Abstract
Steroidal glycoalkaloids (SGAs) existing in most potato tissues are toxic to humans when the fresh weight is over 200 mg kg−1, and high SGAs content would also damage the quality of potato tubers. Sterol side chain reductase 2 (StSSR2) is a key enzyme in the biosynthetic of SGAs in potatoes. To reduce the concentration of SGAs in tetraploid cultivated potato (Solanum tuberosum L.), the gene StSSR2 was edited by the CRISPR/Cas9 system. A total number of 64 mutant lines were generated, and the mutation efficiency was 46%. The percentage of insertion was 6.25%, and the percentage of deletion was 93.75%. Compared to the wild-type (WT), the content of SGAs reduced significantly in the mutated potatoes. The lowest SGAs concentration was 54% of the WT level, which was recorded in the peels of line #360. In the tuber fleshes, the lowest SGAs were 66% of WT level, which was found in line #378. In the leaves, the lowest SGAs concentration was 44% of the WT level as found in lines #204 and #378. In a word, our results showed that the StSSR2 gene of tetraploid cultivated potato could be successfully edited by the CRISPR/Cas9 system to reduce toxic SGAs.
Introduction
As one of the staple crops worldwide, potatoes (Solanum tuberosum L.) can provide energy and nutrients to humans, including carbohydrates, proteins and vitamins (Hameed et al. Citation2018). However, potato is also known to contain some toxic substances, the foremost of which are the steroidal glycoalkaloids (SGAs). SGAs are nitrogen-containing secondary metabolites naturally produced in several species of Solanaceae, such as eggplant (Solanum melongea), tomato (Solanum lycopersicum) and potato (Heftmann Citation1983). Around 95% of SGAs are α-chaconine and α-solanine in cultivated potato tubers (Nahar et al. Citation2017). SGAs are beneficial to potato production for providing resistance to bacterial and viral diseases (Austin et al. Citation1988; Pehu et al. Citation1990; Rokka et al. Citation1994), antifungal activity (Fewell and Roddick Citation1997; Percival et al. Citation1998) and insect resistance (Sanford et al. Citation1992, Citation1997). Desirable flavors can be produced in prepared potato foods containing low concentrations of SGAs. However, SGAs become toxic to humans when their concentrations reach 200 mg kg−1 fresh weight (FW) in potato tubers (Valkonen et al. Citation1996).
The entire plant of the potato contains SGAs, which are particularly rich in sprouts, tuber peels and organs on the ground (Smith et al. Citation1996). Drought (Bejarano et al. Citation2000), high temperature (Lafta and Lorenzen Citation2000), light exposure of tubers (Dale et al. Citation1993; Percival et al. Citation1994) and wounding (Bergenstråhle et al. Citation1992; Choi et al. Citation1994) are the problems encountered in the process of growth, harvest and post-harvest treatments, showing to raise the SGAs contents of potato tubers. Light exposure hada great influence on SGAs in potato tubers, 841/13 (S. phureja lines) raised by almost 140 mg kg−1 FW, reaching 192 mg kg−1 FW after being exposed to light for 96 h in tubers (Griffiths and Dale Citation2001). The SGAs content also varied from year to year, the total SGAs level of Karin exceed 200 mg kg−1 FW in 1996, but <200 mg kg−1 FW in the year 1997, 1998 and 1999 (Hajslova et al. Citation2005). The storage of tubers at low temperature in the dark may maintain SGAs at relatively constant levels, but without substantial reduction (Kozukue and Mizuno Citation1990). SGAs could not be destroyed by the cooking or frying process (Smith et al. Citation1996). Chips are the most common potato processed products, it produces a large number of peels as a by-product, but are mostly abandoned (Stearns et al. Citation1994). According to the peeling method, potato peels accounted for 15–40% of the quality of potato products (Sepelev and Galoburda Citation2015), which caused great waste and economic loss. On the other hand, the above-ground of the plant is a huge resource in potato, however, the high SGAs content limits its application and causes waste. As a result, it is highly desirable for the exploration of commercial cultivars containing low levels of SGAs through breeding or biotechnological approaches.
The biosynthesis of SGAs is catalyzed by a series of enzymatic reactions, for example, the reaction of the previous steps are catalyzed through 3-hydroxy-3-methylglutaryl coenzyme A reductase (HMGR) (Suzuki and Muranaka Citation2007; Nes Citation2011), squalene synthase (PSS1/SQS1), and vetispiradiene (sesquiterpene) cyclase (PVS1/VS1) (Krits et al. Citation2007), squalene epoxidase (SQE1), cycloartenol synthase (CAS) and lanosterol synthase (LAS) (Kumar et al. Citation2017). Cholesterol, a precursor to SGAs, was synthesized by a sterol side chain reductase 2 (SSR2) and a delta(7)-sterol-C5(6)-desaturase (C5-SD) from cycloartenol (Rahier Citation2011; Bergenstråhle et al. Citation1996; Ohyama et al. Citation2013; Itkin et al. Citation2013; Petersson et al. Citation2013; Sawai et al. Citation2014; Cárdenas et al. Citation2016). Over-expression of an Arabidopsis thaliana LAS (AtLAS) in potato resulted in SGA accumulation reduced in tuber flesh and increased in leaves (Kumar et al. Citation2017). The gene StSSR2 silenced by RNAi and mutated by transcription activator-like effector nucleases (TALEN) could reduce the accumulation of cholesterol, sitosterol and SGAs in potato (Sawai et al. Citation2014). SGAs levels were not significantly affected in the leaves or tubers in the sense 35 S:DWF1 (SSR1) and 35 S:DWF1-L1 (SSR2) transformants, whereas SGAs levels were reduced both in leaves and tubers from asDWF1 and asDWF1-L transformants (Nahar et al. Citation2017). A group of GLYCOALKALOID METABOLISM (GAME) genes was involved in the core pathway to SGAs in potato and tomato. Silencing GAME4 gene in potato and tomato leading to the reductions of SGAs concentrations (Itkin et al. Citation2013). The final step of SGAs biosynthesis in potatoes is catalyzed by solanidine glycosyltransferases, SGT1, SGT2 and SGT3 (Moehs et al. Citation1997; McCue et al. Citation2005, Citation2007). Sterol C-24-methyltransferase type 1 (SMT1) and SSR1 (the homolog of SSR2) participate in downstream synthetic steps for phytosterols, sitosterol and campesterol (Diener et al. Citation2000; Sawai et al. Citation2014). The over-expression of soybean SMT1 in potato raised the contents of alkylated sterols converted from cycloartenol, but decreased the accumulation of cholesterol and total SGAs (Arnqvist et al. Citation2003). Over-expression and knockout GAME9/JRE4, the ERF transcription factor, also alter the expression patterns of SGAs and related genes such as SSR2 and C5-SD both in potato and tomato (Cárdenas et al. Citation2016; Thagun et al. Citation2016).
Because of tetrasomic inheritance and high heterozygosity of the cultivated potato, breeding new elite cultivars is generally difficult, complicated and time-consuming (Muthoni et al. Citation2015). The recent advance in genome editing technologies like Zinc finger nucleases (ZFNs), and TALENs are limited by technical complicacy and limited efficiency, yet the CRISPR/Cas9 is easy to apply with great efficiency (Karkute et al. Citation2017). The targeted mutation of StIAA2 (Wang et al. Citation2015), StALS1 (Butler et al. Citation2015, Citation2016; Veillet et al. Citation2019a, Citation2019b), StMYB44 (Zhou et al. Citation2017), StGBSS (Andersson et al. Citation2017, Citation2018; Kusano et al. Citation2018; Veillet et al. Citation2019a, Citation2019b) and St16DOX (Nakayasu et al. Citation2018) genes using the CRISPR/Cas9 system has been reported in diploid or tetraploid potatoes. Knocking out the St16DOX gene which encoded steroid 16α-hydroxylase in the biosynthesis of SGAs caused the complete abolition of SGAs accumulation in potato hairy roots (Nakayasu et al. Citation2018). However, recently, we can’t find a study on reducing SGAs in other tissues of potato by the CRISPR/Cas9 system.
Many environmental factors increased the content of SGAs in potato tubers while preventing SGAs from rising is time-consuming, and uneconomical through cultivation, post-harvest management and processing. On the other hand, above-ground plants are a huge resource in potato production, which is difficult to utilize due to the high content of SGAs. Therefore, breeding low SGAs potato resources is the most effective way to solve these problems. The help of new biotechnology and new genetic improvement strategy is an important way of cultivating resources. As its high efficiency, easy to use and transgene-free via selfing or hybridization, gene editing is widely applied in crop improvement and breeding. StSSR2 knocked out by TALEN resulted in significant reductions of predominant SGAs contents in stems, tuber peels and leaves of potato plants, respectively (Sawai et al. Citation2014). The experiment process of CRISPR/Cas9 is easier than TALEN to apply with great efficiency, high accuracy through designing sgRNA can specifically recognize many target sites the sequence of the target gene. As a result, in the current study, the targeted mutagenesis of StSSR2 was employed using the CRISPR/Cas9 system in tetraploid potato. The results revealed that the knockout of StSSR2 by the CRISPR/Cas9 system decreased the SGAs level in leaves and tubers effectively, and reduced the relative transcript levels of genes involved in SGAs biosynthesis pathways.
Materials and methods
Sanger sequencing of StSSR2 gene in Atlantic
Genome DNA of the leaves in S. tuberosum cv. Atlantic were extracted by the DNAsecure Plant Kit (TIANGEN, Beijing, China) according to the instructions. The partial fragment of the first exon of the StSSR2 gene was obtained by polymerase chain reaction (PCR) using primers, CAS-F and CAS-R (Supplemental Table S1). The primers were designed by Primer-BLAST with the template sequence PGSC-0003DMG400021142 (https://www.ncbi.nlm.nih.gov/tools/primer-blast/). The PCR fragments were cloned into pLB-T4 DNA Ligase Vector using Lethal Based Fast Cloning Kit (TIANGEN) following the instructions. DNA sequences of the obtained 30 clones were determined using Sanger sequencing (Sangon Biotech company, Shanghai, China) and analyzed using a biological sequence alignment editor, Invitrogen-AlignX.
Construction of CRISPR/Cas9 vector
The guide RNA (gRNA) was designed based on the partial fragments of the first exon of the StSSR2 gene in S. tuberosum cv Atlantic using CRISPR-P 2.0 (http://crispr.hzau.edu.cn/CRISPR2/) (Liu et al. Citation2017). The gRNA was selected according to the predicted efficiency and no off-target site in the potato reference genome. Consequently, two candidate sequences named gRNA1 and gRNA2 were constructed into the VK005-03 vector and named as VK005-03-SSR2-g1 and VK005-03-SSR2-g2, respectively; VK005-03-SSR2-g1 was digested by AscI and SpeI enzyme, Short fragment (atU6 : 590 bp) was recovered from agarose gel, then inserted into the large fragment of VK005-03-SSR2-g2 digested by AscI and AvrII, named as VK005-StSSR2 (Figure ), the gRNA sequences were confirmed by sequencing as correct. Both gRNA1 and gRNA2 were ligated into the backbone vector VK005-03 behind the A. thaliana U6 promoter (AtU6) which is known to be suitable for expressing dicot gRNAs (Viewsolid Biotech Company, Beijing, China). The VK005-03 vector also contains a plant codon-optimized Cas9 protein driven by an Ubi promoter, expression of which could be further enhanced by the use of a 3'UTR derived from a dicot plant. The VK005-StSSR2 was introduced into A. tumefaciens strain GV3101 by heat shock.
Figure 1. Schematic representation of the CRISPR/Cas9 vector VK005-StSSR2. AtU6, Arabidopsis thaliana U6 promoter, was used to control the expression of gRNAs (gRNA1 and gRNA2) which were derived from the StSSR2 gene in Solanum tuberosum cv Atlantic. dpCas9, dicotyledonous plants Cas9, was driven by maize ubiquitine promoter (Ubi). 35S, CaMV35S promoter; Hyg, the hygromycin-resistant marker expression cassette; RB, right border of T-DNA; LB, left border of T-DNA.

Agrobacterium-mediated transformation
The vector VK005-StSSR2 was transformed into S. tuberosum cv Atlantic by A. tumefaciens-mediated transformation. Stem segments which prepared from 3- to 4-week-old seedlings were cut into pieces of ∼1 cm in length and incubated for 10 min in a culture (0.6 A600 nm) of A. tumefaciens GV3101 harboring VK005-StSSR2. Following blotting dry, the explants were placed on basal medium and incubated for 2 days in the dark. The basal medium is constituted with 4.43 g/L MS medium (M519, PhytoTechnology, lenexa, KS, USA), 30 g/L sucrose, 5 g/L agar, 0.01 mg/L α-naphthalene acetic acid (BBI Life Science, Shanghai, China) and 0.25 mg/L Zeatin (BBI Life Science), pH = 5.8. This was followed by transferring the explants to a fresh basal medium containing Cefotaxime (300 mg/L) and incubated for 2 more weeks under illumination 4000 lx at 25°C. Selection were then started by transferring the explants on basal medium containing Cefotaxime (300 mg/L) and hygromycin (10 mg/L, Diamond, Shanghai, China). When the green shoots emerging from the explant reached ∼1.5 cm in length, they were transferred onto sterile culture vessels with MS medium containing Cefotaxime (300 mg/L) and hygromycin (10 mg/L), and maintained at 25/20°C (day/night).
Positive and mutation detection of transgenic plants
The genomic DNA was extracted from wild-type S. tuberosum cv Atlantic and the T0 transformant plants using the DNAsecure Plant Kit System (Tiangen). The transgene-positive lines were identified by PCR using the primers vk005-03-F and vk005-03-R (Supplemental Table S1). The transgene-positive lines were further detected for analysis insertion/deletion (indel) by Sanger sequencing using the primers CAS-F and CAS-R (Supplemental Table S1).
The growing conditions of mutant potato plants
The mutant potato plants and wild-type Atlantic were grown in a greenhouse (Xining, China) under natural conditions from spring to autumn; the average temperature was 16–22°C.
Extraction of SGAs
We collected the leaves and tubers from greenhouse as the sample to detect the SGAs. The leaves and tubers were sampled at the period of flowering and tuber maturation, respectively. Tuber peels about 1.5 mm in thickness; tuber fleshes were sliced in the center. To ensure the same conditions, all the samples were sampled at the same time. Drying fresh samples in the oven immediately (50°C, 60 min). Then grind all samples into powder and pack them in a −80°C refrigerator for storage. Each sample to be tested is a mixture of three biological replicates.
Sample treatment: Accurate weighing sample powder of 500 mg, then added 20 ml acetic acid of 5%, ultrasonic treatment for 10 min, after that centrifuged of 10,000r/min for 5 min, filter and collect liquid supernatant, and resuspended filter residue by 10 ml acetic acid of 5%, repeated two times after filtering. Then combined liquid supernatant together, adjusted PH to ∼11 by Ammonium Hydroxide and precipitated SGAs, after that placed the alkaline solution in a 50°C water bath for 50 min and put them in 4°C refrigerator overnight. The next day, centrifuged the alkaline solution of 10,000r/min for 10 min, after that washed the precipitate twice with ammonia water of 2%, then dissolve the precipitate in methanol and fix the volume to 10 mL, shake well, filtered by the filter membrane of 0.22μm and waited for detection.
The pure chemical samples α-solanine and α-chaconine were from Sigma-Aldrich Company. α-solanine and α-chaconine were detected using Agilent 1260Inifity II-Agilent 6470 LC-MS (liquid chromato-graphy-mass spectrometry) produced by American Agilent Company. An aliquot (10μL) was analyzed by LC-MS using methanol–0.1% formic acid (9:11, v/v) as eluent at a flow rate of 0.2 mL min−1 at 30°C operated in ESI mode by Agilent Zorbax SB-C18 Narrow Bore RR,100×2.1 mm (Agilent, American). Using MRM mode calculated, the content of α-solanine and α-chaconine at m/z 868.5 and m/z 852.5, respectively. The total SGA was the sum of α-solanine and α-chaconine.
qRT–PCR
Another set of samples of leaf and tuber peel were collected for Real-Time Quantitative Reverse Transcri-ption-Polymerase Chain Reaction (qRT–PCR) analysis when the above-mentioned tissue sampling for SGAs analysis was conducted. These samples were frozen immediately in liquid nitrogen and kept at −80 °C until use. Total RNAs from leaf and tuber peel were extracted using TaKaRa MiniBEST Universal RNA Extraction Kit (TaKaRa, Beijing, China) and RNAprep Pure Plant Plus Kit (Polysaccharides & Polyphenolics-rich) (DP441) (Tiangen), respectively, according to the protocols. Reverse transcription reactions were performed using PrimeScript™ RT Master Mix (Perfect Real Time) (TaKaRa) and TB Green® Premix Ex Taq™ (Tli RNaseH Plus) (TaKaRa). At least three biological replicates were conducted for each sample. The result value of each sample was normalized to the level of ef1a (AB061263) as the reference gene and used to calculate the average relative expression level of a gene in the transformed line. qRT–PCR primers we used are all listed in Supplementary Table S1.
Statistical analysis
All the data were all at least three biological replicates and shown as mean ± SE. The significance of difference for SGAs concentration was analyzed by Dunnett’s test (P < .05) using SPSS 18.0. The significance of difference for qRT-PCR was analyzed by Student’s t-test (P < .05).
Results
Analysis of the target sequence of StSSR2 in Atlantic
In potato, the StSSR2 protein is predicted to have a flavin adenine dinucleotide binding domain and a transmembrane region, encoded by a single gene that contains two exons (Sawai et al. Citation2014). The loci of interest were sequenced in the cultivar Atlantic to assess inter-allelic polymorphism prior to the selection of target sequences (Figure and Supplementary Figure S3). The locus of StSSR2 in Atlantic had two subgenome types, StSSR2-Atlantic (A) and StSSR2-Atlantic (B) (Supplementary Figure S3). Seven out of 30 single copies in DNA were StSSR2-Atlantic (A) (23.3%) and the rest single copies were StSSR2-Atlantic (B) (76.7%). The ratio between StSSR2-Atlantic (A) and StSSR2-Atlantic (B) was ∼1:3. The contribution of each StSSR2 allele in SGA biosynthesis was unclear. How it is working could be further determined by the separation ratio of self-crossing. The gRNA targets for StSSR2 were designed based on the conserved domains as elucidated in Figure . There were no allelic differences among all the sequenced StSSR2 fragments in both gRNA1 and gRNA2.
Highly efficient knockout of StSSR2 gene using Agrobacterium-based transformation
To create insertion/deletion (indels) at the targeted gRNA1 and gRNA2 using Agrobacterium-mediated transformation was we wanted. We applied the two gRNAs simultaneously on the same DNA strand which naturally spaced 33 bp apart in order to improve editing efficiency and arise larger deletions. 144 transgenic plantlets of hygromycin-resistant were gotten and the DNA of leaves were extracted. 140 out of 144 Atlantic transgenic plantlets were found to harbor the introduced VK005-StSSR2 vector, resulting in a 97% positive probability, as revealed by PCR amplification using the primers vk005-03-F and vk005-03-R (Supplementary Table S1). Then PCR amplicons by primers CAS-F and CAS-R were sequenced to obtain the SSR2 sequences in each transgenic line. Mutations were identified in 64 out of 140 transgenic plantlets, resulting in a 46% editing efficiency at the gRNA1 and gRNA2 targets.
Most deletions were detected at the StSSR2 gene matching the gRNA2 adjacent to the PAM site, whereas the deletions at gRNA1 were relatively less frequent (4.7%) (Supplementary Figures S1 and S2). The majority of the mutations consisted of small deletions of between 3 and 12 nucleotides, among which deletions of 3 bp were the most commonly observed. In contrast, four mutated lines were confirmed to have one or two nucleotide insertions and one line showed a 53 bp deletion (Supplementary Figure S2).
Deletions and insertions were all emerged in the StSSR2 targeted site and a few nucleotides upstream of the PAM site (Supplementary Figure S2). Similar to the previous report of StSSR2 editing using TALEN (Sawai et al. Citation2014), indels of different sizes at all four homologous chromosomes also could generated in the genome of tetraploid potato using CRISPR–Cas9 system (Supplementary Figure S4). As in this study, the proportion of the two subgenome types StSSR2-Atlantic (A) and StSSR2-Atlantic (B) was 1:3 approximately. The majority (62 out of 64) of the subgenome type in mutated lines were StSSR2-Atlantic (B), this outcome is consistent with the proportion of the two subgenomes above.
Concentrations of SGAs in the mutated potato plants
The predominant SGAs levels of 6 mutated lines (Figure ) were detected according to the mutation type and genome type. Five of the six mutated lines were StSSR2-Atlantic (B), and one (#360) was StSSR2-Atlantic (A) (Supplementary Figure S4). SGAs analysis was performed with leaves, tuber peels and tuber fleshes of greenhouse-grown plants, together with WT plants. In the tuber peels of these mutated lines, a significant reduction of SGAs (Figure (A)) was observed in four of the six mutated lines, with the lowest amount (#360 in Figure (A)) being 0.18 mg g−1 FW, which was about 54% of that in WT (Figure (A)). It is unknown what caused the #96 and #110 SGAs contents to be higher than the WT. It is possible that SSR2 was activated by the mutation and the varying levels of SGAs in genome-edited plants with intact StSSR2 sequences are caused by their mutation pattern. In the tuber fleshes of these mutated lines, significant reduction of SGAs (Figure (B)) was found in only one of the six mutated lines, being 0.018 mg g−1 FW, which (#378 in Figure (B)) was about 66% of that in WT (Figure (B)). In the leaves of these mutated lines, significant reduction of SGAs (Figure (C)) showed in all six lines, with the lowest amount (#204 and #378 in Figure (C)) being 0.57 mg g−1 FW, which was about 44% of that in WT (Figure (C)). All the six mutated lines showed normal growth and tuber yield which were not significantly different from WT (Figure (D)).
Analyses of the relative transcript levels of genes in SGA and phytosterol pathways
We chose two CRISPR transformed lines (#360, #378 in Figures and ) to evaluate the relative transcript levels of StSSR2 and a set of other key genes associated with SGAs and phytosterol biosynthetic pathways in leaves and tuber peels of potato. As expected, the expression of StSSR2 in both transgenic lines was significantly decreased in leaves relative to WT. The expression levels of StHMG1, StSQS1 and StCAS that participated in the biosynthesis of phytosterol and SGAs pathways were also reduced in the leaves of these two CRISPR transformed lines (Figure ). At the same time, the expression levels of StSMT1, StCYP51G and StSSR1 participated in the biosynthesis of phytosterol pathways were not changed significantly. In contrast, transcript levels of StGAME8a, StGAME4 and StSGT1 related to the biosynthesis of SGAs were downregulated significantly in all the two CRISPR transformed lines. Overall, the knockout of StSSR2 by CRISPR/Cas9 in leaves resulted in a decreased abundance of transcripts of SGA-related genes and SGAs contents.
Figure 4. The mutation potato plants #360, #378, #171, #204, #110 and #96. (A) α-solanine, α-chaconine and total SGAs in the tuber peels of StSSR2-knocked out mutation plants. (B) α-solanine, α-chaconine and total SGAs in the tuber fleshes of StSSR2-knocked out mutation plants. (C) α-solanine, α-chaconine and total SGAs in the leaves of StSSR2-knocked out mutation plants. (D) The tuber yield of StSSR2-knocked out mutation plants. FW, fresh weigh; WT, wild-type.
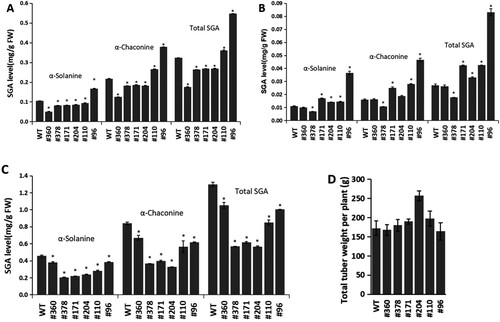
Figure 5. The mutated type of potato plants with reduced SGAs. The nucleotide sequences of #360 and #378, compared to wild-type (WT). The number of deleted and inserted nucleotide sequences is on the right.
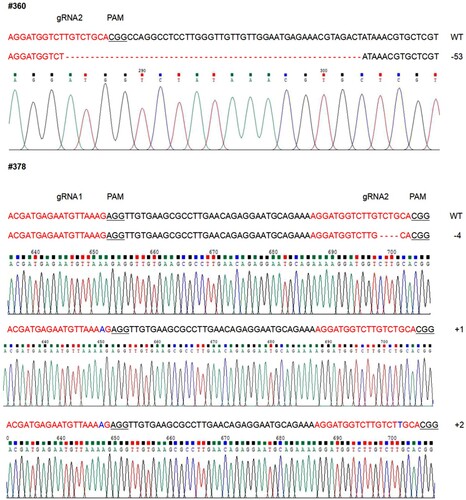
Similarly, StSSR2 expression was significantly decreased relative to WT in all the tested transgenic lines in the tuber peels. StHMG1 transcript abundance was below detection level in tuber peels of both mutated lines and WT. Figure (A) showed that the total SGAs of #360 in tuber peels was the lowest, as a result, the relative transcription levels compared to WT of different genes in #360 were analyzed. The transcription level of all the genes in the SGAs and phytosterol synthesis pathways we detected were reduced in #360. The transcript levels of StSQS1 upstream of mevalonate pathway reduced significantly compared to WT, the expression levels of StSMT1 and StCYP51G in phytosterol synthesis pathways were downregulated significantly. The results showed that the gene transcription levels we detected of SGAs and phytosterol synthesis pathway in tuber peels were all affected, and decreased to varying degrees. Overall, the knockout of StSSR2 by CRISPR/Cas9 also reduced the abundance of SGA-related transcripts and SGAs contents in tuber peels.
Discussion
The CRISPR/Cas9 editing of potato StSSR2 has not only significantly reduced the SGA content, but also modulated the entire SGAs biosynthesis pathway. Leaves and tuber peels of CRISPR/Cas9 transformed potatoes exhibited significantly reduced expression of StSSR2 and as a result, the expression of StSQS1, StGAME8a, StGAME4 and StSGT1 were all reduced. GAME4 is essential in SGAs biosynthesis for potato, silencing StGAME4 gene resulted in the reductions of SGAs concentrations in tubers of potato (Itkin et al. Citation2013). SMT1 is also critical in sterol metabolism, as the over-expression of a soybean SMT1 in potato plants decrease in the accumulation of cholesterol and total SGAs (Arnqvist et al. Citation2003). However, GAME9 over-expression in potato up-regulated the expression of SGA-related gene including StSSR2, StGAME4 whereas the expression of StSMT1 was not significantly altered (Cárdenas et al. Citation2016). Over-expression of an Arabidopsis thaliana LAS (AtLAS) in potato leaves resulted in the increment in SGAs accumulation, levels of SGA-related genes StSSR2 and StGAME4 were up-regulated significantly with the expression levels of StSMT1 didn’t alter (Kumar et al. Citation2017). In our study, the expression of StSMT1 also wasn’t significantly affected but the expression of the gene in SGAs biosynthesis was decreased, hence indicating that CRISPR/Cas9 editing of SSR2 resulted in the downregulation of SGAs biosynthesis in potato leaves and tuber peels.
gRNA is important for the editing efficiency of the CRISPR/Cas9 system. As we all know, it is difficult to induce site-directed mutagenesis in polyploid crops, for example, in tetraploid potato is more complicated than in diploid crops, it requires high level of editing efficiency (Nakayasu et al. Citation2018). In rice, the CRISPR/Cas9 multiplex gene editing system involving more than one gRNA was found to enhance mutagenesis efficiency (Xie et al. Citation2015). We had employed a dual gRNA CRISPR/Cas9 vector, two gRNA genes were introduced into the CRISPR/Cas9 vectors in our study. As a result, 64 of 140 (46%) transgenic plantlets were found to harbor mutations. Interestingly, most deletions (97%) were detected at gRNA2 adjacent to the PAM site (Supplementary Figure S2). It illustrated that the mutagenesis efficiency of gRNA2 was apparently higher than gRNA1 and the two-guide strategy of CRISPR/Cas9 system by A. tumefaciens-mediated transformation may have enhanced the targeted mutation efficacy in transgenic potato. Further, the editing efficiency was improved by optimizing the promoters that was used to drive the Cas9 and gRNA could also be highly relevant to the high rate of targeted mutagenesis (Du et al. Citation2016; Long et al. Citation2018). A recent study also pointed out that the incubation temperature for the infected explants during Agrobacterium transformation may also improve editing efficiency (Liu et al. Citation2020).
In our study, the SGAs content of mutated potato reduced significantly compared to WT. In the peels of these mutated lines, the lowest SGAs level was reduced to 54% (#360) relative to WT. In the tuber fleshes, SGAs level in line #378 was reduced to 66% of WT. In leaves, the lowest SGAs level was recorded as 44% of the WT level. The reduction of SGAs level in potato observed in this study is comparable to previous reports. For example, the SGAs levels in leaves and tubers were reduced by ∼50% and 52% in asDWF1-L (SSR2) transformants, respectively (Nahar et al. Citation2017). On the other hand, StSSR2 silenced by RNAi and knocked out by TALEN resulted in significantly reductions of predominant SGAs contents to ∼10% of the nontransformant in stems, tuber peels and leaves of potato plants, respectively (Sawai et al. Citation2014). The SGAs levels reduced more than our data. Wild-type sequence was observed and the mutations may be mosaic in our study. The subgenome of mutated sequence in six mutations were just one type which illustrated that mutations did not arise at all four homologous chromosomes. These might be the two main reasons. Furthermore, StSSR1 protein had the weak D24(25) reduction activity toward cycloartenol or desmosterol, leading to the accumulation of SGAs in StSSR2-disrupted plant (Sawai et al. Citation2014). A slight change in transcript levels of StSRR1 was observed in the two mutated lines relative to WT in the present study (Figures and ). Moreover, compared to the WT, SGAs levels were lower both in leaves and tubers from asDWF1 (SSR1) transformants in potato (Nahar et al. Citation2017). These illustrated that the StSSR1 protein had a relatively minor role in accumulating SGAs in potato.
Figure 6. The relative transcript levels of genes related to the biosynthesis of SGAs and phytosterol in leaves of StSSR2-CRISPR transformed potato plants. 1, #360; 2, #378.
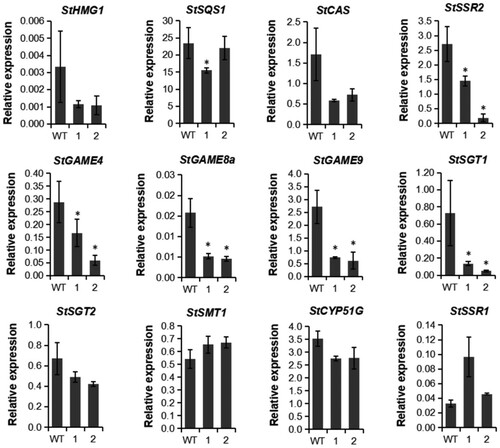
Figure 7. The relative transcript levels of genes related to the biosynthesis of SGAs and phytosterol in tuber peels of StSSR2-CRISPR transformed potato plants. 1, #360; 2, #378.
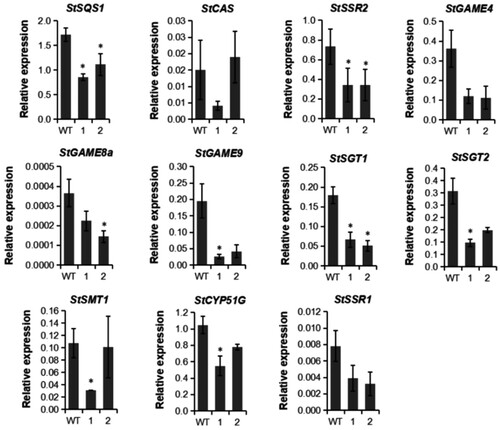
The high frequency of mosaic usually occurs in primary mutated plants through Agrobacterium-mediated transformation (Fauser et al. Citation2014; Pan et al. Citation2016; Peng et al. Citation2017). Agrobacterium-mediated gene editing is related to the integration of the Cas9-harboring transgene and protoplast transformation can produce non-transgenic plants (Veillet et al. Citation2019a, Citation2019b). To obtain effective transgene-free with non-mosaic and all four alleles mutated edited desirable potato, the next step is to use self-crossing homozygosity to remove foreign genes, or to acquire effective editing potato without foreign genes by protoplast method. Recently, Yasumoto et al. developed a new method infected potato with A. tumefaciens harboring TALEN-expression vector targeting StSSR2 gene and regenerated shoots without antibiotic selection and obtained regenerated lines with disrupted-StSSR2 gene and without transgene of the TALEN gene, revealing that gene disruption should be caused by transient gene expression (Yasumoto et al. Citation2020). There is no doubt that further studies leading to the improvements on the efficiency of gene editing and generation of transgene-free potato is warranted.
Conclusions
The study demonstrated that the StSSR2 gene could be successfully knockout by CRISPR/Cas9 in tetraploid cultivated potato and reduced the content of SGAs. Reduce SGAs level is not only benefit to ensure the palatability and edible safety of potato products, but also useful to develop the commercial use of the above-ground portion. Due to the international restrictions on genetically modified (GM) crops, it is necessary to achieve the edited desirable potato which is all four alleles mutated without transgene. Next, foreign genes are removed through self-crossing, or editing potato without foreign genes by protoplast method. Cytidine Base Editor and Geminivirus mediated genome editing by Agrobacterium-mediated transformation also helpful to get the transgene-free potato.
Supplemental Material
Download MS Word (8.7 MB)Disclosure statement
No potential conflict of interest was reported by the author(s).
Data availability statement
The data that support the findings of this study are available in the figshare repository (https://figshare.com/) at https://figshare.com/s/790ef87161f5d84bf-cad.
Additional information
Funding
References
- Andersson M, Turesson H, Nicolia A, Fält AS, Samuelsson M, Hofvander P. 2017. Efficient targeted multiallelic mutagenesis in tetraploid potato (Solanum tuberosum) by transient CRISPR-Cas9 expression in protoplasts. Plant Cell Rep. 36:117–128.
- Andersson M, Turesson H, Olsson N, Fält AS, Ohlsson P, Gonzalez MN, Samuelsson M, Hofvander P. 2018. Genome editing in potato via CRISPR-Cas9 ribonucleoprotein delivery. Physiol Plant. 164:378–384.
- Arnqvist L, Dutta PC, Jonsson L, Sitbon F. 2003. Reduction of cholesterol and glycoalkaloid levels in transgenic potato plants by overexpression of a type 1 sterol methyltransferase cDNA. Plant Physiol. 131:1792–1799.
- Austin S, Lojkowska E, Ehlenfeldt MK, Kelman A, Helgeson JP. 1988. Fertile interspecific somatic hybrids of solanum: a novel source of resistance to Erwinia soft Rot. Phytopathology. 78:1216–1220.
- Bejarano L, Mignolet E, Devaux A, Espinola N, Carrasco E, Larondelle Y. 2000. Glycoalkaloids in potato tubers: the effect of variety and drought stress on the α-solanine and α-chaconine contents of potatoes. J Sci Food Agric. 80:2096–2100.
- Bergenstråhle A, Borga P, Jonsson MV. 1996. Sterol composition and synthesis in potato tuber discs in relation to glycoalkaloid synthesis. Phytochemistry. 41:155–161.
- Bergenstråhle A, Tillberg E, Jonsson L. 1992. Regulation of glycoalkaloid accumulation in potato tuber discs. J Plant Physiol. 140:269–275.
- Butler NM, Atkins PA, Voytas DF, Douches DS. 2015. Generation and Inheritance of Targeted Mutations in Potato (Solanum tuberosum L.) Using the CRISPR/Cas system. Plos One. 10:e0144591.
- Butler NM, Baltes NJ, Voytas DF, Douches DS. 2016. Geminivirus-mediated genome editing in potato (Solanum tuberosum L.) using sequence-specific nucleases. Front Plant Sci. 7:1045.
- Cárdenas PD, Sonawane PD, Pollier J, Vanden Bossche R, Dewangan V, Weithorn E, Tal L, Meir S, Rogachev I, Malitsky S, et al. 2016. GAME9 regulates the biosynthesis of steroidal alkaloids and upstream isoprenoids in the plant mevalonate pathway. Nat Commun. 7:10654.
- Choi D, Bostock RM, Avdiushko S, Hildebrand DF. 1994. Lipid-derived signals that discriminate wound- and pathogen-responsive isoprenoid pathways in plants: methyl jasmonate and the fungal elicitor arachidonic acid induce different 3-hydroxy-3-methylglutaryl-coenzyme A reductase genes and antimicrobial isoprenoids in Solanum tuberosum L. Proc Natl Acad Sci USA. 91:2329–2333.
- Dale MFB, GriYths DW, Bain H, Todd D. 1993. Glycoalkaloid increase in Solarium tuberosum on exposure to light. Ann Appl Biol. 123:411–418.
- Diener AC, Li H, Zhou W, Whoriskey WJ, Nes WD, Fink GR. 2000. Sterol methyltransferase 1 controls the level of cholesterol in plants. Plant Cell. 12:853–870.
- Du H, Zeng X, Zhao M, Cui X, Wang Q, Yang H, Cheng H, Yu D. 2016. Efficient targeted mutagenesis in soybean by TALENs and CRISPR/Cas9. J Biotechnol. 217:90–97.
- Fauser F, Schiml S, Puchta H. 2014. Both CRISPR/Cas-based nucleases and nickases can be used efficiently for genome engineering in Arabidopsis thaliana. Plant J. 79:348–359.
- Fewell AM, Roddick JG. 1997. Potato glycoalkaloid impairment of fungal development. Mycol Res. 101:597–603.
- Griffiths DW, Dale MFB. 2001. Effect of light exposure on the glycoalkaloid content of Solanum phureja tubers. J Agric Food Chem. 49:5223–5227.
- Hajslova J, Schulzova V, Slanina P, Janne K, Hellenas KE, Andersson C. 2005. Quality of organically and conventionally grown potatoes: four-year study of micronutrients, metals, secondary metabolites, enzymic browning and organoleptic properties. Food Addit Contam. 22:514–534.
- Hameed A, Zaidi SS, Shakir S, Mansoor S. 2018. Applications of New Breeding Technologies for Potato Improvement. Front Plant Sci. 9:925.
- Heftmann E. 1983. Biogenesis of steroids in solanaceae. Phytochemistry. 22:1843–1860.
- Itkin M, Heinig U, Tzfadia O, Bhide AJ, Shinde B, Cardenas PD, Bocobza SE, Unger T, Malitsky S, Finkers R, et al. 2013. Biosynthesis of antinutritional alkaloids in solanaceous crops is mediated by clustered genes. Science. 341:175–179.
- Karkute SG, Singh AK, Gupta OP, Singh PM, Singh B. 2017. CRISPR/Cas9 Mediated Genome Engineering for Improvement of Horticultural Crops. Front Plant Sci. 8:1635.
- Kozukue N, Mizuno S. 1990. Effects of light exposure and storage temperature on greening and glycoalkaloid content in potato tubers. J Jpn Soc Hortic Sci. 59:673–677.
- Krits P, Fogelman E, Ginzberg I. 2007. Potato steroidal glycoalkaloid levels and the expression of key isoprenoid metabolic genes. Planta. 227:143–150.
- Kumar A, Fogelman E, Weissberg M, Tanami Z, Veilleux RE, Ginzberg I. 2017. Lanosterol synthase-like is involved with differential accumulation of steroidal glycoalkaloids in potato. Planta. 246:1189–1202.
- Kusano H, Ohnuma M, Mutsuro-Aoki H, Asahi T, Ichinosawa D, Onodera H, Asano K, Noda T, Horie T, Fukumoto K, et al. 2018. Establishment of a modified CRISPR/Cas9 system with increased mutagenesis frequency using the translational enhancer dMac3 and multiple guide RNAs in potato. Sci Rep. 8:13753.
- Lafta AM, Lorenzen JH. 2000. Influence of high temperature and reduced irradiance on glycoalkaloid levels in potato leaves. J Am Soc Hortic Sci. 125:563–566.
- Liu H, Ding Y, Zhou Y, Jin W, Xie K, Chen LL. 2017. CRISPR-P 2.0: an improved CRISPR/Cas9 tool for genome editing in plants. Mol Plant. 10:530–532.
- Liu CX, Gui HP, Wen Q, Liu YB, Gao Y, Zhang XJ, Cui RJ, Xu JP. 2020. CRISPR/cas9 genome editing system optimization in tomato. Mol Plant Breed. 18:6716–6724.
- Long L, Guo DD, Gao W, Yang WW, Hou LP, Ma XN, Miao YC, Botella JR, Song CP. 2018. Optimization of CRISPR/Cas9 genome editing in cotton by improved sgRNA expression. Plant Methods. 14:85.
- McCue KF, Allen PV, Shepherd LVT, Blake A, Maccree MM, Rockhold DR, Novy RG, Stewart D, Davies HV, Belknap WR. 2007. Potato glycosterol rhamnosyltransferase, the terminal step in triose side-chain biosynthesis. Phytochemistry. 68:327–334.
- McCue KF, Shepherd LVT, Allen PV, Maccree MM, Rockhold DR, Corsini DL, Davies HV, Belknap WR. 2005. Metabolic compensation of steroidal glycoalkaloid biosynthesis in transgenic potato tubers: using reverse genetics to confirm the in vivo enzyme function of a steroidal alkaloid galactosyltransferase. Plant Sci. 168:267–273.
- Moehs CP, Allen PV, Friedman M, Belknap WR. 1997. Cloning and expression of solanidine UDP-glucose glucosyltransferase from potato. Plant J. 11:227–236.
- Muthoni J, Kabira J, Shimelis H, Melis R. 2015. Tetrasomic inheritance in cultivated potato and implications in conventional breeding. Aust J Crop Sci. 9:185–190.
- Nahar N, Westerberg E, Arif U, Huchelmann A, Guasca AO, Beste L, Dalman K, Dutta PC, Jonsson L, Sitbon F. 2017. Transcript profiling of two potato cultivars during glycoalkaloid-inducing treatments shows differential expression of genes in sterol and glycoalkaloid metabolism. Sci Rep. 7:43268.
- Nakayasu M, Akiyama R, Lee HJ, Osakabe K, Osakabe Y, Watanabe B, Sugimoto Y, Umemoto N, Saito K, Muranaka T, Mizutani M. 2018. Generation of α-solanine-free hairy roots of potato by CRISPR/Cas9 mediated genome editing of the St16DOX gene. Plant Physiol Biochem. 131:70–77.
- Nes WD. 2011. Biosynthesis of cholesterol and other sterols. Chem Rev. 111:6423–6451.
- Ohyama K, Okawa A, Moriuchi Y, Fujimoto Y. 2013. Biosynthesis of steroidal alkaloids in Solanaceae plants: Involvement of an aldehyde intermediate during C-26 amination. Phytochemistry. 89:26–31.
- Pan C, Ye L, Qin L, Liu X, He Y, Wang J, Chen L, Lu G. 2016. CRISPR/Cas9-mediated efficient and heritable targeted mutagenesis in tomato plants in the first and later generations. Sci Rep. 6:24765.
- Pehu E, Gibson RW, Jones MGK, Karp A. 1990. Studies on the genetic basis of resistance to potato leaf roll virus, potato virus Y and potato virus X in Solanum brevidens using somatic hybrids of Solanum brevidens and Solanum tuberosum. Plant Sci. 69:95–101.
- Peng A, Chen S, Lei T, Xu L, He Y, Wu L, Yao L, Zou X. 2017. Engineering canker-resistant plants through CRISPR/Cas9-targeted editing of the susceptibility gene CsLOB1 promoter in citrus. Plant Biotechnol J. 15:1509–1519.
- Percival G, Dixon G, Sword A. 1994. Glycoalkaloid concentration of potato tubers following continuous illumination. J Sci Food Agric. 66:139–144.
- Percival GC, Karim MS, Dixon GR. 1998. Influence of light-enhanced glycoalkaloids on resistance of potato tubers to Fusarium sulphureum and Fusarium solani var. coeruleum. Plant Pathol. 47:665–670.
- Petersson EV, Nahar N, Dahlin P, Broberg A, Tröger R, Dutta PC, Jonsson L, Sitbon F. 2013. Conversion of exogenous cholesterol into glycoalkaloids in potato shoots, using two methods for sterol solubilisation. PLoS One. 8:e82955.
- Rahier A. 2011. Dissecting the sterol C-4 demethylation process in higher plants. From structures and genes to catalytic mechanism. Steroids. 76:340–352.
- Rokka VM, Xu YS, Kankila J, Kuusela A, Pulli S, Pehu E. 1994. Identification of somatic hybrids of dihaploid Solanum tuberosum lines and S. brevidens by species specific RAPD patterns and assessment of disease resistance of the hybrids. Euphytica. 80:207.
- Sanford LL, Deahl KL, Sinden SL, Ladd TL. 1992. Glycoalkaloid contents in tubers from Solanum tuberosum populations selected for potato leafhopper resistance. Am Potato J. 69:693–703.
- Sanford LL, Kobayashi RS, Deahl KL, Sinden SL. 1997. Diploid and tetraploid Solanum chacoense genotypes that synthesize leptine glycoalkaloids and deter feeding by Colorado potato beetle. Am Potato J. 74:15–21.
- Sawai S, Ohyama K, Yasumoto S, Seki H, Sakuma T, Yamamoto T, Takebayashi Y, Kojima M, Sakakibara H, Aoki T, et al. 2014. Sterol side chain reductase 2 is a Key enzyme in the biosynthesis of cholesterol, the common precursor of toxic steroidal glycoalkaloids in potato . Plant Cell. 26:3763–3774.
- Sepelev I, Galoburda R. 2015. Industrial potato peel waste application in food production: a review. Res Rural Dev. 1:130–136.
- Smith DB, Roddick JG, Jones JL. 1996. Potato glycoalkaloids: some unanswered questions. Trends Food Sci Technol. 7:126–131.
- Stearns LD, Petry TA, Krause MA. 1994. Potential food and nonfood utilization of potatoes and related byproducts in North Dakota. Agricultural Economics Report Number 322. p. 2–6.
- Suzuki M, Muranaka T. 2007. Molecular genetics of plant sterol backbone synthesis. Lipids. 42:47–54.
- Thagun C, Imanishi S, Kudo T, Nakabayashi R, Ohyama K, Mori T, Kawamoto K, Nakamura Y, Katayama M, Nonaka S, et al. 2016. Jasmonate-responsive ERF transcription factors regulate steroidal glycoalkaloid biosynthesis in tomato. Plant Cell Physiol. 57:961–975.
- Valkonen JPT, Keskitalo M, Vasara T, Pietila L. 1996. Potato glycoalkaloids: a burden or a blessing? Crit Rev Plant Sci. 15:1–20.
- Veillet F, Chauvin L, Kermarrec MP, Sevestre F, Merrer M, Terret Z, Szydlowski N, Devaux P, Gallois JL, Chauvin JE. 2019a. The Solanum tuberosum GBSSI gene: a target for assessing gene and base editing in tetraploid potato. Plant Cell Rep. 38:1065–1080.
- Veillet F, Perrot L, Chauvin L, Kermarrec MP, Guyon-Debast A, Chauvin JE, Nogué F, Mazier M. 2019b. Transgene-Free Genome Editing in Tomato and Potato Plants Using Agrobacterium-Mediated Delivery of a CRISPR/Cas9 Cytidine Base Editor. Int J Mol Sci. 20:402.
- Wang S, Zhang S, Wang W, Xiong X, Meng F, Cui X. 2015. Efficient targeted mutagenesis in potato by the CRISPR/Cas9 system. Plant Cell Rep. 34:1473–1476.
- Xie K, Minkenberg B, Yang YY. 2015. Boosting CRISPR/Cas9 multiplex editing capability with the endogenous tRNA-processing system. Proc Natl Acad Sci USA. 112:3570–3575.
- Yasumoto S, Sawai S, Lee HJ, Mizutani M, Saito K, Umemoto N, Muranaka T. 2020. Targeted genome editing in tetraploid potato through transient TALEN expression by Agrobacterium infection. Plant Biotechnol. 37:205–211.
- Zhou X, Zha M, Huang J, Li L, Imran M, Zhang C. 2017. StMYB44 negatively regulates phosphate transport by suppressing expression of PHOSPHATE1 in potato. J Exp Bot. 68:1265–1281.