Abstract
Abnormal proliferation and migration of human airway smooth-muscle cells (hASMC) play crucial roles in child asthma. We investigated the effect of Six-ingredient-Xiao-qing-long decoction (SXD) on the transforming growth factor (TGF)-β1-stimulated hASMC. Cell viability and migration of TGF-β1-induced hASMC were determined by CCK-8 and transwell assays. The expressions of FK506 binding protein 51 (FKBP51), protein kinase B (AKT), p-AKT were determined by RT-qPCR and western blotting. In addition, FKBP51 overexpression (oeFKBP51) and FKBP51 knockdown (siFKBP51) were carried out to further confirm the results. SXD dose-dependently suppressed the cell proliferation and migration of TGF-β1-induced hASMC. TGF-β1 markedly inhibited the mRNA and protein levels of FKBP51 in a time-dependent manner. Nevertheless, compared to the TGF-β1 group, SXD increased the expression of FKBP51 while suppressed p-AKT. Moreover, both oeFKBP51 and SXD reduced the migration ability of TGF-β1-induced hASMC, as well as elevated the expressions of FKBP51 but decreased p-AKT. Finally, siFKBP51 promoted cell migration and caused the up-regulation of p-AKT in hASMC, but SXD could reverse these changes. SXD could suppress the proliferation and migration of hASMC via activating FKBP51/AKT signaling, which may provide a novel possible target for child asthma therapy.
Abbreviations: SXD, Six-ingredient-Xiao-qing-long decoction; hASMC, human airway smooth muscle cells; TGF-β1, transforming growth factor β1; ECM, extracellular matrix; ERK, extracellular signal-regulated kinase; JNK, C-Jun NH(2)-terminal kinase; FKBP51, FK506 binding protein 51; TCM, traditional Chinese medicine; oeFKBP51, FK506 binding protein 51 overexpression; PI3K, phosphatidylinositol 3-kinase; AKT, protein kinase B; p-AKT, phosphorylation AKT; qRT-PCR, real-time fluorescence quantitative PCR; TCM, traditional Chinese medicine; CCK-8, Cell Counting Kit-8, SRA, Steroid-resistant asthma
Introduction
Asthma is a chronic inflammatory disease characterized by airway obstruction and bronchial hyperresponsiveness. Clinically, it is manifested as respiratory symptoms, such as recurrent wheezing, cough, chest tightness and shortness of breath (Ish et al. Citation2020). The incidence of asthma in children shows an increasing trend year by year, and its pathological basis is related to airway hyperreactivity, inflammation, airway obstruction and airway remodeling caused by lymphocytes, mast cells and eosinophils (Malmström et al. Citation2013; Noutsios and Floros Citation2014). Although the pathogenesis of childhood asthma is roughly the same as that of adults, childhood asthma has a higher recovery rate and better prognosis than adults. Nevertheless, the clinical symptoms alleviated with age are not equal to the disappearance of underlying pathology, and childhood asthma is very likely to develop into adult asthma under various inducements (Fuchs et al. Citation2017). The treatment principles of childhood asthma are symptom control and maintenance of normal activity levels, while treatment of adult asthma focuses on relieving symptoms and improving quality of life. Currently, inhaled corticosteroids are the first choice for prevention and treatment of childhood asthma. Furthermore, the application of advanced omics techniques (genomics, epigenomics, transcriptomics, and metabolomics) provides potential clinical biomarkers for the precise treatment of childhood asthma (Golebski et al. Citation2020). Nevertheless, the existing treatment methods still have significant side effects and a high frequency of recurrence (Allen Citation2020). In this case, the combination of safe and effective natural medicines could improve therapeutic efficacy of childhood asthma (Wang et al. Citation2019).
In the pathophysiology of asthma, repeated injury and repair during airway remodeling lead to changes in airway wall structure, which are mainly characterized by airway smooth muscle hyperplasia, migration and hypertrophy, extracellular matrix deposition, mucosal hypertrophy, airway wall thickening, inflammatory cell infiltration, etc. (Hirota and Martin Citation2013). And abnormal proliferation and migration of hASMC in the progress of airway remodeling will lead to airway stenosis and airflow obstruction (Bentley and Hershenson Citation2008). The cytokine transforming growth factor (TGF)-β1 is an important mediator involved in the proinflammatory response and airway remodeling of asthma. In previous studies, both real-time PCR and immunohistochemistry showed higher levels of TGF-β1 in asthmatic patients than in non-asthmatic patients (Xie et al. Citation2007). Furthermore, Ojiaku et al. suggested that TGF-β1 could enhance hASMC excitation-contraction coupling pathways to induce excessive airway narrowing and hyperresponsiveness (Ojiaku et al. Citation2018). TGF-β1 could promote the proliferation and migration of hASMC by activating extracellular signal-regulated kinase (ERK), p38MAPK, C-Jun NH(2)-terminal kinase (JNK) and Smad2/3 signaling pathway leading to airway remodeling (Baarsma et al. Citation2011; Pan et al. Citation2018). Therefore, TGF-β1 has been used to induce abnormal proliferation and migration of hASMC to construct airway remodeling model in many in vitro studies (Li et al. Citation2015; Gao et al. Citation2018; Liu et al. Citation2019).
FK506 binding protein 51 (FKBP51), a 51-kDa protein that belongs to the FK506-binding proteins family, is a steroid hormone receptor signaling regulator and is expressed in various tissues and cell types (Stechschulte and Sanchez Citation2011). Tajiri et al. reported that the lung function of asthmatic patients was significantly improved after glucocorticosteroid treatment, which was correlated with reduced expression of FKBP51 mRNA in airway epithelial cells, peripheral blood mononuclear cells and sputum cells (Tajiri et al. Citation2013). The protein kinase B (AKT) is a serine/threonine protein kinase that regulates various cellular functions, including cell growth, cell migration, cell cycle, protein synthesis, glycogen synthesis and metabolism (Liao and Hung Citation2010). It has been reported that the phosphatidylinositol 3-kinase (PI3K)/AKT pathways and the expression level of FKBP51 may regulate hASMC proliferation and migration in airway remodeling (Woodruff et al. Citation2007; Liu et al. Citation2018). Recent studies showed that FKBP51 participate in regulating cell proliferation, tumorigenesis & chemoresistance, decidualization of human endometrial stromal cells and glucocorticoid-induced skin atrophy by decreasing Akt signaling (Luo et al. Citation2017; Boulet Citation2018; Wei et al. Citation2018). However, the effects of FKBP51/AKT signaling pathway on TGF-β1 induced hASMC proliferation and migration remain unknown.
Xiao-qing-long decoction (XQLD) is a famous formula in traditional Chinese medicine (TCM) from Shang-Han-Lun (Chang et al. Citation2018), and Six-ingredient-Xiao-qing-long decoction (SXD) derived from XQLD. The components of SXD include Ephedra sinica Stapf, Pinellia ternata Breitenbach, Schisandra chinensis Baill, Glycyrrhiza uralensis Fisch, Zingiber officinale Roscoe and Asarum sieboldii Miq. The effectiveness of XQLD on asthma has been studied in both clinical trials and animal models, which mainly focused on XQLD’s ability to relieve inflammation and anaphylaxis, inhibit mucus hypersecretion and increase the clearance function of ciliated epithelial cells within the respiratory tract (Zhang et al. Citation2003; Qi et al. Citation2017; Song et al. Citation2018). However, as a common traditional Chinese medicine for pediatric respiratory diseases in China, the underlying molecular mechanism of SXD in the treatment of childhood asthma has not been reported, which limits its further application.
In the present study, we aimed to investigate the effects of SXD on TGF-β1-induced hASMC abnormal proliferation and migration in vitro. Furthermore, the potential molecular mechanisms of SXD regulating the FKBP51/AKT signaling pathway were also studied in our present study.
Material and methods
Extraction of six-ingredient-Xiao-qing-long decoctionThe 6 herbal medicines, including Ephedra sinica (HS190231001#), Pinellia ternate (HS190231002#), Schisandra chinensis (HS190231003#), Glycyrrhiza uralensis (HS190231004#), Zingiber officinale (HS190231005#) and Asarum sieboldii (HS190231006#), were decocted using water, and the filtrates was further dried using a freeze dryer to afford the freeze-dried powder of SXD.
Cell culture
The hASMC cell line was obtained from ScienCell Research laboratories (Carlsbad, CA, USA). Cells were cultured in Dulbecco’s modified Eagle’s medium (DMEM, GIBCO, Ltd., Carlsbad, CA, USA) containing 10% fetal bovine serum (FBS, GIBCO, Ltd., Carlsbad, CA, USA), 100 μg/mL penicillin, and 100 μg/mL streptomycin at 37°C in a humidified 5% CO2 atmosphere.
Cell viability assay
Cell viability was determined using cell counting kit-8 assay (CCK-8; SAB, USA) under 10% FBS conditioned medium (Zhang et al. Citation2020). TGF-β1 (Abcam, Cambridge, MA, USA) was initially dissolved in 10 mM, pH3.0 citric acid to make a stock solution (50 μg/mL) and then diluted to 10 ng/mL with serum-free culture medium. In brief, the number of hASMCs was calculated using a hemocytometer and then plated in 96-well plate at a density of 1 × 104 cells/mL for 24 h. Cells were pretreated with different concentrations of SXD (0.1, 0.2 and 0.5 mg/mL) for 6 h, followed by incubation with or without 10 ng/mL TGF-β1 for 24 h, and subsequently CCK-8 reagents were added for 1 h at 37°C. The cell proliferation was determined by measuring the absorbance at 450 nm using DNM-9602 microplate reader (Perlong, China).
Transwell migration assay
Cell migration was measured using transwell cell culture chambers (BD Biosciences, Franklin Lakes, NJ, USA). In accordance with previous experiments, 1 × 104 hASMC were added to upper chamber of the transwell plates (without FBS), and the lower chamber were filled with DMEM containing 10% FBS (Li et al. Citation2017). After 24 h, cells remaining on the upper surface of the membrane were wiped off using a cotton swab. The migrated cells on the lower surface of the filter were fixed in 4% paraformaldehyde in PBS for 10 min, and then stained with 1 mL 0.5% crystal violet. Finally, cells were counted under a 200× magnification microscope. All experiments were carried out three times.
RT-qPCR
Total RNA was isolated from hASMC using Trizol Reagent (Invitrogen, Carlsbad, CA, USA) according to the manufacturer’s protocol. The isolated RNA was reversed transcribed into cDNA by using RevertAid First-Strand cDNA Synthesis Kit (Fermentas, Hanover, USA). RT-qPCR was carried out using SYBR Green Mix (Thermo Fisher Scientific, Waltham, MA, USA) in the ABI 7300 Real-Time PCR System (ABI, Foster City, CA, USA). β-actin was amplified as the reference gene. The mRNA levels of each sample were evaluated by using ΔΔCT method. The primer sequences of FKBP51 and β-actin are listed in Table . Each experiment was repeated three times.
Table 1. Sequences of primers used for RT-qPCR.
Western blotting
Total protein was extracted from hASMC using RIPA lysis buffer. Protein concentrations were determined using the bicinchoninic acid (BCA) protein concentration detection kit (Thermo Fisher Scientific, Waltham, MA, USA). Equal amounts of protein from each sample were loaded on 10% sodium dodecylsulphate (SDS)–polyacrylamide gels. After electrophoresis, proteins were transferred onto a polyvinylidene fluoride (PVDF) membrane (Millipore, Billerica, MA, USA) and incubated with Tris-buffered saline Tween 20 buffer (TBST) containing 5% skim milk powder at 4°C for 1 h. Afterwards, the membranes were incubated with primary antibodies for 2 h at room temperature, and then incubated with horseradish peroxidase-conjugated secondary antibodies (1:1000; Beyotime, Shanghai, China) for 1 h. The primary antibodies included antibodies against FKBP51 (1:1000; Abcam, Cambridge, MA, USA), AKT (1:1000; Abcam, Cambridge, MA, USA), p-AKT (1:500; Abcam, Cambridge, MA, USA) and β-actin (1:1000; Abcam, Cambridge, MA, USA). Immunoreactive bands were detected using an ECL detection kit (Millipore, Bedford, USA) and visualized in Tanon-5200 system (Tanon, Shanghai, China). Each experiment is repeated three times.
Cell transfection
To over-express FKBP51 in hASMC, the oeFKBP51 was constructed by inserting FKBP51 gene fragment into vector plasmid pcDNA3.1 (Addgene, USA). Moreover, three siRNAs were designed to knock down FKBP51 in hASMC. Lipo2000 (Invitrogen, Carlsbad, CA, USA) was used to transfect oeFKBP51 or siRNAs into cells according to the manufacturer’s protocol. β-actin was amplified as the reference gene. The sequences of siFKBP51-1 (5′-CCUAAUGCUGAGCUUAUAUTT-3′), siFKBP51-2 (5′-GCUGCUCAUGAACGAGUUUTT-3′), siFKBP51-3(5′-GGAUAUACGCCAACAUGUUTT-3) and siNC (5′-CAGUACUUUUGUGUAGUACAA-3′) used to synthesis siRNAs, and all experiments were repeated three times.
Statistical analysis
At least 3 separate experiments of each method were made, and data were shown as mean ± SD and then analyzed in prism ver. 8.0.2 (GraphPad, USA). The differences among the experimental groups were evaluated using one-way analysis of variance (ANOVA) and posterior multiple comparisons were determined by Tukey test. P-value < 0.05 has significance in statistics.
Results
SXD inhibited TGF-β1-induced abnormal proliferation and migration of hASMC
The hASMC cells were treated with TGF-β1 (10 ng/mL) for 24 h. The result of CCK-8 assay showed that TGF-β1 promoted proliferation of hASMC (p < 0.0001) compared with the control group (Figure A). However, as shown in Figure A, treatment with SXD (0.1, 0.2, and 0.5 mg/mL) significantly reduced the cell viability of TGF-β1-induced hASMC (p < 0.05, vs. cells treatment with TGF-β1 alone) in a dose-dependent manner. The transwell assays examined the effect of TGF-β1 on the migration ability of hASMC, and the results showed that TGF-β1 (10 ng/mL) can significantly increase cell migration of hASMC (Figure B&C) (p < 0.001). When these stimulated cells were exposed to different concentrations of SXD (0.1, 0.2, and 0.5 mg/mL), the migration ability was significantly inhibited (p < 0.001, vs. cells treatment with TGF-β1 alone) (Figure B & C) in a dose-dependent manner. Interestingly, SXD (0.5 mg/mL) had no obvious effect on cell viability and migration ability of hASMC in absence of TGF-B1 treatment.
Figure 1. TGF-β1 promoted the proliferation and migration of hASMC. Cells were treated with 10 ng/mL TGF-β1 for 24 h. (A) The cell viability was measured by CCK8 assay. (B and C) The cell migration was assessed by transwell assay. Data are expressed as mean ± SD (n = 3). ***p < 0.001 and ****p < 0.0001 vs vehicle.
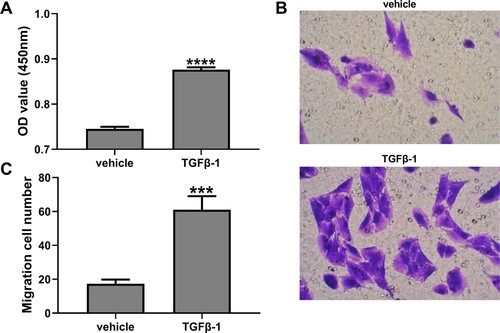
Figure 2. SXD inhibited TGF-β1-induced proliferation and migration of hASMC. Cells were pretreated with different concentrations of SXD (0.1, 0.2 and 0.5 mg/mL) for 6 h, and then treated with or without 10 ng/mL TGF-β1 for 24 h. (A) Cell viability was detected by CCK8 assay. (B and C) Cell migration was assessed by transwell assay. Data are expressed as mean ± SD (n = 3). ****p < 0.0001 vs. control; #p < 0.05, ##p < 0.01, ###p < 0.001 vs. TGF-β1; SXD, Six-ingredient-Xiao-qing-long decoction.
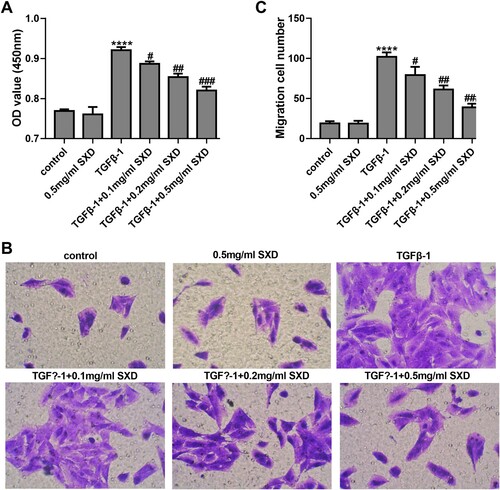
TGF-β1 repressed FKBP51 expression in hASMC
The present study initially measured the expression levels of FKBP51 in TGF-β1-treated hASMC. As presented in Figure A, hASMC were treated with TGF-β1 (10 ng/mL) at different time points (6–24 h). Real-time RT-PCR showed that TGF-β1 markedly decreased the mRNA expression level of FKBP51 after 6 h treatment (p < 0.01), reaching a minimum at 24 h. Similar to the FKBP51 mRNA, the protein expression of FKBP51 was decreased by TGF-β1 (Figure B) in a time-dependent manner.
SXD increased FKBP51 and attenuated p-AKT in TGF-β1-induced hASMC
In order to identify whether SXD affected FKBP51 and the phosphorylation of AKT or not, western blot assays were carried out to quantify the expression of FKBP51, AKT and p-AKT in TGF-β1-induced hASMC. Our results (Figure ) showed that TGF-β1 (10 ng/mL) stimulation distinctly suppressed FKBP51 expression and increased the phosphorylation of AKT in hASMC, compared to the control cells. However, pretreatment with SXD could increase FKBP51 and decrease p-AKT in TGF-β1-induced hASMC, compared to the cells treated TGF-β1 alone.
Figure 4. SXD attenuated TGF-β1-mediated FKBP51 expression and AKT phosphorylation. Cells were pretreated with different concentrations of SXD (0.1, 0.2 and 0.5 mg/mL) for 6 h, and then treated with 10 ng/mL TGF-β1 for 24 h. The protein levels of FKBP51, AKT, p-AKT were measured by western blotting. Data are expressed as mean ± SD (n = 3). SXD, Six-ingredient-Xiao-qing-long decoction.
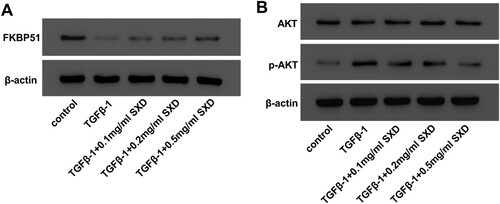
SXD inhibited the migration of TFG-β1-induced hASMC by regulating FKBP51/AKT signaling
AKT is a core component of PI3 K/AKT signaling pathway that plays a key role in proliferation and migration of hASMC, and its phosphorylation has been reported to be regulated by FKBP51 (Wang Citation2011; Zhou et al. Citation2018). To further explore the underlying mechanisms of SXD, the effects of SXD on activation of FKBP51/AKT signaling in TGF-β1-stimulated hASMC were examined. Cells were treated using TGF-β1 (10 ng/mL) with oeFKBP51 or SXD (0.5 mg/mL) for 24 h. The expression efficiency of oeFKBP51 was examined by western blot and RT-qPCR analysis (Figure S1). Transwell assay indicated that oeFKBP51 or SXD (0.5 mg/mL) could decrease the migration of TGF-β1-induced hASMC (Figure A&B). Furthermore, we analyzed the effect of oeFKBPk51 and SXD on expression of FKBP51, AKT and p-AKT proteins by western blotting assays. As shown in Figure C, these results showed that oeFKBP51 could elevate the expression of FKBP51 but decreased the phosphorylation of AKT, compared to hASMC treated with TGF-β1 alone. In addition, western blotting results also indicated that SXD up-regulates FKBP51 and therefore attenuates AKT activation in hASMC. Therefore, these results indicated that SXD inhibited cell migration of TGF-β1-induced hASMC via regulating the expression level of FKBP51.
Figure 5. SXD inhibited TGF-β1-induced cell migration by regulating FKBP51/AKT signaling. Cells were transfected with oeFKBP51 or vector. After 24 h of transfection, cells were treated using 10 ng/mL TGF-β1 with or without 0.5 mg/mL SXD for 24 h. (A and B) Cell migration was assessed by transwell assay. (C) The protein levels of FKBP51, AKT and p-AKT were measured by western blotting. Data are expressed as mean ± SD (n = 3). **p < 0.01 vs control; #p < 0.05, ##p < 0.01 vs TGF-β1; SXD, Six-ingredient-Xiao-qing-long decoction.
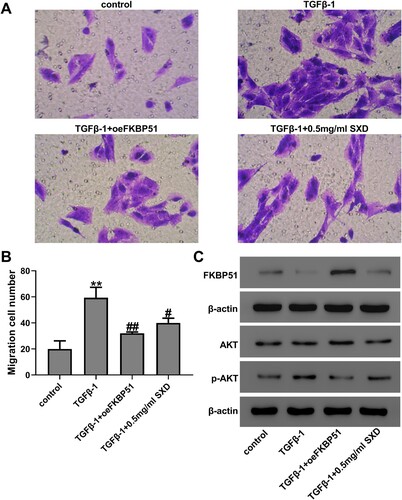
SXD inhibited FKBP51 knockdown-induced migration of hASMC
hASMC was transfected with FKBP51 siRNA, and the efficiency of transfection was evaluated by western blot and RT-qPCR analysis. RT-qPCR analysis demonstrated that all three FKBP51 siRNAs markedly inhibited the mRNA expression levels of FKBP51, among which siFKBP51-2 had the most obvious effect (Figure S1). Consistent with the results of RT-qPCR, western blot data revealed that transfection of siFKBP51 decreased the expression levels of FKBP51 protein (Figure S1). The effect of SXD on FKBP51 knockdown-induced migration of hASMC was examined using a transwell migration assay. As presented in Figure , cells were transfected with siFKBP51 or siNC and then cultured with or without SXD (0.5 mg/mL) for 24 h. The result indicated that SXD could significantly inhibited FKBP51 knockdown-induced cell migration and up-regulation of p-AKT, but increased the protein levels of FKBP51 compared to the siFKBP51 group (p < 0.01).
Figure 6. SXD inhibited siFKBP51-induced hASMC migration. Cells were transfected with siFKBP51 or siNC and then cultured with or without 0.5 mg/mL SXD for 24 h. (A and B) Cell migration was assessed by Transwell assay. (C) The protein levels of FKBP51, AKT and p-AKT were measured by western blotting. Data are expressed as mean ± SD (n = 3). ***p < 0.001 vs siNC; ##p < 0.01 vs siFKBP51; SXD, Six-ingredient-Xiao-qing-long decoction.
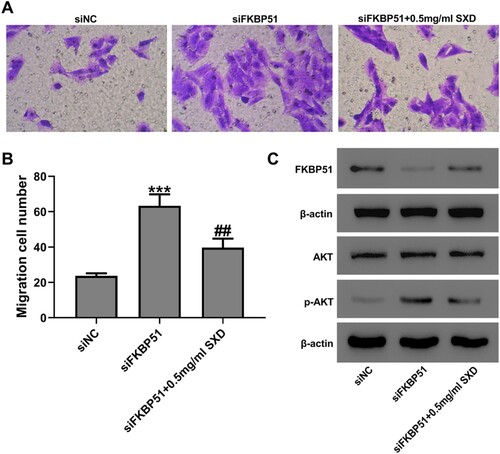
Discussion
Asthma is a multifactorial disorder that features chronic inflammatory, airway remodeling and bronchial hyperresponsiveness. Among them, airway remodeling has been well documented to be one of the important factors of asthma onset (Boulet Citation2018). Liu et al reported that protocatechuic acid pre-treatment substantially suppressed asthma airway remodeling by suppressing TGF-β1-stimulated hASMC proliferation, migration and extracellular matrix protein deposition via inactivation of Smad2/3 signaling pathway (Liu et al. Citation2019). Meanwhile, triptolide suppressed TGF-β1-induced hASMC proliferation and migration through decreasing NF-κB p65 phosphorylation (Chen et al. Citation2014). Therefore, how to effectively inhibit the abnormal proliferation and migration of hASMC is considered as the main therapeutic approach to suppress airway remodeling development. In previous studies, SXD has a long history as a common formula for childhood asthma in TCM clinical practice, and has achieved ideal results with minor side effects (Jiang and Li Citation2015). However, there are few reports about the underlying molecular mechanisms. In this study, we showed for the first time that SXD inhibited the proliferation and migration of hASMC exposed to TGF-β1.
FKBP51, as a glucocorticoid receptor co-chaperone, plays an important role in the development of steroid-resistant asthma. Based on the study of Chun et al., a time-series microarray analysis was performed on a murine model of asthma treated with a single dose of dexamethasone, and the results showed that FKBP51 had a stronger response to corticosteroids during asthma treatment and could be used as a reliable biomarker for corticosteroids treatment (Chun et al. Citation2011). Moreover, over-expression of FKBP51 leads to corticosteroids resistance syndrome and plays a negative feedback role in asthma treatment (Zhang et al. Citation2008). Nevertheless, a potential association between FKBP51 expression and airway remodeling has not been reported. In the current study, we observed that TGF-β1 significantly inhibited the expression of FKBP51 in a time-dependent way, but SXD could reverse the decline in a concentration-dependent manner. In addition, transwell assay indicated that oeFKBP51 or SXD could decrease hASMC migration that induced by TGF-β1. And after hASMC transfected with FKBP51 siRNA, SXD could significantly inhibit FKBP51 knockdown-induced migration and reverse the down-regulated of FKBP51 expression caused by siFKBP51. Therefore, these data suggested that FKBP51 may serve a critical role in asthmatic airway remodeling, and SXD may exert a therapeutic effect via upregulating the content of FKBP51.
AKT also known as protein kinase B, is activated by various stimuli, growth factors and hormones (Risso et al. Citation2015; Song et al. Citation2019). It has been reported that Akt plays a crucial role in cell survival and development (Li et al. Citation2020), and the activation of AKT is involved in regulating the proliferation and migration of hASMC (Deng et al. Citation2017; Lin et al. Citation2019). A previous study demonstrated that AKT activation was significantly increased in hASMC induced by TGF-β1, while inhibition of the AKT signaling pathway significantly decreases hASMC migration (Lv et al. Citation2019). FKBP51 has been shown to participate in the AKT regulation process and one finding indicate FKBP51 inhibits cell proliferation by decreasing AKT signaling (Dong et al. Citation2017). In this study, we examined FKBP51 and AKT expression in hASMC to test the hypothesis that SXD could increase the expression of FKBP51 to down-regulate the phosphorylation levels of AKT and thus inhibit TGF-β1-induced proliferation and migration. The present study revealed that the increased protein level of p-AKT stimulated by presence of TGF-β1 was markedly suppressed oeFKBP51 or after SXD treatment. In addition, knockdown of FKBP51 in hASMC increased the protein expression of p-AKT, but its activation was reversed after SXD treatment. From these findings, we concluded that SXD acts as a specific FKBP51/AKT channel opener to restrain TGF-β1-induced cell proliferation and migration.
Conclusions
In conclusion, the present results have revealed that SXD inhibits asthma airway remodeling by inhibiting proliferation and migration in TGF-β1-induced hASMC via regulating FKBP51/AKT signaling in vitro, and these findings could be used for further studies in vivo. Therefore, our results suggested that SXD would be beneficial for the airway remodeling in childhood asthma.
Supplemental Material
Download Zip (746.6 KB)Data availability statement
Due to the nature of this research, participants of this study did not agree for their data to be shared publicly, so supporting data is not available.
Disclosure statement
The authors declare that there is no conflict of interests concerning this paper.
Additional information
Funding
References
- Allen DB. 2020 Dec. Inhaled corticosteroids and endocrine effects in childhood. Endocrinol Metab Clin North Am. 49(4):651–665.
- Baarsma HA, Menzen MH, Halayko AJ, Meurs H, Kerstjens HA, Gosens R. 2011. β-Catenin signaling is required for TGF-β1-induced extracellular matrix production by airway smooth muscle cells. Am J Physiol Lung Cell Mol Physiol. 301(6):L956–65.
- Bentley JK, Hershenson MB. 2008. Airway smooth muscle growth in asthma: proliferation, hypertrophy, and migration. Proc Am Thorac Soc. 5(1):89–96.
- Boulet LP. 2018. Airway remodeling in asthma: update on mechanisms and therapeutic approaches. Curr Opin Pulm Med. 24(1):56–62.
- Chang X, Zhang T, Meng QY, Zhang QX. 2018. Research progress of xiaoqinglong decoction in treatment of bronchial asthma. J Hubei Univ Chin Med. 20(04):121–125. Chinese.
- Chen M, Shi JT, Lv ZQ, Huang LJ, Lin XL, Zhang W, Liang RY, Li YQ, Jiang SP. 2014. Triptolide inhibits TGF-β1 induced proliferation and migration of rat airway smooth muscle cells by suppressing NF-κB but not ERK1/2. Immunology. 144(3):486–94.
- Chun E, Lee HS, Bang BR, Kim TW, Lee SH, Kim JH, Cho SH, Min KU, Kim YY, Park HW. 2011. Dexamethasone-induced FKBP51 expression in peripheral blood mononuclear cells could play a role in predicting the response of asthmatics to treatment with corticosteroids. J Clin Immunol. 31(1):122–127.
- Deng Y, Zhang Y, Wu H, Shi Z, Sun X. 2017. Knockdown of FSTL1 inhibits PDGF-BB-induced human airway smooth muscle cell proliferation and migration. Mol Med Rep. 15(6):3859–3864.
- Dong J, Jiao Y, Mu W, Lu B, Wei M, Sun L, Hu S, Cui B, Liu X, Chen Z, Zhao Y. 2017. FKBP51 decreases cell proliferation and increases progestin sensitivity of human endometrial adenocarcinomas by inhibiting Akt. Oncotarget. 8(46):80405–80415.
- Fuchs O, Bahmer T, Rabe KF, von Mutius E. 2017. Asthma transition from childhood into adulthood. Lancet Respir Med. 5(3):224–234.
- Gao Y, Wang B, Luo H, Zhang Q, Xu M. 2018. miR-217 represses TGF-β1-induced airway smooth muscle cell proliferation and migration through targeting ZEB1. Biomed Pharmacother. 108:27–35.
- Golebski K, Kabesch M, Melén E, Potočnik U, van Drunen CM, Reinartz S. 2020. Maitland-van der Zee AH, Vijverberg SJH; PERMEABLE consortium. 2020. Childhood asthma in the new omics era: challenges and perspectives. Curr Opin Allergy Clin Immunol. 20(2):155–161.
- Hirota N, Martin JG. 2013. Mechanisms of airway remodeling. Chest. 144(3):1026–1032.
- Ish P, Malhotra N, Gupta N. 2020. GINA 2020: what’s new and why? J Asthma. 2:1–5.
- Jiang ZY, Li X. 2015. Introduction of “six-ingredient xiaoqinglong decoction” in Xu’s pediatrics. Shanghai J. TCM. 49(02):63–64. Chinese.
- Li J, Guo Y, Duan L, Hu X, Zhang X, Hu J, Huang L, He R, Hu Z, Luo W, et al. 2017. AKR1B10 promotes breast cancer cell migration and invasion via activation of ERK signaling. Oncotarget. 8(20):33694–33703.
- Li LH, Lu B, Wu HK, Zhang H, Yao FF. 2015. Apigenin inhibits TGF-β1-induced proliferation and migration of airway smooth muscle cells. Int J Clin Exp Pathol. 8(10):12557–12563.
- Li RL, Zhang Q, Liu J, Sun JY, He LY, Duan HXY, Peng W, Wu CJ. 2020. Hydroxy-α-sanshool possesses protective potentials on H2O2-stimulated PC12 cells by suppression of oxidative stress-induced apoptosis through regulation of PI3K/Akt signal pathway. Oxid. Med. Cell. Longev. 2020:3481758.
- Liao Y, Hung MC. 2010. Physiological regulation of Akt activity and stability. Am J Transl Res. 2(1):19–42.
- Lin L, Li Q, Hao W, Zhang Y, Zhao L, Han W. 2019. Upregulation of LncRNA Malat1 induced proliferation and migration of airway smooth muscle cells via miR-150-eIF4E/Akt Signaling. Front Physiol. 10:1337.
- Liu Y, Miao Y, Gao X, Wang YY, Wang H, Zheng YW, Zhao ZY. 2018. MicroRNA-200a affects the proliferation of airway smooth muscle cells and airway remodeling by targeting FOXC1 via the PI3K/AKT signaling pathway in ovalbumin-induced asthmatic mice. Cell Physiol Biochem. 50(6):2365–2389.
- Liu YD, Sun X, Zhang Y, Wu HJ, Wang H, Yang R. 2019. Protocatechuic acid inhibits TGF-β1-induced proliferation and migration of human airway smooth muscle cells. J Pharmacol Sci. 139(1):9–14.
- Luo K, Li Y, Yin Y, Li L, Wu C, Chen Y, Nowsheen S, Hu Q, Zhang L, Lou Z, Yuan J. 2017. USP49 negatively regulates tumorigenesis and chemoresistance through FKBP51-AKT signaling. EMBO J. 36(10):1434–1446.
- Lv X, Li Y, Gong Q, Jiang Z. 2019. TGF-β1 induces airway smooth muscle cell proliferation and remodeling in asthmatic mice by up-regulating miR-181a and suppressing PTEN. Int J Clin Exp Pathol. 12(1):173–181.
- Malmström K, Pelkonen AS, Mäkelä MJ. 2013. Remodeling, inflammation and airway responsiveness in early childhood asthma. Curr Opin Allergy Clin Immunol. 13(2):203–210.
- Noutsios GT, Floros J. 2014. Childhood asthma: causes, risks, and protective factors; a role of innate immunity. Swiss Med Wkly. 144:w14036.
- Ojiaku CA, Cao G, Zhu W, Yoo EJ, Shumyatcher M, Himes BE, An SS, PanettieriJrRA. 2018. TGF-β1 evokes human airway smooth muscle cell shortening and hyperresponsiveness via Smad3. Am J Respir Cell Mol Biol. 58(5):575–584.
- Pan Y, Liu L, Li S, Wang K, Ke R, Shi W, Wang J, Yan X, Zhang Q, Wang Q, et al. 2018. Activation of AMPK inhibits TGF-β1-induced airway smooth muscle cells proliferation and its potential mechanisms. Sci Rep. 8(1):3624.
- Qi M, Zhou J, Zhang X, Zhong X, Zhang Y, Zhang X, Deng X, Li H, Wang Q. 2017. Effect of xiaoqinglong decoction on mucus hypersecretion in the airways and cilia function in a murine model of asthma. J Trad Chin Med Sci. 4(3):290–296.
- Risso G, Blaustein M, Pozzi B, Mammi P, Srebrow A. 2015. Akt/PKB: one kinase, many modifications. Biochem J. 468(2):203–214.
- Song M, Bode AM, Dong Z, Lee MH. 2019. AKT as a therapeutic target for cancer. Cancer Res. 79(6):1019–1031.
- Song G, Zhang Y, Zhao K, Sun M, Cheng P, Wang J. 2018. Regulatory effect of xiaoqinglong decoction on thymic stromal lymphopoietin (TSLP) inflammation promoter in mice with cold asthma. Iran J Allergy Asthma Immunol. 17(1):39–46.
- Stechschulte LA, Sanchez ER. 2011. FKBP51-a selective modulator of glucocorticoid and androgen sensitivity. Curr Opin Pharmacol. 11(4):332–337.
- Tajiri T, Matsumoto H, Niimi A, Ito I, Oguma T, Nakaji H, Inoue H, Iwata T, Nagasaki T, Kanemitsu Y, et al. 2013. Association of eosinophilic inflammation with FKBP51 expression in sputum cells in asthma. PLoS One. 8(6):e65284.
- Wang L. 2011. FKBP51 regulation of AKT/protein kinase B phosphorylation. Curr Opin Pharmacol. 11(4):360–364.
- Wang W, Liu QB, Jing W. 2019. Astragalus membranaceus improves therapeutic efficacy of asthmatic children by regulating the balance of Treg/Th17 cells. Chin J Nat Med. 17(4):252–263.
- Wei M, Gao Y, Lu B, Jiao Y, Liu X, Cui B, Hu S, Sun L, Mao S, Dong J, et al. 2018. FKBP51 regulates decidualization through Ser473 dephosphorylation of AKT. Reproduction. 155(3):283–295.
- Woodruff PG, Boushey HA, Dolganov GM, Barker CS, Yang YH, Donnelly S, Ellwanger A, Sidhu SS, Dao-Pick TP, Pantoja C, et al. 2007. Genome-wide profiling identifies epithelial cell genes associated with asthma and with treatment response to corticosteroids. Proc Natl Acad Sci USA. 104(40):15858–15863.
- Xie S, Sukkar MB, Issa R, Khorasani NM, Chung KF. 2007. Mechanisms of induction of airway smooth muscle hyperplasia by transforming growth factor-beta. Am J Physiol Lung Cell Mol Physiol. 293(1):L245–L253.
- Zhang X, Clark AF, Yorio T. 2008. FK506-binding protein 51 regulates nuclear transport of the glucocorticoid receptor beta and glucocorticoid responsiveness. Invest Ophthalmol Vis Sci. 49(3):1037–47.
- Zhang Q, Liu J, Li R, Zhao R, Zhang M, Wei S, Ran D, Jin W, Wu CJ. 2020. A network pharmacology approach to investigate the anticancer mechanism and potential active ingredients of Rheum palmatum L. against lung cancer via induction of apoptosis. Front Pharmacol. 11:528308.
- Zhang X, Wang L, Shi Q. 2003. Effect of fluticasone inhalation combined with xiaoqinglong decoction on pulmonary function and serum interleukin-16 level in asthma patients. Chin J Integr Trad West. 23(6):426–429. Chinese.
- Zhou H, Wu Q, Wei L, Peng S. 2018. Paeoniflorin inhibits PDGF-BB-induced human airway smooth muscle cell growth and migration. Mol Med Rep. 17(2):2660–2664.