Abstract
Low molecular weight organic acids (LMWOAs) are common and abundant compounds in the environment, responsible for the release of the nutrients from rhizosphere into the soil solution and, therefore, facilitating the availability of these nutrients for plants. Sewage sludge biochar (SSB) is considered to be a potential source of phosphorus (P) in soil. In this context, the efficacy of twelve LMWOAs in releasing SSB-bearing P was tested in a laboratory extraction experiment. Moreover, the potential effects of (i) biochar pyrolysis temperature (varying from 220°C to 620°C) and (ii) other elements in SS, to estimate the P bonds attacked predominantly by the LMWOAs, were also assessed. The results revealed citric acid was the most effective extraction agent enhancing the mobility of the SSB-derived P. P release was strongly affected by biochar pyrolysis temperature (the highest P release was from the 320°C biochar, significant at p < 0.05). Moreover, the results showed that P was released preferably from Fe-P bearing compounds in SS. Citric acid was recorded as less effective at releasing other nutrients, such as Ca, Mg, K, indicating the exceptional performance of citric acid in P release from biochar without substantial effect on the other nutrients.
Introduction
Phosphorus belongs among the most important nutrients for plant growth but 30–40% of arable soils are low in phosphorus (P), all over the world (Kirkby and Johnston Citation2008). Low P availability is one of the primary limiting factors for crop production in soils, which is attributed to its low solubility, chemical fixation, and complex chelation (Li et al. Citation2016). To provide plants with a balanced nutrient status inorganic (Pi) fertilizers are applied, where the P compounds are mainly derived from non-renewable resources, i.e. phosphate rocks, which, based on current production rates of P fertilizers, will only last for the next 300–400 years (Glaser and Lehr Citation2019). However, P-rich waste materials can be used as a potential P source for plants. For instance, sewage sludge (SS) contains high amount of P (14%) (Qian et al. Citation2019). The predominant position of sewage sludge within the P rich wastes documents that 35% of P wasted from consumption sector is wasted through sewage sludge (Van Dijk Citation2016).
In this context, the potential risk of SS application to the soil from the enhanced contents of risk elements such as various organic contaminants including pharmaceuticals and personal care products. Jones et al. (Citation2014) surveyed 28 wastewater treatment works in Great Britain for over a period of 12 months and contaminants such as trace metals, pharmaceuticals, polycyclic aromatic hydrocarbons (PAHs), ‘emerging’ and regulated organic pollutants were detected in at least some of the sludges sampled. Many investigations documented that the regular long-term application of SS can result in the regular increase of hardly degradable organic pollutants in soils (Singh and Agrawal Citation2008; Pulkrabová et al. Citation2019). Major limitation of the SS use in agriculture is a potential release of heavy metals from the SS and their accumulation to toxic levels in the topsoil (Hanč et al. Citation2007). In the Czech Republic, the required parameters of sewage sludge suitable for landfill application are given by the public notice of the Ministry of the Environment No. 437/2016. According to these regulations, the maximum allowable contents of the risk elements in SS are 30 mg/kg of As, 5 mg/kg of Cd, 200 mg/kg of Cr, 500 mg/kg of Cu, 100 mg/kg of Ni, 200 mg/kg of Pb, 2500 mg/kg of Zn in SS dry matter. In order to suppress the potential harmful effects of SS in the soil, the pyrolytic thermal treatment of this material resulting in biochar has been widely investigated. Biochar is a carbon-rich solid product of the thermochemical conversion of biomass residues in conditions of minimal or zero oxygen supply, and most of the P in SS can be concentrated in biochar (Qian et al. Citation2019).
Many previous studies have documented the role of organic acids in P solubilization in the soil. Generally, an acidic environment can significantly enhance the solubility of P minerals (Chen et al. Citation2006; Li et al. Citation2016; Wang et al. Citation2018). Soil organic acids (OAs) can be broadly classified into two groups, namely high molecular weight OAs (HMWOAs) and low molecular weight OAs (LMWOAs). The HMWOAs are characterized by their high molecular weight and low solubility in water whereas the LMWOAs (molecular weight ranging from 46 DA to a few 100 DA) are present in the soil solution (Adeleke et al. Citation2017). Generally, the major sources of organic acids in the soil are plant root exudations and decomposing microbial as well organic matter (Adeleke et al. Citation2017). It has been demonstrated that some dicotyledonous plant roots, and especially non-mycorrhizal plants such as Lupinus albus (but not L. angustifolia) and Brassica napus, are capable of releasing large amounts of organic acids into the rhizosphere in response to P deficiency, while other dicotyledonous (e.g. Sisymbrium officinale) and graminaceous (wheat, maize) plants do not appear to express the trait (Jones Citation1998).
Among microorganisms, bacteria, fungi, and lichen species produce significant amounts of soil OAs. Similarly, decomposed organic matter also contributes to high concentrations of OAs that enrich the soil environment (Adeleke et al. Citation2017). Chen et al. (Citation2006) stated that phosphate-solubilizing bacteria P release LMWOAs to solubilize phosphorus. Jones (Citation1998) also reported that rhizospheric bacteria and plant roots release large amounts of organic acid, especially in nutrient-limited conditions, for example, malate and citrate appear to be the primary components released by roots under P deficiency. In addition, the co-incubation of biochar and phosphate-solubilizing bacteria can promote the degradation of organic contaminants (for instance the herbicide atrazine), where biochar increases the total and bioavailable P content of the soil (Tao et al. Citation2020). Carboxyl and hydroxyl anions of organic acids chelate cations or reduce the pH to release P (Alori et al. Citation2017). These organic acid anions can therefore solubilize P from mineral surfaces either by ligand exchange or by ligand-promoted dissolution (Oburger et al. Citation2011). In the case of biochars, the effect of LMWOAs on the release of P is dominated by the molecular structure of LMWOAs, the dissociation degree of LMWOAs, and the mineral element stoichiometric ratio of biochars (Zhang et al. Citation2020). However, the informations concerning the role of LMWOAs in the release of P from biochars are still limited.
This study describes the P solubilizing efficiency of twelve different organic acids (acetic, quinic, benzoic, oxalic, mucic, malic, succinic, maleic, phthalic, trans-aconitic, citric, and kojic acid) from sewage sludge biochar (SSB) produced at different pyrolysis temperatures. The ability of LMWOAs to release P from SSB has an impact on the potential enrichment of the soil with microorganisms containing these acids predominantly in their exudates. A laboratory experiment was conducted to: (i) evaluate the availability of SSB-bearing P as related to both pyrolysis conditions and particular organic acids, and, (ii) recommend the most effective organic acids for further choice of the rhizospheric organisms facilitating the bioaccessibility of the biochar-based P. Therefore, the main objective of the study was to identify the most effective organic acids able to release the biochar-based P for the enhancement of the soil P status via a short-term extraction experiment. In this context, the microorganisms dominating in the exudation of the most effective P-releasing acids could be selected for the further experiments.
Materials and methods
Biochar preparation
SS was sampled from a wastewater treatment plant (WWTP) in the Czech Republic with a design capacity of 29,000 population equivalent (PE) where Fe2(SO4)3 is used for P precipitation. The SS used was anaerobically stabilized (mesophilic anaerobic digestion) and dewatered using a decanter centrifuge (dry matter content 24 wt%). After collection, the sample was air-dried in thin layers at 105°C to constant mass. The dried SS was milled and passed through a 1 mm stainless sieve before thermal treatment. The biochar preparation process is described by Mercl et al. (Citation2020). Briefly: the thermal treatment of the dried SS was performed in an inert atmosphere of N2 (99.99%) using an electric laboratory tube furnace (GHA 12/600, Carbolite Gero Ltd., Hope Valley, United Kingdom). The torefaction temperatures were: 220°C, and 320°C, the pyrolysis temperatures were 420°C, 520°C, and 620°C. In addition, non-pyrolyzed SS, dried at 105°C, was included in the experimental design. The detailed chemical composition and physicochemical characteristics of the biochars were assessed by Mercl et al. (Citation2020). The contents of risk elements, as well as the emerging organic contaminants in the SS, as well as their changes with increasing pyrolysis temperature were summarized by Mercl et al. (Citation2021) in the Supplementary tables. These materials document that the risk element contents in the SS used in this study was far below the limits given by the Public notice No, 437/2016.
Experimental design and analytical procedure
Three monocarboxylic acids (acetic, quinic, benzoic), six dicarboxylic acids (oxalic, mucic, malic, succinic, maleic, phthalic), two tricarboxylic acids (trans-aconitic, citric), and one representing the enols, kojic acid, were used for P extraction. The main characteristics of the individual organic acids with commercial reagent grade are summarized in Table . The appropriate amount of each acid was dissolved in deionized water to make 1 mM solution, and pH of the solution was immediately determined using a Sentron SI400 pH meter. The concentration of organic acids took into account the rhizosphere value reported by Jones (Citation1998). Each treatment was prepared in triplicate. The 0.15 g aliquots of biochar samples (220°C, 320°C, 420°C, 520°C, and 620°C biochar and non-pyrolyzed SS), were weighed into a 50 mL Falcon tube and equilibrated with 15 mL of the 1 mM acid solution. The acid solutions without biochar were included as a blank. The flasks were then incubated at 25°C for 4 h with shaking in an orbital shaker at 150 rpm. At the end of the extraction period, centrifugation was performed at 10,000 × g for 5 min followed by filtration using filter paper (Whatman 42). The concentrations of P, K, Ca, Mg, Fe, and Al were determined using an inductively coupled plasma-optical emission spectrometer (ICP-OES, Agilent 720, Agilent Technologies Inc., USA), equipped with a two-channel peristaltic pump, a Sturman-Masters spray chamber, and a V-groove pneumatic nebulizer made of inert material.
Table 1. The main chemical characteristics of organic acids used for extraction of phosphorus from biochar.
Data analysis
Factorial analysis of variance (ANOVA, p < 0.05), and Tukey’s honestly significant difference (HSD) post-hoc test was performed using Statistica 12.0 software (TIBCO Software Inc.) to determine significant differences between the individual variables. A correlation analysis was used for the assessment of relationships between individual variables; Pearson’s correlation was used with p < 0.05 as the criterion for significance. Principal component analysis (PCA) was performed using XLSTAT (a statistical add-in for Microsoft Excel) software.
Results and discussion
Phosphorus release with the LMWOAs
The total element contents of the biochar have been presented elsewhere (Mercl et al. Citation2020), where the P content of the non-pyrolyzed dry SS was 32.6 ± 0.3 g/kg, and increased with increasing pyrolysis temperature from 36.4 ± 0.40 g/kg (220°C) to 60.8 ± 0.39 g/kg (620°C). Table presents the proportions of extractable P associated with the individual LMWOAs from the biochars pyrolyzed at different temperatures. Among the twelve organic acids, citric acid extracted the highest P proportions (significant at p < 0.05), regardless of the biochar pyrolysis temperature; mucic and oxalic acid also showed quite good extraction potential. This result is well supported by the findings of Ivanova et al. (Citation2006) where citric acid was confirmed as the most efficient organic acid for P extraction from Tunisian phosphorite. Wei et al. (Citation2010) also found the same result in forest soils where citric acid significantly enhanced the solubilization of organic P by 34.7%, as compared with water; whereas no significant differences were observed in the mobilization of organic P by maleic acid, oxalic acid, or water. The efficiency of citric acid to release soil P was also confirmed by Chatterjee et al. (Citation2015). In the wastewater treatment plants, Fe3+ is for P precipitation as FeCl3 and/or Fe2(SO4)3. Therefore, the total Fe contents in the SS reached up to 4.8% (Mercl et al. Citation2020), and the presence of P as FePO4 and other iron bearing phosphates is expected. Pyrolysis degrade the organic P present in the SS on inorganic, which is again associated predominantly with Fe (due to its high content), but also with Al and Ca (Huang et al. Citation2017). As stated by Zhang et al (Citation2020), deprotonated citric acid is polyvalent anions able to exchange with P in biochar with a high ratio of trivalent metals to P, such as Fe3+ in this case.
Table 2. Extractable phosphorus content from the biochar matrix by the individual acids (mg/kg); data expressed as mean ± standard deviation (n = 3); averages marked by the same letter did not significantly differ at p < 0.05 within individual rows (lower case letters), and columns (upper case letters).
Conversely, Khademi et al. (Citation2009) and Ström et al. (Citation2005) found that oxalate was slightly more effective than citrate in mobilizing P from calcareous soils. The superiority of oxalic acid compared to other LMWOAs in releasing P from calcareous soils was also reported by Moradi et al. (Citation2012). The release of P with the LMWOAs differed for inorganic and organic forms of P, where the extractability of organic P followed the order oxalic acid > citric acid > malic acid, whereas the release of inorganic P followed the order citric acid > oxalic acid > malic acid (Wang et al. Citation2018). These findings indicate the importance of the P speciation in the soil/biochar, as well as the physicochemical parameters of the soil, and the fate of P having contact with LMWOAs. In the case of biochar, the presence of residual organic compounds of P can be expected and, therefore, citric acid should be preferred to achieve effective release of biochar-bearing P. A comparison of the P release with LMWOAs is limited also due to different composition of both materials. Moreover, in soil, citric acid is an excellent substrate for soil microorganisms, resulting in the fast degradation of citric acid compared to for instance oxalic acid (Oburger et al. Citation2009). Thus, lower effect of citric acid compared to other LMWOAs in soils is expectable. In this context, Wang et al. (Citation2020) reported an increase in microbial P after the addition of citric acid, malic acid, oxalic acid, or their mixture to the soil, where the changes in microbial P were related to the changes in organic C, soil pH, and available P in the soils.
Basak (Citation2019) tested the efficiency of the LMWOAs on P release from rock phosphates, where the proportions of the extracted P with the individual acids followed the order oxalic acid > citric acid > tartaric acid > formic acid > malic acid > succinic acid > acetic acid. Moreover, the proportions of P released increased as the pH of the extracting solution decreased. Similarly, an inverse relationship between the amounts of P released from soils and the pKa values of the organic acids was reported by Harrold and Tabatabai (Citation2006). The importance of the extractant concentrations was also highlighted by Yang et al. (Citation2019a), where when the concentrations were ≤1 mM, oxalic acid lower proportion of P than citric acid, and when the concentrations were ≥1.5 mM, oxalic acid was more effective. Thus, the similar pattern was expected also in this study, if 1 mM concentration of the acids was used. The pH values of the extracting agents varied between 2.87 and 5.62 (Table ), and the different efficiency of the individual acids could be at least partially related to their different degrees of acidity. Yang et al. (Citation2019b) showed the importance of pH in the P-biochar interactions. However, although the extractable proportion of P tended to decrease with increases in the pH of the extracting agent, Pearson’s correlation coefficients did not indicate any significant (p < 0.05) relationship between the extracting solution’s pH and extractable P levels, and, therefore, the role of the solution pH remains in question. Moreover, as reviewed by Yang et al. (Citation2021), the soil application of biochar will affect the potential P-release an additional interaction with the soil, where the P mobility in the soil will be a result of (i) the mobile P pool originating from the biochar, (ii) the enhancement of the mobility of the soil-bearing P due to the soil-biochar interaction, and (iii) reduction of the P losses in the biochar treated soils.
Other macro-and micronutrient release with the LMWOAs and their role in the P release
Tables summarize the extractable contents of K, Ca, Mg, and Fe from the biochar samples. The maximum K content (899 ± 8 mg/kg) was extracted from the non-pyrolyzed SS by succinic acid (Table ); pyrolysis resulted in a decrease in the extractable K proportion. Of the other LMWOAs, malic and citric acid also showed quite high extractable K in high-temperature biochar (420, 520, and 620°C), whereas for lower temperature biochars, only occasional significant (p < 0.05) differences in the K extractability were recorded among the LMWOAs. Previous research presented by Khademi et al. (Citation2009) found high concentrations of citric acid were more effective than oxalate in mobilizing Ca, Fe, Mn, and Zn. This study found that trans-aconitic acid was the most effective OA for calcium extraction and the highest amount of extractable calcium was obtained from 220°C biochar (Table ), but Ca was better extracted with citric acid than with oxalic acid. Similarly as in the case of P, the extractable nutrient proportions remained unaffected by the solution pH, where no significant (p < 0.05) correlations were recorded.
Table 3. Extractable potassium content from biochar matrix by the individual acids (mg/kg); data expressed as mean ± standard deviation (n = 3); averages marked by the same letter did not significantly differ at p < 0.05 within individual rows (lower case letters) or columns (upper case letters).
Table 4. Extractable calcium contents from biochar matrix by the individual acids (mg/kg); data expressed as mean ± standard deviation (n = 3); averages marked by the same letter did not significantly differ at p < 0.05 within individual rows (lower case letters) or columns (upper case letters).
Table 5. Extractable magnesium contents from biochar matrix by the individual acids (mg/kg); data expressed as mean ± standard deviation (n = 3); averages marked by the same letter did not significantly differ at p < 0.05 within individual rows (lower case letters) or columns (upper case letters).
Table 6. Extractable iron contents from biochar matrix by the individual acids (mg/kg); data expressed as mean ± standard deviation (n = 3); averages marked by the same letter did not significantly differ at p < 0.05 within individual rows (lower case letters) or columns (upper case letters).
Wang et al. (Citation2016) reported that LMWOAs (oxalic, citric, and malic acids) can enhance P release from soils due to ligand exchange and the chelation of LMWOAs with Fe and Al. The role of Fe/Al (hydr)oxides in the P release from biochar was presented by Peng et al. (Citation2019). The organic acids exhibit chelation and complexing properties, where citric acid belongs to the effective (Kpomblekou and Tabatabai Citation2003; Alori et al. Citation2017) and is a well-known chelating agent (Kubota et al. Citation2010). As mentioned above, in the SS-based biochar, characterized by the high content of Fe-bearing phosphates, the LMWOAs with tri-carboxylic groups have excellent chelating ability with polyvalent metal ions. Similarly, Wu et al. (Citation2015) showed LMWOAs stimulated release of inorganic P from the soil due to their ability to complex or precipitate with cations such as Fe, Al, and Ca. As reviewed by Kratz et al. (Citation2019), in a chemical waste water treatment process, P will be adsorbed to Fe/Al-hydroxides, or precipitate as Fe/Al-phosphate or basic calcium phosphates, and these compounds can be easily dissolved with citric acid. Taghipour and Jalali (Citation2013) found a different pattern for the individual LMWOAs in contact with individual soil fractions, where oxalate mainly released P contained in Ca–P minerals, whereas citrate predominantly released the P contained in Fe–P and Al–P minerals. The interactions of these elements could indicate which acid releases P from particular compounds. In this experiment, Figure indicated that there was a close relationship among the proportions of Al, Fe, and P extractable with citric acid, whereas a weaker relationship was observed for the P – Ca interaction. Moreover, a similar pattern was observed for Ca, Mg, and K, which were extractable with most of the tested LMWOAs (except citric acid) from the non-pyrolyzed SS and from biochar pyrolyzed at 220°C. No relationships were recorded between the LMWOAs and extractable Ca, K, Mg proportions at the higher pyrolysis temperatures. The findings presented in Figure are supported by the significant (p < 0.05) Pearson’s correlations between P and Fe proportions extractable with citric acid (r = 0.97).
Figure 1. Principal component analysis (PCA) plot of biochar pyrolysis temperatures. The first two principal components (PCs) are plotted. PCA was performed using all analyte data. The percentage of variation accounted for by each PC is shown in brackets with the axis label.
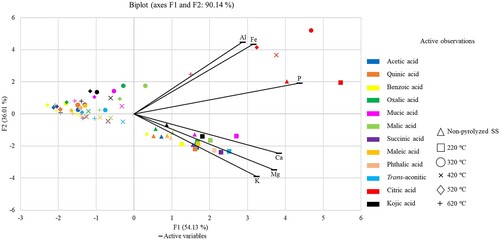
Additionally, Bolan et al. (Citation1994) found (decades ago) decreasing P adsorption by soils after the addition of organic acids in the order tricarboxylic acid > dicarboxylic acid > monocarboxylic acid. In our study, the extractable P proportions correlated significantly (p < 0.05) with the number of carboxylic groups of the acids (the r-value varied between 0.62 and 0.68), partially confirming these findings. Whereas enhanced extraction efficiency was proven for citric acid compared to the other extraction agents tested, P extractability with trans-aconitic acid did not differ from the dicarboxylic acids such as succinic, phthalic, and maleic acids (Table ). Thus, the results showed the exceptional performance of citric acid for potential enhancement of P release from SS-derived biochar, where the release of the other nutrients remained unaffected.
Moreover, the efficiency of the individual LMWOAs can be modified in real conditions by the presence of other acids. For instance, P release from sediments was enhanced by using an oxalate/citrate mixture, compared to the application of individual extracting agents, indicating the potential synergetic effect of these LMWOAs (Gaviglio et al. Citation2019). Similarly, Mondala et al. (Citation2017) reported that 2:1 and 1:1 (molar ratios) oxalate-citrate mixtures were able to release up to 61% of total bound P from sediments. LMWOAs are used by rhizosphere microorganisms as sources of carbon and energy, and citric acid favored the proliferation of the Clostridiaceae, Micrococcaceae, and Pseudomonadaceae families (Macias-Benitez et al. Citation2020). In addition, the enhanced release of P from various P-bearing minerals and rocks due to enhanced soil LMWOAs produced by various soil microorganisms, such as Penicillium spp., Talaromyces spp., Aspergillus niger, etc. has been described (Arcand and Schneider Citation2006; Scervino et al. Citation2010; Xiao et al. Citation2013; Solans et al. Citation2019).
The role of biochar pyrolysis temperature in the nutrient release performances
Among the biochars, a significantly higher amount of P was extracted (4174.6 ± 76.6 mg/kg) from 320°C biochar compared to the non-pyrolyzed SS. In contrast, the highest pyrolysis temperature, 620°C, resulted in a significantly (p < 0.05) lower proportion of P released with citric acid compared to all the biochars pyrolyzed at the lower temperatures and to the non-pyrolyzed SS (Table ). Compared to citric acid, the other investigated LMWOAs showed increased (p < 0.05), or similar proportions of P vs. the non-pyrolyzed SS, with regular decreases in extractable P with increasing biochar pyrolysis temperatures (Table ).
The extractable calcium proportion was highest in the case of the 220°C biochar, as also reported by Mercl et al. (Citation2020). The extractability of Mg showed a similar pattern, where the highest value of Mg (1362.7 ± 68.1 mg/kg) was released by trans-aconitic acid (Table ). A significant (p < 0.05) decrease in extractable Mg was observed for the biochars prepared at temperatures >220°C. Citric acid was the most effective OA for all types of biochars (and the non-pyrolyzed SS) in the case of iron (Table ). Citric acid showed the highest iron extraction (60451 ± 104 mg/kg) with the 320°C biochar. Kojic acid, malic acid, and mucic acid showed a small amount of extractable iron for the high-temperature biochars (520°C and 620°C). One important finding was that as the pyrolysis temperature increased from 420°C to 620°C iron extraction decreased. Apart from the elements mentioned above, the extractability of Al was tested, where most of the measured data were under the detection limit of the analytical technique used. Detectable Al content was found only in the case of five LMWOAs, and their extractability decreased in the order citric > mucic > kojic > malic > oxalic acids. The extractable contents of Al in the case of non-pyrolyzed SS varied between 268 mg/kg (citric acid) and 7.3 mg/kg (oxalic acid). In regard to the biochars, the citric acid extractable proportions of Al slightly increased compared to the non-pyrolyzed SS, and the pyrolysis temperature did not affect Al extractability. For malic, kojic, and mucic acids the extractable Al proportions from biochars significantly (p < 0.05) increased with increasing pyrolysis temperature, and the ambiguous results obtained for the Al content extractable with oxalic acid can be related to the low levels of this element, which are close to the detection limit.
Conclusions
The results showed a different response for P extractability depending on the application of different LMWOAs, but the effect of the chemical properties and the composition of the individual acids, and the presence of other elements also impacted the response. Moreover, the results showed specific interactions between LMWOAs and SS-based biochar, which could modify the P mobility in biochar treated soils. Among the P solubilizing microorganisms, those producing preferably citric acid should be considered as potential bioaugmentation measures for the effective enhancement of P release from SS-derived biochar, and, subsequently, to improve the availability of P originating from biochar. Therefore, in further experiments, the role of the LMWOAs in the P release should be tested in the soil-biochar system.
Acknowledgements
The authors thank NAZV QK1710379 project and European Regional Development Fund – Project No. CZ.02.1.01/0.0/0.0/16_019/0000845 for financial support. Correction and improvement of language was provided by Proof-Reading-Service.com Ltd., Devonshire Business Centre, Works Road, Letchworth Garden City SG6 1GJ, United Kingdom.
Disclosure statement
No potential conflict of interest was reported by the author(s).
Data availability statement
The data that support the findings of this study are openly available in Mendeley Data: https://doi.org/10.17632/5vf53yy24p.1.
Additional information
Funding
References
- Adeleke R, Nwangburuka C, Oboirien B. 2017. Origins, roles and fate of organic acids in soils: a review. South Afr J Bot. 108:393–406.
- Alori ET, Glick BR, Babalola OO. 2017. Microbial phosphorus solubilization and its potential for use in sustainable agriculture. Front Microbiol. 8:971.
- Arcand MM, Schneider KD. 2006. Plant- and microbial-based mechanisms to improve the agronomic effectiveness of phosphate rock: a review. An Acad Bras Cienc. 78:791–807.
- Basak BB. 2019. Phosphorus release by low molecular weight organic acids from low-grade Indian rock phosphate. Waste Biomass Valor. 10:3225–3233.
- Bolan NS, Naidu R, Mahimairaja S, Baskaran S. 1994. Influence of low-molecular-weight organic acids on the solubilization of phosphates. Biol Fertil Soils. 18:311–319.
- Chatterjee D, Datta SC, Manjaiah KM. 2015. Effect of citric acid treatment on release of phosphorus, aluminium and iron from three dissimilar soils of India. Arch Agron Soil Sci. 61:105–117.
- Chen YP, Rekha PD, Arun AB, Shen FT, Lai WA, Young CC. 2006. Phosphate solubilizing bacteria from subtropical soil and their tricalcium phosphate solubilizing abilities. Appl Soil Ecol. 34:33–41.
- Gaviglio K, Shields S, Mondala A. 2019. Synergistic runoff particulate-bound phosphorus extraction by oxalate and citrate ligands in a mixed aqueous extractant system. Clean – Soil Air Water. 47:1700499.
- Glaser B, Lehr V. 2019. Biochar effects on phosphorus availability in agricultural soils: a meta-analysis. Sci Rep. 9:9338.
- Hanč A, Tlustoš P, Szákova J, Balík J. 2007. The changes of cadmium and zinc mobility in sewage sludges after their treatment. Chem Listy. 101:807–810.
- Harrold SA, Tabatabai MA. 2006. Release of inorganic phosphorus from soils by low-molecular-weight organic acids. Commun Soil Sci Plant Anal. 37:1233–1245.
- Huang R, Fang C, Lu X, Jiang R, Tang Y. 2017. Transformation of phosphorus during (hydro)thermal treatments of solid biowastes: reaction mechanisms and implications for P reclamation and recycling. Environ Sci Technol. 51:10284–10298.
- Ivanova RP, Bojinova DY, Gruncharov IN, Damgaliev DL. 2006. The solubilization of rock phosphate by organic acids. Phosphorus Sulfur Silicon Relat Elem. 181:2541–2554.
- Jones DL. 1998. Organic acids in the rhizosphere – a critical review. Plant Soil. 205:25–44.
- Jones V, Gardner M, Ellor B. 2014. Concentrations of trace substances in sewage sludge from 28 wastewater treatment works in the UK. Chemosphere. 111:478–484.
- Khademi Z, Jones DL, Malakouti MJ, Asadi F, Ardebili M. 2009. Organic acid mediated nutrient extraction efficiency in three calcareous soils. Soil Res. 47:213–220.
- Kirkby EA, Johnston AE. 2008. Soil and fertilizer phosphorus in relation to crop nutrition. In: White PJ, Hammond JP, editors. The ecophysiology of plant-phosphorus interactions. Dordrecht: Springer Netherlands; p. 177–223.
- Kpomblekou AK, Tabatabai MA. 2003. Effect of low-molecular weight organic acids on phosphorus release and phytoavailabilty of phosphorus in phosphate rocks added to soils. Agric Ecosyst Environ. 100:275–284.
- Kratz S, Vogel C, Adam C. 2019. Agronomic performance of P recycling fertilizers and methods to predict it: a review. Nutr Cycl Agroecosyst. 115:1–39.
- Kubota T, Rinaldi N, Okumura K, Honma T, Hirayama S, Okamoto Y. 2010. In situ XAFS study of the sulfidation of Co–Mo/B2O3/Al2O3 hydrodesulfurization catalysts prepared by using citric acid as a chelating agent. Appl Catal Gen. 373:214–221.
- Li Z, Bai T, Dai L, Wang F, Tao J, Meng S, Hu Y, Wang S, Hu S. 2016. A study of organic acid production in contrasts between two phosphate solubilizing fungi: Penicillium oxalicum and Aspergillus niger. Sci Rep. 6:25313.
- Macias-Benitez S, Garcia-Martinez AM, Jimenez PC, Gonzalez JM, Moral MT, Rubio JP. 2020. Rhizospheric organic acids as biostimulants: monitoring feedbacks on soil microorganisms and biochemical properties. Frontiers Plant Sci. 11:633.
- Mercl F, Košnář Z, Maršik P, Vojtíšek M, Dušek J, Száková J, Tlustoš P. 2021. Pyrolysis of biosolids as an effective tool to reduce the uptake of pharmaceuticals by plants. J Hazard Mat. 405:124278.
- Mercl F, Košnář Z, Pierdonà L, Ulloa-Murillo LM, Száková J, Tlustoš P. 2020. Changes in availability of Ca, K, Mg, P and S in sewage sludge as affected by pyrolysis temperature. Plant Soil Environ. 66:143–148.
- Mondala A, Shields S, Gaviglio K, Kaczmarek S. 2017. Influence of fungal low molecular weight organic acids on extraction and speciation of runoff particulate-associated phosphorus: implications for nonpoint phosphorus recovery and beneficial reuse. Environ Prog Sustain Energy. 36:1810–1816.
- Moradi N, Sadaghiani MR, Sepehr E, Mandoulakani BA. 2012. Effects of low-molecular-weight organic acids on phosphorus sorption characteristics in some calcareous soils. Turk J Agric For. 36:459–468.
- Oburger E, Jones DL, Wenzel WW. 2011. Phosphorus saturation and pH differentially regulate the efficiency of organic acid anion-mediated P solubilization mechanisms in soil. Plant Soil. 341:363–382.
- Oburger E, Kirk GJD, Wenzel WW, Puschenreiter M, Jones DL. 2009. Interactive effects of organic acids in the rhizosphere. Soil Biol Biochem. 41:449–457.
- Peng Y, Sun Y, Sun R, Zhou Y, Tsang DCW, Chen Q. 2019. Optimizing the synthesis of Fe/Al (Hydr)oxides-biochars to maximize phosphate removal via response surface model. J Clean Product. 237:117770.
- Pulkrabová J, Černý J, Száková J, Švarcová A, Gramblička T, Hajšlová J, Balík J, Tlustoš P. 2019. Is the long-term application of sewage sludge turning soil into a sink for organic pollutants?: Evidence from field studies in the Czech Republic. J Soils Sediments. 19:2445–2458.
- Qian T, Yang Q, Jun DCF, Dong F, Zhou Y. 2019. Transformation of phosphorus in sewage sludge biochar mediated by a phosphate-solubilizing microorganism. Chem Eng J. 359:1573–1580.
- Scervino JM, Mesa MP, Della Monica I, Recchi M, Moreno NS, Godeas A. 2010. Soil fungal isolates produce different organic acid patterns involved in phosphate salts solubilization. Biol Fertil Soils. 46:755–763.
- Singh R. P., Agrawal M. 2008. Potential benefits and risks of land application of sewage sludge. Waste Manag. 28:347–358.
- Solans M, Messuti MI, Reiner G, Boenel M, Vobis G, Wall LG, Scervino JM. 2019. Exploring the response of Actinobacteria to the presence of phosphorus salts sources: metabolic and co-metabolic processes. J Basic Microbiol. 59:487–495.
- Ström L, Owen AG, Godbold DL, Jones DL. 2005. Organic acid behaviour in a calcareous soil implications for rhizosphere nutrient cycling. Soil Biol Biochem. 37:2046–2054.
- Taghipour M, Jalali M. 2013. Effect of low-molecular-weight organic acids on kinetics release and fractionation of phosphorus in some calcareous soils of western Iran. Environ Monit Assess. 185:5471–5482.
- Tao Y, Han S, Zhang Q, Yang Y, Shi H, Akindolie MS, Jiao Y, Qu J, Jiang Z, Han W, Zhang Y. 2020. Application of biochar with functional microorganisms for enhanced atrazine removal and phosphorus utilization. J Clean Prod. 257:120535.
- Van Dijk K. C., Lesschen J. P., Oenema O. 2016. Phosphorus flows and balances of the European Union Member States. Sci.Total Environ. 542:1078–1093.
- Wang Y, Chen X, Lu C, Huang B, Shi Y. 2018. Different mechanisms of organic and inorganic phosphorus release from Mollisols induced by low molecular weight organic acids. Can J Soil Sci. 98:15–23.
- Wang Y, Peng X, Wang X, Gan G, Zhang X. 2020. Microbial phosphorus, available phosphorus, organic carbon and pH changes in calcareous, neutral and acidic soils induced by low molecular weight organic acid addition. Fresenius Environ Bull. 29:693–699.
- Wang Y, Whalen JK, Chen X, Cao Y, Huang B, Lu C, Shi Y. 2016. Mechanisms for altering phosphorus sorption characteristics induced by low-molecular-weight organic acids. Can J Soil Sci. 96:289–298.
- Wei L, Chen C, Xu Z. 2010. Citric acid enhances the mobilization of organic phosphorus in subtropical and tropical forest soils. Biol Fertil Soils. 46:765–769.
- Wu YF, Wang YZ, Zhang XY. 2015. Mobilization of P by low molecular weight organic acids in a calcareous, neutral and acid soil with low available P status. In: Khatib JM, editor. Energy, environmental & sustainable ecosystem development. Singapore: World Scientific Publ Co Pte Ltd.
- Xiao CQ, Chi RA, Hu LH. 2013. Solubilization of aluminum phosphate by specific Penicillium spp. J Centr South Univ. 20:2109–2114.
- Yang X, Chen X, Guo E, Yang X. 2019a. Path analysis of phosphorus activation capacity as induced by low-molecular-weight organic acids in a black soil of Northeast China. J Soils Sedim. 19:840–847.
- Yang L, Wu YC, Wang YC, An WQ, Jin J, Sun K, Wang XK. 2021. Effects of biochar addition on the abundance, speciation, availability, and leaching loss of soil phosphorus. Sci Total Environ. 758:143657.
- Yang F, Zhang S, Sun Y, Tsang DCW, Cheng K, Ok YS. 2019b. Assembling biochar with various layered double hydroxides for enhancement of phosphorus recovery. J Hazard Mat. 365:665–673.
- Zhang H, Li Q, Zhang X, Chen W, Ni J, Yang L, Wei R. 2020. Insight into the mechanism of low molecular weight organic acids-mediated release of phosphorus and potassium from biochars. Sci Total Environ. 742:140416.