Abstract
Heptaphylline, a carbazole alkaloid, has been shown to exert anticancer effects against different human cancers. The study was designed to evaluate the anticancer effects of heptaphylline against the human pancreatic cancer cell and to explore the underlying mechanism. The results showed that heptaphylline inhibited the proliferation of all the pancreatic cancer cell lines with lowest IC50 of 12 µM against the PANC1 cells. In contrary, the IC50 of heptaphylline was around eight times higher against the normal H6c7 cells. The transwell assays revealed heptaphylline inhibited the migration and invasion of pancreatic cancer cells in a concentration-dependent manner. Additionally, the epithelial to mesenchymal transition of the PANC1 cells was also inhibited upon treatment with heptaphylline. The antiproliferative effects of heptaphylline were exerted via induction of apoptosis in pancreatic cancer cells. The apoptosis was associated with an increase in Bax, decrease in Bcl-2 expression, discharge of cytochrome c and cleavage of PARP, caspase-3 and caspase-9. AO staining revealed that heptaphylline induced autophagy in PANC1 cancer cells by increasing the expression of LC3B-II and Beclin-1. Taken together, heptaphylline suppresses the growth of pancreatic cancer via induction of apoptosis and autophagy and may prove a potent lead molecule for the development of chemotherapy.
Introduction
Pancreatic cancer is one of the deadliest forms of human cancer with considerably lower survival rate (Mizrahi et al. Citation2020). The prognosis of pancreatic cancer is extremely poor and in more than 80% of the cases, the malignancy is detected only at unresectable or metastatic stages (Siegel et al. Citation2019). In the USA, the over-all 5-year survival rate reported for pancreatic cancer is below 10%. According to estimates, the pancreatic cancer will be the second most commonly diagnosed cancer in developed countries like USA (Rahib et al. Citation2014). Because of the diagnosis of pancreatic cancer at later stages, heterogenous nature and therapeutic resistance, there is an urgent need to develop efficient treatment strategies against this fatal malignancy (Yao et al. Citation2020). Plant-derived carbazole alkaloids have been shown to exhibit enormous pharmacological potential and have been reported to exhibit anticancer activities against different types of human cancers (Huang et al. Citation2017; Issa et al. Citation2019; Sun et al. Citation2020). The compounds belonging to carbazole alkaloid group of plant secondary metabolites have been shown to inhibit the growth of cancers mainly through cell death induced via multiple mechanisms. The carbazole alkaloids from Murraya koenigii triggered apoptosis and autophagy in oral cancer cells (Utaipan et al. Citation2017). Carbazole alkaloids of other plants like Clausena harmandiana have also been shown to exhibit pro-apoptotic effects against different human cancers (Jantamat et al. Citation2019). Heptaphylline (Figure (A)), a carbazole alkaloid has been traditionally extracted from many plant species of Clausena genus like C. heptaphylla (Joshi et al. Citation1967). However, this compound can also be synthesized through organic chemistry approaches (Thongthoom et al. Citation2011). Heptaphylline has been shown to induce apoptosis in several human cancer cell types such as colon adenocarcinoma cells and bladder cancer cells (Boonyarat et al. Citation2015). Additionally, it has also been shown to induce intrinsic apoptosis in human bladder cancer cells (Xu et al. Citation2020). However, the antiproliferative activity of heptaphylline has as not been studied against the pancreatic cancer cells. Against this backdrop, the present study was designed to investigate the anticancer effects of heptaphylline against the human pancreatic cancer cells and to explore the underlying mechanisms.
Figure 1. Heptaphylline inhibits pancreatic cancer cell growth. (A) Molecular formula of heptaphylline (B) MTT proliferation assay of PANC1 pancreatic cancer cells administered with 0–160 µM heptaphylline (C) MTT proliferation assay of H6c7 normal pancreatic ductal cells administered with 0–160 µM heptaphylline (D) clonogenic assay of PANC1 pancreatic cancer cells administered with 0, 6, 12 µM or 18 µM heptaphylline. Experiments were performed independently in triplicates and data presented as mean ± SD (*P < .05).
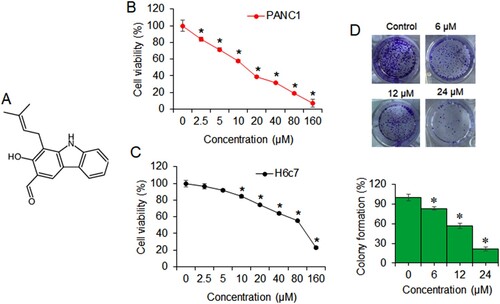
Materials and methods
Chemicals and reagents
Heptaphylline (98% purity by HPLC (High Performance Liquid Chromatography)) was bought from Tauto Biotech, Shanghai, China and other chemicals were purchased from Sigma Chemical Co. (St. Louis, MO, USA) unless otherwise mentioned. Feral bovine serum (FBS), antibiotics and Dulbecco’s Modified Eagle Medium were obtained from Thermo Fisher Scientific (Waltham, MA, USA). The antibodies were obtained from Santa Cruz Biotechnology, Inc. (Dallas, TX, USA)
Culture of cell lines
Human pancreatic cancer cell lines (Capan-1, Capan-2, PANC1 and MIA PaCa-2) and normal pancreatic ductal cells (H6c7) were purchased from American Type Collection Center (ATCC, USA). RPMI 1640 medium (Thermo Fisher Scientific, USA) supplemented with 10% FBS (Sigma-Aldrich, Germany) was used for cell culturing at 37°C. The cells were maintained in a 5% CO2-humidified atmosphere.
Cell viability assays
The cell viability was determined by MTT (3-(4,5-dimethylthiazol-2-yl)-2,5-diphenyltetrazolium bromide, a tetrazole) assay as described previously (Kumar et al. Citation2018). The pancreatic cancer cell lines and H6c7 normal pancreatic cells were seeded at the density of 2 × 104 cells/well in 96-well plates and cultured with heptaphylline concentrations of 0 to160 µM for 24 h. MTT solution was added to each well to make final the final concentration of 0.5% and subsequently incubated for an additional 3 h at 37°C. This was followed by the addition of 250 µL DMSO for dissolving the formazan crystals. Absorbance at 570 nm was recorded on a micro-plate reader. Independent experiments were repeated with three replicates.
Clonogenic assay
The clonogenic assay was performed as described previously (Gou et al. Citation2017). Briefly, the PANC1cancer cells were grown in 6-well plates with initial cell count of 5000 cells/well. The wells were treated with 0, 6, 12 µM or 24 µM of heptaphylline. The cell culturing was performed for 2-weeks at 37°C. The colonies were then fixed with ethanol, stained with 0.5% crystal violet and subsequently visualized under a dissecting microscope for manual counting. The experiments were carried out independently in triplicates.
Cell migration and invasion assays
The cell migration assay was performed as described previously (Valster et al. Citation2005). In brief, 104 PANC1 cells were plated into the upper chamber which besides received heptaphylline at 0, 6, 12 µM or 24 µM. RPMI medium with 10% FBS was added into the lower chamber. After 37°C incubation for 24 h, the cells which had migrated to the lower chamber were fixed with 4% paraformaldehyde and stained with 0.1% crystal violet for 30 min. Light microscope (Olympus, Japan) was used for examining and photographing of migrated cells. The Transwell invasion assay was performed similarly to that of the migration assay except that the upper chamber was fitted with Matrigel matrix (BD Biosciences).
Western blotting
The PANC1 cancer cells treated with 6, 12 or 24 µM along with untreated PANC1 cells were lysed using cell lysing solution of 1% protease inhibitor phenylmethylsulfonyl fluoride (PSMF) and 1 mL RIPA. The extracted proteins were run on denaturing Polyacrylamide gel electrophoresis (PAGE) gels and subsequently transferred to the polyvinylidene fluoride (PVDF) membranes. After blocking with 5% non-fat milk for 1 h at room temperature, the PVDF membranes were treated with specific primary antibodies (Aviva Systems Biology) at 4°C for 12 h. Afterwards, these PVDF membranes incubated with horseradish peroxidase-conjugated secondary antibodies for 2 h at room temperature. The protein bands on PVDF membranes were detected with Odyssey imaging system (LI-COR, US). β-actin protein was used as internal control.
DAPI and Annexin V/PI staining assay
Apoptosis was detected by using 4′,6-diamidino-2-phenylindole (DAPI) staining and Annexin V-FITC Apoptosis Detection Kit (Invitrogen, Carlsbad, CA, USA) as described previously (Zhang et al. Citation2018). In brief, PANC1 cancer cells variably treated with heptaphylline (0, 6, 12 or 24 µM) in 12-well plates at the initial cellular density of 2 × 105 cells per well for 24 h at 37°C were harvested post-treatment followed by trypsinization and their fixing using methanol. The cells were either directly stained with DAPI solution or mixed with binding buffer and then with 10 µL Annexin V-FITC and 20 µL PI in dark at room temperature for 20 min. The DAPI stained cells were examined under the fluorescent microscope while the Annexin V-FITC/PI dually stained cells were analyzed by flow cytometry (BD Biosciences, San Jose, CA, USA).
Acridine orange staining
The analysis of cell autophagy was made using Acridine orange (AO) staining method (Thomé et al. Citation2016). The cancer cells treated with varying doses of heptaphylline were fixed with 70% ethanol and stained using AO. The cells were subsequently visualized for fluorescence using fluorescent microscopy.
Statistical analysis
The SPSS 13.0 software (SPSS, Chicago, USA) was used for all the statistical analyses. Two-tailed Student’s t-test and one-way analysis of variance were used for comparing the difference between the two treatment groups. The statistical differences were significant if p-value was less than .05. Final values were represented as mean ± standard deviation.
Results
Heptaphylline inhibits the growth of human pancreatic cancer cells
The pancreatic cancer cells were treated with varying doses of heptaphylline (0–160 µM) for 24 h at 37°C. The proliferation rate was deduced through MTT assay. The results showed that heptaphylline suppressed the growth of all the pancreatic cancer cells dose-dependent manner. The IC50 of heptaphylline against the pancreatic cancer cells ranged from 12 to 16 µM (Table ). The lowest IC50 of 12 µM was observed against the PANC1 pancreatic cancer cells (Figure (B)). Under similar heptaphylline treatment concentrations, the proliferation of normal pancreatic ductal cells, H6c7, was marginally affected with IC50 of 90 µM (Figure (C)). Colony formation of PANC1 cancer cells was also shown to be inhibited by heptaphylline in dose-dependent manner (Figure (D)). The relative percentage of PANC1 colony formation was 82%, 61% and 19%, respectively, at 6, 12and 18 µM of heptaphylline with reference to that of the untreated control cells.
Table 1. IC50 of Heptaphylline against different human pancreatic cancer cell lines and normal pancreatic cells as determined by MTT assay
Heptaphylline inhibits epithelial to mesenchymal transition of pancreatic cancer cells
The migration and invasion of PANC1 at 6 , 12 and 18 µM of heptaphylline was assessed with reference to control untreated cancer cells with transwell assay. It was found that the PANC1 cell migration decreased proportionally with the treatment concentration (Figure (A)). At 18 µM treatment concentration, the decline in cell migration was more than 70%. The invasion of the PANC1 cancer cells was also affected in similar manner and the cancer cells showed only 20% invasiveness at 18 µM of heptaphylline (Figure (B)). Protein expression analysis indicated that heptaphylline repressed the expression of mesenchymal marker proteins (N-cadherin and vimentin) while the expression of E-cadherin (epithelial marker) protein increased with increasing heptaphylline concentrations in PANC1 cancer cells (Figure (C)). The results thus suggest that heptaphylline inhibits the epithelial to mesenchymal transition (EMT) of pancreatic cancer cells.
Figure 2. Heptaphylline decreases migration, invasion and EMT of pancreatic cancer cells. (A) Transwell invasion assay of PANC1 pancreatic cancer cells administered with 0, 6, 12 or 18 µM heptaphylline (B) transwell migration assay of PANC1 pancreatic cancer cells administered with 0, 6, 12 µM or 18 µM heptaphylline (C) expression analysis of epithelial (E-cadherin) and mesenchymal (N-cadherin and vimentin) marker proteins from PANC1 pancreatic cancer cells administered with 0, 6, 12 or 18 µM heptaphylline. Experiments were performed independently in triplicates and data presented as mean ± SD (*P < .05).
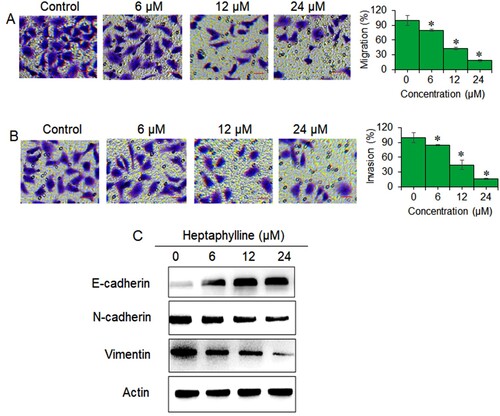
Heptaphylline induces apoptosis in pancreatic cancer cells
The DAPI staining of PANC1 cancer cells administered with heptaphylline (0–18 µM) showed that heptaphylline-treated cells showed signs of nuclear deformation and the lesions were more prominent at higher treatment concentrations which might signify the apoptosis (Figure (A)). Flow cytometry was used for the study of the apoptosis of the PANC1 cancer cells treated with 0, 6, 12 or 18 µM heptaphylline. The relative percentage of both late apoptotic (quadrant 2) and early apoptotic cancer cells (quadrant 4) was shown to be significantly increased by heptaphylline and showed dose-dependence (Figure (B)). The induction of pancreatic cancer cell apoptosis by heptaphylline was also supported by western blotting results. The heptaphylline-treated cells exhibited comparatively higher expression levels of apoptosis marker proteins like Bax, cleaved PARP and cleaved caspases (3 and 9) while the expression of Bcl-2 and cytochrome c was shown to be decreasing in heptaphylline-treated cells dose-dependent fashion (Figure (C)).
Figure 3. Heptaphylline induces apoptosis in pancreatic cancer cells. (A) DAPI staining of PANC1 pancreatic cancer cells administered with 0, 6, 12 µM or 18 µM heptaphylline (B) flow cytometric analysis of PANC1 pancreatic cancer cells administered with 0, 6, 12 or 18 µM heptaphylline (C) expression analysis of apoptosis marker proteins from PANC1 pancreatic cancer cells administered with 0, 6, 12 µM or 18 µM heptaphylline. Experiments were performed independently in triplicates.
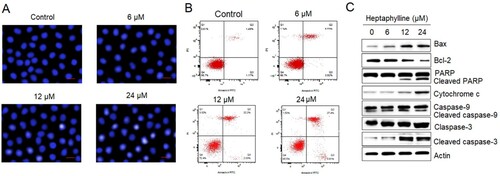
Heptaphylline induces autophagy in pancreatic cancer cells
AO staining was used for analyzing the autophagy of PANC1 cancer cells administered with heptaphylline (0, 6, 12 or 18 µM). The AO stained revealed the development of the autophagic vesicles in the heptaphylline-treated PANC1 suggestive of autophagy (Figure (A)). Moreover, the levels of autophagy marker proteins (Beclin 1, LC3B I and II) in PANC1 cancer cells were shown to be increasing in dose-dependent manner by heptaphylline further confirming the induction of autophagy in pancreatic cancer cells by heptaphylline (Figure (B)).
Figure 4. Heptaphylline induces autophagy in pancreatic cancer cells. (A) AO staining analysis of PANC1 pancreatic cancer cells administered with 0, 6, 12 or 18 µM heptaphylline (Arrows depict autophagic vesicles) (B) expression analysis of autophagy marker proteins from PANC1 pancreatic cancer cells administered with 0, 6, 12 or 18 µM heptaphylline. Experiments were performed independently in triplicates.
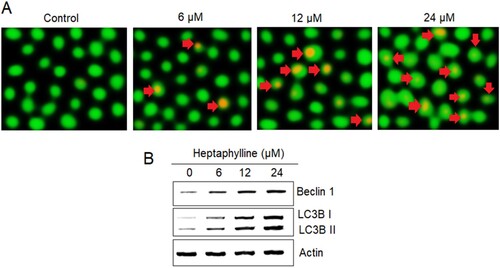
Discussion
During recent times, the natural compounds have been shown to exhibit anticancer activities against different types of human cancers (Ahmad et al. Citation2020). The plant-based natural products were suggested to offer favorable effects in curing human cancers through targeting of the autophagic pathways in cancer cells (Forouzanfar and Mousavi Citation2020). The carbazole plant alkaloids were also shown to exhibit pro-apoptotic and autophagy inducing potential against the human cancer cells (Huang et al. Citation2017). A carbazole alkaloid, heptaphylline has antiproliferative activity against human colon adenocarcinoma cells (Boonyarat et al. Citation2015) and bladder cancer cells (Xu et al. Citation2020). In the present study, heptaphylline inhibited the growth of pancreatic cancer cells and the cytotoxic effects were significantly against the cancer cells while the normal pancreatic cells were minimally affected. The IC50 of heptaphylline was 12 µM against the pancreatic cancer cells while it was 80 µM against the normal pancreatic cells. The cancer cells specific growth inhibitory effects of heptaphylline have also been reported in other studies (Boonyarat et al. Citation2015; Xu et al. Citation2020). However, the underlying reasons for this cancer-specific activity of heptaphylline have not been studied and remain an important area for further investigation. The migration and invasion of the cancer cells is the initial step that eventually leads to cancer metastasis. In the present study, we found that heptaphylline suppressed the migration and invasion of the cancer cells suggestive of its anti-metastatic potential (Liotta Citation1992). The reason for the decline in cancer cells migration and invasion could be the inhibition of the EMT of pancreatic cancer cells as suggested by the western blotting of EMT molecular marker proteins. DAPI staining showed that apoptosis was induced in pancreatic cancer cells by heptaphylline. The results indicated that the administration of pancreatic cancer cells with heptaphylline enhanced the activation of apoptosis marker proteins like PARP, Bax and Caspases (3 and 9) while the Bcl-2 protein expression was repressed suggesting the onset of programmed cell death (Fribley et al. Citation2009). Furthermore, the AO/EB dual staining results showed that heptaphylline induced autophagy in pancreatic cancer cells which is in coherence with its previous established activity (Xu et al. Citation2020). The induction of autophagy in pancreatic cancer cells by heptaphylline were also evidenced from the expression analysis of autophagy marker proteins. Cancer cells treated with heptaphylline expressed higher levels of autophagy marker proteins like Beclin1, LC3B I and II which confirmed the autophagy induction (Mizushima Citation2004). Summing up, heptaphylline inhibits epithelial to mesenchymal transition and induces apoptosis and autophagy in pancreatic cancer cells to restrict their in vitro proliferation, migration and invasion and might emerge as a vital anticancer lead molecule against pancreatic cancer in future however the results must need in vivo confirmation.
Conclusions
The results of the current study are suggestive of anticancer effects of heptaphylline against the pancreatic cancer cells. The cancer cells exhibited significantly reduced proliferation, migration, and invasion upon treatment with heptaphylline, in vitro. Besides, heptaphylline inhibited the EMT of pancreatic cancer cells. The induction of apoptosis and autophagy was found to be responsible for the anticancer activity of heptaphylline against the pancreatic cancer cells. The results suggest the use of heptaphylline as the lead molecule for the treatment of pancreatic cancer. Semi-synthetic chemistry approaches might prove helpful in enhancing the anticancer activity of heptaphylline in future.
Acknowledgements
The authors acknowledge Department of Gastroenterology, Shenzhen People’s Hospital (The Second Clinical Medical College, Jinan University; The First Affiliated Hospital, Southern University of Science and Technology), Shenzhen, Guangdong China, for providing the laboratory facility for conducting the experiments.
Disclosure statement
No potential conflict of interest was reported by the author(s).
Data availability statement
That data which supports the findings of the present study are available at the figshare repository (https://figshare.com/) at https://figshare.com/s/00d5684af02f6e56e993.
Additional information
Funding
References
- Ahmad R, Khan MA, Srivastava AN, Gupta A, Srivastava A, Jafri TR, Siddiqui Z, Chaubey S, Khan T, Srivastava AK. 2020. Anticancer potential of dietary natural products: a comprehensive review. Anticancer Agents Med Chem. 20:122–236.
- Boonyarat C, Yenjai C, Vajragupta O, Waiwut P. 2015. Heptaphylline induces apoptosis in human colon adenocarcinoma cells through bid and Akt/NF-κB (p65) pathways. Asian Pac J Cancer Prev. 15:10483–7.
- Forouzanfar F, Mousavi SH. 2020. Targeting autophagic pathways by plant natural compounds in cancer treatment. Curr Drug Targets. 21(12):1237–1249.
- Fribley A, Zhang K, Kaufman RJ. 2009. Regulation of apoptosis by the unfolded protein response. In: Apoptosis. Totowa, NJ: Humana Press; p. 191–204.
- Gou S, Liu T, Wang C, Yin T, Li K, Yang M, Zhou J. 2007 May 1. Establishment of clonal colony-forming assay for propagation of pancreatic cancer cells with stem cell properties. Pancreas. 34(4):429–435.
- Huang L, Zhe-Ling FE, Yi-Tao WA, Li-Gen LI. 2017. Anticancer carbazole alkaloids and coumarins from Clausena plants: a review. Chin J Nat Med. 15:881–888.
- Issa S, Prandina A, Bedel N, Rongved P, Yous S, Le Borgne M, Bouaziz Z. 2019. Carbazole scaffolds in cancer therapy: a review from 2012 to 2018. J Enzyme Inhib Med Chem. 34:1321–1346.
- Jantamat P, Weerapreeyakul N, Puthongking P. 2019. Cytotoxicity and apoptosis induction of coumarins and carbazole alkaloids from Clausena harmandiana. Molecules. 24:3385.
- Joshi BS, Kamat VN, Saksena AK, Govindachari TR. 1967. Structure of heptaphylline, a carbazole alkaloid from clausena heptaphylla wt. & arn. Tetrahedron Lett. 8:4019–4022.
- Kumar P, Nagarajan A, Uchil PD. 2018. Analysis of cell viability by the MTT assay. Cold Spring Harb Protoc. 6:pdb-rot095505.
- Liotta LA. 1992. Cancer cell invasion and metastasis. Sci Am. 266(2):54–63.
- Mizrahi JD, Surana R, Valle JW, Shroff RT. 2020. Pancreatic cancer. Lancet. 395:2008–2020.
- Mizushima N. 2004. Methods for monitoring autophagy. Int J Biochem Cell Biol. 36:2491–2502.
- Rahib L, Smith BD, Aizenberg R, Rosenzweig AB, Fleshman JM, Matrisian LM. 2014. Projecting cancer incidence and deaths to 2030: the unexpected burden of thyroid, liver, and pancreas cancers in the United States. Cancer res. 74:2913–2921.
- Siegel RL, Miller KD, Jemal A. 2019. Cancer statistics, 2019. CA Cancer J Clin. 69:7–34.
- Sun XY, Ma J, Li CJ, Zang YD, Huang JW, Wang XY, Chen NH, Chen XG, Zhang DM. 2020. Carbazole alkaloids with bioactivities from the stems of Clausena lansium. Phytochem Lett. 38:28–32.
- Thomé MP, Filippi-Chiela EC, Villodre ES, Migliavaca CB, Onzi GR, Felipe KB, Lenz G. 2016 Dec. Ratiometric analysis of Acridine orange staining in the study of acidic organelles and autophagy. J Cell Sci. 129(24):4622–4632.
- Thongthoom T, Promsuwan P, Yenjai C. 2011. Synthesis and cytotoxic activity of the heptaphylline and 7-methoxyheptaphylline series. Eur J Med Chem. 46(9):3755–3761.
- Utaipan T, Athipornchai A, Suksamrarn A, Jirachotikoon C, Yuan X, Lertcanawanichakul M, Chunglok W. 2017. Carbazole alkaloids from Murraya koenigii trigger apoptosis and autophagic flux inhibition in human oral squamous cell carcinoma cells. J Nat Med. 7:158–169.
- Valster A, Tran NL, Nakada M, Berens ME, Chan AY, Symons M. 2005. Cell migration and invasion assays. Methods. 37(2):208–215.
- Xu A, Yang GG, Zhang Y, Zhao ST. 2020. Heptaphylline suppresses the proliferation and migration of human bladder cancer cells via induction of intrinsic apoptosis, autophagy and inhibition of β-catenin signalling pathway. J BUON. 25:274–279.
- Yao W, Maitra A, Ying H. 2020. Recent insights into the biology of pancreatic cancer. EBiomed. 2:102655.
- Zhang J, Liu L, Wang J, Ren B, Zhang L, Li W. 2018. Formononetin, an isoflavone from Astragalus membranaceus inhibits proliferation and metastasis of ovarian cancer cells. J Ethnopharmacol. 221:91–99.