this study
This study evaluated the involvement of DAF in the regulation of the inflammatory cytokines such as TNF-α, IL-1β and IL-6 in the protection of the heart against I/R injury (Figure ). Although heart resident macrophages are reported in the literature (Hulsmans et al. Citation2017; Bajpai et al. Citation2018), an extensive amount of evidence highlights the production of cytokines by cardiomyocytes when stressed for example by hypoxia (Loyer et al. Citation2018). Similar to our findings the cytokines were shown by many studies to contribute to cardiac dysfunction, cardiomyocyte necrosis and apoptosis in I/R injury (Babiker et al. Citation2011; Juggi et al. Citation2011; Babiker et al. Citation2012; Babiker et al. Citation2013). Long-term and short-term treatment with DAF resulted in a significant decrease in the levels of these cytokines. These findings are consistent with the findings reported by de Souza and his co-workers (Citation2016), who summarized the beneficial anti-inflammatory effects of flavonoids. However, a lack of effect on lowering inflammatory cytokines at reperfusion observed in this study could be due to the short period of exposure to DAF or possibly a higher dose is required for that purpose. Previously we reported that lowering TNF-α was essential to protect the heart against I/R injury (Babiker et al. Citation2011; Juggi et al. Citation2011; Babiker et al. Citation2012; Babiker et al. Citation2013), a finding which is now confirmed by this study. Lowering IL-1 and IL-6 levels is associated with reduced heart injury and cardiomyocyte apoptosis (Suzuki et al. Citation2001; Ridker et al. Citation2012; Chen et al. Citation2018). Reduced expression levels of TNF-α, IL-1β, and IL-6, observed in this study are comparable to those reported by Lui et al. (Citation2019). On the other hand, the anti-inflammatory cytokine IL-10 appeared to be a central player in the protection of the heart against I/R injury (Zhang et al. Citation2015), however, in this study, DAF treatment did not affect the IL-10 levels. This discrepancy could be due to the different protocols used in the studies or the protection seen in our study could be mediated by other anti-inflammatory cytokines like IL-4, IL-13 or TNF-β.
This study investigated the effects of DAF, on oxidant and antioxidant levels before and after I/R (Figure ). Although ROS form a basis for many diseases and pathological conditions (Sugamura and Keaney Citation2011), they are indeed important molecules in the signaling of many physiological processes (D'Autreaux and Toledano Citation2007). Some studies suggest a dose-dependent anti-redox activity of antioxidants (Trommer and Neubert Citation2005). In this study, DAF treatment resulted in a significant increase in TAS and a significant decrease in TOS in the cardiomyocytes before I/R injury. However, this scenario was not seen following I/R. This is in contrast to results reported by other research groups where ROS production was increased in the cardiomyocytes following ischemic insult and was exacerbated by reperfusion (Levraut et al. Citation2003; Braunersreuther and Jaquet Citation2012). The controversy in these results may be attributed to the presence of DAF which increased TAS levels, neutralizing the oxidative species and reducing TOS levels (Shah et al. Citation2013). Interestingly, this study showed a significant decrease in TOS levels after I/R procedure in the effluent following the administration of DAF. The decreased levels of TOS in the effluent indicate the indirect effect of DAF treatment by blocking mPTP which is responsible for ROS production (Cadenas Citation2018). It could also be due to a direct ROS neutralizing effect of DAF (Unlu et al. Citation2003). Our findings are in consensus with the antioxidant activity of DAF reported by Shah et al. (Citation2013). However, the lack of antioxidant effects of DAF was also reported (Halliwell et al. Citation2005). The protection of DAF to the heart could be by the increase of the antioxidants SOD and CAT (Abdel-Rafei et al. Citation2017). Our study is the first to evaluate the effects of DAF on I/R injury to the heart. Further studies may be required to clarify the issue of the antioxidant activity of DAF. The lack of protection to the heart by acute infusion of DAF at reperfusion could be due to the doses used in this study. However, the dose of DAF used in this study was similar to the dose used in the clinic.
Conclusions
Long-term and short-term treatment with DAF prevents the deterioration of cardiac function and ischemic injury caused by oxidative stress and inflammatory cytokines. The protective effects of DAF may be due to its antioxidant and anti-inflammatory effects. According to the result of this study, DAF may be used as a prophylactic treatment for people with a high risk of ischemia and people undergoing heart surgeries.
Acknowledgments
This study was supported by Kuwait University, Kuwait.
Data availability statement
The authors confirm that the data supporting the findings of this study are available at this data repository: https://dataverse.harvard.edu/dataset.xhtml?persistentId=doi%3A10.7910%2FDVN%2FPOK8ES&showIngestSuccess=true&version=DRAFT.
Disclosure statement
No potential conflict of interest was reported by the author(s).
Additional information
Funding
References
- Abdel-Rafei M, Amin MM, Hasan HF. 2017. Novel effect of Daflon and low-dose gamma-radiation in modulation of thioacetamide-induced hepatic encephalopathy in male albino rats. Hum Exp Toxicol. 36(1):62–81. doi: https://doi.org/10.1177/0960327116637657
- Abwainy A, Babiker F, Akhtar S, Benter IF. 2016. Endogenous angiotensin-(1-7)/Mas receptor/NO pathway mediates the cardioprotective effects of pacing postconditioning. Am J Physiol Heart Circ Physiol. 310(1):H104–H112. eng. doi: https://doi.org/10.1152/ajpheart.00121.2015
- Al-Herz W, Babiker F. 2017. Acute intravenous infusion of immunoglobulins protects against myocardial ischemia-reperfusion injury through inhibition of caspase-3. Cell Physiol Biochem. 42(6):2295–2306. doi: https://doi.org/10.1159/000480002
- Babiker F, Al-Jarallah A, Al-Awadi M. 2019. Effects of cardiac hypertrophy, diabetes, aging, and pregnancy on the cardioprotective effects of postconditioning in male and female rats. Cardiol Res Pract. 2019:3403959. doi: https://doi.org/10.1155/2019/3403959
- Babiker FA, Elkhalifa LA, Moukhyer ME. 2013. Awareness of hypertension and factors associated with uncontrolled hypertension in Sudanese adults. Cardiovasc J Afr. 24(6):208–212. doi: https://doi.org/10.5830/CVJA-2013-035
- Babiker FA, Hoteit LJ, Joseph S, Mustafa AS, Juggi JS. 2012. The role of 17-beta estradiol in ischemic preconditioning protection of the heart. Exp Clin Cardiol. 17(3):95–100. eng.
- Babiker FA, Joseph S, Juggi JS. 2011. What's new in salvage of the ischemic myocardium: estrogen postconditioning. Med Princ Pract Int J Kuwait Univ Health Sci Cent. 20(6):495–496. eng.
- Bajpai G, Schneider C, Wong N, Bredemeyer A, Hulsmans M, Nahrendorf M, Epelman S, Kreisel D, Liu Y, Itoh A, et al. 2018. The human heart contains distinct macrophage subsets with divergent origins and functions. Nat Med. 24(8):1234–1245. doi: https://doi.org/10.1038/s41591-018-0059-x
- Banerjee SK, Dinda AK, Manchanda SC, Maulik SK. 2002. Chronic garlic administration protects rat heart against oxidative stress induced by ischemic reperfusion injury. BMC Pharmacol. 2:16. doi: https://doi.org/10.1186/1471-2210-2-16
- Bellandi F, Maioli M, Gallopin M, Toso A, Dabizzi RP. 2004. Increase of myocardial salvage and left ventricular function recovery with intracoronary Abciximab downstream of the coronary occlusion in patients with acute myocardial infarction treated with primary coronary intervention. Catheter Cardiovasc Interv. 62(2):186–192. doi: https://doi.org/10.1002/ccd.20041
- Benjamin EJ, Muntner P, Alonso A, Bittencourt MS, Callaway CW, Carson AP, Chamberlain AM, Chang AR, Cheng S, Das SR, et al. 2019. Heart disease and stroke statistics-2019 update: a report from the American Heart Association. Circulation. 139(10):e56–e528. doi: https://doi.org/10.1161/CIR.0000000000000659
- Boisseau MR. 2007. Leukocyte involvement in the signs and symptoms of chronic venous disease. Perspectives for therapy. Clin Hemorheol Microcirc. 37(3):277–290.
- Bolli R, Becker L, Gross G, Mentzer R Jr, Balshaw D, Lathrop DA, NHLBI Working Group on the Translation of Therapies for Protecting the Heart from Ischemia. 2004. Myocardial protection at a crossroads: the need for translation into clinical therapy. Circ Res. 95(2):125–134. doi: https://doi.org/10.1161/01.RES.0000137171.97172.d7
- Braunersreuther V, Jaquet V. 2012. Reactive oxygen species in myocardial reperfusion injury: from physiopathology to therapeutic approaches. Curr Pharm Biotechnol. 13(1):97–114. doi: https://doi.org/10.2174/138920112798868782
- Burgoyne JR, Oka S, Ale-Agha N, Eaton P. 2013. Hydrogen peroxide sensing and signaling by protein kinases in the cardiovascular system. Antioxid Redox Signal. 18(9):1042–1052. doi: https://doi.org/10.1089/ars.2012.4817
- Burns RJ, Gibbons RJ, Yi Q, Roberts RS, Miller TD, Schaer GL, Anderson JL, Yusuf S, Investigators CS. 2002. The relationships of left ventricular ejection fraction, end-systolic volume index and infarct size to six-month mortality after hospital discharge following myocardial infarction treated by thrombolysis. J Am Coll Cardiol. 39(1):30–36. doi: https://doi.org/10.1016/S0735-1097(01)01711-9
- Cadenas S. 2018. ROS and redox signaling in myocardial ischemia-reperfusion injury and cardioprotection. Free Radic Biol Med. 117:76–89. doi: https://doi.org/10.1016/j.freeradbiomed.2018.01.024
- Canty JM Jr, Suzuki G. 2012. Myocardial perfusion and contraction in acute ischemia and chronic ischemic heart disease. J Mol Cell Cardiol. 52(4):822–831. doi: https://doi.org/10.1016/j.yjmcc.2011.08.019
- Cassar A, Holmes DR, Jr, Rihal CS, Gersh BJ. 2009. Chronic coronary artery disease: diagnosis and management. Mayo Clin Proc. 84(12):1130–1146. doi: https://doi.org/10.4065/mcp.2009.0391
- Chen X, Li X, Zhang W, He J, Xu B, Lei B, Wang Z, Cates C, Rousselle T, Li J. 2018. Activation of AMPK inhibits inflammatory response during hypoxia and reoxygenation through modulating JNK-mediated NF-kappaB pathway. Metabolism. 83:256–270. doi: https://doi.org/10.1016/j.metabol.2018.03.004
- Dare AJ, Logan A, Prime TA, Rogatti S, Goddard M, Bolton EM, Bradley JA, Pettigrew GJ, Murphy MP, Saeb-Parsy K. 2015. The mitochondria-targeted anti-oxidant MitoQ decreases ischemia-reperfusion injury in a murine syngeneic heart transplant model. J Heart Lung Transplant. 34(11):1471–1480. eng. doi: https://doi.org/10.1016/j.healun.2015.05.007
- D'Autreaux B, Toledano MB. 2007. ROS as signalling molecules: mechanisms that generate specificity in ROS homeostasis. Nat Rev Mol Cell Biol. 8(10):813–824. doi: https://doi.org/10.1038/nrm2256
- Delbarre B, Delbarre G, Calinon F. 1995. Effect of Daflon 500 mg, a flavonoid drug, on neurological signs, levels of free radicals and electroretinogram in the gerbil after ischemia-reperfusion injury. Int J Microcirc Clin Exp. 15(Suppl 1):27–33. doi: https://doi.org/10.1159/000179092
- de Souza M, Cyrino FZ, Mayall MR, Virgini-Magalhaes CE, Sicuro FL, Carvalho JJ, Verbeuren TJ, Bouskela E. 2016. Beneficial effects of the micronized purified flavonoid fraction (MPFF, daflon(R) 500 mg) on microvascular damage elicited by sclerotherapy. Phlebology. 31(1):50–56. eng. doi: https://doi.org/10.1177/0268355514564414
- Dinarello CA. 2000. Proinflammatory cytokines. Chest. 118(2):503–508. doi: https://doi.org/10.1378/chest.118.2.503
- Erel O. 2004. A novel automated direct measurement method for total antioxidant capacity using a new generation, more stable ABTS radical cation. Clin Biochem. 37(4):277–285. doi: https://doi.org/10.1016/j.clinbiochem.2003.11.015
- Erel O. 2005. A new automated colorimetric method for measuring total oxidant status. Clin Biochem. 38(12):1103–1111. doi: https://doi.org/10.1016/j.clinbiochem.2005.08.008
- Ferrera R, Benhabbouche S, Bopassa JC, Li B, Ovize M. 2009. One hour reperfusion is enough to assess function and infarct size with TTC staining in Langendorff rat model. Cardiovasc Drugs Ther. 23(4):327–331. doi: https://doi.org/10.1007/s10557-009-6176-5
- Gersh BJ, Stone GW, White HD, Holmes DR Jr. 2005. Pharmacological facilitation of primary percutaneous coronary intervention for acute myocardial infarction: is the slope of the curve the shape of the future? JAMA. 293(8):979–986. doi: https://doi.org/10.1001/jama.293.8.979
- Halliwell B, Rafter J, Jenner A. 2005. Health promotion by flavonoids, tocopherols, tocotrienols, and other phenols: direct or indirect effects? Antioxidant or not? Am J Clin Nutr. 81(1 Suppl):268S–276S. doi: https://doi.org/10.1093/ajcn/81.1.268S
- Hausenloy DJ, Yellon DM. 2013. Myocardial ischemia-reperfusion injury: a neglected therapeutic target. J Clin Invest. 123(1):92–100. doi: https://doi.org/10.1172/JCI62874
- Hulsmans M, Clauss S, Xiao L, Aguirre AD, King KR, Hanley A, Hucker WJ, Wulfers EM, Seemann G, Courties G, et al. 2017. Macrophages facilitate electrical conduction in the heart. Cell. 169(3):510–522.e520. doi: https://doi.org/10.1016/j.cell.2017.03.050
- Jong WM, Ten Cate H, Linnenbank AC, de Boer OJ, Reitsma PH, de Winter RJ, Zuurbier CJ. 2016. Reduced acute myocardial ischemia-reperfusion injury in IL-6-deficient mice employing a closed-chest model. Inflamm Res. 65(6):489–499. doi: https://doi.org/10.1007/s00011-016-0931-4
- Juggi JS, Hoteit LJ, Babiker FA, Joseph S, Mustafa AS. 2011. Protective role of normothermic, hyperthermic and estrogen preconditioning and pretreatment on tumour necrosis factor-alpha-induced damage. Exp Clin Cardiol. 16(2):e5–e10. eng.
- Khalaf A, Babiker F. 2016. Discrepancy in calcium release from the sarcoplasmic reticulum and intracellular acidic stores for the protection of the heart against ischemia/reperfusion injury. J Physiol Biochem. 72(3):495–508. doi: https://doi.org/10.1007/s13105-016-0498-0
- Kobo PI, Ayo JO, Aluwong T, Zezi AU, Maikai V, Ambali SF. 2014. Flavonoid mixture ameliorates increase in erythrocyte osmotic fragility and malondialdehyde concentration induced by Trypanosoma brucei brucei-infection in Wistar rats. Res Vet Sci. 96(1):139–142. eng. doi: https://doi.org/10.1016/j.rvsc.2013.10.005
- Levraut J, Iwase H, Shao ZH, Vanden Hoek TL, Schumacker PT. 2003. Cell death during ischemia: relationship to mitochondrial depolarization and ROS generation. Am J Physiol Heart Circ Physiol. 284(2):H549–H558. doi: https://doi.org/10.1152/ajpheart.00708.2002
- Lim SH. 2017. Larch arabinogalactan attenuates myocardial injury by inhibiting apoptotic cascades in a rat model of ischemia-reperfusion. J Med Food. 20(7):691–699. doi: https://doi.org/10.1089/jmf.2016.3886
- Liu H, Li S, Jiang W, Li Y. 2019. MiR-484 protects rat myocardial cells from ischemia-reperfusion injury by inhibiting caspase-3 and caspase-9 during apoptosis. Korean Circ J. 50(3):250–263. doi: https://doi.org/10.4070/kcj.2019.0107
- Loyer X, Zlatanova I, Devue C, Yin M, Howangyin KY, Klaihmon P, Guerin CL, Kheloufi M, Vilar J, Zannis K, et al. 2018. Intra-cardiac release of extracellular vesicles shapes inflammation following myocardial infarction. Circ Res. 123(1):100–106. doi: https://doi.org/10.1161/CIRCRESAHA.117.311326
- Lyseng-Williamson KA, Perry CM. 2003. Micronised purified flavonoid fraction: a review of its use in chronic venous insufficiency, venous ulcers and haemorrhoids. Drugs. 63(1):71–100. doi: https://doi.org/10.2165/00003495-200363010-00005
- Marquez Loza A, Elias V, Wong CP, Ho E, Bermudez M, Magnusson KR. 2017. Effects of ibuprofen on cognition and NMDA receptor subunit expression across aging. Neuroscience. 344:276–292. doi: https://doi.org/10.1016/j.neuroscience.2016.12.041
- Mulvihill NT, Foley JB. 2002. Inflammation in acute coronary syndromes. Heart. 87(3):201–204. doi: https://doi.org/10.1136/heart.87.3.201
- Nijveldt RJ, van Nood E, van Hoorn DE, Boelens PG, van Norren K, van Leeuwen PA. 2001. Flavonoids: a review of probable mechanisms of action and potential applications. . Am J Clin Nutr. 74(4):418–425. eng. doi: https://doi.org/10.1093/ajcn/74.4.418
- Pagliaro P, Penna C. 2015. Redox signalling and cardioprotection: translatability and mechanism. Br J Pharmacol. 172(8):1974–1995. doi: https://doi.org/10.1111/bph.12975
- Palazzo AL, Evensen E, Huang YW, Cesano A, Nolan GP, Fantl WJ. 2011. Association of reactive oxygen species-mediated signal transduction with in vitro apoptosis sensitivity in chronic lymphocytic leukemia B cells. PloS one. 6(10):e24592. doi: https://doi.org/10.1371/journal.pone.0024592
- Penna C, Mancardi D, Rastaldo R, Pagliaro P. 2009. Cardioprotection: a radical view free radicals in pre and postconditioning. Biochim Biophys Acta. 1787(7):781–793. doi: https://doi.org/10.1016/j.bbabio.2009.02.008
- Raffetto JD., Eberhardt RT., Dean SM., Ligi D., Mannello F. 2016. Pharmacologic treatment to improve venous leg ulcer healing. J Vascular Surg Venous Lymphat Disord. 4(3):371–374. english. doi: https://doi.org/10.1016/j.jvsv.2015.10.002
- Ramelet AA. 2001. Clinical benefits of Daflon 500 mg in the most severe stages of chronic venous insufficiency [Review]. Angiology. 52(Suppl 1):S49–S56. eng. doi: https://doi.org/10.1177/0003319701052001S07
- Ridker PM, Howard CP, Walter V, Everett B, Libby P, Hensen J, Thuren T, Group CPI. 2012. Effects of interleukin-1beta inhibition with canakinumab on hemoglobin A1c, lipids, C-reactive protein, interleukin-6, and fibrinogen: a phase IIb randomized, placebo-controlled trial. Circulation. 126(23):2739–2748. doi: https://doi.org/10.1161/CIRCULATIONAHA.112.122556
- Shah S, Mohan M, Kasture S, Ballero M, Maxia A, Sanna C. 2013. Protective effect of Hypericum hircinum on doxorubicin-induced cardiotoxicity in rats. Nat Prod Res. 27(16):1502–1507. doi: https://doi.org/10.1080/14786419.2012.724409
- Sirlak M, Akar AR, Eryilmaz S, Cetinkanat EK, Ozcinar E, Kaya B, Elhan AH, Ozyurda U. 2010. Micronized purified flavonoid fraction in pretreating CABG patients. Tex Heart Inst J. 37(2):172–177.
- Sugamura K, Keaney JF Jr. 2011. Reactive oxygen species in cardiovascular disease. Free Radic Biol Med. 51(5):978–992. doi: https://doi.org/10.1016/j.freeradbiomed.2011.05.004
- Suzuki K. 2009. Anti-oxidants for therapeutic use: why are only a few drugs in clinical use? [Review]. Adv Drug Deliv Rev. 61(4):287–289. eng. doi: https://doi.org/10.1016/j.addr.2009.03.002
- Suzuki K, Murtuza B, Smolenski RT, Sammut IA, Suzuki N, Kaneda Y, Yacoub MH. 2001. Overexpression of interleukin-1 receptor antagonist provides cardioprotection against ischemia-reperfusion injury associated with reduction in apoptosis. Circulation. 104(12 Suppl 1):I308–I303. doi: https://doi.org/10.1161/hc37t1.094871
- Techapiesancharoenkij N, Fiala JL, Navasumrit P, Croy RG, Wogan GN, Groopman JD, Ruchirawat M, Essigmann JM. 2015. Sulforaphane, a cancer chemopreventive agent, induces pathways associated with membrane biosynthesis in response to tissue damage by aflatoxin B1. Toxicol Appl Pharmacol. 282(1):52–60. doi: https://doi.org/10.1016/j.taap.2014.11.004
- Trommer H, Neubert RH. 2005. The examination of polysaccharides as potential antioxidative compounds for topical administration using a lipid model system. Int J Pharm. 298(1):153–163. doi: https://doi.org/10.1016/j.ijpharm.2005.04.024
- Unlu A, Sucu N, Tamer L, Coskun B, Yucebilgic G, Ercan B, Gul A, Dikmengil M, Atik U. 2003. Effects of Daflon on oxidative stress induced by hindlimb ischemia/reperfusion. Pharmacol Res. 48(1):11–15.
- Yu M, Wen N, Wenzhong Z, Yuanchang X, Xiaoming D, Yongjin L. 2010. Effect of repeated ischaemic preconditioning on TLR4 and proinflammatory cytokines TNF-alpha and IL-1beta in myocardial ischaemia-reperfusion injury in a rat model. Arch Med Sci. 6(6):843–847. doi: https://doi.org/10.5114/aoms.2010.19289
- Zhang ML, Li ZP, Xiao H. 2007. [Different expressions of inflammatory cytokines in two types of cardiac hypertrophy in rats]. Beijing da Xue Xue Bao Yi Xue Ban = J Peking Univ Health Sci. 39(6):570–575.
- Zhang W, Xing B, Yang L, Shi J, Zhou X. 2015. Icaritin attenuates myocardial ischemia and reperfusion injury via anti-inflammatory and anti-oxidative stress effects in rats. Am J Chin Med. 43(6):1083–1097. doi: https://doi.org/10.1142/S0192415X15500627