Abstract
Gender research shows excellent significance in the breeding and production of poultry, achieving the actual needs of poultry through targeted gender regulation could increase economic benefits. However, the mechanism of sex determination in poultry remains unclear. We respectively constructed HMGCS1 overexpression and interference vectors for lentivirus packaging. Vascular microinjection was performed when fertilized eggs developed to 2.5 days. qRT-PCR and ELISA results indicated that HMGCS1 could promote the expression of male germ-cell development-related genes (SOX9) and inhibit the expression of female germ-cell development-related genes (CYP19A1 and DMRT1). Furthermore, the synthesis of cholesterol and testosterone in the gonads were increased. In addition, the injection of lovastatin into chicken embryos, an inhibitor of cholesterol synthesis, showed a significant decrease in gonadal testosterone. This experiment revealed that HMGCS1 could regulate the synthesis of testosterone by affecting the content of cholesterol in gonads, and promote the differentiation of chicken gonads into males.
Introduction
In poultry farming, male and female individuals have different applications in production, which was attributed to the difference of physiological, such as the great demand for hens in the layer breeding industry. Due to the fast growth of roosters and the high feed conversion rate, more roosters are wanted in the broiler breeding industry. If sex identification or sex control is carried out in the early embryo period, the sex ratio can be controlled according to the production needs, which will bring huge economic benefits to the breeding industry. Therefore, it is of great significance to find out the sex regulation genes of poultry and develop the sex regulation technology based on the sex determination mechanism. Up to now, only mammalian SRY gene and medaka DMY gene have been discovered to play a sex-determining role in Y chromosome (Kattar-Cooley et al. Citation1990; Smith and Sinclair Citation2004). DMW gene in Xenopus africanus has been identified as the ovarian determining gene in W chromosome (Bruggeman et al. Citation2002). However, no key sex-determining gene similar to the mammalian SRY gene has been found in poultry. In the early stage, our research group conducted transcriptome sequencing on embryonic stem cells (ESCs) and primordial germ cells (PGCs) cells of male and female chickens respectively, analyzed the genes differentially expressed between males and females, and screened out the potential key gene HMGCS1 for gender determination.
HMGCS1 (3-hydroxy-3-methylglutaryl coenzyme A synthetase 1) is a member of the HMGCS family located in the cytoplasm (Eacker et al. Citation2008). Xu et al. reported that the expression of HMGCS1 was positively correlated with the cholesterol content of Goose liver, which is a key gene in the process of cholesterol synthesis in foie gras (Elbrecht and Smith Citation1992). Wang et al. discovered that the cholesterol involved in the synthesis of HMGCS1 can synthesize and metabolize steroid hormone through a series of regulatory proteins (Kashimada and Koopman Citation2010). Li et al. added an appropriate amount of cholesterol to rat testicular mesenchymal cells cultured in vitro, which can increase the synthesis of testosterone (Lambeth et al. Citation2013), indicating that cholesterol improved the synthesis of testosterone. During the growth of chicken embryos, changes in the content of sex hormones influenced the gender differentiation of chicken embryos. Zhang et al. confirmed this view. During 0–6 days of early embryo incubation, male embryonic gonads can be transformed into females by injecting estradiol through the air chamber of fertilized eggs. Similarly, testosterone injection leads to abnormal male-oriented differentiation of female eggs (Matsuda et al. Citation2002). However, the study of HMGCS1 in the process of chicken embryo gonadal differentiation has not been reported.
In order to clarify the effect of HMGCS1 gene on gender differentiation during the development of chicken embryos, this study constructed overexpression and interference vectors of HMGCS1 and then injected into the blood vessel of chicken embryos after lentivirus packaging. It proved that HMGCS1 in chicken embryos could improve the content of cholesterol and testosterone in the gonads, and regulate the differentiation of chicken embryos into males. This study is the first to explore the role of HMGCS1 in the process of chicken sex differentiation, laying a foundation for improving the molecular mechanism theory of poultry sex determination.
Materials and methods
Materials
Fresh fertilized eggs came from Rugao Yellow chicken at an experimental poultry farm of poultry research institute of the Chinese academy of agricultural sciences. TRIZOL (TIANGEN, Beijing, China), reverse transcription kit (TIANGEN, Beijing, China), qRT-PCR kit (TIANGEN, Beijing, China), de-endotoxin plasmid small-scale extraction kit (TIANGEN, Beijing, China), gel cutting recovery kit (TIANGEN, Beijing, China), cholesterol (Solarbio, Beijing, China), lovastatin (Solarbio, Beijing, China), and methyl-choncyclodextrin (Solarbio, Beijing, China). Chicken Cholesterol Elisa kit, Chicken Testosterone Elisa kit (YILI Biotechnology. Shanghai). FuGene HD Transfection Reagent (Promega, Wisconsin, United States). SABC immunohistochemical staining kit (Boster, China). DAB color development kit (Boster, China). DAZL antibody (Abcam, United States).
Construction of pcDNA-HMGCS1 expression vector
The amplification primers were designed according to the CDS region of the target gene. Insert the 5′ ends of the designed primers into the restriction sites KpnI (GGTACC), Xhol (CTCGAG), and protective bases. The primer sequences were as follows (synthesized by Shengong Biotechnology Co., Ltd. Shanghai):
F-pcDNA-HMGCS1: 5′ CGGGGTACCGGAGGCAGGAGTTGAAGTTGTC 3′
R-pcDNA-HMGCS1: 5′ CCGCTCGAGAGGGATTCTTGGCACTTTCTTAGC 3′
Total RNA was extracted from chicken embryo testis tissue, and cDNA was synthesized using a reverse transcription kit as a template for PCR amplification. The PCR program was: pre-denaturation at 98 °C for 3 min; denaturation at 98 °C for 25 s, annealing at 56 °C for 30 s, extension at 72 °C for 1 min and 30 s, 35 cycles; 72 °C for 7 min. The PCR product was detected by agarose gel electrophoresis, and the target gene HMGCS1 was collected by gel cutting. KpnI and Xhol enzymes were used to digest the target gene and pcDNA3.0 vector to recover the product by double digestion, and then ligated for 16 h under the action of T4 ligase. The ligated product was transformed into DH5α cells, spread on an ampicillin-resistant plate and cultured overnight. PCR was performed for the target gene detection and sequencing verification. The constructed vector was sent to the company (Genecreate, China) to obtain the lentivirus-mediated vector.
Construction of HMGCS1 interference vector
According to the principle of RNA interference sequence design, Shanghai Gima Company used the gene CDS to design three shRNA interference target sites (Table ). The RNA interference gatcc-TTCAAGAGA-TTTTTTg sequence was added to form shRNA oligo. After annealing, it was combined with plasmid pGMLV-SC5. Connected to form recombinant plasmids shRNA-1, shRNA-2, shRNA-3. After being transformed into competent cells, sequencing was performed at Biotech Bioengineering Co., Ltd Shanghai. The extracted sh-HMGCS1 plasmid was transfected into DF1 cells using FuGene HD Transfection Reagent. The fluorescence of the cells was observed at 72 h and the expression of HMGCS1 in the cells was detected by qRT-PCR. The constructed vector was sent to the company (Genecreate, China) to obtain the lentivirus-mediated vector.
Table 1. Primers used for qRT-PCR analyses.
Chicken embryo sex identification
Take chicken embryo blood as a template, and then identify the gender of the embryo through PCR amplification using the CHD1 (Chromo-helicase-DNA-Binding gene)(Wang et al. Citation2009) primer sequences: F: GTTACTGATTCGTCTACGAGA and R: ATTGAAATGATCCAGTGCTTG in a polymerase chain reaction (PCR) system consisting of 30 cycles of: 98°C for 10 s, 52°C for 5 s, and 72°C for 30 s, followed by long-term storage at 4°C. The results showed by gel electrophoresis that the female (ZW) had two bands at 600 and 450 bp, while the male (ZZ) had only one band at 600 bp.
Chicken embryo vascular injection
The fertilized eggs were incubated at 38°C for 2.5 days. Wiped the blunt end of the fertilized egg with alcohol for disinfection. Put the egg under the microscope to open the tip. We observed the chicken embryo to find a circle of blood vessels surrounded by the heart, which was the injection site. Each egg was injected with 2 µL of the mixed solution. After injection, 20 µL of the mixture of penicillin (100 U/mL) and streptomycin (100 U/mL) was injected into the fertilized egg, sealed with medical tape and transferred back to the incubator to continue incubation. The experiments were divided into three groups, each group was injected with 80 fertilized eggs (The samples should be sampled eight times): Blank control group: The chicken embryo had a hole on the top with no treatment, and then the egg was sealed and hatched. Overexpression group: 20 µL of lentiviral vector-mediated OE-HMGCS1 was added to 80 µL of ordinary culture medium, and then mixed. Interference group: 10 µL of lentiviral vector-mediated sh-HMGCS1 was added to 90 µL of ordinary culture medium.
Chicken embryo air chamber injection
We took the fertilized eggs incubated at 38°C for 2.5 days, wiped the blunt end of them with alcohol, and then made a small opening in the air chamber position with a syringe needle. Each egg was injected with 0.1 mL of solution at an angle of 30°.
qRT-PCR
The RNA of cultured cells and tissues was extracted with TRIZOL, and after reverse transcription into cDNA, the target genes HMGCS1, sex-related genes SOX9, DMRT1 and CYP19A1, and testosterone synthesis-related genes CYP11A1 were detected by quantitative PCR. Real-time quantitative analysis was performed using the CFX96 TouchTM real-time PCR instrument. The instruction manual of the real-time PCR reaction system was referred to. The reaction procedure used a two-step method: pre-denaturation at 95 °C for 15 min; denaturation at 95 °C for 10 s, annealing at 60 °C for 32 s, and cycling 40 times. Internal reference gene: β-actin, number of repetitions: n = 3. The relative expression of each gene was calculated using the 2-ΔΔCt method. The primer sequences of more genes are shown in Table .
ELISA test
The fertilized eggs were injected at 2.5 days after hatching and then sampled every two days until 18.5 days. The male and female chicken embryos were separated by sex determination by blood, and three complete gonad tissues were taken from each group into 1.5 mL enzyme-free tubes. As the gonad tissues continued to grow, an appropriate amount of PBS solution was added with the same amount in the same batch of the same group. We placed it in an ice box and used an ultrasonic crusher to break the tissues. The crusher program was to crush for 10 s and then stop for 30 s lasts for 5 min. Then centrifuged at 2000–3000 rpm/min for 20 min at 4 °C. The supernatant was collected for testing, or stored in an enzyme-free tube at −20 °C. The Chicken Cholesterol Enzyme-linked Immunoassay kit and Chicken Testosterone Enzyme-linked Immunoassay kit were used to detect the cholesterol and testosterone content according to the instructions.
Tissue immunofluorescence
The obtained tissues were immersed in tissue fixation solution for 24 h, then 70% alcohol overnight, 80%, 90%, and 100% dehydrated for half an hour, and immersed in xylene solution for 10 min. Finally, after soaking in paraffin for 1 h, they could be embedded with a copper frame. The thickness of the slice was preferably 5–7 µm. After patching, they were dewaxed and rehydrated for 2–3 min by xylene, 100%, 90%, 80%, and 70% alcohol. Then hematoxylin–eosin staining was performed. We observed and took pictures under an upright microscope (Leica, Germany). The sections were labeled with DAZL antibody according to the instructions of the SABC immunohistochemical staining kit (Boster, China), and finally the DAB color development kit (Boster, China) was used for color development. Image J was used to analyze the proportion column of convoluted tubules.
Preparation of cholesterol solution
Of 0.012 g of cholesterol was dissolved in 1 mL of absolute ethanol to prepare a cholesterol ethanol solution with a concentration of 12 mg/mL. 0.05 g of methyl β-cyclodextrin was added to 2 mL EP tube, and 1 mL of distilled water was added to prepare a methyl β-cyclodextrin solution. The cholesterol solution and the methyl β-cyclodextrin solution were heated in a water bath to 65 °C. Methyl β-cyclodextrin solution (916.7 mL) and cholesterol solution (83.3 mL) were added to prepare a cholesterol solution (1 mg/mL) into a new 2 mL EP tube. The cholesterol solution was mixed with normal saline in a ratio of 2:3 to make the final concentration 0.4 mg/ mL to form the cholesterol injection solution (Muller et al. Citation1979).
Dissolution of lovastatin
About 1 mg lovastatin was ‘dissolved in 1 mL DMSO and made into a lovastatin solution. The lovastatin solution and physiological saline were heated in a water bath at a ratio of 1: 3 and mixed to prepare 0.25 mg / mL lovastatin injection.
Data analysis
SPSS statistical software was used to establish a database and process the data. Differences between the data groups were analyzed by single-factor analysis of variance, and multiple comparisons were performed using the LSD method. The test data was significantly different at P < .05 and extremely significant at P < .01. Results were expressed as mean.
Results
Cholesterol promotes the synthesis of hormones during gonadal development
To verify the effect of cholesterol on sex hormones in chicken embryos, six different concentrations of cholesterol (1, 0.5, 0.4, 0.3, 0.2 and 0.1 mg/mL), and five different concentrations of cholesterol inhibitor lovastatin (0.5, 0.4, 0.3, 0.25 and 0.1 mg/mL) were first injected into chicken embryos at the third day of development. The survival rate and cholesterol levels in the gonads were measured after two days, and the concentration with the highest difference in cholesterol and survival rate was found higher than 90%. Therefore, the optimum injection concentration of cholesterol and lovastatin was 0.4 mg/mL and 0.25 mg/mL, respectively.
ELISA showed that cholesterol and testosterone levels in the embryonic gonadal of chicken significantly decreased (2.1% and 3.9%, respectively) after lovastatin injection at 6.5 days (Figure ). Cholesterol injections had the opposite effect, and ELISA showed significant increases in gonadal testosterone (5.7% and 3.2%, respectively). The results explained that cholesterol could promote the synthesis of testosterone in chicken embryo gland.
Figure 1. The expression levels of cholesterol and testosterone in chicken embryo gonads. (A) The content of cholesterol and testosterone in the gonads of chicken embryos at day 6.5. The mean ± sd. is shown; n = 3 independent experiments. P by t-test. (B) The content of cholesterol in the gonadal gland of chicken after the injection of cholesterol and lovastatin respectively. The mean ± sd. is shown; n = 3 independent experiments. P by t-test. *P < .05.
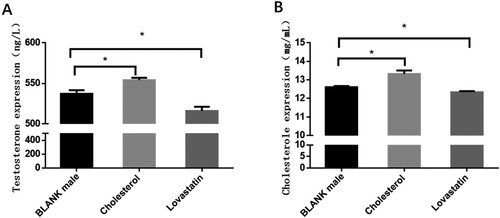
HMGCS1 is potentially the key gene associated with male differentiation of chicken embryos
In the early stage, transcriptional sequencing was carried out on male and female embryonic stem cells (ESCs), primordial germ cells (PGCs) and spermatogenic stem cells (SSCs) and oocyte stem cell (Figure (A)), and regulatory network analysis was carried out on the obtained male and female genes. According to the existing literature and the potential regulatory relationship, the potential sex-determining gene HMGCS1 on the Z chromosome was screened out.
Figure 2. Expression pattern of HMGCS1 in chicken embryo. (A) High-throughput transcriptome sequencing analyzed the expression of HMGCS1 on ESCs to PGCs and SSCs. (B) Expression of HMGCS1 in different tissues and organs of chicks just after hatching. (C) Quantitative detection of HMGCS1 expression in ESCs, PGCs and SSCs (or oocyte in the female) of female and male chicken embryos. The mean ± sd. is shown; n = 3 independent experiments. P by t-test. *P < .05.
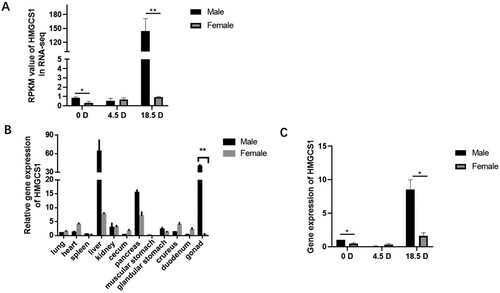
In this study, we obtained heart, liver, spleen, lung, kidney, cecum, duodenum, muscle stomach, glandular stomach, pancreas, leg muscle and gonadal tissues of male and female chickens respectively. qRT-PCR detection revealed that HMGCS1 was highly expressed in gonads. The expression level in the male testis was significantly higher than that in female ovaries (P < .01) (Figure (B)). In addition, male and female ESCs, PGCs and SSCs (oocyte stem cell in female) were isolated from the gonads of chicken embryos at 0, 4.5 and 18.5 d, respectively. We detected that in male, HMGCS1 was highly expressed in ESCs, but the expression was very low in PGCs. However, the high expression was restored in the SSCs stage, which was significantly higher than the other two stages. The expression of HMGCS1 was very low in all three types of cells in females, consistent with the sequencing results (Figure (C)). Therefore, HMGCS1 is likely to be a key candidate gene in the process of chicken embryo sexual differentiation.
Construction of HMGCS1 interference and overexpression vectors
In order to further verify the role of the HMGCS1 gene, we have separately constructed interference (sh-HMGCS1) and overexpression (OE-HMGCS1) vectors for HMGCS1, and obtained the lentivirus-mediated vector. The lentivirus-mediated vector was transfected into DF-1 cells and observed under a microscope after 72 h. Green fluorescence was observed in the cells after transfection with lentiviral vector-mediated sh-HMGCS1 and red fluorescence was observed in the cells after transfection with lentiviral vector-mediated OE-HMGCS1 (Figure (A)). RNA was extracted after sample collection, and qRT-PCR showed that the expression of HMGCS1 increased significantly after transfection of the overexpression vector (P < .05), and the expression of HMGCS1 decreased significantly after interference with HMGCS1 (P < .05) (Figure (B)). In summary, we successfully constructed the HMGCS1 interference and overexpression vectors.
Figure 3. Construction of HMGCS1 interference and overexpression vectors. (A) Fluorescence observation of DF-1 cells transfected with HMGCS1 interference (sh-HMGCS1) and overexpression vectors (OE-HMGCS1). NC stands for empty carrier. The magnification is 100×. (B) The relative expression of HMGCS1 in DF-1 cells after transfection with different vectors. The mean ± sd. is shown; n = 3 independent experiments. P by t-test. *P < .05.
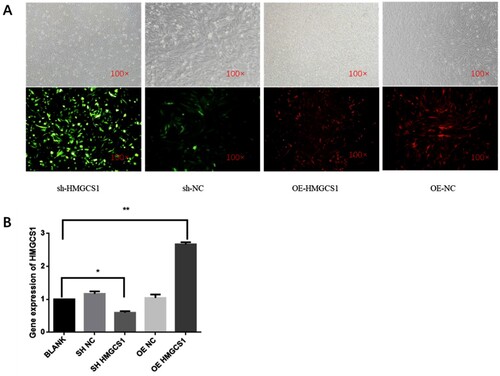
HMGCS1 promotes male differentiation in chicken embryos
In order to investigate the role of HMGCS1 in the sex differentiation of chicken embryos, gonads were collected for detection after interference and overexpression of HMGCS1 in chicken embryos. The qRT-PCR results showed that after female chicken embryos overexpressed HMGCS1, CYP11A1 and SOX9 after gonadal differentiation were up-regulated at 4.5 and 18.5 days, while the expression of DMRT1 and CYP19A1 was suppressed. After HMGCS1 interfered with male chicken embryos, CYP11A1 and SOX9 in the gonads were down-regulated on day 4.5 and 18.5, while the expression of DMRT1 and CYP19A1 was up-regulated (Figure (A,B)). The result was exactly the opposite of that in overexpression group. The above results indicated that overexpression of HMGCS1 could promote the expression of genes related to male orientation differentiation.
Figure 4. HMGCS1 promotes male differentiation in chicken embryos. (A) The expression levels of some sex-related genes (HMGCS1, CYP19A1, DMRT1, SOX9) were detected when the embryonic body interfered with and overexpressed HMGCS1 and developed to 4.5 days. F is for female and M is for male. The mean ± sd. is shown; n = 3 independent experiments. P by t-test. *P < 0.05. (B) The expression levels of some sex-related genes (HMGCS1, CYP19A1, DMRT1, SOX9) were detected when the chicken embryos interfered and overexpressed HMGCS1 and developed to 18.5 days. The mean ± sd. is shown; n = 3 independent experiments. P by t-test. *P < .05. (C) In the detection of testicular morphology after HMGCS1 overexpression and interference. The brown color is produced by immunofluorescence labeling of germ cells with DAZL antibody, the arrows indicate convoluted seminiferous tubule. (D) Quantification of figure C, the Y-axis represents the ratio of convoluted tubule area to total testicular cross-sectional area.
To further explore the effect of HMGCS1 on chicken embryo gonads, after incubation for 18.5 days, the testes were embedded in paraffin and the germ cells were labeled with DAZL immunofluorescence. In the testicular section, there were numerous dense convoluted tubules surrounded by a monolayer of cells. There was no significant difference between the testicular tissue and the control group after HMGCS1 overexpression. However, after interference with HMGCS1 expression, the convoluted seminiferous tubule in testicular sections was significantly sparse (Figure (C,D)). These results suggested that the expression level of HMGCS1 could affect the gonadal differentiation of chicken embryos.
HMGCS1 promotes testosterone synthesis in chicken embryo gonads
In order to further elucidate the regulatory mechanism of HMGCS1 on gonadal differentiation, we detected cholesterol and testosterone in the gonads of chicken embryos. Detection results explained that female chicken embryos overexpressed HMGCS1, cholesterol content fluctuated in different periods, and the expression level was higher than that of the control group (Figure (A)). And the level of testosterone in the gonads was detected to be higher than the control group (Figure (B)).
Figure 5. Changes of testis and cholesterol content in chicken embryo. (A and B) Changes in cholesterol and testosterone levels from 4.5 to 18.5 days after overexpression of HMGCS1 in female chicken embryos. C and D, Changes in cholesterol and testosterone levels from 4.5 to 18.5 days after interfering with HMGCS1 in male chicken embryos. The X axis represents different days of incubation. Error bars indicate SD.
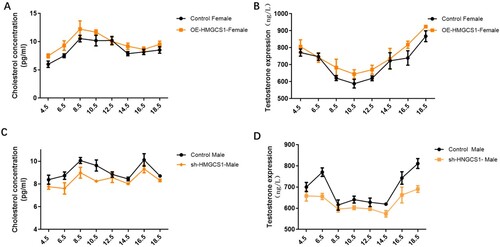
In male chicken embryos, interference with the expression of HMGCS1 leaded to the opposite expression pattern. The cholesterol level in the testes was lower than that expressed in the control group during the continuous test (Figure (C)). At the same time, the content of testosterone in the gonads was significantly higher than the control group in each test period (Figure (D)). These results suggested that HMGCS1 could promote the synthesis of cholesterol in the gonads and thus increase the content of testosterone.
Discussion
As early as 1990, Cooley et al. successfully cloned the cDNA sequence of the HMGCS1 gene in chickens, detected high expression in chicken livers, and participated in the synthesis of cholesterol enzymes in the liver (Scheib Citation1983). In this experiment, gene expression HMGCS1 was tested from different tissues and found to be the highest in chicken liver, consistent with previous studies.(Smith et al. Citation2009). In addition, we also found that HMGCS1 was highly expressed in the gonads, the expression level was only lower than that of the liver, and the expression level in male testes was significantly higher than that in female ovaries, which was consistent with high-throughput sequencing results. It indicated that HMGCS1 may play an important role in the regulation of chicken embryo male differentiation.
As a very important economic animal in birds, its sex is not only controlled by chromosomes, but also affected by some other factors. For example, the sex hormone content in the body also plays an important role in its sex differentiation process(Vock et al. Citation2008). Chicken embryonic gonads have the ability of bidirectional differentiation on the third day. After that, male chicken embryos will first develop male gonads and secrete male hormones, promoting the development of male characteristics and inhibiting the development of female characteristics. Similarly, female chicken embryos will develop their female gonads (Widenmaier et al. Citation2017). Wang confirmed that in the early stage of chicken embryo development (0–7 days of age), changing the content of estrogen and androgen in the embryo can induce the feminization or virilization of chicken embryos (Jianping et al. Citation2001). This experiment found that after the overexpression of HMGCS1, cholesterol content fluctuated in different periods and was all higher than the control group. This fluctuation may be related to the body’s feedback regulation (Chuan and He Citation2011). The testosterone content decreased significantly after interference with HMGCS1, and feminization of the testicular tissue was observed at 18.5 days. In addition, we found that the change of testosterone was consistent with trends in cholesterol. Therefore, HMGCS1 is highly likely to control the expression of testosterone by affecting cholesterol synthesis.
Unlike mammals, key SRY-like sex-determining genes have not been identified in poultry. However, many genes closely related to the sex differentiation of chicken embryos have gradually been discovered, such as the aromatase gene CYP19A1, which is a key enzyme in the process of testosterone conversion to estradiol (Jianping et al. Citation2001; Yang Citation2017). Interfering with CYP19A1 in chicken embryos inhibits the synthesis of estrogen in the gonads, leading to virilization of the gonads (Qirong and Zeyi Citation2004; Jing Citation2014; Jing et al. Citation2014). In early male chicken embryos, Smith et al. inhibited the expression of the male marker gene SOX9 after interfering with DMRT1, but activated the expression of CYP19A1, thereby reducing the testosterone content in the gonads of embryos, leading to feminization of the gonads (Yunfeng Citation2008). In our study, it was found that overexpression of HMGCS1 can inhibit the expression of aromatase CYP19A1 and increase the testosterone content in the gonad. After interference with HMGCS1, the expression of CYP19A1 significantly increased, and a decrease in testosterone content was detected, which was consistent with previous research results. In conclusion, this study verified the role of HMGCS1 in the process of gonadal differentiation, and provided a theoretical basis for subsequent studies on sex determination in poultry.
Conclusion
Through genetic analysis and functional verification experiments, this study confirmed that HMGCS1 can affect some key genes related to sex differentiation, such as SOX9, CYP19A1 and DMRT1. In addition, HMGCS1 is responsible for the synthesis of cholesterol and testosterone in the body. Therefore, the expression level of HMGCS1 can affect the differentiation and development of in chicken embryo gonads.
Acknowledgments
The authors would like to thank the research farm of poultry research institute, Chinese Academy of Agricultural Science for providing the Rugao yellow chicken eggs. The authors specially thank Huang Xiajuan, master of Zhejiang Sci-Tech University, for her language modification. Finally, the authors are very grateful to our colleagues and teachers in the laboratory for their mutual help.
Ethics declarations
All procedures involving the care and use of animals conformed to the U.S. National Institute of Health guidelines (NIH Pub. No. 85-23, revised 1996) and were approved by the Laboratory Animal Management and Experimental Animal Ethics Committee of Yangzhou University.
Data availability statement
The data that support the findings of this study are available in figshare at https://doi.org/10.6084/m9.figshare.13076942, reference number [206217573].
Disclosure statement
No potential conflict of interest was reported by the author(s).
Correction Statement
This article has been corrected with minor changes. These changes do not impact the academic content of the article.
Additional information
Funding
References
- Bruggeman V, As PV, Decuypere E. 2002. Developmental endocrinology of the reproductive axis in the chicken embryo. comp biochem physiol. Part A. 131(4):839–846.
- Chuan H, He M. 2011. Advances in molecular mechanisms of chicken sex determination and differentiation. J Agric Biotechnol. 19(6):1144–1149.
- Eacker SM., Agrawal N, Qian K, Dichek HL., Gong E-Y, Lee K, Braun RE. 2008. Hormonal regulation of testicular steroid and cholesterol homeostasis. Mol Endocrinol. 22(3):623–635.
- Elbrecht A, Smith RG. 1992. Aromatase enzyme activity and sex determination in chickens. Science. 255(5043):467–470.
- Jianping W, Youzhi P, Ning L. 2001. Sexual determination and sexual reversion of chickens. Chin Poult. 3:28–30.
- Jing X. 2014. Effect of gavage on the expression of genes related to cholesterol synthesis in goose liver. Nanjing, China: Nanjing Agricultural University.
- Jing X, Qian Y, Jinjun L, Yong T, Fang C, Lizhi L. 2014. CDNA cloning of 3-hydroxy-3-methylglutaryl coenzyme A synthase gene (HMGCS1) from lande goose and its mRNA in gut liver study on expression in tissue. J Agric Biotechnol. 22(1):79–86.
- Kashimada K, Koopman P. 2010. Sry: the master switch in mammalian sex determination. Development. 137(23):3921–3930.
- Kattar-Cooley PA, Wang HH, Mende-Mueller LM, Miziorko HM. 1990. Avian liver 3-hydroxy-3-methylglutaryl-CoA synthase: distinct genes encode the cholesterogenic and ketogenic isozymes. Arch Biochem Biophys. 283(2):523–529.
- Lambeth LS, Cummins DM, Doran TJ, Sinclair AH, Smith CA, Schubert M. 2013. Overexpression of aromatase alone is sufficient for ovarian development in genetically male chicken embryos. Plos One. 8(6):e68362.
- Matsuda M, Nagahama Y, Shinomiya A, Sato T, Matsuda C, Kobayashi T, Morrey CE, Shibata N, Asakawa S, Shimizu N, et al. 2002. DMY is a Y-specific DM-domain gene required for male development in the medaka fish. Nature. 417(6888):559–563.
- Muller U, Zenzes MT, Wolf U, Engel W, Weniger JP. 1979. Appearance of H-W (H-Y) antigen in the gonads of oestradiol sex-reversed male chicken embryos. Nature. 280(5718):142–144.
- Qirong W, Zeyi Y. 2004. Astrocytes synthetic acute regulatory protein (StAR) and testosterone biosynthesis. Chin J Sports Med. 5:591–595.
- Scheib D. 1983. Effects and role of estrogens in avian gonadal differentiation. Differentiation. 23(Suppl):S87–S92.
- Smith CA, Roeszler KN, Ohnesorg T, Cummins DM, Farlie PG, Doran TJ, Sinclair AH. 2009. The avian Z-linked gene DMRT1 is required for male sex determination in the chicken. Nature. 461(7261):267–271.
- Smith CA, Sinclair AH. 2004. Sex determination: insights from the chicken. BioEssays. 26(2):120–132.
- Vock C, Doring F, Nitz I. 2008. Transcriptional regulation of HMG-CoA synthase and HMG-CoA reductase genes by human ACBP. Cell Physiol Biochem. 22(5-6):515–524.
- Wang JL, Meng H, Jia CL, Su LH, Wei ZH. 2009. The establishment of rapid PCR sexing method by using CHD1 gene for domestic fowl (Gallus domesticus) and early chicken embryo. Poult Sci. 12:3–7.
- Widenmaier SB, Snyder NA, Nguyen TB, Arduini A, Lee GY, Arruda AP, Saksi J, Bartelt A, Hotamisligil GS. 2017. NRF1 is an ER membrane sensor that Is central to cholesterol homeostasis. Cell. 171(5):1094–1109.e15.
- Yang L. 2017. Effect of cholesterol on testosterone secretion in leydig cells of rats in vitro. Taiyuan, China: Shanxi Medical University.
- Yunfeng Z. 2008. Study on T and E on early chicken embryo gonadal differentiation and growth. Yangling, China: Northwest Agriculture & Forestry University.