Abstract
The biocontrol potential of antagonistic bacteria and yeasts isolated from tomato phyllosphere and fructoplane was evaluated against fruit rot of tomato caused by Alternaria alternata. A total of 48 bacterial and 24 yeast isolates were obtained from field-grown tomato leaves/fruits collected from Barka, Oman. The antagonistic activities of these isolates were evaluated against A. alternata using in vitro dual culture assay. Among the 72 bacterial and yeast isolates tested, one yeast (Meyerozyma guilliermondii) and two bacteria (Enterobacter roggenkampii and Pseudomonas aeruginosa) showed inhibitory activity against A. alternata. Examination of hyphal morphology of A. alternata at the edge of inhibition zone using scanning electron microscopy revealed abnormalities such as twisting, shriveling, distortion and collapsing of hyphae. Postharvest treatment of tomato fruits with E. roggenkampii, P. aeruginosa and M. guilliermondii reduced the fruit rot lesion size by 60%, 57% and 50%, respectively, compared to untreated control. The antifungal metabolites produced by the antagonistic yeast M. guilliermondii were fractionated using thin-layer chromatography. Characterization of the bioactive fractions using gas chromatography-mass spectrometry revealed the presence of different types of alkanes, predominantly tricosane and pentacosane, suggesting that volatile compounds, especially alkanes, produced by M. guilliermondii might also be involved in the suppression of A. alternata.
Introduction
Tomato (Solanum lycopersicum L.) is the world’s second most important vegetable crop, with a total production of 182 million tonnes in 2018 (FAO Citation2020). The productivity of tomato is affected by multiple biotic stresses worldwide. More than 200 diseases cause damage to tomato (Singh et al. Citation2017). Alternaria alternata (Fr.) Keissler (= A. tenuis Auct.) is a common pathogen of tomato causing fruit rot disease. This fungus invades the tomato fruits through injured or weakened tissues (Pearson and Hall Citation1975). The infection of fruits by A. alternata occurs in the field or during harvesting, post-harvest handling and storage (Barth et al. Citation2009). Presence of copious nutrients, high moisture content and thin exocarp make tomato fruits vulnerable to infection and colonization by A. alternata (da Cruz Cabral et al. Citation2016). Ripe fruits are highly susceptible to infection by A. alternata compared to tomatoes at early developmental stages (Alkan and Fortes Citation2015). Furthermore, several species of Alternaria produce a vast range of mycotoxins including tentoxin (TEN), alternariol (AOH), altertoxin I (ALTX-I) and tenuazonic acid (TeA) (Escriva et al. Citation2017), which may contaminate tomato fruits. Exposure to these foodborne mycotoxins results in carcinogenic, mutagenic, genotoxic and cytotoxic effects on humans and animals (Escriva et al. Citation2017; Meena et al. Citation2017). Alternaria fruit rot of tomato can be controlled by dipping tomato fruits in Imazalil solution (0.01–0.1% a.i) for 2 min (Spalding Citation1980). As a result of increasing public concern about the detrimental effects of synthetic chemical pesticides, the use of antagonistic microorganisms specifically bacteria and yeasts that are naturally present on the surfaces of vegetables and fruits has been preferred for the management of postharvest diseases (Nunes Citation2012). Several commercial products based on microbial antagonists such as Shemer (Metschnikowia fructicola), BOTRY-Zen (Ulocladium oudemansii), PomaVita (Pantoea agglomerans), BlossomBless (Pantoea agglomerans), Biosave 100/110 (Pseudomonas syringae), Yieldplus (Cryptococcus albidus), Nexy (Cryptococcus oleophila), BoniProtect (Aureobasidium pullulans) and Candifruit (Candida sake) are available in other countries to manage postharvest diseases (Shi and Sun Citation2017; Zhang et al. Citation2018; Freimoser et al. Citation2019). Many reports indicate the superiority of pre-harvest application of biocontrol agents in protecting fruits and vegetables against postharvest diseases (Ippolito and Nigro Citation2000; Karabulut et al. Citation2004; Larena et al. Citation2005). Under the hot and dry conditions in Oman, native and habitat-specific antagonistic microorganisms, which may show better adaptability to the prevailing local climatic conditions, may be suitable for use as biocontrol agents for the management of pre- and post-harvest diseases of fruits and vegetables. The study is aimed to: (i) isolate and identify native antagonistic yeasts and bacteria from tomato phyllosphere and fructoplane; (ii) evaluate their antagonistic activity against Alternaria fruit rot of tomato; and (iii) examine the antifungal activity of volatile compounds produced by selected antagonist(s).
Materials and methods
A. alternata from tomato fruits
Infected tomato fruits were collected from field-grown plants from an agricultural farm in Barka, Oman. Isolation of fungus from the infected samples was on potato dextrose agar (PDA; Oxoid Ltd., Basingstoke, UK), according to Meena et al. (Citation2017). The identification of the fungus was based on ribosomal DNA (rDNA) internal transcribed spacer (ITS) sequences (Al-Sadi et al. Citation2012). The nucleotide sequence of the isolate SQUCC-S4 was deposited in GenBank (accession number MK583589). Pathogenicity of the fungal isolate was established by artificially inoculating tomato (cv. Pomodoro) fruits according to da Cruz Cabral et al. (Citation2016).
Isolation of yeasts and bacteria from tomato phyllosphere and fructoplane
Healthy tomato leaf or fruit samples were placed in 100 ml of sterile distilled water (SDW) and shaken gently for 3 h on a rocking platform. The leaf and fruit-wash suspensions (100 µl) were plated on Sabouraud dextrose agar medium and nutrient agar medium for isolation of yeasts and bacteria, respectively. The culture plates were incubated at 26 ± 2°C for 48 h. Colonies with different morphologies were selected and pure cultures were obtained (Chanchaichaovivat et al. Citation2007).
In vitro antagonistic activity of bacteria and yeasts against A. alternata
The yeasts and bacterial isolates were evaluated against A. alternata by using a dual culture technique. Each yeast or bacterium was applied as a streak near one end of the PDA plate about 1 cm from the edge and the mycelial disc of A. alternata (5 mm in diameter) taken from a vigorously growing culture was placed at the opposite end (∼1 cm from the edge) of the Petri dish. The inhibition of growth of A. alternata was measured after incubation of the plates at 26 ± 2°C for 10–12 days or until complete mycelial growth was achieved in the control plate (Chanchaichaovivat et al. Citation2007).
Scanning electron microscopy (SEM) analysis
To examine the effect of antagonistic yeast and bacteria on A. alternata hyphal morphology, 5 mm mycelial plug samples of A. alternata were taken from the edge of inhibition zone from the dual culture plate and the samples were prepared for SEM as described by Goldstein et al. (Citation2003). The specimens were examined and images were documented with a ‘JEOL Model JSM-7800F’ (JEOL, MA, USA) SEM. The culture of A. alternata grown in the absence of antagonists served as control.
Identification of antagonistic yeast
DNA was extracted from the yeast cells by following the method of Lee and Taylor (Citation1990). Quantification of DNA was done using a NanoDrop 1000 Spectrophotometer. The internal transcribed spacer (ITS) regions of the ribosomal DNA (rDNA) were amplified by PCR with primers ITS4 and ITS5 (White et al. Citation1990) as previously described (Al-Sadi et al. Citation2012). The PCR amplified product was then sequenced at Macrogen Inc., Korea. The nucleotide sequence was subjected to NCBI BLAST search (www.ncbi.nlm.nih.gov) in order to identify the fungus.
Identification of antagonistic bacteria
Identification of the antagonistic bacteria was based on the 16S rRNA gene sequences. DNA extraction was through the use of a commercial ‘foodproof StarPrep Two DNA extraction kit’ (BIOTECON Diagnostics, Germany). The universal primers 27F and 1429R (Frank et al. Citation2008) were used for amplification of 16S rRNA gene by PCR as described by Al-Hussini et al. (Citation2019). The amplified products were then sequenced at Macrogen Inc., Korea. A database search of homologous sequences was performed using the BLAST software.
Antagonistic activity of bacteria and yeast against A. alternata
Mature tomato fruits (cv. Pomodoro) were surface-disinfected with 1% NaOCl for 2 min, washed with SDW, and then air-dried in a laminar flow hood. A few wounds (3 × 3 mm) were made at the equator of each fruit. An aliquot (25 µl) of the yeast (1 × 108 CFU/ml) or bacterial cell suspension (1 × 108 CFU/ml) was applied on the wounded surface. Three hours later, the wounded surface was inoculated with a mycelial plug of A. alternata (5 mm in diameter). The treated fruits were kept at 25°C and 95% relative humidity. The diameter of the lesions was measured 7 days after challenge inoculation (Zhao et al. Citation2008). Three replicates of 4 fruits each were used per treatment (12 fruits/treatment) and the experiment was repeated twice.
Antifungal activity of volatile organic compounds (VOCs) of M. guilliermondii
The antifungal activity of VOCs produced by M. guilliermondii was tested as described by Huang et al. (Citation2011). Briefly, a 7 mm mycelial agar plug of A. alternata obtained from a 5-day-old culture was placed in the center of a PDA plate. One hundred µl of M. guilliermondii cell suspension (109 yeast cells/ml) was applied on to Sabouraud dextrose agar plate and spread evenly on the agar medium using a sterile spreader. The PDA plate with A. alternata was covered with the Sabouraud dextrose plate inoculated with the yeast and the paired Petri dishes were sealed using two layers of parafilm. The plates were incubated at 25°C for 5–7 days and the diameter of the mycelial growth of A. alternata was measured. The % inhibition of mycelial growth was calculated. For the control, PDA plate with A. alternata disc was paired with an un-inoculated Sabouraud dextrose plate.
Analysis of VOCs of M. guilliermondii
Preparation of extract
M. guilliermondii SQUCC-33Y was grown for 72 h on a rotary shaker at 25°C in 500 ml conical flasks having 200 ml of Sabouraud dextrose broth medium. After incubation, the yeast culture was extracted with ethyl acetate (1:1, v/v) by using a separating funnel. The solvent fraction was dried under vacuum at 50°C using a Buchi Rotavapor Model R-215 (Buchi Labortechnik AG, Switzerland) and the residue was weighed and dissolved in methanol.
Thin-layer chromatography (TLC) analysis
The compounds present in the above preparation were fractionated by TLC on silica gel 60 F254 pre-coated plates (Merck, Germany). The plates were developed at room temperature (25 ± 2°C) in toluene: ethyl acetate: formic acid (60:35:5, by volume) and after air-drying visualized under ultraviolet light (254 nm). The distances moved by the compounds and solvent front on the TLC plate were measured and the retention factor (Rf) of the fluorescent spot (s) was calculated (Kagan and Flythe Citation2014). The fluorescent bands at the Rf values of 0.16, 0.31, 0.44 and 0.61 were scraped separately, eluted in methanol (spectrophotometric grade) and the components of the fractions were identified using gas chromatography coupled with mass spectrometry (GC-MS).
GC-MS analysis
A PerkinElmer- Clarus 600 GC/MS, fitted with Rtx-5MS capillary column (30 m × 0.25 mm i.d. × 0.25 µm film thickness) was used for identification of compounds. Flow rate of Helium gas (grade 6.0) was set at 1.0 ml/min. One µl of the sample with a split ratio of 10:1 was injected in to the column. The ionization electron energy was 70 eV. The mass spectral scan range was set at 33–550 amu. The other conditions were as described by Al-Rawahi et al. (Citation2018). The total run time was 53.5 min. The GC/MS system was controlled with PerkinElmer TurboMass (version 6.1.0) software for run and analysis of the samples. The NIST (version 2.3) and Wiley (9th edition) mass spectrum libraries were used to identify the compounds.
Antifungal activity assay
The four fractions viz., Rf 0.16, 0.31, 0.44 and 0.61, eluted from the TLC plate were evaluated for their antifungal activity against A. alternata by determining the % inhibition of spore germination, using cavity slide method. To prepare spore suspension of A. alternata, 10 ml of SDW was added to 1-week-old PDA culture of the fungus and the spores were gently scraped with a sterile cell scraper. The resulting spore suspension was adjusted to a concentration of 104 spores ml−1 using a hemocytometer. One hundred µl of each fraction eluted from the TLC plate was placed in the cavity slide and air-dried. The spore suspension (100 µl) was then applied to the cavity and mixed well. For the control, 100 µl of spore suspension alone was applied in the cavity. The slides were then placed in Petri plates (9 cm diameter) lined with two layers of moist blotter papers to provide humidity. Three hours after incubation of the plates at 23 ± 2°C, the slides were examined under a compound microscope. The % germination of the spores was counted in different microscopic fields and the percent inhibition of spore germination was determined.
Statistical analysis
Analysis of the data was done by ‘Duncan’s multiple range test, DMRT’ using the statistical analysis software SAS v8, (SAS Institute, NC, USA) at P ≤ 0.05.
Results
Isolation of antagonists and their antifungal activity against A. alternata
A total of 72 phyllosphere and fructoplane microorganisms (24 yeasts and 48 bacteria) were isolated from tomato. In the in vitro antagonistic activity test, isolate SQUCC-33Y (yeast), isolate SQUCC-LB11 (bacterium) and isolate SQUCC-BY1 (bacterium) alone showed inhibitory activities against A. alternata and recorded mycelial growth inhibitions of 29.7%, 35.8% and 50.1%, respectively (Table ). The other isolates caused no inhibition of mycelial growth of A. alternata. The mycelial growth inhibition of A. alternata by M. guilliermondii is shown in Figure .
Figure. 1. In vitro inhibition of A. alternata by M. guilliermondii isolated from tomato phyllosphere.
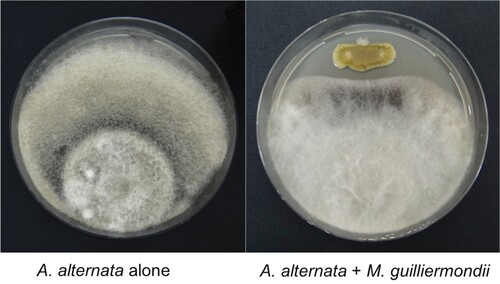
Table 1. In vitro screening of phyllosphere and fructoplane microorganisms of tomato for antagonistic potential against A. alternata.
Scanning electron microscopy (SEM) analysis
SEM studies revealed apparent morphological changes in the hyphae of A. alternata sampled from the inhibition zone compared to control (Figure ). Abnormalities such as twisting, shriveling, distortion and collapsing of hyphae were noticed in A. alternata hyphae grown in the presence of antagonists. Loss of turgidity of A. alternata hyphae was observed in general when exposed to antagonists, whereas the untreated control hyphae were normal in growth, cylindrical in shape and with a smooth surface.
Figure 2. Scanning electron micrographs showing abnormalities in the hyphae of A. alternata due to antagonistic activity of yeast and bacteria isolated from tomato phyllosphere and fructoplane. A – A. alternata grown in the absence of antagonists (control); B – A. alternata grown in the presence of E. roggenkampii; C – A. alternata grown in the presence of P. aeruginosa, D – A. alternata grown in the presence of M. guilliermondii.
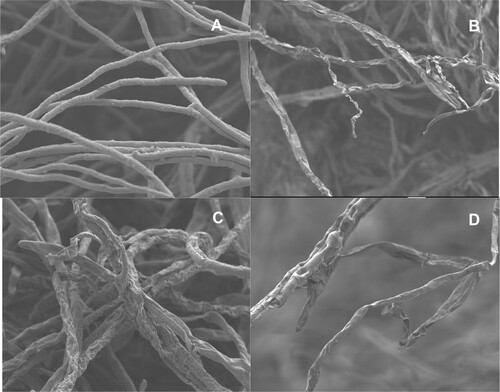
Identification of the yeast and bacterial antagonists
The yeast and bacterial isolates showing antagonistic activities were identified by ITS rDNA and 16S rDNA sequence analysis, respectively. On the basis of DNA sequences these isolates were identified as Meyerozyma guilliermondii (SQUCC-33Y) (Formerly known as Pichia guilliermondii), Enterobacter roggenkampii (SQUCC-LB11) and Pseudomonas aeruginosa (SQUCC-BY1) and the nucleotide sequence data were deposited in GenBank with the accession numbers MK583585, MK583581 and MT176503, respectively.
In vivo antagonistic activity of bacteria and yeasts against A. alternata
E. roggenkampii was the most effective antagonist for controlling Alternaria fruit rot of tomato followed by P. aeruginosa and M. guilliermondii (Table and Figure ). Tomato fruits when treated with E. roggenkampii reduced the severity of fruit rot by 60%. The treatment with P. aeruginosa and M. guilliermondii reduced the disease severity by 57 and 50%, respectively relative to infected control. Since yeasts are preferred biocontrol agents, further research on identification of antifungal metabolites of antagonist was conducted with M. guilliermondii.
Figure 3. Suppression of Alternaria fruit rot of tomato by antagonistic yeast and bacteria isolated from tomato phyllosphere and fructoplane. A – Untreated control; B – Treated with M. guilliermondii; C – Treated with P. aeruginosa, D – Treated with E. roggenkampii.
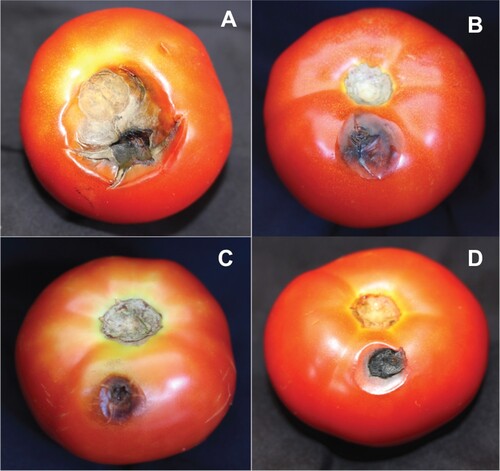
Table 2. Effect of antagonistic yeast and bacteria isolated from tomato on control of fruit rot of tomato caused by A. alternata.
Antifungal activity of VOCs of M. guilliermondii
The production of antifungal VOCs by M. guilliermondii was tested in vitro using double dishes method. The results revealed that the VOCs of M. guilliermondii inhibited the mycelial growth of A. alternata and recorded an inhibition of 38.7% compared to control (data not shown). TLC analysis of the ethyl acetate extract of M. guilliermondii culture revealed the appearance of four fluorescent bands with Rf values of 0.16, 0.31, 0.44 and 0.61 (Figure ). Each fraction was recovered from the TLC plate and tested for in vitro antifungal activity against A. alternata by using spore germination inhibition assay. The results revealed that all the four fractions significantly inhibited the spore germination and resulted in 67-77% inhibition over control (Table ). Analysis of the bioactive fractions recovered from the TLC plate by GC-MS revealed the presence of several alkanes (Table ; Figure ). The major compounds identified in all the four fractions were pentacosane and tricosane. Besides alkanes, palmitic acid, 2-(tetradecyloxy) ethyl ester was identified in the fraction with Rf value of 0.31. The MS library match quality was more than 80% for all the compounds.
Figure 5. Gas chromatogram of the biologically active fractions recovered from the TLC plate. A – Rf 0.16; B – Rf 0.31; C – Rf 0.44; D – Rf 0.61.
Table 3. Inhibition of spore germination of A. alternata by TLC fractions obtained from ethyl acetate extract of M. guilliermondii SQUCC-33Y.
Table 4. Compounds identified in the bioactive fractions obtained from ethyl acetate extract of M. guilliermondii.
Discussion
It is evident from the present study that the native microorganisms viz., M. guilliermondii, E. roggenkampii and P. aeruginosa isolated from tomato phyllosphere and fructoplane showed in vitro inhibitory activity against A. alternata. Several antagonistic yeasts produce lytic enzymes such as chitinases (Saravanakumar et al. Citation2009; Banani et al. Citation2015), glucanases (Lopes et al. Citation2015; Zhang et al. Citation2011), lipases (Sommer et al. Citation2016) and proteases (Banani et al. Citation2014) and other fungitoxic metabolites (Prasongsuk et al. Citation2018). Similarly, P. aeruginosa produces several secondary metabolites including pyocyanin and phenazine-1-carboxylic acid (PCA) that show strong antimicrobial activities (Illakkiam et al. Citation2013; Spago et al. Citation2014; Simionato et al. Citation2017). Some species of Enterobacter, such as E. agglomerans are reported to produce chitinolytic enzymes and antibiotic pyrrolnitrin, which show broad-spectrum antifungal and antibacterial activities (Chernin et al. Citation1995; Chernin et al. Citation1996). The restriction of mycelial growth of A. alternata and formation of inhibition zone in the dual culture may be attributed to secretion of diffusible antifungal substances by these antagonistic yeasts and bacteria (Freimoser et al. Citation2019; Kohl et al. Citation2019).
The SEM observations of the hyphae of A. alternata showed morphological abnormalities such as shrinkage, distortion and loss of turgidity. These results agree with the findings of Minaxi (Citation2010) who reported lysis of Macrophomina phaseolina hyphae due to the effect of P. aeruginosa. Deformations and loss of cytoplasmic contents from the hyphae of Pythium aphanidermatum due to antagonistic effect of Aspergillus terreus have been reported by Al-Shibli et al. (Citation2019). Al-Badri et al. (Citation2020) demonstrated that antagonistic strains of Bacillus subtilis and B. cereus induced pit formation, shrinkage and loss of turgidity on the hyphae of some fungi, including Rhizoctonia solani and Neocosmospora keratoplastica. Al-Daghari et al. (Citation2020) reported morphological deformities on the hyphae of P. aphanidermatum due to antagonistic activities of Pseudomonas resinovorans and P. aeruginosa. Alterations in the intracellular osmotic pressure in general lead to distortion of fungal hyphae. The shrinkage of A. alternata hyphae in the present study suggests a possible loss of cytoplasmic volume (Garg et al. Citation2010). The disintegration or collapsing of hyphae of A. alternata in the inhibition zone suggests changes in cell membrane permeability (Halo et al. Citation2018). The results of the present study suggest that M. guilliermondii, E. roggenkampii and P. aeruginosa exert their antagonism towards A. alternata through secretion of diffusible antifungal metabolites and lytic enzymes, which cause damage to fungal membrane and the leakage of cytoplasmic contents (Nagarajkumar et al. Citation2004; Chaurasia et al. Citation2005).
In the present investigation, post-harvest treatment of tomato fruits with the native antagonists isolated from tomato significantly reduced the severity of Alternaria fruit rot. Among the antagonistic microorganisms tested, E. roggenkampii was the most effective for controlling Alternaria fruit rot of tomato followed by P. aeruginosa and M. guilliermondii. However, the differences in their efficacies were not statistically significant (P < 0.05). A few reports have shown that species of Enterobacter such as E. cloacae, E. cowanii and E. agglomerans could be used as biocontrol agents for fungal plant pathogens (Hadar et al. Citation1983; Park and Kim Citation1989; Shi and Sun Citation2017). On the other hand, E. sakazakii has been described as an opportunistic foodborne pathogen (Healy et al. Citation2010). Similarly, several strains of P. aeruginosa have been reported as opportunistic human pathogens (De Bentzmann and Plesiat Citation2011). Yeasts are considered as ideal biocontrol agents of post-harvest diseases of fruits and vegetables because they (i) colonize rapidly and survive for long periods of time on the surfaces of fruits and vegetables under different environmental conditions; (ii) proliferate quickly by utilizing available nutrients and thereby restricting availability of nutrients to the pathogens; (iii) are generally not affected by fungicides commonly used under postharvest conditions; (iv) are easy to produce in large-scale on inexpensive culture media due to their fast growing trait; and (v) do not produce allergenic spores, toxins or antibiotics (Wilson and Wisniewski Citation1989; El-Tarabily and Sivasithamparam Citation2006; Pretscher et al. Citation2018; Freimoser et al. Citation2019). Furthermore, yeast exerts beneficial effects in foods because it consists of high amounts of vitamins, essential amino acids and minerals (Chanchaichaovivat et al. Citation2007). The United States Food and Drug Administration classified the yeast strain C. guillermondii (ATCC No. 20474) as a non-pathogenic and non-toxigenic organism, making it microbiologically safe (FDA Citation2013). A number of yeasts, including Candida oleophila (Lima et al. Citation1997), Pichia guilliermondii (Wszelaki and Mitcham Citation2003), Candida intermedia (Huang et al. Citation2011), C. ciferrii (Vero et al. Citation2002), C. saitoana (El-Ghaouth et al. Citation2003), C. sake (Nunes et al. Citation2002), Debaryomyces hansenii (Droby et al. Citation1989), Metschnikowia pulcherrima (Saravanakumar et al. Citation2008), Pichia kluyveri, Hanseniaspora clermontiae and H. uvarum (Cordero-Bueso et al. Citation2017) and Wickerhamomyces sp. (Perez et al. Citation2016) are reported as biocontrol agents against fungal postharvest diseases. Lahlali et al. (Citation2011) reported the efficacy of Pichia guilliermondii strain Z1 against blue mold of citrus. Celis Zambrano et al. (Citation2014) demonstrated the potential of Candida guilliermondii in controlling Rhizopus stolonifer in postharvest tomato.
Several mechanisms such as direct inhibition of hyphal growth and germination of conidia, production and release of lytic enzymes and toxins, production of VOCs and triggering of host plant defense mechanisms are proposed for the biocontrol activity of yeasts against fungal plant pathogens (Freimoser et al. Citation2019). In our study, the involvement of VOCs produced by M. guilliermondii in inhibiting A. alternata growth was demonstrated by double dishes method. The partially purified compounds from the culture extracts of M. guilliermondii exhibited strong inhibitory effect on A. alternata conidial germination. Furthermore, the presence of several hydrocarbons, more specifically tricosane and pentacosane in the biologically active fractions was demonstrated by GC-MS analysis. Previous studies have shown that VOCs produced by antagonistic yeasts could inhibit the growth and sporulation of fungal pathogens (Buck Citation2002; Fialho et al. Citation2010; Di Francesco et al. Citation2015; Contarino et al. Citation2019), suggesting that VOCs produced by these yeasts could be another mechanism of control of Alternaria fruit rot of tomato. Contarino et al. (Citation2019) reported the production of VOCs such as alcohols (phenylethyl alcohol and ethyl alcohol) and esters (isoamyl acetate and ethyl acetate) by biocontrol yeast strains viz., Aureobasidium pullulans, Metschnikowia pulcherrima, Saccharomyces cerevisiae and Wickerhamomyces anomalus. Di Francesco et al. (Citation2015) demonstrated the production of antifungal volatiles such as 1-butanol-3-methyl, 2-phenyl and 1-butanol-2-methyl by biocontrol yeast species A. pullulans.
VOCs of yeasts have been suggested as ideal antimicrobial compounds since physical contact between the yeast antagonists and the postharvest pathogens or between the yeast antagonists and harvested fruits or vegetables is not necessary for their biocontrol activity (Contarino et al. Citation2019). Parafati et al. (Citation2017) suggested that VOC-producing biocontrol yeasts could be used as bio-emitters in postharvest disease management. Our findings are exciting since the compounds tricosane and pentacosane have been identified predominantly in plants and in a few actinobacteria (Geissberger and Sequin Citation1991). Marrufo et al. (Citation2013) reported the presence of pentacosane and tricosane in the essential oil of Moringa oleifera that exhibit antimicrobial activity. Fitsiou et al. (Citation2007) reported that the essential oils of Tilia cordata, which showed antibacterial activities, contained predominantly hydrocarbons (87.4%) with tricosane (32.2%) as the main constituents. El-Naggar et al. (Citation2017) demonstrated the presence of many alkanes including tricosane and pentacosane as constituents in the fermentation broth of a strain of Streptomyces anulatus showing antagonistic activity against Staphylococcus aureus. The hydrocarbons viz., tricosane and pentacosane present in the bioactive fractions of M. guilliermondii culture extract in this study could be involved in the antifungal activity (Kalemba and Kunicka Citation2003; Marrufo et al. Citation2013). This is the first study to report the production of alkanes viz., tricosane and pentacosane by antagonistic yeast M. guilliermondii.
In summary, our data show that the yeast strain M. guilliermondii has potent antifungal activity as demonstrated by in vitro inhibitory activity against A. alternata and induction of morphological abnormalities in the hyphae of the fungus. Further, post-harvest treatment of tomato fruits with M. guilliermondii significantly reduced the Alternaria fruit rot. The present investigation was carried out under high inoculum conditions. Under normal postharvest conditions, treatment of tomato with M. guilliermondii would be expected to give better protection against fruit rot because of usually low inoculum levels. Further studies on M. guilliermondii as a biocontrol agent for management of Alternaria- induced postharvest diseases other fruits and vegetables are warranted. Furthermore, we demonstrated the production of VOCs, specifically alkanes by M. guilliermondii, many of which have known antimicrobial activity. The antagonistic activity of M. guilliermondii might be due to the synergistic effects of diffusible and volatile antimicrobial compounds. Further studies are ongoing to investigate the efficacy of pre-harvest application of M. guilliermondii in managing postharvest Alternaria fruit rot of tomato.
Authors’ contributions
RV, AMA, SSA designed the study, SSA, SAKA, JNA conducted lab experiments, RV, AMA supervised the research project, SSA, RV, AMA, JNA wrote the manuscript.
Ethical approval
This article is original and has not been published elsewhere. All authors discussed the results, read and approved the final manuscript. The authors confirm that there are no ethical issues in the publication of the manuscript.
Acknowledgements
We thank Ms Huda Khalfan Al-Ruqaishi for helping in GC-MS analysis. The authors thank Dr S. Muthukrishnan, Kansas State University, USA for critically reading the manuscript.
Disclosure statement
No potential conflict of interest was reported by the author(s).
Data availability
All data generated or analyzed during this study are included in this article. The nucleotide sequences of antagonistic yeast and bacteria have been deposited in the NCBI database under GenBank accession numbers MK583585, MK583581, and MT176503. The nucleotide sequence of Alternaria alternata SQUCC-S4 can be found in the NCBI database (www.ncbi.nlm.nih.gov) under GenBank accession number MK583589.
Additional information
Funding
References
- FAO. 2020. FAOSTAT. Available online at: http://www.fao.org/faostat/en/#data/QC/visualize.
- FDA. 2013. CFR-Code of Federal Regulations Title 21. http://www.accessdata.fda.gov/scripts/cdrh/cfdocs/cfcfr/CFRSearch.cfm?fr=173.160.
- Al-Badri BAS, Al-Maawali SS, Al-Balushi ZM, Al-Mahmooli IH, Al-Sadi AM, Velazhahan R. 2020. Cyanide degradation and antagonistic potential of endophytic Bacillus subtilis strain BEB1 from Bougainvillea spectabilis Willd. Front Life Sci. 13:92–98.
- Al-Daghari DSS, Al-Abri SA, Al-Mahmooli IH, Al-Sadi AM, Velazhahan R. 2020. Efficacy of native antagonistic rhizobacteria in the biological control of Pythium aphanidermatum-induced damping-off of cucumber in Oman. J Plant Pathol. 102:305–310.
- Al-Hussini HS, Al-Rawahi AY, Al-Marhoon AA, Al-Abri SA, Al-Mahmooli IH, Al- Sadi AM, Velazhahan R. 2019. Biological control of damping-off of tomato caused by Pythium aphanidermatum by using native antagonistic rhizobacteria isolated from Omani soil. J Plant Pathol. 101:315–322.
- Al-Rawahi AY, Al-Mahmooli IH, Al-Sadi AM, Al-Sabahi JN, Velazhahan R. 2018. Toxin production by melon root rot fungus, Monosporascus cannonballus. Australas Plant Pathol. 47:543–546.
- Al-Sadi AM, Al-Ghaithi AG, Al-Balushi ZM, Al-Jabri AH. 2012. Analysis of diversity in Pythium aphanidermatum populations from a single greenhouse reveals phenotypic and genotypic changes over 2006 to 2011. Plant Dis. 96:852–858.
- Al-Shibli H, Dobretsov S, Al-Nabhani A, Maharachchikumbura S, Velazhahan R, Al-Sadi AM. 2019. Aspergillus terreus obtained from mangrove exhibits antagonistic activities against Pythium aphanidermatum-induced damping-off of cucumber. Peer J. 7:e7884.
- Alkan N, Fortes AM. 2015. Insights into molecular and metabolic events associated with fruit response to post-harvest fungal pathogens. Front Plant Sci. 6:889.
- Banani H, Spadaro D, Zhang D, Matic S, Garibaldi A, Gullino ML. 2014. Biocontrol activity of an alkaline serine protease from Aureobasidium pullulans expressed in Pichia pastoris against four postharvest pathogens on apple. Int J Food Microbiol. 182:1–8.
- Banani H, Spadaro D, Zhang D, Matic S, Garibaldi A, Gullino ML. 2015. Postharvest application of a novel chitinase cloned from Metschnikowia fructicola and overexpressed in Pichia pastoris to control brown rot of peaches. Int J Food Microbiol. 199:54–61.
- Barth M, Hankinson TR, Zhuang H, Breidt F. 2009. Microbiological spoilage of fruits and vegetables. In: Sperber WH, Doyle MP, editor. Compendium of the microbiological spoilage of foods and beverages. New York: Springer; p. 135–183.
- Buck JW. 2002. In vitro antagonism of Botrytis cinerea by phylloplane yeasts. Can J Bot. 80:885–891.
- Celis Zambrano C, Moreno Duran G, Sequeda-Castaneda LG, Garcia Caicedo A, Albarracín DM, Barreto Charry LC. 2014. Determining the effectiveness of Candida guilliermondii in the biological control of Rhizopus stolonifer in postharvest tomatoes. Univ Sci. 19:51–62.
- Chanchaichaovivat A, Ruenwongsa P, Panijpan B. 2007. Screening and identification of yeast strains from fruits and vegetables: potential for biological control of postharvest chilli anthracnose (Colletotrichum capsici). Biol Control. 42:326–335.
- Chaurasia B, Pandey A, Palni LMS, Trivedi P, Kumar B, Colvin N. 2005. Diffusible and volatile compounds produced by an antagonistic Bacillus subtilis strain cause structural deformations in pathogenic fungi in vitro. Microbiol Res. 160:75–81.
- Chernin L, Brandis A, Ismailov Z, Chet I. 1996. Pyrrolnitrin production by an Enterobacter agglomerans strain with a broad spectrum of antagonistic activity towards fungal and bacterial phytopathogens. Curr Microbiol. 32:208–212.
- Chernin L, Ismailov Z, Haran S, Chet I. 1995. Chitinolytic Enterobacter agglomerans antagonistic to fungal plant pathogens. Appl Environ. Microbiol. 61:1720–1726.
- Contarino R, Brighina S, Fallico B, Cirvilleri G, Parafati L, Restuccia C. 2019. Volatile organic compounds (VOCs) produced by biocontrol yeasts. Food Microbiol. 82:70–74.
- Cordero-Bueso G, Mangieri N, Maghradze D, Foschino R, Valdetara F, Cantoral JM, Vigentini I. 2017. Wild grape-associated yeasts as promising biocontrol agents against Vitis vinifera fungal pathogens. Front Microbiol. 8:2025.
- da Cruz Cabral L, Pinto VF, Patriarca A. 2016. Control of infection of tomato fruits by Alternaria and mycotoxin production using plant extracts. Eur J Plant Pathol. 145:363–373.
- De Bentzmann S, Plesiat P. 2011. The Pseudomonas aeruginosa opportunistic pathogen and human infections. Environ Microbiol. 13:1655–1665.
- Di Francesco A, Ugolini L, Lazzeri L, Mari M. 2015. Production of volatile organic compounds by Aureobasidium pullulans as a potential mechanism of action against postharvest fruit pathogens. Biol Control. 81:8–14.
- Droby S, Chalutz E, Wilson CL, Wisniewski ME. 1989. Characterization of the biocontrol activity of Debaryomyces hansenii in the control of Penicillium digitatum on grapefruit. Can J Microbiol. 35:794–800.
- El-Ghaouth A, Wilson CL, Wisniewski M. 2003. Control of postharvest decay of apple fruit with Candida saitoana and induction of defense responses. Phytopathology. 93:344–348.
- El-Naggar NEA, El-Bindary AAA, Abdel-Mogib M, Nour NS. 2017. In vitro activity, extraction, separation and structure elucidation of antibiotic produced by Streptomyces anulatus NEAE-94 active against multidrug-resistant Staphylococcus aureus. Biotechnol Biotechnol Equip. 31:418–430.
- El-Tarabily KA, Sivasithamparam K. 2006. Potential of yeasts as biocontrol agents of soil-borne fungal plant pathogens and as plant growth promoters. Mycoscience. 47:25–35.
- Escriva L, Oueslati S, Font G, Manyes L. 2017. Alternaria mycotoxins in food and feed: an overview. J. Food Qual. 2017:1569748.
- Fialho MB, Toffano L, Pedroso MP, Augusto F, Pascholati SF. 2010. Volatile organic compounds produced by Saccharomyces cerevisiae inhibit the in vitro development of Guignardia citricarpa, the causal agent of citrus black spot. World J Microbiol. Biotechnol. 26:925–932.
- Fitsiou L, Tzakou O, Hancianu M, Poiata A. 2007. Volatile constituents and antimicrobial activity of Tilia tomentosa Moench and Tilia cordata Miller oils. J Essent Oil Res. 19:183–185.
- Frank JA, Reich CI, Sharma S, Weisbaum JS, Wilson BA, Olsen GJ. 2008. Critical evaluation of two primers commonly used for amplification of bacterial 16S rRNA genes. Appl Environ Microbiol. 74:2461–2470.
- Freimoser FM, Rueda-Mejia MP, Tilocca B, Migheli Q. 2019. Biocontrol yeasts: mechanisms and applications. World J Microbiol Biotechnol. 35:154.
- Garg H, Li H, Sivasithamparam K, Kuo J, Barbetti MJ. 2010. The infection processes of Sclerotinia sclerotiorum in cotyledon tissue of a resistant and a susceptible genotype of Brassica napus. Ann Bot. 106:897–908.
- Geissberger P, Sequin U. 1991. Constituents of Bidens pilosa L.: do the components found so far explain the use of this plant in traditional medicine? Acta Trop. 48:251–261.
- Goldstein J, Newbury DE, Joy DC, Lyman CE, Echlin P, Lifshin E, Sawyer L, Michael JR. 2003. Scanning electron microscopy and X-Ray microanalysis, 3rd ed. Berlin: Springer; p. 689.
- Hadar Y, Harman GE, Taylor AG, Norton JM. 1983. Effects of pre-germination of pea and cucumber seeds and of seed treatment with Enterobacter cloacae on rots caused by Pythium spp. Phytopathology. 73:1322–1325.
- Halo BA, Al-Yahyai RA, Al-Sadi AM. 2018. Aspergillus terreus inhibits growth and induces morphological abnormalities in Pythium aphanidermatum and suppresses Pythium-induced damping-off of cucumber. Front Microbiol. 9:95.
- Healy B, Cooney S, O’Brien S, Iversen C, Whyte P, Nally J, Callanan JJ, Fanning S. 2010. Cronobacter (Enterobacter sakazakii): an opportunistic foodborne pathogen. Foodborne Pathog Dis. 7:339–350.
- Huang R, Li GQ, Zhang J, Yang L, Che HJ, Jiang DH, Huang HC. 2011. Control of postharvest Botrytis fruit rot of strawberry by volatile organic compounds of Candida intermedia. Phytopathology. 101:859–869.
- Illakkiam D, Ponraj P, Shankar M, Muthusubramanian S, Rajendhran J, Gunasekaran P. 2013. Identification and structure elucidation of a novel antifungal compound produced by Pseudomonas aeruginosa PGPR2 against Macrophomina phaseolina. Appl Biochem. Biotechnol. 171:2176–2185.
- Ippolito A, Nigro F. 2000. Impact of preharvest application of biological control agents on postharvest diseases of fresh fruits and vegetables. Crop Prot. 19:715–723.
- Kagan IA, Flythe MD. 2014. Thin-layer chromatographic (TLC) separations and bioassays of plant extracts to identify antimicrobial compounds. J Vis Exp. 85:51411.
- Kalemba D, Kunicka A. 2003. Antibacterial and antifungal properties of essential oils. Curr Med Chem. 10:813–829.
- Karabulut OA, Tezcan H, Daus A, Cohen L, Wiess B, Droby S. 2004. Control of preharvest and postharvest fruit rot in strawberry by Metschnikowia fructicola. Biocontrol Sci Technol. 14:513–521.
- Kohl J, Kolnaar R, Ravensberg WJ. 2019. Mode of action of microbial biological control agents against plant diseases: relevance beyond efficacy. Front Plant Sci. 10:845.
- Lahlali R, Hamadi Y, Jijakli MH. 2011. Efficacy assessment of Pichia guilliermondii strain Z1, a new biocontrol agent, against citrus blue mould in Morocco under the influence of temperature and relative humidity. Biol Control. 56:217–224.
- Larena I, Torres R, De Cal A, Linan M, Melgarejo P, Domenichini P, Bellini A, Mandrin JF, Lichou J, De Eribe XO, Usall J. 2005. Biological control of postharvest brown rot (Monilinia spp.) of peaches by field applications of Epicoccum nigrum. Biol Control. 32:305–310.
- Lee SB, Taylor JW. 1990. Isolation of DNA from fungal mycelia and single spores. In: Innis MA, Gelfand DH, Sninsky JJ, White TJ, editor. PCR protocols: A guide to methods and applications. San Diego: Academic Press; p. 282–287.
- Lima G, Ippolito A, Nigro F, Salerno M. 1997. Effectiveness of Aureobasidium pullulans and Candida oleophila against postharvest strawberry rots. Postharvest Biol Technol. 10:169–178.
- Lopes MR, Klein MN, Ferraz LP, da Silva AC, Kupper KC. 2015. Saccharomyces cerevisiae: a novel and efficient biological control agent for Colletotrichum acutatum during pre-harvest. Microbiol Res. 175:93–99.
- Marrufo T, Nazzaro F, Mancini E, Fratianni F, Coppola R, De Martino L, Agostinho AB, De Feo V. 2013. Chemical composition and biological activity of the essential oil from leaves of Moringa oleifera Lam. cultivated in Mozambique. Molecules. 18:10989–11000.
- Meena M, Swapnil P, Upadhyay RS. 2017. Isolation, characterization and toxicological potential of Alternaria-mycotoxins (TeA, AOH and AME) in different Alternaria species from various regions of India. Sci Rep. 7:8777.
- Minaxi SJ. 2010. Characterization of Pseudomonas aeruginosa RM-3 as a potential biocontrol agent. Mycopathologia. 170:181–193.
- Nagarajkumar M, Bhaskaran R, Velazhahan R. 2004. Involvement of secondary metabolites and extracellular lytic enzymes produced by Pseudomonas fluorescens in inhibition of Rhizoctonia solani, the rice sheath blight pathogen. Microbiol Res. 159:73–81.
- Nunes CA. 2012. Biological control of postharvest diseases of fruit. Eur J Plant Pathol. 133:181–196.
- Nunes C, Usall J, Teixido N, Abadias M, Vinas I. 2002. Improved control of postharvest decay of pears by the combination of Candida sake (CPA-1) and ammonium molybdate. Phytopathology. 92:281–287.
- Parafati L, Vitale A, Restuccia C, Cirvilleri G. 2017. Performance evaluation of volatile organic compounds by antagonistic yeasts immobilized on hydrogel spheres against gray, green and blue postharvest decays. Food Microbiol. 63:191–198.
- Park JH, Kim HK. 1989. Biological control of Phytophtora crown and root rot of greenhouse pepper with Trichoderma harzianum and Enterobacter agglomerans by improved method of application. Korean J. Plant Pathol. 5:1–12.
- Pearson RC, Hall DH. 1975. Factors affecting the occurrence and severity of black mold of ripe tomato fruit by Alternaria alternata. Phytopathology. 65:1352–1359.
- Perez MF, Contreras L, Garnica NM, Fernández-Zenoff MV, Farías ME, Sepulveda M, Ramallo J, Dib JR. 2016. Native killer yeasts as biocontrol agents of postharvest fungal diseases in lemons. PLoS one. 11:e0165590.
- Prasongsuk S, Lotrakul P, Ali I, Bankeeree W, Punnapayak H. 2018. The current status of Aureobasidium pullulans in biotechnology. Folia Microbiol. 63:129–140.
- Pretscher J, Fischkal T, Branscheidt S, Jager L, Kahl S, Schlander M, Thines E, Claus H. 2018. Yeasts from different habitats and their potential as biocontrol agents. Fermentation. 4:31.
- Saravanakumar D, Ciavorella A, Spadaro D, Garibaldi A, Gullino ML. 2008. Metschnikowia pulcherrima strain MACH1 outcompetes Botrytis cinerea, Alternaria alternata and Penicillium expansum in apples through iron depletion. Postharvest Biol. Technol. 49:121–128.
- Saravanakumar D, Spadaro D, Garibaldi A, Gullino ML. 2009. Detection of enzymatic activity and partial sequence of a chitinase gene in Metschnikowia pulcherrima strain MACH1 used as post-harvest biocontrol agent. Eur J Plant Pathol. 123:183–193.
- Shi JF, Sun CQ. 2017. Isolation, identification, and biocontrol of antagonistic bacterium against Botrytis cinerea after tomato harvest. Braz J Microbiol. 48:706–714.
- Simionato AS, Navarro MOP, de Jesus MLA, Barazetti AR, da Silva CS, Simões GC, Balbi-Peña MI, de Mello JCP, Panagio LA, de Almeida RSC, et al. 2017. The effect of phenazine-1-carboxylic acid on mycelial growth of Botrytis cinerea produced by Pseudomonas aeruginosa LV strain. Front Microbiol. 8:1102.
- Singh VK, Singh AK, Kumar A. 2017. Disease management of tomato through PGPB: current trends and future perspective. 3 Biotech. 7:255.
- Sommer B, Overy DP, Haltli B, Kerr RG. 2016. Secreted lipases from Malassezia globosa: recombinant expression and determination of their substrate specificities. Microbiology. 162:1069–1079.
- Spago FR, Mauro CSI, de Oliveira AG, Beranger JPO, Cely MVT, Stanganelli MM, Simionato AS, San Martin JAB, Andrade CGTJ, Mello JCP, Andrade G. 2014. Pseudomonas aeruginosa produces secondary metabolites that have biological activity against plant pathogenic Xanthomonas species. Crop Prot. 62:46–54.
- Spalding DH. 1980. Control of Alternaria rot of tomatoes by postharvest application of imazalil. Plant Dis. 64:169–171.
- Vero S, Mondino P, Burgueño J, Soubes M, Wisniewski M. 2002. Characterization of biocontrol activity of two yeast strains from Uruguay against blue mold of apple. Postharvest Biol Technol. 26:91–98.
- White TJ, Bruns T, Lee S, Taylor J. 1990. Amplification and direct sequencing of fungal ribosomal RNA genes for phylogenetics. In: Innis MA, Gelfand DH, Sninsky JJ, White TJ, editor. PCR protocols: A guide to methods and applications. San Diego: Academic Press; p. 315–322.
- Wilson CL, Wisniewski ME. 1989. Biological control of postharvest diseases of fruits and vegetables: an emerging technology. Ann Rev Phytopathol. 27:425–441.
- Wszelaki AL, Mitcham EJ. 2003. Effect of combinations of hot water dips, biological control and controlled atmospheres for control of gray mold on harvested strawberries. Postharvest Biol Technol. 27:255–264.
- Zhang H, Mahunu GK, Castoria R, Yang Q, Apaliya MT. 2018. Recent developments in the enhancement of some postharvest biocontrol agents with unconventional chemicals compounds. Trends Food Sci Technol. 78:180–187.
- Zhang D, Spadaro D, Garibaldi A, Gullino ML. 2011. Potential biocontrol activity of a strain of Pichia guilliermondii against grey mold of apples and its possible modes of action. Biol Control. 57:193–201.
- Zhao Y, Tu K, Shao XF, Jing W, Su ZP. 2008. Effects of the yeast Pichia guilliermondii against Rhizopus nigricans on tomato fruit. Postharvest Biol Technol. 49:113–120.