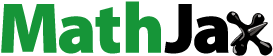
ABSTRACT
Several plant phytochemicals have been reported to possess therapeutic properties. However, most of them are capable of causing oxidation of the human haemoglobin into the ferric state making them hazardous to cells and tissues. This study was aimed at investigating the non-oxidative effect of the flavonoid-rich fraction of Lasianthera africana leaves (LAFRF) on human haemoglobin. Isolation and purification of LAFRF was carried out by solvent extraction, partitioning and ion-exchange chromatography. Six test groups were setup as follows: A reaction tube containing 800 µl of oxyhaemoglobin (OxyHb) served as the normal control, while the test groups were made up of different concentrations of LAFRF (5, 10, 20, 40 and 80 mg/ml) incubated with 100 µl of OxyHb. The non-oxidative effects of the LAFRF on the human haemoglobin was assessed by UV-visible spectroscopy. The results indicated that LAFRF possess non-oxidative effects on human haemoglobin, as it did not cause any disappearance of the absorbance maxima at 576 nm and 541 nm at all concentrations investigated. Furthermore, a concentration-dependent increase in the absorbance of the treated OxyHb was recorded when compared to the untreated OxyHb, thus signifying its potential as a safe pharmacological agent for in vivo investigations.
Introduction
Lasianthera africana P. Beauv. belongs to the plant family, icacinaceae, and is a fibrous woody plant, smaller than a tree with several stems arising at or close to the ground. It grows up to 4 meters high with terete branchlets and white flowers in umbellate head-like clusters, found as under storey in secondary jungle and thickets in the rain forest of Southern Nigeria and Western Cameroon extending to Zaïre in Congo (Burkill Citation1994; Neuwinger Citation2000). The plant is locally known as editan in Ibibio and Anang tribes of Southern Nigeria, kpurugiza in Igbo tribe of Eastern Nigeria, belele and itebebele by the Kpe and Muni tribes of West Cameroon respectively. Some pharmacological benefits of the plant include; possession of analgesic, antipyretic, antimalarial and anti-ulcerogenic properties (Okokon et al. Citation2009), antimicrobial (Andy et al. Citation2008; Ebana et al. Citation2016), antidiabetic (Inyang et al. Citation2015; Ekanem et al. Citation2016), anti-inflammatory (Nwidu and Teme Citation2018), antioxidant (Atiko et al. Citation2016), and hepatoprotective (Nwidu and Teme Citation2018), cardioprotective as well as renoprotective effects (Ekpo et al. Citation2020).
Phytochemical analysis of L. africana has shown the leaves of the plant to be rich in flavonoids among other phytochemical constituents (Ebana et al. Citation2016). A recent study by (Ekpo et al. Citation2020), identified the presence of flavonoids such as rutin, quercetin, isorhamnetin and luteolin in the flavonoid enriched fraction of the plant leaves, as well as other non-phenolics such as soyasaponin III ion, soyasapogenol-B-3-O-β-glucuronate, and oleanolate-3-O-β-D-glucoside. Flavonoids are a class of low molecular weight phenolic compounds, widely distributed in plants. They possess variable phenolic structures, and are widely distributed in fruits, vegetables, grains, bark, roots, stems, flowers, tea and wine (Panche et al. Citation2016). Chemically, flavonoids are based upon a 15-carbon skeleton consisting of two benzene rings (A and B) linked by a heterocyclic pyrane ring (C). They are divided into various classes which differ in their level of oxidation and pattern of substitution of the C ring, while the individual compounds within a class differ in the pattern of substitution of the A and B rings (Middleton Citation1998). These classes include; flavones (e.g. apigenin and luteolin), flavonols (e.g. quercetin, kaempferol, myricetin, and rutin), flavanones (e.g. flavanone, hesperetin, and naringenin), flavanonol (e.g. taxifolin), isoflavones (e.g. genistein and daidzein), and flavan-3-ols (e.g. catechin and epicatechin) amongst others (Kumar and Pandey Citation2013). Flavonoids occur as aglycones, glycosides, and methylated derivatives. The basic flavonoid structure is aglycone, a six-member ring condensed with the benzene ring which is either a α-pyrone (flavonols and flavanones) or its dihydroderivative (flavonols and flavanones) (Kumar and Pandey Citation2013).
One of the unique features of flavonoids which makes them pharmacologically active is their antioxidative property (Kumar and Pandey Citation2013). Unlike other phytochemicals which are shown to undergo oxidation with the human haemoglobin when ingested either as food or drug, flavonoids have been reported to be safe for human consumption without undergoing any oxidative reactions with the human haemoglobin (Takahashi and Ohnishi Citation2004). However, this study was designed to provide scientific credence to this claim. Hence, the aim of this research was to investigate the non-oxidative effect of the flavonoid-rich fraction of L. africana leaves (LAFRF) on human haemoglobin.
Materials and methods
Collection, identification and authentication of plant material
Fresh leaves of L. africana P. Beauv. (Figure ) were collected from a local vegetable farm in Ukana Akpautong, Ikot Akpantia village in Essien Udim Local Government Area of Akwa Ibom State, Nigeria, in October, 2018. The leaf sample was identified and authenticated by Mr. Felix Nwafor, a Plant Taxonomy and Biosystematics’ specialist of the Herbarium of the Department of Pharmacognosy and Environmental Medicine, University of Nigeria, Nsukka, Nigeria, with a voucher specimen (PCG/UNN/0315) deposited. The identity and authenticity of the plant was also confirmed with the one deposited at the following linked databases: http://www.theplantlist.org/ and http://www.ipni.org/.
Chemicals and reagents
All chemicals and reagents used for the conduct of this study were of analytical grade, and products of British Drug House (BDH), England (Ethanol, Methanol, and concentrated hydrochloric acid); Qualikems, India (Sodium chloride, Sodium trioxocarbonate (IV), Sodium hydroxide, Aluminum chloride and Potassium acetate); Sigma Aldrich, St. Louis, MO (Quercetin, Gallic acid, Ethyl acetate, Dowex® ion-exchange resin, 50WX8, hydrogen form, mesh size 100-200); Panhong Chemical Company, China (Potassium cyanide, % purity ≤ 96%; Potassium phosphate salts, % purity ≤ 97%); Thomas Scientific, New Jersey, USA (Sephadex G-150); Coral Health Care, Hyderbad, India (95% methylated spirit). Distilled water, deionized water, and potassium phosphate buffer of pH 7.6 were procured from the National Centre for Energy Research and Development (NCERD), University of Nigeria, Nsukka.
Instrument and equipment
Legend X1R centrifuge (LABSCO, Germany), adjustable micropipette (Perfect, USA), analytical chemical balance (AR3130, Ohaus Corp., China), lyophilizer (JLab45885, Ambala, India), refrigerator (Thermocool, England), stop watch (Traceable® Dublin, Ohio, USA), test tubes, beakers and ion exchange column (Pyrex, England), UV-vis Spectrophotometer and glass cuvette (Jenway 6305, China), water bath (Gellenkamp, England), analytical chemical balance (AR3130, Ohaus Corp., China).
Preparation and processing of plant material
The fresh leaves of L. africana P. Beauv. were carefully destalked, washed with potable water to remove sand and debris, after which they were spread under shade to drain completely (Figure ). The drained leaves were air-dried for a period of three weeks until a constant weight was achieved. The dried plant leaves were then pulverized into powdered form and stored in air-tight containers for extraction of bioactive components and subsequent isolation and purification of the flavonoid-enriched fraction.
Extraction of crude plant material
The crude sample preparation in this study has been previously reported (Ekpo et al. Citation2020). Briefly, 3200 g of the dried and powdered plant material was extracted with an appropriate volume of 80% methanol in double distilled water using cold maceration method for 72 h. The crude extract obtained was filtered using a muslin cloth, and the filtrate collected was centrifuged at 6000 rpm for 15 min to remove the fine suspended particles. The remaining solvent was removed at reduced pressure to obtain the methanol extract of L. africana (MELA).
Isolation and purification of L. africana flavonoid-enriched fraction
The isolation and purification of LAFRF was carried out by the method of Omotuyi et al. (Citation2018), and is previously reported (Ekpo et al. Citation2020). Briefly, 100 g of the cationic exchanger, Dowex® 50WX8 hydrogen form (100–200 mesh size), was activated by overnight treatment with 2 bed volumes (BV) of distilled ethanol followed by rinsing with 5 BV of distilled water at room temperature (25 ± 1°C). The activated resin was loaded on a glass column. In every cycle, 200 ml of the extract was loaded on a 50 ml resin volume and allowed to settle for 1 h. The column was washed thoroughly with distilled and deionized water to elute sugars and unbound impurities. Bound flavonoids were eluted using a solvent mixture of 95% ethanol and 5% HCl (1% v/v) until the absorbance of the eluted golden-yellowish fraction at 260 nm is close to 0.00. Eluted samples were pooled, freeze-dried and referred to as the flavonoid-rich fraction of L. africana (LAFRF).
Determination of total flavonoid content of LAFRF
The determination of the total flavonoid content of the LAFRF have been previously reported (Ekpo et al. Citation2020).
Dose range
To study the oxidative effects of LAFRF on human haemoglobin, LAFRF at concentrations of 5 mg/ml, 10 mg/ml, 20 mg/ml, 40 mg/ ml and 80 mg/ml were chosen based on previous studies conducted using LAFRF, and also because these range of concentrations correspond to the circulating amount of LAFRF found after oral administration in animal models used for the previous study (Ekpo et al. Citation2020). However, these range of doses are not consistent with any prior or current clinical use as this is the first in vitro trial conducted using the range of doses.
Ethical approval
The study was conducted in accordance with the regulations and ethical approval of the Ethics and Biosafety Committee of the Faculty of Biological Sciences, University of Nigeria, Nsukka, with Approval No. UNN/FBS/EC/2019/1007.
Collection of blood sample
Whole blood sample was collected from a 27 years old healthy human volunteer (a non-smoking male postgraduate student of the University of Nigeria, Nsukka), who was confirmed to be of genotype AA, and blood group O+ with sufficient Hb level at Renanscent Hospital, Nsukka, Nigeria. Whole blood volume of 8 mL was collected from the volunteer by venepuncture and emptied into a Venoject tube containing ethylenediamine tetra-acetic acid (EDTA) as an anticoagulant and kept at 4°C until use for a maximum duration of 24 h.
Preparation of haemoglobin
Haemoglobin purification was carried out according to the method previously described by Meng and Alayash (Citation2017). An aliquot of 5 ml of whole blood sample was twice washed with normal saline and centrifuged at 2500 rpm for 45 min using a Legend X1R centrifuge (LABSCO, Germany). The supernatant was carefully decanted, followed by addition of 5 ml of distilled water to the pellets. The mixture was rocked gently at room temperature for 5 min after which it was lysed in an ice bath for 3 h. The lysate was then centrifuged at 5,000 rpm for 45 min. The supernatant was carefully separated and loaded onto a well packed Sephadex G-150 fast-flow column (bed dimension 4 × 40 cm, 400 ml), which was equilibrated with 4 column volume of 10 mM potassium phosphate buffer of pH 7.6. Haemoglobin AA was eluted at 4°C with a linear gradient of 25–100% of the buffer in 2 column volumes. The column was eluted at a flow rate of 2.5 ml/min and the effluent was monitored at 541 nm, and 576 nm. Hb AA was collected and stored at −4°C for future use. For spectrophotometry, stock solution of the haemoglobin and phosphate buffer were mixed in the ratio 5:1 with different pipettes for the sample and reference.
Measurement of haemoglobin concentration
The Beer–Lambert law (Robert et al. Citation1994) was used for the determination of the oxyhaemoglobin (OxyHb) concentration. The absorption spectrum was scanned between the visible range (400–700 nm). The extinction coefficients derived by Meng and Alayash (Citation2017) was used for the determination of the oxyhaemoglobin concentration. The equation given below was used to calculate the concentration of oxyhaemoglobin (oxyHb) in the sample, and the results were expressed as mM.
Where C is the oxyHb concentration, A is the absorbance at 541 nm and 576 nm, e is the molar extinction coefficient at 541 nm and 576 nm, and l is the path length of the cuvette.
Study design
Six research groups were setup to monitor the non-oxidative effect of LAFRF on human red cell haemoglobin. Oxyhaemoglobin served as the normal control, while the test groups were made of different concentrations of LAFRF incubated with 800 µl of oxyHb for 3 h as indicated below:
Group 1: 800 µl of oxyHb (control)
Group 2: 100 µl of 5 mg/ml LAFRF + 800 µl OxyHb
Group 3: 100 µl of 10 mg/ml LAFRF + 800 µl OxyHb
Group 4: 100 µl of 20 mg/ml LAFRF + 800 µl OxyHb
Group 5: 100 µl of 40 mg/ml LAFRF + 800 µl OxyHb
Group 6: 100 µl of 80 mg/ml LAFRF + 800 µl OxyHb
UV-visible spectral analysis
The mixtures in each test tube representing the different test groups presented above were subjected to UV-visible spectral analysis. The UV spectrophotometer was calibrated to zero with the blank, and the wavelength scan range was set at 400–700 nm. The sample in the cuvette was introduced into the spectrophotometer and scanned at the set range of wavelength until completion. A desktop computer connected to the UV spectrophotometer displayed the UV scan spectrum of each of the test sample.
Results
Percentage yield of MELA and LAFRF
The extraction of 3200 g of the powdered L. africana leaves using methanol, and subsequent solvent partitioning in ethyl acetate and purification of 171.20 g (5.36%) of the MELA by ion-exchange chromatography yielded 2.853 g of the LAFRF (11.41%) as previously reported (Ekpo et al. Citation2020).
Total flavonoid concentration of LAFRF
The total flavonoid concentration of the LAFRF was reported to be 281.05 ± 7.44 mg QE/g of freeze-dried LAFRF, while that of the methanol extract (MELA) was 13.95 ± 0.65 mg QE/g of dry plant extract (Ekpo et al. Citation2020).
Non-oxidative effects of LAFRF
Characterization of the absorption spectrum of unreacted oxyhaemoglobin revealed two distinct and prominent peaks (namely β and α band) at 541 nm and 576 nm respectively (Figure ). With this as a baseline, the effect of different concentrations of LAFRF on normal human oxyhaemoglobin was determined.
Figure 2. Absorption spectrum of 800 µl of OxyHb (16 mM) in 10 Mm phosphate buffer at pH 7.6 after 3 h incubation at 25°C.
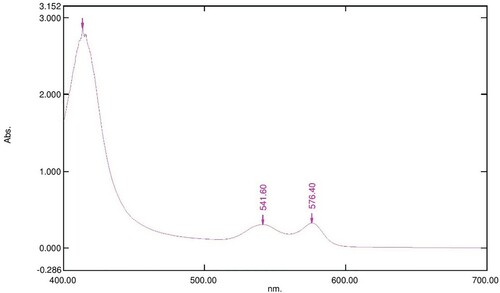
The UV-visible spectra of Figures – reveal that the absorption spectrum of the different concentrations of LAFRF (5, 10, 20, 40, and 80 mg/ml) did not cause any oxidative changes in the characteristic peaks of normal OxyHb; rather there was an increase in the absorbance values of the different concentrations of LAFRF tested when compared to that of the normal OxyHb (Table ).
Figure 3. Absorption spectrum of 800 µl of 16 mM OxyHb in 10 Mm phosphate buffer at pH 7.6 with 100 µl of 5 mg/ml LAFRF for 3 h at 25°C.
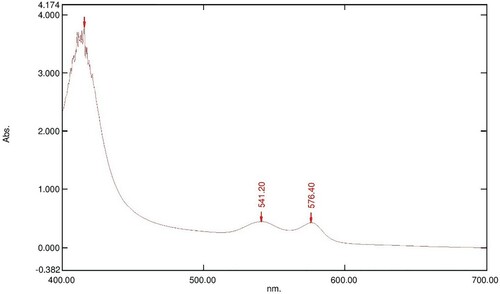
Figure 4. Absorption spectrum of 800 µl of 16 mM OxyHb in 10 Mm phosphate buffer at pH 7.6 with 100 µl of 10 mg/ml LAFRF for 3 h at 25°C.
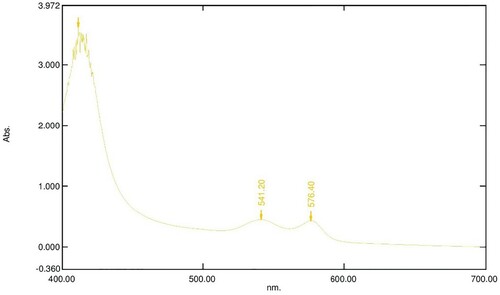
Figure 5. Absorption spectrum of 800 µl of 16 mM OxyHb in 10 Mm phosphate buffer at pH 7.6 with 100 µl of 20 mg/ml LAFRF for 3 h at 25°C.
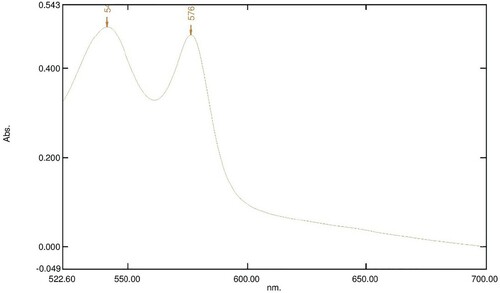
Figure 6. Absorption spectrum of 800 µl of 16 mM OxyHb in 10 Mm phosphate buffer at pH 7.6 with 100 µl of 40 mg/ml LAFRF for 3 h at 25°C.
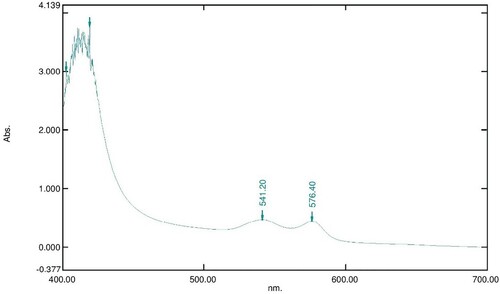
Figure 7. Absorption spectrum of 800 µl of 16 mM OxyHb in 10 Mm phosphate buffer at pH 7.6 with 100 µl of 80 mg/ml LAFRF for 3 h at 25°C.
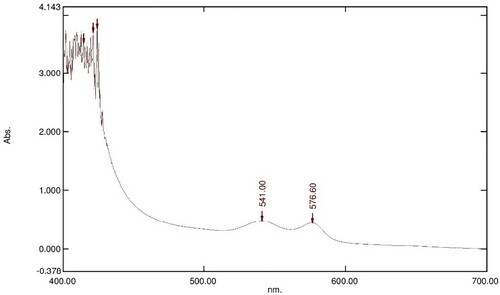
Table 1. Absorbance at 541 and 576 nm and OxyHb concentration.
Discussion
Certain plants used in herbal and traditional medicine are capable of causing oxidation of the human haemoglobin. Studies have shown that the disappearance of the absorbance maxima at 576 nm and 541 nm is indicative of oxidation of OxyHb (Meng and Alayash Citation2017). Interestingly, the absorption spectrum of different concentrations of LAFRF on OxyHb starting from a concentration of 5 mg/ml to a concentration of 80 mg/ml did not show disappearance of the absorbance maxima at 576 nm and 541 nm. This implies that OxyHb was not oxidized when treated with different concentrations of LAFRF. Also, when the absorption spectrum of different concentrations of LAFRF were compared to that of the normal OxyHb, we observed a concentration-dependent increase in the absorbance maxima of the treated OxyHb compared to the untreated OxyHb, with the highest increase seen at 20 mg/ml of LAFRF. From Beer-Lambert law, it has been shown that absorbance is directly proportional to concentration; therefore, increase in absorption maxima of the treated OxyHb absorption spectra is indicative of increase in OxyHb concentration (Robert et al. Citation1994). Thus, instead of the different concentration of LAFRF oxidizing OxyHb, they caused an increase in OxyHb concentration. The non-oxidation of OxyHb by LAFRF could be acrued to its anti-oxidative effects. Several works have reported the anti-oxidative effects of flavonoid rich fraction of different plants on experimental models (Aviram Citation2004; Sudheesh and Vijayalakshmi Citation2005; Zhang et al. Citation2018; Zakaria et al. Citation2019; Zakaria et al. Citation2020).
Although to the best of our knowledge, no work on spectral analysis of the effect of flavonoid-rich fraction of any plant on OxyHb has been done, our findings corroborate the report of other studies on the anti-oxidative effects and effect of flavonoid-rich fraction of some plants on human haemoglobin concentration. For instance, Nabavi et al. (Citation2012) reported that flavonoid-rich fraction of Eryngium caucasicum exhibited very good anti-oxidative activity against haemoglobin-induced linoleic acid peroxidation, and increase in oxygen carrying ability of the haemoglobin. Ekeanyanwu and Njoku (Citation2014) reported that after 28 days of oral administration, the flavonoid-rich fraction of Monodora tenuifolia caused a significant increase (p < 0.05) in haemoglobin concentration of albino rats. Ankita et al. (Citation2015) reported that flavonoid-rich fraction of Punica granatum exhibited improvement in antioxidant enzymatic activity of diabetic rats and improved the antioxidant parameters of diabetic rats. Furthermore, Ankita et al. (Citation2015) reported that flavonoid-rich fraction of Punica granatum caused improvement in glycosyalated haemoglobin of diabetic rats hence preventing the development of diabetic nephropathy. Kaviarasan et al. (Citation2008) reported that flavonoid rich fraction of Spermacoce hispida possesses strong antioxidant properties evidenced by a significant increase in the levels of enzymic and non-enzymic antioxidants. From the study of Ezeonu et al. (Citation2017) it was also found that flavonoid-rich fraction of methanol extract of Jatropha tanjorensis leaves contains anti-oxidants which scavenged the CCl4-induced free radical species. Studies by Vuppalapati et al. (Citation2016) showed that the flavonoid-rich fraction of Acanthophora specifera also caused an overall improvement in haemoglobin level and possesses anti-oxidative properties.
The non-oxidative effects of LAFRF on OxyHb and the increased absorbance maxima of the absorption spectrum for the treated OxyHb test groups has many biological significances. Firstly, it shows that LAFRF could stimulate the overproduction of haemotopoitic regulatory elements such as thrombopoietin, colony-stimulating factors and erythropoietin by the stroma cells and macrophages in the bone marrow, thus leading to production and increase in haemoglobin concentration (Ekeanyanwu and Njoku Citation2014). Also, it shows that LAFRF could induce the production of free radical-scavenging enzymes such as catalase, superoxide dismutase and glutathione peroxidase, and/or increase the level of non-enzymatic antioxidants such as glutathione, vitamin C and vitamin E that may help stabilise the spontaneous oxidation of OxyHb to methaemoglobin (Kaviarasan et al. Citation2008; Ezeonu et al. Citation2017). Furthermore, it shows that LAFRF may not possesscompounds that are electron donors; it has been shown that reaction of OxyHb with electron donors could lead to oxidation of Fe2+ to F3+ of the heme group of the haemoglobin (Faivre et al. Citation1998). Therefore, the non-oxidation of the treated OxyHb might be attributed to absence of electron donors in LAFRF (Faivre et al. Citation1998). More so, it is indicative that LAFRF might have a weak Fe2+ chelating ability preventing the chelation of Fe2+ of the OxyHb with harmful or deleterious compounds that may oxidize the human haemoglobin (Nabavi et al. Citation2012). Pharmacologically, the increase in the concentration of OxyHb by LAFRF make it a suitable and potent pharmacological agent which could be administered for the treatment of some physiological conditions where there is a drop in haemoglobin levels such as in cases of menstruation, pregnancy, anaemia, hyperglycaemia and hyperlipidemia, and could also be used as a hematinic in stress-induced depletion of blood (Ezeonu et al. Citation2017).
Furthermore, the non-oxidation of OxyHb by LAFRF suggests its potential usefulness as a pharmacological agent in treatment of hypoxia and haemolytic anaemia. It has been reported that Fe2+ have higher affinity for oxygen than F3+ at 576 and 541 nm within the visible range of UV-visible spectrophotometry, and that the increase in absorbance maxima of OxyHb is directly proportional to increase in oxygen affinity (Ezeawgula and Onwubiko Citation2016). Since LAFRF did not oxidize Fe2+ of the OxyHb to F3+, but rather caused an increase in its oxygen affinity as indicated by the increase in the absorbance maxima, it suggests that LAFRF could be useful in hypoxic and anaemic conditions where there is a decrease in oxygen carrying ability of haemoglobin (Ezeawgula and Onwubiko Citation2016). It has also been shown that oxidation of oxyhaemoglobin to methaemoglobin could be caused by xenobiotic-induced oxidative stress. Since, LAFRF did not oxidize OxyHb to methaemoglobin but rather caused an overall improvement in OxyHb concentration, this suggests that LAFRF could be a useful pharmacological agent in pathophysiological conditions triggered by oxidative stress (Faivre et al. Citation1998).
Conclusion
The present study investigated the anti-oxidative property of LAFRF on human oxyhaemoglobin. From the results obtained, we found that at increasing concentrations of the LAFRF ranging from 5 mg/ml to 80 mg/ml, no oxidation of the human haemoglobin occurred. However, LAFRF caused an increase in the in absorbance maxima and corresponding increase in the concentration of OxyHb of the treated groups, thus signifying its potential as a suitable pharmacological agent for the amelioration of oxidative stress in cells, improvement of haemoglobin concentration in haemolytic anaemia, and increase in oxygen binding affinity for the red cell haemoglobin in hypoxic conditions.
Supplemental Material
Download MS Word (589.3 KB)Acknowledgements
The authors are thankful to Dr. Anthony Afomata (PhD) of the National Centre for Energy Research and Development Commission (NCERD), Energy Commission of Nigeria, University of Nigeria, Nsukka, for conducting of the spectral analysis. Author E.C. Anorue: Conceptualized and designed the study; Contributed reagents, materials and analysis tools; Performed the experiments; Analysed and interpreted the data; Wrote the paper. Author D.E. Ekpo: Conceptualized and designed the study; Contributed reagents, materials and analysis tools; Performed the experiments; Analysed and interpreted the data; Supervised the work; Wrote the paper.
Disclosure statement
No potential conflict of interest was reported by the author(s).
Data availability statement
Due to the nature of this research, participants of this study did not agree for their data to be shared publicly. So supporting data is not available due to ethical concerns.
References
- Andy IE, Eja ME, Mboro CI. 2008. An evaluation of the antimicrobial potency of Lasianthera africana (Beauv) and Heinsia crinata (G. Taylor) on Escherichia coli, Salmonella typhi Staphylococcus aureus and Candida albicans. Malays J Microbiol. 4(1):25–29.
- Ankita P, Deepti B, Nilam M. 2015. Flavonoid rich fraction of Punica granatum improves early diabetic nephropathy by ameliorating proteinuria and disturbed glucose homeostasis in experimental animals. Pharm Biol. 53(1):61–71.
- Atiko R, Onocha PA, Oyedemi SO. 2016. Phytochemical analysis and antioxidant properties of Lasianthera africana leaves, stems and roots extracts. Res J Chem Sci. 6(9):19–26.
- Aviram M. 2004. Flavonoids-rich nutrients with potent anti-oxidant activity prevent atherosclerosis development: the licorice example. Int Congr Ser. 1262:320–327.
- Burkill HM. 1994. The useful plants of West Tropical Africa. 2nd ed. Vol. 2, Families E–I. Kew: Royal Botanic Gardens, Richmond, United Kingdom; p. 636.
- Ebana RUB, Asamudo NU, Etok CA, Ede UO, Onyebuis CS. 2016. Phytochemical screening, nutrient analysis and antimicrobial activity of the leaves of Lasianthera africana and Dennettia tripetala on clinical isolates. J Appl Biotechnol Bioeng. 8(4):1–9.
- Ekanem NG, Mbagwu HOC, Harry GI. 2016. Phytochemical screening and hypoglycaemic activity of Lasianthera africana Beauv. (Aquifoliales: Stemonuraceae) leaf extract on diabetic rats. Braz J Biol Sci. 3(6):293–298.
- Ekeanyanwu RC, Njoku OU. 2014. Acute and subacute oral toxicity study on the flavonoid rich fraction of Monodora tenuifolia seed in albino rats. Asian Pac J Trop Biomed. 4(3):194–202.
- Ekpo DE, Joshua PE, Ogidigo JO, Nwodo OFC. 2020. High Resolution UPLC-PDA- QTOF-ESI-MS/MS analysis of the flavonoid-rich fraction of Lasianthera africana leaves, and in vivo evaluation of its renal and cardiac function effects. Helyion. 6(6):e04154.
- Ezeawgula BA, Onwubiko HA. 2016. Haemoglobin oxidation: a possible mechanism of crude oil toxicity in fish. Int J Biosci. 9(1):25–33.
- Ezeonu D, Anosike CA, Njoku OU. 2017. Hepatoprotective and antioxidant effects of the flavonoid rich fraction of methanol extract of Jatropha tanjorensis leaves in CCl4- induced liver injury in rats. IOSR J Pharm Biol Sci. 12(1):54–61.
- Faivre B, Menu P, Labrude P, Vigneron C. 1998. Haemoglobin autooxidation/oxidation mechanisms and methaemoglobin prevention or reduction processes in the bloodstream. Artif Cells Blood Substit Biotechnol. 26(1):17–26.
- Inyang UE, Nwafor PA, Asamudo UN. 2015. Assessment of acute toxicity profile of Lasianthera africana leaf extract in normal rats and its ameliorative effect in alloxan-induced diabetic rats. Afr J Biotechnol. 14(15):1318–1324.
- Kaviarasan K, Kalaiarasi P, Pugalendi V. 2008. Antioxidant efficacy of flavonoid rich fraction from Spermacoce hispida in hyperlipidemic rats. J Appl Biomed. 6:165–176.
- Kumar S, Pandey AK. 2013. Chemistry and biological activities of flavonoids: an overview. Sci World J. 13:1–16.
- Meng F, Alayash AI. 2017. Determination of extinction coefficients of human haemoglobin in various redox states. Anal Biochem. 521:11–19.
- Middleton EJ. 1998. Effect of plant flavonoids on immune and inflammatory cell function. Adv Exp Med Biol. 439:175–182.
- Nabavi SM, Nabavi SF, Alinezhad H, Zare M, Azimi R. 2012. Biological activities of flavonoid-rich fraction of Eryngium Caucasicum Trautv. Eur Rev Med Pharmacol Sci. 3:81–87.
- Neuwinger HD. 2000. African traditional medicine: a dictionary of plant use and applications. Stuttgart, Germany: Medpharm Scientific; p. 589.
- Nwidu LL, Teme RE. 2018. Hot aqueous leaf extract of Lasianthera africana (Icacinaceae) attenuates rifampicin-isoniazid-induced hepatotoxicity. J Integr Med. 16(4):263–272.
- Okokon JE, Antia BS, Umoh EE. 2009. Antiulcerogenic activity of ethanolic leaf extract of Lasianthera africana. Afr J Tradit Complement Altern Med. 6(2):150–154.
- Omotuyi OI, Nash O, Inyang OK, Ogidigo J, Enejoh O, Okpalefe O, Hamada T. 2018. Flavonoid-rich extract of Chromolaena odorata modulate circulating GLP-1 in Wistar rats: Computational evaluation of TGR5 involvement. Biotech. 8(2):124–132.
- Panche AN, Diwan AD, Chandra SR. 2016. Flavonoids: an overview. J Nutr Sci. 47(5):1–15.
- Robert WR, Mauri D, Lisa PN. 1994. Discovering the Beer-Lambert Law. J Chem Educ. 71(11):983.
- Sudheesh S, Vijayalakshmi NR. 2005. Flavonoids from Punica granatum-potential antiperoxidative agents. Fitoterapia. 76:181–186.
- Takahashi A, Ohnishi T. 2004. The significance of the study about the biological effects of solar ultraviolet radiation using the exposed facility on the international space station. Biol Sci. 18:255–260.
- Vuppalapati L, Velayudam KFH, Ahamed N, Cherukuri S, Kesavan BR. 2016. The protective effects of dietary flavonoid fraction from Acanthophora specifera on induced oxidative stress in diabetic rats. Food Sci Human Wellness. 5(2):57–64.
- Zakaria ZA, Kamisan FH, Kek TL, Salleh MZ. 2020. Hepatoprotective and antioxidant activities of Dicranopteris linearis leaf extract against paracetamol-induced liver intoxication in rats. Pharm Biol. 58(1):478–489.
- Zakaria ZA, Mahmood ND, Omard MH, Tahere M, Basirf R. 2019. Methanol extract of Muntingia calabura leaves attenuates CCl4-induced liver injury: Possible synergistic action of flavonoids and volatile bioactive compounds on endogenous defense system. Pharm Biol. 57(1):335–344.
- Zhang W, Dong Z, Chang X, Zhang C, Rong G, Gao X, Zeng Z, Wang C, Chen Y, Rong Y, et al. 2018. Protective effect of the total flavonoids from Apocynum venetum L. on carbon tetrachloride-induced hepatotoxicity in vitro and in vivo. J Physiol Biochem. 74:301–312.