Abstract
Studies have reported frequent downregulation of microRNA-125-5p (miR-125) in osteosarcoma tissues. However, the molecular role and mechanism of action of miR-125-5p in osteosarcoma via regulation of Kruppel-like factor 13 (KLF13) is yet to be explored. The results of the present study miR-125-5p are significantly (P < .05) downregulated in human osteosarcoma tissues and cell lines. Overexpression of miR-125-5p resulted in a significant (P < .5) decrease of the osteosarcoma cells via induction of autophagy. Additionally, overexpression of miR-125-5p also decreased the migration and invasive potential of the osteosarcoma cells. Bioinformatic analysis and dual-luciferase assays revealed that miR-125-5p targets KLF13. Post-transcriptional suppression of KLF13 by miR-125-5p results in the inhibition of osteosarcoma cells. Nonetheless, overexpression of KLF13 could abolish the tumor-suppressive effects of miR-125-5p. Taken together, these results suggest the tumor-suppressive role of miR-125-5p in and point toward its therapeutic potential in the treatment of osteosarcoma.
Introduction
Osteosarcoma (or osteogenic sarcoma) is the most common type of neoplastic malignancy of bone tissues emerging from bone-forming mesenchymal cells. It exhibits higher prevalence among children and young adults (Picci Citation2007). Although the teenage population group is most frequently affected with osteosarcoma, this disease can develop in people of any age group. According to a report, the incidence rates of osteosarcoma in most of the countries were higher in males than females (Mirabello et al. Citation2009). In the USA, the black people have been reported to have a slightly higher osteosarcoma incidence rate than the whites (Sadykova et al. Citation2020 may 11). Regarding the mortality of osteosarcoma sarcoma, it has been reported that around 40% of people diagnosed with this disorder die within five years after disease diagnosis (Lindsey et al. Citation2017). The treatment strategies currently used against osteosarcoma include surgery and chemotherapy. However, radiotherapy might be useful in certain cases. There is growing research support for the employment of targeted molecular therapy against human cancers which is aimed at addressing the molecular irregularities associated with the growth and development of cancer (Lee et al. Citation2018). The aberrant expression patterns of micro-RNAs (miRs) are among such well-recognized molecular abnormalities and stated as the leading cause of tumorogenesis (Sun et al. Citation2018). The miRs are a group of 19–25 nucleotides long regulatory RNAs involved in a vast array of biological processes including carcinogenesis (Rupaimoole et al. Citation2016). The RNA class is believed to affect the crucial hallmarks of cancer such as cell division, apoptosis, angiogenesis and metastasis (Tan et al. Citation2018). MicroRNA-125-5p (miR-125-5p) functions as a tumor suppressor in human cancers, such as colorectal cancer and breast cancer, to inhibit the growth and metastatic behavior of cancer cells (Guo et al. Citation2009; Yang et al. Citation2019 Dec 20). Moreover, the low transcript levels of miR-125-5p are associated with the poor prognosis of gastric cancer (Wang et al. Citation2012). Previously, miR-125-5p was shown to target E2F2 transcription factor to regulate osteosarcoma cell growth and proliferation (Tao et al. Citation2017). Nevertheless, the role of miR-125-5p and its mechanism of action in osteosarcoma via zinc-finger transcription factor, Kruppel-like factor 13 (KLF13) are elusive. KLF13 is known for its tumor promotive role and has been shown to affect cell differentiation, proliferation, cell cycle and apoptosis (Wu et al. Citation2019). The present study showed that miR-125 has sufficient downregulation in osteosarcoma and directly targets KLF13 to regulate the growth and viability of osteosarcoma cells. Overexpression of miR-125 induced autophagy in osteosarcoma cells to inhibit in vitro proliferation, migration and invasion of osteosarcoma cells. The study elucidated the regulatory role of miR-125-5p/KLF13 and its possible therapeutic utility against the osteosarcoma but lacks in vivo characterization.
Materials and methods
Clinical tissues
A total of 55 pairs of osteosarcoma and normal adjacent human tissues were collected at the time of surgery from osteosarcoma patients with informed consents at ChangSha Central Hospital Affiliated to South China University, ChangSha, Hunan, China from June 2018 to June 2019. Tissue specimens were transported in liquid nitrogen and preserved in a deep freezer until use. The experimental study on human tissues was approved by the research ethics committee of ChangSha Central Hospital Affiliated to South China University, ChangSha, Hunan, China, under approval 65657/CCHA/2019.
Cell lines, culture conditions and transfection
The Cell Bank of the Chinese Academy of Sciences (Shanghai, China) provided all three osteosarcoma cell lines (MG-63, Saos2 and HOS) and the normal osteoblast cell line (hFOB1.19). The RPMI-1640 medium (HyClone, USA) carrying 10% FBS was used for culturing of the cell lines at 37°C with 5% CO2. The cells were transfected with the help of Lipofectamine 2000 reagent (Invitrogen, USA). Cells were transfected with miR-125 mimics to induce miR-125-5p overexpression and the miR-NC transfected cancer cells were treated as negative control. The experimental knock-down of KLF13 was performed through the transfection of si-KLF13 RNAi construct, while si-NC served as control. pcDNA3.1 overexpression vector was used to clone KLF13 for its overexpression, while the vector alone was used as negative control.
Quantitative real-time PCR (qRT-PCR) analysis
Total RNA was isolated by Trizol (Invitrogen, USA) from the cell lines and tissue samples. 1 µg of RNA was used for the synthesis of complementary DNA (cDNA) with the help of GoScript Reverse Transcriptase (Promega, USA). The qRT-PCR reactions were set to estimate the gene expression levels performed in triplicates in Roche Real-Time PCR System. The relative expression was quantified with the help of 2‐ddCt method. U6 and GADPH were used as normalization controls for miR-125-5p and KLF13 expression analysis, respectively.
3-(4,5-dimethylthiazol-2-yl)-2,5-diphenyl Tetrazolium bromide (MTT) cell viability assay
After 24 h transfection, the Saos-2 cells were seeded into 96-well plates at an inoculation cell count of 2.5 × 104 cells per well. The cells were either grown for 0, 12, 24, 48 and 96 h at 37°C or immediately mixed with 15 µL MTT reagent (Sigma-Aldrich) followed by re-incubation at 37°C for 2 h. After centrifugation, the cells were resuspended in dimethyl sulfoxide (DMSO). Finally, the samples were read for OD570 measurements using a spectrophotometer.
Colony formation assay
For colony formation assay, 1 mL culture medium, containing 5000 Saos-2 transfected cells, was added to each well of 6-well plate and the cells were allowed to grow for 14 days at 37°C with 5% CO2. The medium was removed from the wells and the colonies were visualized and counted after ethanol fixation and crystal-violet staining.
Acridine orange staining (AO) staining
To analyse the nuclear viability of Saos2 transfected cells, acridine orange staining assay was performed. Briefly, the transfected cancer cells were seeded into 12-well plate and allowed to grow till cell density reached 106 cells/well. Cells were harvested through centrifugation, phosphate-buffered saline (PBS) was washed and fixed with methanol. The methanol fixed cells were stained using an AO stain. At the end, fluorescence microscope was used for the examination of cells.
Transmission electron microscopy (TEM) analysis
For TEM, the transfected cells were harvested after 24 h culturing at 37°C. Glutaraldehyde (2%) was used for fixing the cell pellets at 4°C for 12 h. This was followed by washing with 0.1 M PBS (Sigma-Aldrich, Germany). Post-fixing of cell pellets was carried out using 1% OsO4 at room temperature for 2 h and PBS washing was repeated. Applying a series of graded ethanol and absolute acetone, the dehydration of samples was performed at room temperature. After embedding, the ultrathin sections (less than 80 nm thick) were obtained with the help of Leica Ultracut UCT Ultra-microtome which was followed by their staining with Reynold’s lead citrate for 20 min. Finally, the TEM (Hitachi, Japan) was used for visualizing the samples.
Scratch-heal and transwell assays
Transfected Saos2 cancer cells were grown in 6-well plate until saturated cell growth was attained. The cell layer was then scratched with the help of a 200-µL pipette tip and photographed. After 24 h incubation at 37°C, the scratch area was again photographed under microscope. Three random fields were used for the determination of the relative percentage of cell migration.
For invasion analysis, a transwell assay was performed to assess the relative number of cells that traversed a porous polycarbonate membrane in response to higher serum concentration serving as chemo-attractant. Invasion was determined using BioCoat Matrigel invasion chamber (BD Bioscience). Briefly, 2.5 × 105 transfected Saos2 cancer cells were added in the upper chamber, while the lower chamber was supplied with culture media containing 10% fetal bovine serum (FBS). The chamber was incubated for 24 h at 37°C after which the cells, which had migrated through polycarbonate membrane, were fixed, stained, examined and manually counted under light microscope.
Western blotting
The transfected Saos2 cells were lysed with radio-immuno precipitation assay buffer (Sigma-Aldrich, Germany) for total protein extraction which were electrophoretically separated on 10% SDS-polyacrylamide gels and then transferred to polyvinylidene difluoride (PVDF) membranes. The membranes were exposed to specific protein antibodies (Cell Signaling Technology, Boston) in dark at 4°C overnight. Afterward, the treatment of secondary antibodies conjugated with a horseradish peroxidase (1:1000; Cell Signaling Technology) was given at room temperature for 2.5 h. Finally, FluroChem E-system (Protein Simple, Silicon Valley) was used for visualizing the specific protein bands. β actin was used for standardizing the protein loading.
Bioinformatics and luciferase interaction assay
Using TargetScan Human 7.2 (http://www.targetscan.org/vert72/) online database, prediction of specific regulatory target of miR-125-5p was made. The prediction was confirmed by the dual luciferase reporter assay. In brief, the KLF13 3′UTR nucleotide sequence with the predicted binding site of miR-125-5p, wild type or mutated were cloned into the pmirGLO reporter vector. Saos-2 cancer cells were then co-transfected with the luciferase reporter plasmid and miR-125-5p mimics or miR-NC, negative control. After 48 h, the relative luciferase activity was quantified using dual-luciferase reporter assay system (Promega, USA).
Statistical data validation
Statistical data were validated by analysing them with the SPSS software (version 17.0, IBM Corp., Armonk, NY, USA). The Student’s t-test (two-tailed) or one-way analysis of variance (ANOVA) was used for examining the statistical difference. Statistical significance was taken on the basis of two-sided P values and considered significant at P < .05. The final results are presented as the mean ± standard deviation (SD).
Results
miR-125-5p is downregulated in osteosarcoma and regulates cell proliferation.
To infer about the expression pattern of miR-125-5p in osteosarcoma, qRT-PCR was performed. The malignant bone tissues were shown to express significantly (P < .05) higher transcript levels of miR-125-5p relative to the normal bone tissues (Figure (A)). Consistent with its tissue expression pattern, significantly higher miR-125-5p expression was reported from the osteosarcoma cell lines (MG-63, Saos-2 and HOS) in comparison to its expression in normal bone cell line, Hfob1.19 (Figure (B)). On an average, the osteosarcoma cell lines showed 5-fold lower miR-125-5p expression than the normal cell lines. For characterizing the role of miR-125-5p in osteosarcoma, miR-125-5p was overexpressed in Saos-2 cell line which was achieved by transfecting the same with miR-125 mimics with miR-NC transfection as negative control (Figure (C)). MTT assay showed that Saos-2 cells overexpressing miR-125-5p proliferated at significantly (P < .05) lower rate than control cancer cells at varying growth intervals (Figure (D)). The effect of the miR-125-5p overexpression on osteosarcoma cell viability was also analyzed through colony-forming assay. The miR-125-5p overexpression was shown to reduce the colony formation of Saos-2 cancer cells by approximately 57% (Figure (E)). The results indicate the inhibitory effect of miR-125-5p on osteosarcoma cell growth.
Figure 1. The miR-125-5p is downregulated in osteosarcoma to promote sustained cell growth. (A) Expression of miR-125-5p in osteosarcoma and normal adjacent bone tissues (B) miR-125-5p expression in osteosarcoma cell lines (MG-63, Saos2 and HOS) and normal osteoblast cells, hFOB1.19 (C) overexpression of miR-125-5p in Saos2 cells (D) MTT assay for proliferation analysis of transfected Saos2 cells and (E) relative colony formation of miR-NC or miR-125 mimics transfected Saos2 cells. The experiments were performed in triplicate and expressed as mean ± SD (*P < .05).
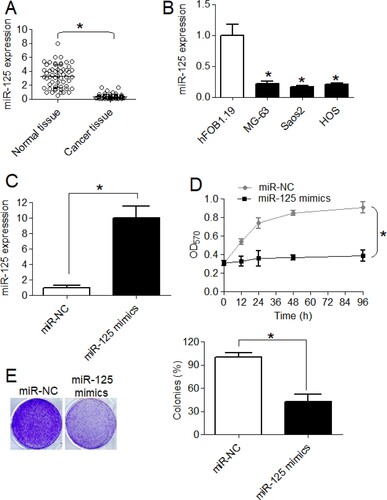
miR-125-5p overexpression induced autophagy in osteosarcoma cells in vitro
To specifically figure out the mechanism regulating the growth inhibitory role of miR-125-5p, the Saos-2 cancer cells, upregulating cells were treated with AO stain. Significantly higher proportion of EB-positive cells, indicative of cell apoptosis, were noticed under miR-125-5p overexpression (Figure (A)). The results were verified using the TEM analysis wherein the autophagosome structures were clearly observed in the ultra-structure details of Saos-2 cells overexpressing miR-125-5p (Figure (B)). More support was gained from the western blot analysis of marker proteins such as beclin 1, p62, LC3B I and LC3B II. Except the proliferation marker p62, all other proteins were repressed under miR-125-5p overexpression (Figure (C)). The results thus suggest that miR-125-5p induced autophagy to decline Saos-2 cell proliferation.
Figure 2. The miR-125-5p induced autophagy in osteosarcoma cells. (A) AO staining of miR-NC or miR-125 mimics transfected Saos2 cancer cells (D) TEM study of miR-NC or miR-125 mimics transfected Saos2 cells (C) western blotting of beclin 1, p62, LC3B I and LCEB II proteins from transfected Saos2 cancer cells. The experiments were performed in triplicate.
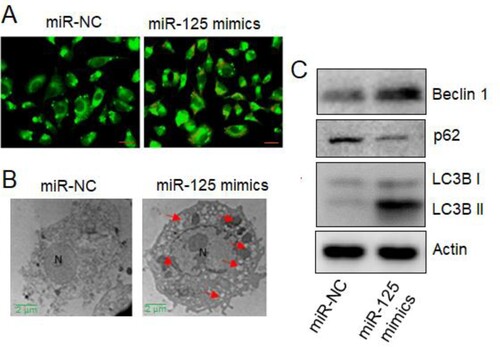
Upregulation of miR-125-5p declined osteosarcoma cell migration and invasion
Effect of miR-125-5p overexpression on migration and invasion of Saos-2 cancer cells was studied by performing scratch-heal and transwell assays, respectively. The migration of Sao-2 cancer cells upregulating miR-125-5p was shown to be significantly lower than that of control cancer cells (Figure (A)). Similarly, the invasion of Saos-2 cancer cells was also markedly lower under miR-125-5p overexpression (Figure (B)). The overexpression of miR-125-5p was shown to decrease the migration and invasion of Saos-2 by 83% and 85%, respectively. These results are indicative of the anti-metastatic role of miR-125-5p in osteosarcoma.
Figure 3. The miR-125-5p reduced migration and invasion of osteosarcoma cells. (A) Scratch-heal migration assay of miR-NC or miR-125 mimics transfected Saos2 cells (B) transwell assay for quantitative analysis of miR-NC or miR-125 mimics transfected Saos2 cell invasion. The experiments were performed in triplicate and expressed as mean ± SD (*P < .05).
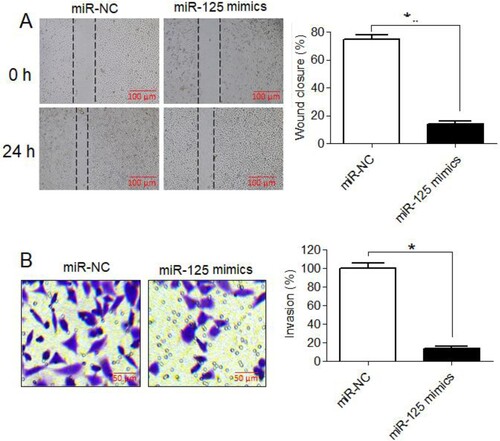
KLF13 acts as direct and functional regulatory target of miR-125-5p in osteosarcoma
Online bioinformatics was used for predicting the post-transcriptional target of miR-125-5p which was shown to be KLF13. The 3′-UTR of KLF13 was shown to possess a specific miR-125-5p binding site (Figure (A)). Dual-luciferase reporter assay showed that miR-125-5p interacted with 3′-UTR of KLF13 in a sequence-specific manner leading to a decrease in the luciferase activity of Saos-2 cells which was not noticed when miR-125-5p binding site was mutated in KLF13 3′-UTR (Figure (B)). The expression profile of KLF13 in tissues and cell lines also correlated negatively with miR-125-5p expression pattern in the same (Figure (C)). Again, the KLF13 protein levels were shown to decrease under miR-125-5p upregulation in Saos-2 cancer cells suggestive of post-transcriptional repression of former by miR-125-5p (Figure (D)). Interestingly, the KLF13 transcriptional knock-down decreased the proliferation of Saos-2 cells in the same manner as under miR-125-5p upregulation (Figure (E)). Furthermore, the upregulation of KLF13 mitigated the growth inhibitory effects of miR-125-5p overexpression on Saos-2 cell proliferation (Figure (G)). The results support miR-125-5p targets KLF13 in osteosarcoma to experience its cancer regulatory role.
Figure 4. The miR-125-5p targets KLF13 in osteosarcoma. (A) Bio-informatics-based prediction of miR-125-5p regulatory target (B) dual-luciferase reporter assay for the interaction analysis of miR-125-5p with KLF13 (C) transcript level of KLF13 in osteosarcoma and normal bone tissues (D) KLF13 transcript level in osteosarcoma cell lines (MG-63, Saos2 and HOS) and normal osteoblast cells, hFOB1.19 (E) KLF13 protein expression in miR-125-5p overexpressing Saos2 cancer cells and negative control cells (F) MTT assay for proliferation analysis of si-NC or si-KLF13 transfected Saos2 cells (G) analysis of proliferation of Saos2 cancer cells transfected with miR-125-5p mimics, miR-125-5p mimics plus pcDNA-KLF13 or miR-125-5p negative control (miR-NC). The experiments were performed in triplicate and expressed as mean ± SD (*P < .05).
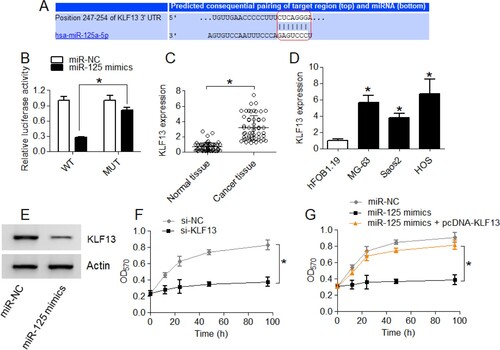
Discussion
Micro-RNAs (miRs) constitute an essential class of regulatory short-length RNAs which are shown to affect the expression of nearly 30% of protein-coding genes in eukaryotes (Zeng Citation2006). The miRs regulate a diverse array of biological and physiological parameters of human body (Vienberg et al. Citation2017). Regulatory errors, resulting in transcriptional enhancement or repression of miRs, have been reported to be associated with various disorders including the cancer (Cummins and Velculescu Citation2006). The dysregulated expressional pattern of miRs is critical for sustaining the increased rates of proliferation, evading the cell death pathways, promotion of invasion and metastasis of cancer cells (Le et al. Citation2010). Osteosarcoma, symbolizing the malignant form of primary bone tumor, has also been shown to exhibit dysregulation of a number of miRs and the same has been shown to alter the mechanics of specific cellular pathways regulating the malignant features of osteosarcoma cells like growth, migration and invasion (Kumar et al. Citation2016). In the present study, miR-125-5p was confirmed by expressional analysis to exhibit significant downregulation in osteosarcoma tissues and cell lines. The transcriptional drop off of miR-125-5p has been established to feature many other types of human cancers along with the osteosarcoma (Liang et al. Citation2017; Tao et al. Citation2017). The miR-125-5p expression thus might serve as a diagnostic marker for multiple human cancers besides its utility in disease prognosis. miR-125-5p is known as a tumor suppressor of many prominent human cancers and the negative osteosarcoma cell growth regulatory role, as indicated by the current study results convey the similar relationship (Zhang et al. Citation2018). The inhibition of cancer cell growth by miR-125-5p was previously shown to be modulated through cell cycle arrest or apoptosis induction (Chen et al. Citation2015; Song et al. Citation2015). Herein, the osteosarcoma cancer cells were inducted with autophagy under ectopic overexpression of miR-125-5p. The therapeutic targeting of miRs is highly advocated against the human cancer because a single miR can target multiple pathways in modulating a particular molecular signal. Consistently, miR-125-5p acts a repressor of cancer cell migration and invasion through multiple regulatory targets like GALNT7 in cervical cancer, LIN28B in ovarian cancer, HAX1 in liver cancer, etc. (Cao et al. Citation2020; Zeng et al. Citation2020; Zha and Li Citation2020 nov). The osteosarcoma cancer cells were shown to exhibit significantly lower migration and invasion under miR-125-5p overexpression as per the results of the present study. Interestingly, the KLF13 was identified as a functional regulatory target of miR-125-5p in osteosarcoma. The present study showed that osteosarcoma tissues and cells express higher KLF13 levels emerging from lower miR-125-5p transcript abundance. The higher KLF13 expression levels are also reported in oral cancer cells (Henson and Gollin Citation2010). Moreover, the functional targeting of KLF13 by miR-125a is also involved in maintaining the elevated chemokine RANTES levels in systematic lupus erythematosus (Zhao et al. Citation2010). The present study thus deduced the regulatory role of miR-125-5p/KLF13 axis in osteosarcoma and signifies its prognostic and therapeutic value. The main limitation of the present study is that only one cell line was used for the transfection experiments. Therefore, in vitro studies with more cell lines and in vivo studies are required to establish miR-125-5p as therapeutic target for the management of osteosarcoma.
Conclusion
The study results depict the downregulation of miR-125-5p in osteosarcoma. The miR-125-5p regulates growth and viability of osteosarcoma cells. The study further confirmed that overexpression of miR-125-5p has a potential to reduce the proliferation, migration and invasion of osteosarcoma cells through autophagy induction. The miR-125-5p was shown to exercise its role through KLF13 in osteosarcoma. The findings of the present study show the therapeutic potential of miR-125-5p in the management of osteosarcoma.
Acknowledgements
The authors acknowledge ChangSha Central Hospital Affiliated to South China University, ChangSha, Hunan, China, to conduct the presented study.
Availability of data
That data that support the findings of this study are available at the figshare repository (https://figshare.com/) at https://figshare.com/s/e53b31a2e09ab7c90434
Ethics approval and consent to participate
The study was approved by the research ethics committee of ChangSha Central Hospital Affiliated to South China University, ChangSha, Hunan, China, under approval 65657/CCHA/2019. Informed consent was obtained from the patients who participated in the study.
Disclosure statement
No potential conflict of interest was reported by the author(s).
References
- Cao Q, Wang N, Ren L, Tian J, Yang S, Cheng H. 2020. Patient-derived xenografts of different grade gliomas retain the heterogeneous histological and genetic features of human gliomas. Cancer Cell Int. 20:1–3.
- Chen D, Li Y, Su Z, Yu Z, Yu W, Li Y, Gui Y, Yang S, Lai Y. 2015. Identification of miR-125a-5p as a tumor suppressor of renal cell carcinoma, regulating cellular proliferation, migration and apoptosis. Mol Med Rep. 11(2):1278–1283.
- Cummins JM, Velculescu VE. 2006. Implications of micro-RNA profiling for cancer diagnosis. Oncogene. 25(46):6220–6227.
- Guo X, Wu Y, Hartley R. 2009. MicroRNA-125a represses cell growth by targeting HuR in breast cancer. RNA Biol. 6(5):575–583.
- Henson BJ, Gollin SM. 2010. Overexpression of KLF13 and FGFR3 in oral cancer cells. Cytogenet Genome Res. 128(4):192–198.
- Kumar RMR, Boro A, Fuchs B. 2016. Involvement and clinical aspects of microRNA in osteosarcoma. Int J Mol Sci. 17(6):877.
- Le XF, Merchant O, Bast RC, Calin GA. 2010. The roles of microRNAs in the cancer invasion-metastasis cascade. Cancer Microenviron. 3(1):137–147.
- Lee YT, Tan YJ, Oon CE. 2018. Molecular targeted therapy: treating cancer with specificity. Eur J Pharmacol. 834:188–196.
- Liang Y, Ye J, Jiao J, Zhang J, Lu Y, Zhang L, Wan D, Duan L, Wu Y, Zhang B. 2017. Downregulation of miR-125a-5p is associated with salivary adenoid cystic carcinoma progression via targeting p38/JNK/ERK signal pathway. Am J Transl Res. 9(3):1101.
- Lindsey BA, Markel JE, Kleinerman ES. 2017. Osteosarcoma overview. Rheumatology and Therapy. 4(1):25–43.
- Mirabello L, Troisi RJ, Savage SA. 2009. International osteosarcoma incidence patterns in children and adolescents, middle ages and elderly persons. Int J Cancer. 125(1):229–234.
- Picci P. 2007. Osteosarcoma (osteogenic sarcoma). Orphanet J Rare Dis. 2(1):6.
- Rupaimoole R, Calin GA, Lopez-Berestein G, Sood AK. 2016. miRNA deregulation in cancer cells and the tumor microenvironment. Cancer Discov. 6(3):235–246.
- Sadykova LR, Ntekim AI, Muyangwa-Semenova M, Rutland CS, Jeyapalan JN, Blatt N, Rizvanov AA. 2020 May 11. Epidemiology and risk factors of osteosarcoma. Cancer Investig. 38(5):259–269.
- Song C, Wu G, Xiang A, Zhang Q, Li W, Yang G, Shi XE, Sun S, Li X. 2015. Overexpression of miR-125a-5p inhibits proliferation in C2C12 myoblasts by targeting E2F3. Acta Biochim Biophys Sin. 47(4):244–249.
- Sun Z, Shi K, Yang S, Liu J, Zhou Q, Wang G, Song J, Li Z, Zhang Z, Yuan W. 2018. Effect of exosomal miRNA on cancer biology and clinical applications. Mol Cancer. 17(1):147.
- Tan W, Liu B, Qu S, Liang G, Luo W, Gong C. 2018 Mar 1. MicroRNAs and cancer: Key paradigms in molecular therapy. Oncol Lett. 15(3):2735–2742.
- Tao T, Shen Q, Luo J, Xu Y, Liang W. 2017. MicroRNA-125a regulates cell proliferation via directly targeting E2F2 in osteosarcoma. Cell Physiol Biochem. 43(2):768–774.
- Vienberg S, Geiger J, Madsen S, Dalgaard LT. 2017. MicroRNAs in metabolism. Acta Physiol. 219(2):346–361.
- Wang F, Sun GP, Zou YF, Hao JQ, Zhong F, Ren WJ. 2012. MicroRNAs as promising biomarkers for gastric cancer. Cancer Biomark. 11(6):259–267.
- Wu R, Yun Q, Zhang J, Bao J. 2019. Downregulation of KLF13 through DNMT1-mediated hypermethylation promotes glioma cell proliferation and invasion. Onco Targets Ther. Volume 12:1509–1520.
- Yang M, Tang X, Wang Z, Wu X, Tang D, Wang D. 2019 Dec 20. miR-125 inhibits colorectal cancer proliferation and invasion by targeting TAZ. Biosci Rep. 39(12).BSR20190193.
- Zeng Y. 2006. Principles of micro-RNA production and maturation. Oncogene. 25(46):6156–6162.
- Zeng J, Li YK, Quan FF, Zeng X, Chen CY, Zeng T, Zou J, Tong WJ. 2020. Propofol–induced miR–125a–5p inhibits the proliferation and metastasis of ovarian cancer by suppressing LIN28B. Mol Med Rep. 22(2):1507–1517.
- Zha Z, Li J. 2020 Nov. MicroRNA–125a–5p regulates liver cancer cell growth, migration and invasion and EMT by targeting HAX1. Int J Mol Med. 46(5):1849–1861.
- Zhang Y, Zhang D, Lv J, Wang S, Zhang Q. 2018. MiR-125a-5p suppresses bladder cancer progression through targeting FUT4. Biomed Pharmacother. 108:1039–1047.
- Zhao X, Tang Y, Qu B, Cui H, Wang S, Wang L, Luo X, Huang X, Li J, Chen S, Shen N. 2010. MicroRNA-125a contributes to elevated inflammatory chemokine RANTES levels via targeting KLF13 in systemic lupus erythematosus. Arthritis Rheum. 62(11):3425–3435.