Abstract
Intracellular signaling complexes of the innate immune system orchestrate the development of inflammation. Porphyromonas gingivalis is a commensal bacterium that induces periodontal pyroptosis. The transcription factor Dec1 can regulate the expression of inflammatory molecules. In this study, we investigated whether a deficiency of Dec1 activates/represses Caspase-1 and/or Gasdermin-D in periodontal pyroptosis, resulting in the release of the proinflammatory mediator interleukin-1β. Dec1 expression in human periodontal ligament fibroblasts (HPDLFs) treated with P. gingivalis lipopolysaccharide (LPS) was assessed and pyroptosis activation was determined by microscopy, qRT-PCR and Western blot analysis. Moreover, a siRNA targeting Dec1 was introduced into HPDLFs to determine the function of Dec1 in periodontal pyroptosis and a Dec1-deficient experimental mouse model of periodontitis was used. Treatment of HPDLFs with P. gingivalis LPS-stimulated levels of cleaved-Caspase-1 and cleaved-GSDMD and increased levels of phosphorylated NF-κB and interleukin-1β. In contrast, Dec1 knockdown maintained cellular homeostasis with low levels of pyroptosis. Treatment with P. gingivalis alleviated periodontal pyroptosis in PDLFs of wild-type mice and a Dec1 deficiency subsequently repressed the inflammatory effect of P. gingivalis. These results reveal for the first time the novel function of Dec1 in periodontal pyroptosis, suggesting that Dec1 is a crucial target to prevent periodontal inflammation.
1. Introduction
Periodontal homeostasis is regulated by multiple complex factors that are coordinated in the local environment. The initiation and progression of periodontal disease is due to an imbalance in host-microbe interactions (Cheng et al. Citation2015). Important etiological factors of periodontitis include multiple Gram-negative microaerophilic and anaerobic bacteria, such as Porphyromonas gingivalis, Tannerella forsythia, Treponima denticola and Aggregatibacter actinomycetemcomitans (A. actinomycetemcomitans), and the resulting host responses (Kinane Citation2001), which lead to an inflammation-mediated pyroptotic process.
Inflammatory Caspases contribute to a variety of biological functions (Martinon and Tschopp Citation2004; Man and Kanneganti Citation2016). Caspase-1, a cysteine proteinase, is activated by pathological bacteria, which further leads to the activation and secretion of interleukin (IL)-1β that mediates the activation of immune cells such as monocytes (Peleg et al. Citation2011). The mature form of cleaved pro-IL-1β plays a critical role in inflammation (Lamkanfi and Dixit Citation2014). Recent evidence demonstrates that activation of the Caspase pathway triggers pyroptosis and results in the extracellular release of inflammatory cytokines (Shi et al. Citation2017; Walle and Lamkanfi Citation2016).
A form of programmed cell death mediated by Caspase-1 and Gasdermin-D (GSDMD), termed pyroptosis, has been recently identified (Shi et al. Citation2015). The activation of Caspase-1 and pyroptosis are both involved in periodontitis (Cheng et al. Citation2018). Intracellular lipopolysaccharides (LPS) activate murine Caspase-11 and human Caspases-4/5, which can also lead to pyroptosis (Hagar et al. Citation2013; Kayagaki et al. Citation2013; Shi et al. Citation2014). The activation of Caspase-11 occurs through its action as a receptor for cytosolic bacterial LPS and the resultant binding (Shi et al. Citation2014). GSDMD is cleaved by Caspases-1/4/5/11 to induce the release of inflammatory cytokines such as IL-1β and to facilitate pyroptosis (Shi et al. Citation2015; Kayagaki et al. Citation2015; Aglietti et al. Citation2016; Chen et al. Citation2016; Ding et al. Citation2016; He et al. Citation2015).
LPS is known to trigger the transcription of several genes in many signaling pathways. The basic helix–loop–helix transcription factor Dec1 can directly influence the regulation of inflammation processes (Bhawal et al. Citation2012; Martínez-Llordella et al. Citation2013), through the repression of its target genes in a wide variety of cells. In aging Dec1-deficient (Dec1KO) mice, there is an increased production of IL-4 and IL-10 and the associated development of autoimmune diseases, without any effect on interferon-γ (Martínez-Llordella et al. Citation2013). A previous study reported that PI-3K signaling mediates the over-expression of Dec1 and the phosphorylation of Akt (Bhawal et al. Citation2011). The protective nature of Dec1 in adverse conditions is evident through the rapid induction of Dec1 target proteins in response to environmental stimuli. Dec1 is involved in maintaining the homeostasis of metabolism and energy in mammals (Iizuka and Horikawa Citation2008; Zvonic et al. Citation2006). Dec1 plays an important protective role in the defense against reactive oxygen species (ROS) induced oxidative damage in podocytes and muscle cells (Bek et al. Citation2003; Vercherat et al. Citation2009). The defective T cell-mediated recall responses that lead to autoimmune disease in mice with inadequate Dec1 also support the above statement (Martínez-Llordella et al. Citation2013). However, studies of Dec1KO mice suggest a rare or exceedingly low penetrance of spontaneous autoimmune diseases (Jiang et al. Citation2008; Miyazaki et al. Citation2010).
Pathological organisms are found in contact with the sulcular epithelium, with many penetrating deep into the connective tissue of the gingiva (Tribble and Lamont Citation2010). The periodontal ligament is sensitive to bacterial infections, and native cells present among periodontal ligament fibroblasts can recognize an infection process in the local environment (Kim et al. Citation2016). Cells with multipotency, namely periodontal ligament fibroblasts, have been shown to modulate the remodeling of osseous structures and formation of the ligament (Matsuda et al. Citation1998; Yang et al. Citation2010).
The biological insights into pyroptosis gained from the use of Dec1KO mice should be revisited. The results of this study demonstrate that the cleavage of Caspase-1 is highly activated in LPS-induced human periodontal ligament fibroblasts (HPDLFs). Interactions of LPS with Dec1 result in the activation of GSDMD. The role of Dec1 in the innate immune response can be better understood through our elucidation of the role of GSDMD activation by LPS. The ex vivo inhibition of Dec1 in mouse periodontal tissues and in HPDLFs in vitro reduced inflammatory expression, suggesting that a Dec1 deficiency may represent a novel strategy to prevent the inflammatory acceleration of periodontitis. Thus, these findings provide a new potential approach for the regulation of pyroptosis in PDLFs to prevent periodontal diseases.
2. Methods
2.1. Cell culture
HPDLFs were obtained from Lonza (Tokyo, Japan) and were cultured in SingleQuots Supplements (CC-4181, Insulin, hFGF-beta, GA-1000 and FBS). HPDLFs were sub-cultured and used for experiments when they became 70-80% confluent.
2.2. Animals
C57BL/6 (wild-type (WT), n = 12) and Dec1 knock-out (Dec1KO, n = 12) mice aged 12 weeks old were generated and used as models for periodontitis. The periodontitis in experimental animals was established as per the protocol used in our previous study (Zhang et al. Citation2018). The mice were divided into control and P. gingivalis treated groups and were sacrificed after 30 days, after which their upper jaws were dissected, fixed, and processed for immunohistochemistry as detailed below. Prior approval of the Animal Ethics Committee of Kanagawa Dental University was obtained for all animal experiments.
2.3. siRNA transfection
About 1.2 × 105 HPDLFs were seeded in 6-well plates in antibiotic-free medium for 16 h and were then transfected with Dec1 siRNA using RNAiMAX (Thermo Fisher Scientific, Waltham, MA, USA) for 48 h. Cell lysates were collected after transfection, and real-time polymerase chain reaction (RT–PCR) and Western blot were employed to determine the transfection efficiency.
2.4. RT–PCR
Total RNA extraction was done using QIAZOL (Qiagen KK, Tokyo, Japan) according to the manufacturer’s protocol. One µg RNA was transcribed to cDNA using a High-Capacity cDNA Archive Kit (Applied Biosystems, Foster City, CA, USA). TaqMan probes of target genes, including Dec1 (Assay ID: Hs01041212_m1), IL-1β (Assay ID: Hs01555410_m1) and ACTB (Assay ID Hs01060665_g1) were used for RT–PCR.
2.5. Western blot
RIPA lysis buffer (Santa Cruz Biotechnology, Santa Cruz, CA, USA) was used to solubilize HPDLFs. Twenty µg protein was applied in each lane of 10% or 15% polyacrylamide-SDS gels (Wako, Osaka, Japan). After blocking with 5% skim milk, the membranes were subjected to overnight incubation at 4°C with anti-Caspase-1 (1:500, Abcam, Tokyo, Japan), anti-cleaved-GSDMD (1:500, Cell Signaling Technology, Danvers, MA, USA), anti-p-NF-κB (1:800, Bioss, Woburn, MA, USA), anti-NF-κB (1:800, Cell Signaling Technology, Danvers, MA, USA), anti-IL-1β (1:1000, Cell Signaling Technology, Danvers, MA, USA) and anti-GAPDH (1:1000, #2118, Cell Signaling Technology, Danvers, MA, USA). Horseradish peroxidase-conjugated anti-mouse/rabbit IgG (1:2000; Cell Signaling Technology, Danvers, MA, USA) was used to visualize bound antibodies in an ECL Plus Western Blotting Detection System (GE Healthcare, Tokyo, Japan). The relative intensities of the bands were quantified using NIH ImageJ software.
2.6. Immunocytofluorescence
HPDLFs were seeded in chamber slides and were treated with 10 µg/ml P. gingivalis LPS for 24 h. The cells were fixed with 4% paraformaldehyde followed by 1 h of protein blocking and overnight incubation at 4°C with anti-Caspase-1 (1:50, Abcam, Tokyo, Japan), anti-Caspase-11 (1:50, Abcam, Tokyo, Japan) and anti-p-NF-κB (1:100, Bioss, Woburn, MA, USA). The cells were then incubated with IgG-Alexa Fluor 488 secondary antibody and mounted with DAPI (ProLong™ Gold, Foster City, CA, USA). A microscope (KEYENCE, Tokyo, Japan) was used to capture the images.
2.7. Immunohistochemistry
Antigen retrieval (10× Citrate Buffer pH 6.0, Abcam, Tokyo, Japan) was performed for specimens of the upper jaws and peroxidase blocking (Wako, Tokyo, Japan) was carried out, followed by incubation overnight at 4°C with anti-GSDMD (1:100, Abcam, Tokyo, Japan), anti-p-NF-κB (1:100, Bioss, Woburn, MA, USA) and anti-IL-1β (Abcam, Tokyo, Japan). The same DAB staining conditions were performed on each specimen after incubation with the secondary antibody.
2.8. Statistical analysis
Statistical analyses were performed using SPSS 16.0 and the results were assessed by an independent two-tailed Student’s t-test or analysis of variance (ANOVA). Differences are considered significant when the p value was less than 0.05.
3. Results
3.1. Dec1 is involved in LPS-induced cell pyroptosis
To evaluate the effects of LPS on HPDLFs, the cells were treated with 10 µg/ml P. gingivalis LPS for 24 h as previously reported (Cheng et al. Citation2018; Oka et al. Citation2021). The expression level of IL-1β was significantly and consistently induced by P. gingivalis LPS as shown by RT–PCR and by Western blot (Figure (A,C), p < 0.05). Interestingly, the expression level of Dec1 was also significantly increased by LPS treatment (Figure (B,C), p < 0.05). Coincidently, the cleavage of Caspase-1 (Figure (C), p < 0.05) and of GSDMD (Figure (C), p < 0.01) was increased significantly by treatment with P. gingivalis LPS, as was the level of phosphorylated NF-κB (Figure (C), p < 0.05). These results revealed that Dec1 is involved in cell pyroptosis induced by LPS.
3.2. LPS treatment activates Caspase protein expression
To verify that 10 µg/ml P. gingivalis LPS-induced cell pyroptosis, immunocytofluorescence was performed to detect Caspase and Dec1 protein expression levels. The staining results confirmed that the expression levels of Caspase-1 and Caspase-11 were upregulated in the cytoplasm in terms of LPS-induced pyroptosis (Figure ). Consistently, the downstream protein, phosphorylated NF-κB, and Dec1 were also activated and were translocated into the nuclei.
Figure 1. Dec1 is involved in LPS-induced cell pyroptosis of HPDLFs. A, RT–PCR analysis showing that the expression level of IL-1β was significantly induced by treatment with 10 µg/ml P. gingivalis LPS. B, RT–PCR analysis showing that the expression level of the transcription factor Dec1 was elevated by treatment with LPS. C, Left, Western blot analysis showing that the cleavage of Caspase-1 and GSDMD was activated by LPS, followed by the induction of phosphorylated NF-κB. Right, Quantitation of band density corrected against GAPDH showing means ± SD (n ≥ 3); *p < 0.05, **p < 0.01. All results are representative of at least three independent experiments.
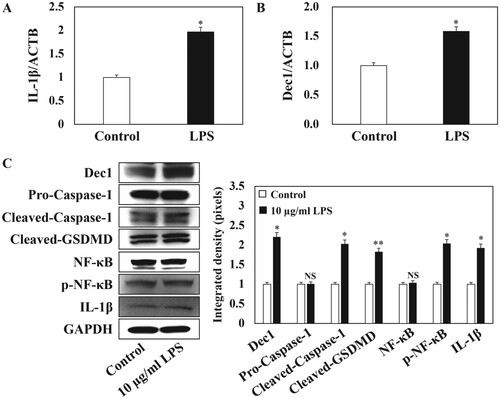
Figure 2. LPS treatment activates Caspase protein expression in HPDLFs. Immunocytofluorescence analysis showing that the expression of Caspase-1 and Caspase-11 was upregulated in the cytoplasm of 10 µg/ml P. gingivalis LPS-induced pyroptosis. Consistently, the downstream protein, p-NF-κB, and Dec1 were also activated and were translocated into the nuclei. The arrows indicate positive cells. Original magnification: 40×, scale bars = 50 µm. All results are representative of at least three independent experiments.
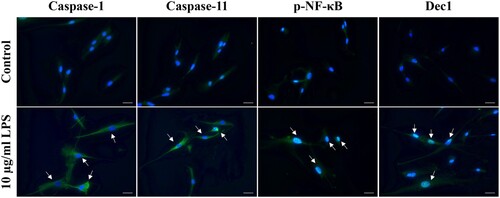
Figure 3. Reduced expression of Dec1 in HPDLFs attenuates LPS-induced pyroptosis. A, RT–PCR analysis showing that the expression of Dec1 was significantly suppressed after Dec1 siRNA transfection with or without LPS treatment. B, RT–PCR analysis showing that the reduced expression of Dec1 attenuated the expression level of IL-1β under LPS induction compared with the control with or without LPS treatment. C, Left, Western blot showing that the inhibition of Dec1 significantly impaired the expression of cleaved-Caspase-1 and cleaved-GSDMD, suppressed the phosphorylation of NF-κB, and decreased IL-1β expression. Right, Quantitation of band density corrected against GAPDH showing means ± SD (n ≥ 3); *p < 0.05, **p < 0.01. All results are representative of at least three independent experiments.
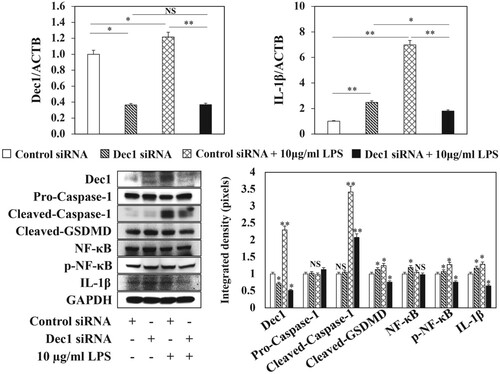
3.3. A dec1 deficiency attenuates LPS-induced pyroptosis
To characterize the role of Dec1 in LPS-induced pyroptosis, a Dec1 siRNA was utilized to silence the expression of the Dec1 gene. The expression levels of Dec1 mRNA and protein were significantly suppressed by the Dec1 siRNA transfection with or without LPS treatment (Figure (A,C), p < 0.05, p < 0.01). The reduced expression of Dec1 attenuated the expression levels of IL-1β mRNA and protein following treatment with LPS compared to the control (Figure (B,C), p < 0.01, p < 0.05). Furthermore, the inhibition of Dec1 impaired the expression of cleaved-Caspase-1 (Figure (C), p < 0.01) and cleaved-GSDMD (Figure (C), p < 0.05), suppressed the phosphorylation of NF-κB (Figure (C), p < 0.05), decreased IL-1β expression (Figure (C), p < 0.05) and reduced cell pyroptosis after treatment with P. gingivalis LPS.
3.4. The absence of Dec1 reduces pyroptosis in P. gingivalis-induced periodontitis
WT and Dec1KO mouse periodontitis models were used to confirm the function of Dec1 in pyroptosis. The expression of GSDMD was elevated in the periodontal ligament of WT mice compared with Dec1KO mice after treatment with P. gingivalis, and the phosphorylation of NF-κB was suppressed by the deficiency of Dec1 (Figure ). The reduced GSDMD and p-NF-κB attenuated the expression of IL-1β and thus reduced inflammation in periodontal tissues with a deficiency of Dec1. These results confirmed that the transcription factor Dec1 is involved in the P. gingivalis-induced periodontal tissue inflammation and is a positive regulator in periodontal cell pyroptosis and tissue destruction.
Figure 4. The absence of Dec1 reduces pyroptosis in P. gingivalis-induced periodontitis in mice. Immunohistochemistry showing that the expression of GSDMD, p-NF-κB and IL-1β in the periodontal ligament of WT and Dec1KO mice was suppressed by the absence of Dec1. Original magnification: 60×, scale bars = 20 µm. All results are representative of at least three independent experiments.
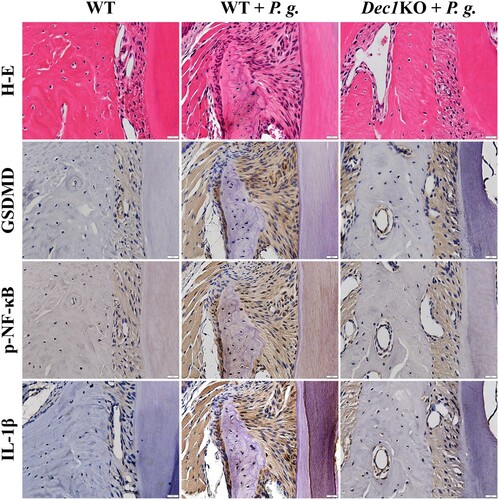
4. Discussion
The results of this study show that treatment with P. gingivalis LPS generates an inflammatory environment, which can exacerbate the functional effects of pyroptosis in the periodontium. This assumption was further explored using Dec1KO mice that serve as a suitable model for studying the P. gingivalis LPS-induced pyroptotic effects. We previously used flow cytometry to confirm an increase in the expression of inflammatory mediators in gingival tissues of WT mice compared to Dec1KO mice with experimentally induced periodontitis. That was further validated using quantitative RT–PCR (Zhang et al. Citation2018). The findings of the present study revealed that the pyroptosis might be due to the activation of Caspase proteins in HPDLFs. Moreover, the results showed that a Dec1 deficiency can block the Caspase pathway and inhibit the cascading consequences of pyroptosis. The Dec1 deficiency regulates the immune response and the defense mechanism against microbial infections, both of which indicate a new mechanism for the pathogenesis of periodontitis. To the best of our knowledge, this is the first study that explores the association between Dec1 function and periodontal pyroptosis.
Caspase-1 is a common instigator and driver of disease that has been implicated in the pathophysiology and severity of inflammatory diseases (Bergsbaken et al. Citation2009). Inflammation and excessive cell death have been associated with an elevation or abnormal activation of caspase-1 (Simon and van der Meer Citation2007). We observed that the expression levels of cleaved-Caspase-1 and Dec1 were increased in LPS-induced HPDLFs (Figure ). NF-κB activation resulted in the Caspase-1 activation, suggesting that the levels of phosphorylated NF-κB may reflect disease severity (Figure ). The phosphorylation of NF-κB is also a critical step to produce inflammasomes that stimulate pyroptosis and IL-1β release. We confirmed the increased mRNA and protein expression levels of IL-1β in LPS-induced HPDLFs. Similar secretion levels of other inflammatory chemokines could be stimulated by activated inflammasomes and cleaved-Caspase-1 leading to pyroptosis.
Bacterial LPS, a major immunogenic component of the cell wall of Gram-negative bacteria, is the essential pathogen-associated molecular pattern (PAMP) responsible for the development of inflammation during Gram-negative bacterial infections. LPS can cause Caspase-1-mediated pyroptosis and promotes the release of proinflammatory cytokines in HPDLFs (Cheng et al. Citation2018). This has also been attributed to the LPS mediated interaction and activation of Caspase, which results in the oligomerization and activation of Caspases followed by pyroptosis (Shi et al. Citation2014). In this study, LPS resulted in the Caspase-1 and Caspase-11 mediated activation of GSDMD in HPDLFs, indicating the distinct nature of this mechanism.
Furthermore, incubation of LPS-stimulated HPDLFs in the presence of a Dec1 siRNA resulted in a significantly reduced expression of IL-1β and NF-κB, supporting a role for Caspase-1 in periodontal pathogenesis. Our observations are the first to show such activities in HPDLFs and are the first to demonstrate that the anti-death effect of Dec1-deficient cells is related to the inhibition of GSDMD gene expression.
Pyroptosis leads to an excessive inflammatory response, the accumulation of inflammatory mediators and the infiltration of inflammatory cells. GSDMD is a direct substrate of Caspase-11 and is critical for cell death and IL-1β release. The expression of GSDMD and inflammatory proteins in HPDLFs increased remarkably in response to a 24 h LPS challenge, indicating that LPS induced the expression and activation of GSDMD, which supports the hypothesis that pyroptosis markers respond to microbial stimuli in an inflammatory manner. Further, the Dec1 deficiency impaired the LPS-induced expression of IL-1β and the activation of GSDMD. Those gene expression patterns showed discrepancies with the higher levels of gene expression in the parental cells (Figure (C)) compared to transfected cells (Figure (C)) following the treatment with LPS. We consider that the high cell density used in the siRNA transfection procedure might cause contact inhibition, resulting in the poor uptake of nucleic acids and/or the decreased expression of target genes.
5. Conclusion
Inflammation in the periodontal ligament may contribute to the resorption of alveolar bone and possibly facilitates the development of apical periodontitis. It is therefore tempting to speculate that the mechanisms identified in this study could effectively be targeted to help limit the inflammation-induced acceleration of disease progression in periodontal inflammation. Our study illustrated the role of GSDMD in LPS-induced inflammatory reactions and pyroptosis via a Caspase-1-related mechanism in PDLFs, demonstrated that a Dec1 deficiency acts as an anti-death molecule, and is the first to show that the anti-death activity of the Dec1 deficiency is related to GSDMD in PDLFs. We identified an association between pyroptosis signaling and Dec1-deficient inflammation, which suggests the therapeutic potential of targeting inflammation pathways and Dec1 function in periodontal inflammation.
Acknowledgements
We would like to thank Dr. Toyama and Dr. Sato for the P. gingivalis treatment and the staff of the animal facility for care of the mice. We thank Dr. Fujita for technical assistance.
Disclosure statement
No potential conflict of interest was reported by the author(s).
Data availability statement
The data that supporting the findings of this study are openly available in figshare at https://doi.org/10.6084/m9.figshare.13102865.v1.
Additional information
Funding
References
- Aglietti RA, Estevez A, Gupta A, et al. 2016. GSDMD p30 elicited by caspase-11 during pyroptosis forms pores in membrane. Proc Natl Acad Sci USA. 113(28):7858–7863.
- Bek MJ, Wahle S, Müller B, et al. 2003. Stra13, a prostaglandin E2-induced gene, regulates the cellular redox state of podocytes. FASEB J. 17(6):682–684.
- Bergsbaken T, Fink SL, Cookson BT. 2009. Pyroptosis: host cell death and inflammation. Nat Rev Microbiol. 7(2):99–109.
- Bhawal UK, Ito Y, Tanimoto K, et al. 2012. IL-1β-mediated up-regulation of DEC1 in human gingiva cells via the Akt pathway. J Cell Biochem. 113(10):3246–3253.
- Bhawal UK, Sato F, Arakawa Y, et al. 2011. Basic helix-loop-helix transcription factor DEC1 negatively regulates cyclin D1. J Pathol. 224(3):420–429.
- Chen X, He WT, Hu L, et al. 2016. Pyroptosis is driven by non-selective gasdermin-D pore and its morphology is different from MLKL channel-mediated necroptosis. Cell Res. 26(9):1007–1020.
- Cheng R, Feng Y, Zhang R, Liu W, Lei L, Hu T. 2018. The extent of pyroptosis varies in different stages of apical periodontitis. Biochim Biophys Acta Mol Basis Dis. 1864(1):226–237.
- Cheng R, Hu T, Bhowmick NA. 2015. Be resistant to apoptosis: a host factor from gingival fibroblasts. Cell Death Dis. 6(12):e2009.
- Ding J, Wang K, Liu W, et al. 2016. Pore-forming activity and structural autoinhibition of the gasdermin family. Nature. 535(7610):111–116.
- Hagar JA, Powell DA, Aachoui Y, Ernst RK, Miao EA. 2013. Cytoplasmic LPS activates caspase-11: implications in TLR4-independent endotoxic shock. Science. 341(6151):1250–1253.
- He WT, Wan H, Hu L, et al. 2015. Gasdermin D is an executor of pyroptosis and required for interleukin-1β secretion. Cell Res. 25(12):1285–1298.
- Iizuka K, Horikawa Y. 2008. Regulation of lipogenesis via BHLHB2/DEC1 and ChREBP feedback looping. Biochem Biophys Res Commun. 374(1):95–100.
- Jiang X, Tian F, Du Y, et al. 2008. BHLHB2 controls bdnf promoter 4 activity and neuronal excitability. J Neurosci. 28(5):1118–1130.
- Kayagaki N, Stowe IB, Lee BL, et al. 2015. Caspase-11 cleaves gasdermin D for non-canonical inflammasome signalling. Nature. 526(7575):666–671.
- Kayagaki N, Wong MT, Stowe IB, et al. 2013. Noncanonical inflammasome activation by intracellular LPS independent of TLR4. Science. 341(6151):1246–1249.
- Kim JH, Kang MS, Eltohamy M, Kim TH, Kim HW. 2016. Dynamic mechanical and nanofibrous topological combinatory cues designed for periodontal ligament engineering. PLoS One. 11(3):e0149967.
- Kinane DF. 2001. Causation and pathogenesis of periodontal disease. Periodontol 2000. 25:8–20.
- Lamkanfi M, Dixit VM. 2014. Mechanisms and functions of inflammasomes. Cell. 157(5):1013–1022.
- Man SM, Kanneganti TD. 2016. Converging roles of caspases in inflammasome activation, cell death and innate immunity. Nat Rev Immunol. 16(1):7–21.
- Martinon F, Tschopp J. 2004. Inflammatory caspases: linking an intracellular innate immune system to autoinflammatory diseases. Cell. 117(5):561–574.
- Martínez-Llordella M, Esensten JH, Bailey-Bucktrout SL, et al. 2013. CD28-inducible transcription factor DEC1 is required for efficient autoreactive CD4+ T cell response. J Exp Med. 210(8):1603–1619.
- Matsuda N, Yokoyama K, Takeshita S, Watanabe M. 1998. Role of epidermal growth factor and its receptor in mechanical stress-induced differentiation of human periodontal ligament cells in vitro. Arch Oral Biol. 43(12):987–997.
- Miyazaki K, Miyazaki M, Guo Y, et al. 2010. The role of the basic helix-loop-helix transcription factor Dec1 in the regulatory T cells. J Immunol. 185(12):7330–7339.
- Oka S, Li X, Sato F, et al. 2021. A deficiency of Dec2 triggers periodontal inflammation and pyroptosis. J Periodontal Res. doi:10.1111/jre.12849.
- Peleg R, Carmi Y, Guttman O, et al. 2011. IL-1α and IL-1β recruit different myeloid cells and promote different stages of sterile inflammation. J Immunol. 187(9):4835–4843.
- Shi J, Gao W, Shao F. 2017. Pyroptosis: gasdermin-mediated programmed necrotic cell death. Trends Biochem Sci. 42(4):245–254.
- Shi J, Zhao Y, Wang K, et al. 2015. Cleavage of GSDMD by inflammatory caspases determines pyroptotic cell death. Nature. 526(7575):660–665.
- Shi J, Zhao Y, Wang Y, et al. 2014. Inflammatory caspases are innate immune receptors for intracellular LPS. Nature. 514(7521):187–192.
- Simon A, van der Meer JW. 2007. Pathogenesis of familial periodic fever syndromes or hereditary autoinflammatory syndromes. Am J Physiol Regul Integr Comp Physiol. 292(1):R86–R98.
- Tribble GD, Lamont RJ. 2010. Bacterial invasion of epithelial cells and spreading in periodontal tissue. Periodontol 2000. 52(1):68–83.
- Vercherat C, Chung TK, Yalcin S, et al. 2009. Stra13 regulates oxidative stress mediated skeletal muscle degeneration. Hum Mol Genet. 18(22):4304–4316.
- Walle LV, Lamkanfi M. 2016. Pyroptosis. Curr Biol. 26(13):R568–R572.
- Yang Y, Rossi FMV, Putnins EE. 2010. Periodontal regeneration using engineered bone marrow mesenchymal stromal cells. Biomaterials. 31(33):8574–8582.
- Zhang F, Suzuki M, Kim IS, et al. 2018. Transcription factor DEC1 is required for maximal experimentally induced periodontal inflammation. J Periodontal Res. 53(5):883–893.
- Zvonic S, Ptitsyn AA, Conrad SA, et al. 2006. Characterization of peripheral circadian clocks in adipose tissues. Diabetes. 55(4):962–970.