ABSTRACT
The total phospholipid fraction (TPF) has anti-inflammatory properties and reduces apoptosis in animal models. Our objective was to assess the effects of TPF on pressure ulcers in a mouse model of cutaneous ischemic-reperfusion (I/R) injury. Cutaneous I/R injury was created by trapping the dorsal skin between two magnetic disks for 12 h, followed by disk removal. TPF administration in I/R areas at the beginning of reperfusion significantly inhibited the formation of pressure ulcers. The number of neutrophils and M1 macrophages and the levels of proinflammatory mediators MCP-1, IL-1β, IL-6, and TNF-α in the wound area were reduced upon TPF treatment. Collagen synthesis and α-SMA-positive microvessel density significantly increased in the wounds of TPF-treated mice. Additionally, TPF inhibited cutaneous I/R injury-induced formation and accumulation of apoptotic cells. Moreover, TPF increased the expression of filaggrin and involucrin. In conclusion, TPF may inhibit cutaneous I/R injury-induced formation of pressure ulcers by regulating inflammation, collagen production, angiogenesis, and skin barrier restoration. Therefore, exogenous application of TPF might have therapeutic potential with respect to cutaneous I/R injuries like decubitus ulcers.
1. Introduction
Pressure ulcers, also known as decubitus ulcer, occur frequently in the elderly and in home-care patients who require extended periods of immobilization and bed rest due to illness or treatment. It is also common in patients who incur exercise-related injuries that affect their sensory or cognitive abilities. Decubitus ulcer is therefore an important health problem, and its incidence is rising with the increase in the elderly population due to improvements in medical science and longevity (Rogenski and de Gouveia Santos Citation2005). Pressure ulcers are caused by persistent pressure exerted on the bony prominences of the body, causing impaired blood circulation and tissue necrosis (ischemic state of the skin and subcutaneous tissue) (Reddy et al. Citation2006). Different tissues have different sensitivities to pressure, and muscles can withstand pressure for the longest time. Skin damage occurs only after severe muscle necrosis; in fact, necrosis never appears on the skin without prior damage to the muscle (Maklebust Citation1987). Therefore, necrosis most commonly occurs in the part of the tissue touching the bone, rather than on the skin surface.
New materials and techniques have been developed for dressing pressure ulcers. Silver-based dressings, including Aquacell®Ag (Jones et al. Citation2004), ACTICOAT® (Dowsett Citation2003), and SILVADENE® (silver sulfadiazine) (Chuangsuwanich et al. Citation2011; Suman et al. Citation2012), can be applied on open pressure ulcer wounds. In the ionic state, silver (Ag) has a broad antibacterial activities and suppresses bacterial growth in the wound effluent. Chitosan is also effectively treats pressure ulcers by facilitating growth factor migration through the wound (Park et al. Citation2009). These materials are continuously being developed to improve absorption, reduce number of dressings, and shortening the treatment time, and are expected to be widely used in the future.
Total phospholipid fraction (TPF) is an alveolar surfactant containing various phospholipids, including phosphatidylcholine (PC) and phosphatidylserine (PS), DPPC (dipalmitoyl phosphatidylcholine) (Shaheen et al. Citation2009). Phospholipids and their metabolites have been demonstrated to have anti-inflammatory properties in various diseases associated with leukocyte activation, such as reperfused ischemia (Duan and Karmazyn Citation1990) and oxidative stress (Aleynik and Lieber Citation2003). Frog skin lipids including phospholipids exhibited a dose-dependent acceleration of wound healing through anti-inflammatory activity (Babu et al. Citation2010). PC, a main component of surfactant lipid, exerts anti-inflammatory effects by inhibiting tumor necrosis factor-α (TNF-α)-induced actin assembly, MAPK activation, and NF-kB transport in human intestinal epithelial cells (Treede et al. Citation2007). PS, another component of surfactant lipids, reduces the levels of pro-inflammatory molecules such as nitric oxide, IL-1β, and TNF-α in LPS-activated microglia and induces the ingestion of apoptotic cells by macrophages through phosphatidylserine-specific receptor (De Simone et al. Citation2002). Lipid-rich surfactant preparations and the major surfactant DPPC markedly downregulated IL-8 expression by inhibiting TLR4 translocation into membrane lipid raft microdomains in LPS-induced A549 cells (Abate et al. Citation2010). Additionally, TPF suppresses the production of IL-2, IL-4, and IgE, and promotes epidermal tissue regeneration in an atopic dermatitis animal model (Moon et al. Citation2012). TPF also inhibits macrophage recruitment and exhibits anti-inflammatory and anti-nociceptive effects (Jung et al. Citation2012; Moon et al. Citation2012).
In this study, we aimed to investigate the effects mechanisms of TPF on cutaneous I/R injury that mimic pressure ulcers and to clarify the underlying mechanisms of the protective effect of TPF on cutaneous I/R injury.
2. Material and methods
2.1. Animals and ethical approval
Seven-week-old male C57BL/6 mice were purchased from Orient Bio (Seongnam, Korea). Mice were fed standard solid feed (antibiotic-free), provided ad libitum to water, and housed in sawdust-lined cages in an air-conditioned environment with a 12-h light/dark cycle. Mice were segregated into four groups (n = 8), negative control (saline), positive control (silver sulfadiazine, SS), TPF 1%, and TPF 2%. All animal procedures were approved by the Institutional Animal Care and Use Committee of Chung-Ang University (201700028) and conformed to all the applicable National Institutes of Health guidelines.
2.2. Ischemia/reperfusion (I/R)-induced injury model and TPF administration
The cutaneous I/R model was generated according to previously published protocols (Stadler et al. Citation2004; Saito et al. Citation2008). Briefly, the dorsal skin (including epidermis, dermis, and hypodermis) was gently pulled up and trapped between two round magnetic disks (12-mm diameter; 113 mm2; 5-mm thick; 2.4 g average weight; 1000 G magnetic force) (NSmagnet, Seoul, Korea). For the ‘decubitus ulcer-like cutaneous I/R model', dorsal skin was trapped for 12 h (ischemic period), followed by a release period of 12 h. All mice developed two circular ulcers separated by a bridge of normal skin. Mice were treated with a wet gauze dressing containing 50 µl of TPF (10 mg/ml or 20 mg/ml), SS (10 mg/ml), or saline, twice daily, until euthanization. For analysis, wound sites were photographed digitally at the indicated time points, and analyzed using Image J (version 1.51, NIH, Bethesda, MD). TPF was manufactured by Lipobiomed Co. (Gangwon, Korea).
2.3. Quantitative RT-PCR
Total RNA was extracted from dorsal skin using TRIzol (Invitrogen, CA, USA). First-strand cDNA was synthesized from the total RNA using PrimeScriptTM RT master mix (Takara, Tokyo, Japan). cDNA was subjected to real-time PCR using qPCR 2x PreMIX SYBR (Enzynomics, Seoul, Korea) on a CFX-96 thermocycler (Bio-Rad, Hercules, CA, USA), with the following conditions: 10 min at 95°C and 40 cycles of 95°C for 15 s and 60°C for 30 s. Expression data were calculated based on the cycle threshold (Ct) value using the 2-ΔΔCt method of quantification (Livak and Schmittgen Citation2001). GAPDH was used for normalization.
2.4. Immunoblotting
Wound skin samples were homogenized (using TissueLyser II, QIAGEN, Tokyo, Japan) in RIPA buffer (50 mM Tris, 150 mM NaCl, 0.1% SDS, 0.5% Na deoxycholate, Triton X-100, and protease inhibitors). Homogenates were incubated on ice for 15 min and centrifuged (GYROZEN, 1730MR, Korea) at 18,000×g for 20 min at 4°C. Total protein concentration was quantified for the collected supernatants using BCA Protein Assay Kit (Thermo Fisher Scientific, Waltham, MA, USA). Proteins (20 mg/sample) were separated on 12% polyacrylamide gels and transferred onto polyvinylidene difluoride membranes (Immobilon, Millipore, Bedford, MA, USA). After blocking with 5% skim milk prepared in Tris-buffered saline containing 0.5% Tween 20, the membranes were probed with primary antibodies against CD68 (ab955, Abcam, Cambridge, UK), iNOS (BD610328, BD Transduction Laboratories, Franklin Lakes, NJ, USA), Arginase (Arg)-1 (PA5-85267, Thermo Scientific),total caspase-3 (#9662, Cell Signaling Technology, Inc., Danvers, MA, USA), Bax (sc-7480, Santa Cruz Biotechnology, Dallas, TX, USA), Bcl-2 (sc-492, Santa Cruz Biotechnology), and β-actin (sc-1616, Santa Cruz Biotechnology) at 4°C for 12 h, followed by incubation with horseradish peroxidase-conjugated secondary antibodies (Vector Labs, Inc., Burlingame, CA, USA) at room temperature for 1 h. Bound antibodies were detected using a SuperSignal™ West Pico Chemiluminescent Substrate (PIERCE Biotechnology Inc., Rockford, IL, USA),and assessed using a ChemiDoc™ XRS+ System (Bio-RAD, Hercules, CA, USA).
2.5. Histological analysis
Wound skin samples were collected and immediately fixed with 10% paraformaldehyde – prepared in PBS – overnight at 4°C. The samples were dehydrated, embedded in paraffin, and sectioned using a microtome. Serial transverse sections (5-µm) were then transferred to treated slides (Thermo Fisher Scientific), deparaffinized, and stained with hematoxylin and eosin (DAKO, Carpinteria, CA, USA), Trichrome Stain Kit (Modified Masson’s; ScyTek Laboratories, Inc., Logan, UT, USA), or Sirius red (ab150681, Abcam). Paraffin-embedded tissue samples were deparaffinized, rehydrated, and subjected to antigen retrieval using Trilogy (1:20, 920P-06-RUO, CELL MARQUE, California, USA). The sections were treated with 3% H2O2 for 30 min at room temperature – to terminate endogenous peroxidase activity – and then blocked using 10% normal serum with 1% bovine serum albumin (BSA) in PBST (0.1% Tween-20). Immunohistochemical staining and analysis of sections were performed as described previously (Uchiyama et al. Citation2015). For immunohistochemistry, slides were incubated with antibodies against myeloperoxidase (MPO) (RB-373, Thermo Scientific), CD68 (ab955, Abcam), and α-smooth muscle actin (α-SMA) (ab5694, Abcam). After washing, samples were probed with biotinylated goat anti-mouse IgG antibody (cat. no. BP-9200; 1:1,000; Vector Laboratories) and biotinylated goat anti-rabbit IgG antibody (cat. no. BP-9500; 1:1,000; Vector Laboratories) (4 h, room temperature), plus a streptavidin-peroxidase complex (Vector Laboratories, Inc.) and brown FAST DAB (Thermo Fisher Scientific, Inc.) staining. After counterstaining with Harris hematoxylin counterstain (Sigma, St. Louis, MO, USA), the sections were dehydrated and mounted on slides. All stained sections were examined by light microscopy (DM750, Leica, Wetzlar, Germany) to assess the histological changes. For immunofluorescence, the gastrocnemius muscle was probed with primary antibodies against iNOS (BD610328, BD Transduction Laboratories) and Arg-1 (PA5-85267, Thermo Scientific) overnight at 4°C, followed by incubation with anti-mouse IgG-FITC (sc2359, Santa Cruz Biotechnology) and anti-rabbit IgG-FITC (sc-2359, Santa Cruz Biotechnology) at 37C for 1 h. Slides were washed with 1x TBS and mounted in fluorescent mounting medium containing DAPI (Golden Bridge International Inc., Mukilteo, WA, USA). Images were acquired on a confocal microscope (Leica DMI400; Leica, Wetzlar, Germany).
2.6. Measurement of apoptosis by TUNEL assay
TUNEL assay was performed on skin wound tissue using the Dead End Fluorometric In Situ Cell Death Detection Kit (Promega Corporation) according to the manufacturer’s protocol (Kyrylkova et al. Citation2012). Images were acquired on a light microscope (DM750, Leica).
2.7. Statistical analysis
All quantitative data are presented as the mean ± standard deviation (SD) for three independent experiments. Statistical analyses were performed using SPSS software (SPSS Inc., Chicago, IL, USA). Analysis of variance (ANOVA) was used for multiple comparisons. Differences between two groups were evaluated using a paired t-test. Significant values were *p < 0.05 and **p < 0.01.
3. Results
3.1. TPF prevents pressure ulcer formation following cutaneous ischemia/reperfusion (I/R) injury in mice
I/R injury-induced cutaneous pressure ulcers were evaluated in C57BL/6 mice using a simple, reproducible, and non-invasive model (Figure (A)) (Stadler et al. Citation2004; Saito et al. Citation2008). Following I/R injury, the pressure ulcer sites were dressed with 50 µl of saline, TPF (10 mg/ml or 20 mg/ml), and SS (10 mg/ml). Comparison of the wound areas shows that TPF treatment significantly inhibited cutaneous pressure ulcer formation. Four days after the dressing, the size of the pressure ulcer area in TPF-treated mice was ∼70% of that in the control mice, showing faster recovery (Figure (B,C); SS: 93%, 1% TPF: 75%, 2% TPF: 73%). Wound closure time was longer in control than that in TPF-treated mice, indicating a significant difference in the recovery rate. Until day 16, TPF showed significantly faster wound closure than SS, and there was no significant difference between 1% and 2% TPF. Subsequently, the trans-epidermal water loss (TEWL) was measured to determine the extent of moisture evaporation from the wound site (Figure (D)). In the case of pressure ulcers or burns – when keratin is eliminated or its function impaired – water evaporation increases (Harrow and Mayrovitz Citation2014). TEWL did not differ in the initial stage of dressing, but tended to decrease more in the TPF-treated group compared to that in the control group 6 days after pressure ulcer recovery. Moreover, on day 8, 2% TPF showed a significant wound recovery effect compared to SS. Thus, TPF partially prevents cutaneous pressure ulcer formation after I/R injury.
Figure 1. Topical application of TPF protects against ulcerogenesis in cutaneous I/R injury mouse model. (A) Schematic illustration of an I/R pressure ulcer model. After shaving the dorsal skin of the mice and conducting the I/R cycle twice, mice were treated with control (PBS) or dressed with 50 µl of the test compounds (SS10 mg/ml, TPF 10 mg/ml, and TPF 20 mg/ml) twice daily, and evaluated for pressure ulcer healing for 20 days. (B) Pressure ulcer site after I/R injury. (C) Changes in the size of the pressure ulcer site after I/R (ulcer size after I/R injury assigned a value of 100%). (D) TEWL at the wound area after I/R injury. Data represent mean ± S.D. *p < 0.05 and **p < 0.01 vs. control, #p < 0.05 and ##p < 0.01 vs. silver sulfadiazine.
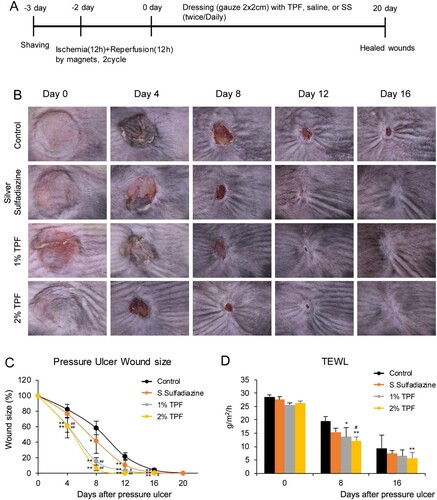
3.2. TPF reduces macrophage infiltration at the wound site after I/R injury in mice
Neutrophils and macrophages regulate inflammation and angiogenesis and are involved in I/R injury induction (Saito et al. Citation2008). Therefore, the effects of TPF on neutrophil and macrophage infiltration and activity after cutaneous I/R injury were analyzed. Four days after dressing, dermal edema and inflammatory cell infiltration were observed in the hypodermal gland. Anti-MPO and anti-CD68 staining revealed that the number of neutrophils and macrophages was significantly reduced in the experimental (SS, 1% TPF, and 2% TPF) groups compared to that in the control group, suggesting that TPF can modulate the accumulation or function of these cells (Figure (A,C)). In addition, 2% TPF treatment was more effective in inhibiting neutrophil and macrophage infiltration compared to SS. To examine the changes in the M1 and M2 macrophages at the I/R injury site, iNOS (M1 marker) and Arg-1 (M2 marker) mRNA levels were assessed in each group (Figure (D)). iNOS mRNA levels – which increased after I/R injury – were significantly reduced in the SS, 1% TPF, and 2% TPF groups. Instead, Arg-1 mRNA levels decreased slightly, but not significantly. Immunoblot assay showed a similar tendency of iNOS and Arg-1 proteins (Figure (D)). Subsequently, tissue infiltration was evaluated by immunofluorescence. M1 and M2 macrophage counts significantly increased at the early stage of I/R injury. Conversely, upon SS and TPF treatment, the total number of iNOS+ M1 macrophages significantly decreased while that of Arg-1+ M2 macrophages decreased slightly (Figure (B,C)). On comparing the changes in iNOS and Arg-1 expression, the macrophage count reduction upon TPF treatment at the early stages of pressure ulcer development seems mainly due to changes in M1 macrophage counts. Next, the effect of TPF on the levels of proinflammatory cytokines and chemokines (MCP-1, IL-1β, IL-6, and TNFα) secreted by M1 macrophages was investigated using real-time PCR. SS, 1% TPF, and 2% TPF dressing significantly lowered the mRNA levels of the inflammatory cytokines and chemokines that were increased in response to I/R injury (Figure (E)). Collectively, results suggest that TPF reduces I/R-injury-induced tissue infiltration of neutrophils and macrophages and alleviates skin inflammation.
Figure 2. Topical application of TPF inhibits macrophage infiltration after cutaneous I/R injury. (A) Cutaneous pressure ulcer site into which the neutrophils and macrophages infiltrated 4 days after I/R injury, were stained with MPO and CD68 antibodies. Scale bar, 100 µm. (B) Cutaneous pressure ulcer site stained with iNOS (M1 macrophage) and Arg-1 (M2 macrophage) antibodies. Scale bar, 100 µm. (C) Neutrophil (MPO+) and macrophage (CD68+) cell counts. (D) Quantitation of iNOS and Arg-1 expression using RT-PCR and immunoblot 4 days after I/R injury. (E) Quantitation of the mRNA levels of MCP-1, IL-1β, IL-6, and TNFα 4 days after I/R injury. Scale bar: 100 µm. Data represent mean ± S.D. *p < 0.05 and **p < 0.01 vs. control, #p < 0.05 and ##p < 0.01 vs. silver sulfadiazine, $p < 0.01 vs. 1% TPF.
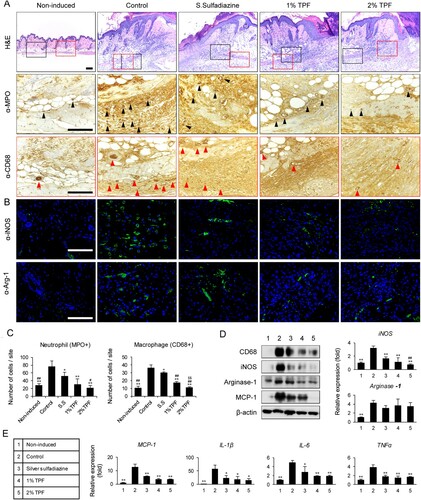
3.3. TPF inhibits apoptosis after I/R injury in mice
TUNEL staining was performed on the skin tissue to verify the effect of TPF on apoptosis of skin cells damaged by I/R injury. Four days after dressing, the number of apoptotic cells in 1 or 2% TPF-treated groups was reduced compared to that in control mice (Figure (A,B)). Additionally, TPF treatment reduced the activity of Bax and cleaved caspase-3 – apoptotic markers – induced upon I/R injury (Figure (C)). Again, the effect of 2% TPF showed a higher statistical significance. Thus, TPF can inhibit cutaneous-I/R-injury-induced apoptosis and accumulation of apoptotic cells.
Figure 3. Topical application of TPF protects against apoptosis after cutaneous I/R injury. (A) H&E and TUNEL staining of cutaneous pressure ulcer 4 days after I/R injury. Scale bar, 100 µm. (B) Number of apoptotic cells in the cutaneous pressure ulcer site 4 days after I/R injury. (C) Bax and cleaved caspase-3 expression quantified by immunoblot 4 days after I/R injury. Scale bar: 100 µm. Data represent mean ± S.D. Significant values were *p < 0.05 and **p < 0.01 vs. control.
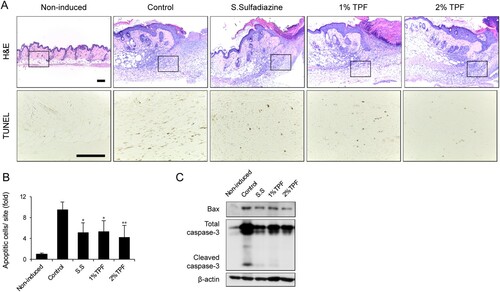
3.4. TPF promotes fibrous tissue recovery and angiogenesis at the wound site after I/R injury in mice
Next, the effect of TPF on fibrous tissue recovery was evaluated on days 12 after cutaneous I/R injury. By day 12, the epidermis had completely regenerated in TPF-treated groups, edema and bleeding were almost recovered (Figure (A)). Masson’s trichrome and Picro-Sirius red were used to compare the collagen content in the dermal tissue (marker of fibrous tissue recovery). Masson’s trichrome staining showed that dermal fibrous tissue levels increased in the SS and TPF groups compared to that in the control group (Figure (A)). Normal dermis contains ∼80% type I collagen and 20% type III collagen. Generally, 24 h after wounding, the infiltrated fibroblasts synthesize and secrete type I and III collagen, and form new matrix. Granulation tissues express higher levels of type III collagen than normal tissues. The proportion of type I collagen increases as the granulation tissue becomes closer to normal tissue. In the control group, the ratio of thin, green, and yellow type III collagen was relatively high in the upper part of the dermis. However, in groups dressed with SS, 1% TPF, and 2% TPF, a thicker, mature red, reddish-yellow type I collagen fiber with cross-configuration was formed in the deep layer. This suggests that these agents induce rapid recovery of normal skin, while promoting tissue remodeling at the later stages of the healing in I/R injury-induced cutaneous pressure ulcers.
Figure 4. Topical application of TPF accelerates fibrous tissue and skin barrier recovery after cutaneous I/R injury. (A) Cutaneous pressure ulcer site stained with H&E, Masson’s trichome, picro-sirius red, and α-SMA antibody 12 days after I/R injury. Scale bar, 100 µm. (B) Cutaneous pressure ulcer site stained with H&E, and filaggrin and involucrin antibodies 16 days after I/R injury. Scale bar, 100 µm.
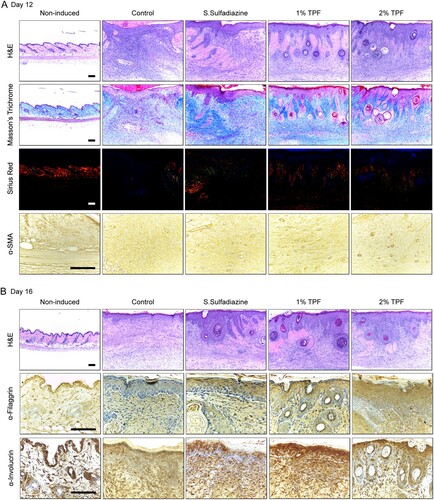
Moreover, α-SMA immunostaining showed microvessels mainly in the dermal papillary layer and in the deep part near the ulcer site (Figure (A)). α-SMA-positive microvessel density significantly increased in the experimental groups compared to that in the control group.
3.5. TPF improves skin barrier formation after I/R injury in mice
Immunohistochemistry was therefore performed to investigate the status of the recovered epithelium 16 days after I/R injury (Figure (B)). Treatment with SS, 1% TPF, and 2% TPF increased the expression of filaggrin and involucrin – differentiation markers of epithelial cells – compared to that in the control group (Figure (B)). Therefore, 1 and 2% TPF dressing effectively recovered normal skin by improving the skin barrier through induction of keratinocyte differentiation and rapid regeneration of the epidermal layer.
4. Discussion
I/R injury induces inflammation via white blood cell and macrophage infiltration and inflammatory cytokine production, and causes endothelial dysfunction, capillary stenosis, vascular infarction, vasospasm, etc. Such vascular damage induces hypoxia and oxidative stress in the tissues, leading to apoptosis and tissue necrosis (Carroll and Esclamado Citation2000; Kasuya et al. Citation2014). The purpose of this study was to investigate the role of TPF with respect to I/R-injury-induced pressure ulcers. TPF dressing increased wound healing rate, partially improved TEWL, and showed an effect similar to or better than that of SS (widely used in pressure ulcer treatment) (Chuangsuwanich et al. Citation2011; Suman et al. Citation2012). Specifically, 2% TPF showed higher efficacy than 1% TPF.
TPF rapidly cured I/R-injury-induced ulcers via (i) inhibition of neutrophil, and macrophage infiltration in the I/R injury site, particularly inhibition of M1 macrophages; (ii) inhibition of proinflammatory cytokine synthesis; (iii) protection of cells from apoptosis; (iv) regeneration of fibrous tissue through increased collagen synthesis; (v) enhanced angiogenesis; and (vi) improvement of the skin barrier via induction of keratinocyte differentiation.
Neutrophil infiltration in response to reactive oxygen species (ROS) leads to a chronic inflammatory state that can delay wound repair. Abnormal healing may also cause fibrosis, which is often associated with increase in mast cell counts. Mast cells generally increase during wound healing and cause inflammation by releasing granules filled with enzymes, histamine, and active amines (Artuc et al. Citation1999). Active amines cause peripheral blood vessel leakage, thereby allowing mononuclear cells to rapidly migrate to the injury site.
Local skin microenvironment promotes the development of classically activated (M1) and alternatively activated (M2) macrophages (Lawrence and Natoli Citation2011; Ferrante and Leibovich Citation2012). M1 macrophages infiltrate at the early stage of tissue damage, and secrete proinflammatory mediators, including MCP-1, NO, IL-1, IL-6, IL-12, and TNFα (Mosser Citation2003; Saito et al. Citation2008; Lawrence and Natoli Citation2011). Our results indicate that TPF treatment inhibits granulocyte and M1 macrophage infiltration and skin inflammation induced by proinflammatory mediators. M2 macrophages are crucial during the early and intermediate stages, and are involved in relieving inflammation and promoting tissue repair (Mosser Citation2003; Lawrence and Natoli Citation2011). M1 macrophage percentage can be reduced by M2 macrophage activation. Here, M2 macrophage percentage was not increased by TPF treatment. Therefore, further studies are needed to determine the effect of TPF on M1/M2 macrophages.
I/R-injury-induced ROS may induce skin cell apoptosis and secondary necrosis-induced inflammatory response (Miksa et al. Citation2009; Aziz et al. Citation2011). TPF treatment reduced the number of apoptotic cells induced by I/R injury, suggesting that TPF increases the phagocytosis and clearance of apoptotic cells after cutaneous injury, thereby preventing their accumulation. Therefore, as well as curing pressure ulcer, TPF exerts protective effects against apoptosis and necrosis. Exogenous porcine surfactants also reduce LPS-induced oxidative stress and apoptosis (Mittal and Sanyal Citation2009).
In an animal model of carrageenan-induced pleurisy used to study inflammation, diets rich in PC, phosphatidylethanolamine, and N-acylphosphatidylethanolamine reduced leukocyte responses and inhibited the activity of pulmonary proinflammatory enzymes (Eros et al. Citation2009). It played an important role in alleviating the inflammatory reactions involved. This result shows that a phospholipid mixture such as TPF analyzed in the current study could play a positive role in anti-inflammation. Furthermore, DPPC was found to modulate the anti-inflammatory activity by increasing the level of anti-inflammatory PGE2 upon phosphorylation of COX-2 by the cyclic AMP response element binding protein (CREB) in monocytes (Morris et al. Citation2008). In addition, Arachidonoyl-lysoPC (Hung et al. Citation2009) and docosahexaenoyl-lysoPC (Huang et al. Citation2010) were found to exert anti-inflammatory effects through inhibition of the plasma leakage in mouse models of zymosan A-induced peritonitis. This indicates that a mixture of phospholipids such as TPF could be one of the requirements for effective anti-inflammatory activity (Jung et al. Citation2012).
Collagen content indicates the level of wound healing. Here, TPF treatment promoted pressure ulcer wound healing, increased collagen content, and facilitated normal tissue recovery after I/R injury. Kasuya (Kasuya et al. Citation2014) reported a marked decrease in blood vessels at the I/R injury site, and that I/R injury-induced ROS may damage these vessels. In our model, the number of perivascular cells in the I/R injury region was significantly higher in TPF-treated than that in control mice. Further studies are required to clarify TPF-mediated protection against I/R-injury-induced oxidative stress in vascular endothelial cells, mediated via hypoxia and ROS like H2O2 and NO2. Our experiments suggest that the protective effect of TPF on cutaneous I/R injury is associated not only with inflammation inhibition but also with regeneration of fibrous tissue and promotion of angiogenesis.
In summary, TPF accelerates the rate of pressure ulcer healing and contributes to tissue regeneration through inhibition of inflammation, increased collagen production, angiogenesis induction, and restoration of the skin barrier, thereby inhibiting cutaneous-I/R-injury-induced pressure ulcer formation. Continuous treatment with TPF demonstrated its efficacy with respect to skin wound healing – including pressure ulcers and other ischemic ulcers – confirming that TPF is industrially applicable.
Disclosure statement
No potential conflict of interest was reported by the author(s).
Data availability statement
Due to the nature of this research, participants of this study did not agree for their data to be shared publicly, so supporting data is not available.
Additional information
Funding
References
- Abate W, Alghaithy AA, Parton J, Jones KP, Jackson SK. 2010. Surfactant lipids regulate LPS-induced interleukin-8 production in A549 lung epithelial cells by inhibiting translocation of TLR4 into lipid raft domains. J Lipid Res. 51:334–344. doi: https://doi.org/10.1194/jlr.M000513
- Aleynik SI, Lieber CS. 2003. Polyenylphosphatidylcholine corrects the alcohol-induced hepatic oxidative stress by restoring s-adenosylmethionine. Alcohol Alcohol. 38:208–212. doi: https://doi.org/10.1093/alcalc/agg066
- Artuc M, Hermes B, Stckelings U, Grützkau A, Henz B. 1999. Mast cells and their mediators in cutaneous wound healing–active participants or innocent bystanders? Exp Dermatol. 8:1–16. doi: https://doi.org/10.1111/j.1600-0625.1999.tb00342.x
- Aziz M, Jacob A, Matsuda A, Wang P. 2011. Milk fat globule-EGF factor 8 expression, function and plausible signal transduction in resolving inflammation. Apoptosis. 16:1077. doi: https://doi.org/10.1007/s10495-011-0630-0
- Babu M, Rajaram R, Sai KP. 2010. Efficacy of frog skin lipids in wound healing. Lipids Health Dis. 9:74. doi: https://doi.org/10.1186/1476-511X-9-74
- Carroll WR, Esclamado RM. 2000. Ischemia/reperfusion injury in microvascular surgery. Head Neck. 22:700–713. doi: https://doi.org/10.1002/1097-0347(200010)22:7<700::AID-HED10>3.0.CO;2-H
- Chuangsuwanich A, Charnsanti O, Lohsiriwat V, Kangwanpoom C, Thong-In N. 2011. The efficacy of silver mesh dressing compared with silver sulfadiazine cream for the treatment of pressure ulcers. J Med Assoc Thail. 94:559.
- De Simone R, Ajmone-Cat MA, Nicolini A, Minghetti L. 2002. Expression of phosphatidylserine receptor and down-regulation of pro-inflammatory molecule production by its natural ligand in rat microglial cultures. J Neuropathol Exp Neurol. 61:237–244. doi: https://doi.org/10.1093/jnen/61.3.237
- Dowsett C. 2003. An overview of Acticoat dressing in wound management. Br J Nurs. 12:S44–S49. doi: https://doi.org/10.12968/bjon.2003.12.Sup4.12215
- Duan J, Karmazyn M. 1990. Protection of the reperfused ischemic isolated rat heart by phosphatidylcholine. J Cardiovasc Pharmacol. 15:163–171. doi: https://doi.org/10.1097/00005344-199001000-00026
- Eros G, Varga G, Varadi R, Czobel M, Kaszaki J, Ghyczy M, Boros M. 2009. Anti-inflammatory action of a phosphatidylcholine, phosphatidylethanolamine and N-acylphosphatidylethanolamine-enriched diet in carrageenan-induced pleurisy. Eur Surg Res. 42:40–48. doi: https://doi.org/10.1159/000167856
- Ferrante CJ, Leibovich SJ. 2012. Regulation of macrophage polarization and wound healing. Adv Wound Care. 1:10–16. doi: https://doi.org/10.1089/wound.2011.0307
- Harrow JJ, Mayrovitz HN. 2014. Subepidermal moisture surrounding pressure ulcers in persons with a spinal cord injury: a pilot study. J Spinal Cord Med. 37:719–728. doi: https://doi.org/10.1179/2045772313Y.0000000193
- Huang LS, Hung ND, Sok D-E, Kim MR. 2010. Lysophosphatidylcholine containing docosahexaenoic acid at the sn-1 position is anti-inflammatory. Lipids. 45:225–236. doi: https://doi.org/10.1007/s11745-010-3392-5
- Hung ND, Kim MR, Sok D-E. 2009. Anti-inflammatory action of arachidonoyl lysophosphatidylcholine or 15-hydroperoxy derivative in zymosan A-induced peritonitis. Prostagland Other Lipid Mediat. 90:105–111. doi: https://doi.org/10.1016/j.prostaglandins.2009.10.001
- Jones SA, Bowler PG, Walker M, Parsons D. 2004. Controlling wound bioburden with a novel silver-containing Hydrofiber® dressing. Wound Repair Regen. 12:288–294. doi: https://doi.org/10.1111/j.1067-1927.2004.012304.x
- Jung H-J, Moon J-S, Park AR, Choi H, Lee JE, Choi S-H, Lim C-J. 2012. Anti-inflammatory, antinociceptive and anti-angiogenic activities of a phospholipid mixture purified from porcine lung tissues. Immunopharmacol Immunotoxicol. 34:398–407. doi: https://doi.org/10.3109/08923973.2011.611137
- Kasuya A, Sakabe J-I, Tokura Y. 2014. Potential application of in vivo imaging of impaired lymphatic duct to evaluate the severity of pressure ulcer in mouse model. Sci Rep. 4:4173. doi: https://doi.org/10.1038/srep04173
- Kyrylkova K, Kyryachenko S, Leid M, Kioussi C. 2012. Detection of apoptosis by TUNEL assay. In: Kioussi C., editor. Odontogenesis. Berlin: Springer; p. 41–47.
- Lawrence T, Natoli G. 2011. Transcriptional regulation of macrophage polarization: enabling diversity with identity. Nat Rev Immunol. 11:750. doi: https://doi.org/10.1038/nri3088
- Livak KJ, Schmittgen TD. 2001. Analysis of relative gene expression data using real-time quantitative PCR and the 2−ΔΔCT method. Methods. 25:402–408. doi: https://doi.org/10.1006/meth.2001.1262
- Maklebust J. 1987. Pressure ulcers: etiology and prevention. Nurs Clin North Am. 22:359–377.
- Miksa M, Wu R, Dong W, Komura H, Amin D, Ji Y, Wang Z, Wang H, Ravikumar TS, Tracey KJ. 2009. Immature dendritic cell-derived exosomes rescue septic animals via milk fat globule epidermal growth factor VIII. J Immunol. 183:5983–5990. doi: https://doi.org/10.4049/jimmunol.0802994
- Mittal N, Sanyal SN. 2009. Exogenous surfactant suppresses inflammation in experimental endotoxin-induced lung injury. J Environ Pathol Toxicol Oncol. 28(4):341–349. doi: https://doi.org/10.1615/JEnvironPatholToxicolOncol.v28.i4.80
- Moon J-S, Jeon B-S, Yoon B-I, Choi S-H, Lim C-J. 2012. Topical application of a phospholipid mixture purified from pig lungs ameliorates 2, 4-dinitrofluorobenzene-induced allergic contact dermatitis in BALB/c mice. Mol Biol Rep. 39:4237–4247. doi: https://doi.org/10.1007/s11033-011-1210-7
- Morris R, Tonks AJ, Jones K, Ahluwalia M, Thomas A, Tonks A, Jackson SK. 2008. DPPC regulates COX-2 expression in monocytes via phosphorylation of CREB. Biochem Biophys Res Commun. 370:174–178. doi: https://doi.org/10.1016/j.bbrc.2008.03.052
- Mosser DM. 2003. The many faces of macrophage activation. J Leukocyte Biol. 73:209–212. doi: https://doi.org/10.1189/jlb.0602325
- Park CJ, Clark SG, Lichtensteiger CA, Jamison RD, Johnson AJW. 2009. Accelerated wound closure of pressure ulcers in aged mice by chitosan scaffolds with and without bFGF. Acta Biomater. 5:1926–1936. doi: https://doi.org/10.1016/j.actbio.2009.03.002
- Reddy M, Gill SS, Rochon PA. 2006. Preventing pressure ulcers: a systematic review. JAMA. 296:974–984. doi: https://doi.org/10.1001/jama.296.8.974
- Rogenski NMB, de Gouveia Santos VLC. 2005. Incidence of pressure ulcers at a university hospital. Rev Lat Am Enfermagem. 13:474–480. doi: https://doi.org/10.1590/S0104-11692005000400003
- Saito Y, Hasegawa M, Fujimoto M, Matsushita T, Horikawa M, Takenaka M, Ogawa F, Sugama J, Steeber DA, Sato S. 2008. The loss of MCP-1 attenuates cutaneous ischemia–reperfusion injury in a mouse model of pressure ulcer. J Invest Dermatol. 128:1838–1851. doi: https://doi.org/10.1038/sj.jid.5701258
- Shaheen MA, Mahmoud MA, Abdel Aziz MM, El Morsy HI, Abdel Khalik KA. 2009. Sputum dipalmitoylphosphatidylcholine level as a novel airway inflammatory marker in asthmatic children. Clin Respir J. 3:95–101. doi: https://doi.org/10.1111/j.1752-699X.2009.00127.x
- Stadler I, Zhang R-Y, Oskoui P, Whittaker MBS, Lanzafame RJ. 2004. Development of a simple, noninvasive, clinically relevant model of pressure ulcers in the mouse. J Invest Surg. 17:221–227. doi: https://doi.org/10.1080/08941930490472046
- Suman P, Ramachandran H, Sahakian S, Gill KZ, Horst BA, Modak SM, Hardy MA. 2012. The use of angiogenic-antimicrobial agents in experimental wounds in animals: problems and solutions. Ann N Y Acad Sci. 1270:28–36. doi: https://doi.org/10.1111/j.1749-6632.2012.06653.x
- Treede I, Braun A, Sparla R, Kühnel M, Giese T, Turner JR, Anes E, Kulaksiz H, Füllekrug J, Stremmel W. 2007. Anti-inflammatory effects of phosphatidylcholine. J Biol Chem. 282:27155–27164. doi: https://doi.org/10.1074/jbc.M704408200
- Uchiyama A, Yamada K, Perera B, Ogino S, Yokoyama Y, Takeuchi Y, Ishikawa O, Motegi S-I. 2015. Protective effect of MFG-E8 after cutaneous ischemia–reperfusion injury. J Invest Dermatol. 135:1157–1165. doi: https://doi.org/10.1038/jid.2014.515