Abstract
The purpose of this study was to determine the potentiality of the composting process and biological pretreatment of using P. florida to improve the nutritive value and enhance the digestibility of rice straw. A total of 40 rice straw samples with and without composting treated with P. florida at different substrate harvesting periods (full ramification, 1st, 2nd, and 3rd flushing) were evaluated by analyzing its chemical composition, in vitro digestibility, and scanning electron microscopy (SEM). Results showed that composted and uncomposted rice straw in the 2nd flush had the highest dry matter (DM) content of 96.07% and 95.56%, respectively. The highest crude protein (CP) content (10.57%) was obtained in composted rice straw in the 3rd flush. The composted rice straw treated with P. florida had low organic matter (OM) content but high concentrations of ash, cellulose, and nutrient digestibility. These results indicate that the composted rice straw in the 3rd flush was found to be the best in terms of sufficient degrading of lignin content, minimum loss in cellulose concentrations, enhanced crude protein content, and nutrient digestibility. Therefore, it is recommended to develop spent mushroom substrate feed supplements for possible use in ruminants.
Introduction
Lignocellulose is the major structural component of plants that represents a major source of renewable organic matter (Jafari et al. Citation2007). The chemical properties of the lignocellulose components such as cellulose, hemicelluloses, and lignin make them a substrate of enormous biotechnological value (Malherbe and Cloete Citation2002). In the Philippines, agricultural lignocellulosic residues such as rice straw are renewable resources for the production of mushroom and ruminant feeds. However, low protein, high silica content and poor digestibility of rice straw limit its use in the diets of ruminants (Baldrian and Gabriel Citation2003).
Several methods such as chemical and physical treatments have been researched and developed to improve the utilization of rice straw and other fibrous by-products as feeds for ruminant or to produce mushroom as human food with varying degree of success (Sarnklong et al. Citation2010). However, they are not only costly but also potential pollutants to the environment (Dashtban et al. Citation2009). Hence, biological pretreatment using microorganisms such as bacteria and fungi has been considered as a promising method that can facilitate the conversion of lignocellulose into sugars or other products without using harsh chemicals and high temperature (Madadi and Abbas Citation2017).
The effective biological delignification relies on ligninolytic enzymes presented by basidiomycetes such as lignin peroxidase, manganese peroxidase and laccase (Kumar et al. Citation2009; Binod et al. Citation2011; Andberg et al. Citation2015). These basidiomycetes are the important identified white-rot fungi, which are regarded as environmentally friendly and economically viable alternatives (Tuyen et al. Citation2013; Machado and Ferraz Citation2017). Oyster mushrooms (Pleurotus florida) are edible fungi, which belong to class basidiomycetes could improve the degradation of cellulose and hemicellulose of plant residues (Mondal et al. Citation2011) that seem to be a promising way for improving nutritive value (Fazaeli Citation2007) and enhancing nutrient digestibility of the fibrous substrate (Zadrazil Citation1997). Thus, this study aimed to determine the potentiality of biological pretreatment of using P. florida to improve the nutrient composition and enhance the digestibility of rice straw at different harvesting periods. This study also aimed to assess the morphological changes on the lignocellulose structure of the rice straw.
Materials and methods
Preparation of rice straw
Dried rice straw was soaked in water for three days and washed continuously with tap water (rice straw without composting). The substrates were piled and covered with plastic to create anaerobic conditions and to ensure the growth of natural decomposers. The piled rice straw was turned every day for three days until they became pliable and turned into dark brown (rice straw with composting).
Formulation and treatment of rice straw-based substrate
The following treatments of rice straw-based substrate were chopped manually to approximately 2–3 cm in length. The substrate was formulated as 90% rice straw + 10% rice bran and assigned in (2 × 4) two-factor factorial design in completely randomized design (CRD) with five replicates:
Factor A: Preparation of rice straw
Rice straw with composting
Rice straw without composting
Factor B: Rice straw treated with P. florida at different substrate harvesting periods
full mycelial ramification (27 days)
1st flush (4 days from bag opening to flushing)
2nd flush (14 days from bag opening to flushing)
3rd flush (21 days from bag opening to flushing)
Source of fungal culture and inoculant
Pure culture of Pleurotus florida was obtained from the culture collections of the Center for Tropical Mushroom Research and Development (CTMRD) in Central Luzon State University (CLSU), Science City of Munoz, Nueva Ecija, Philippines. This was aseptically revived into sterilized potato dextrose agar (PDA) plates and incubated at room temperature for seven days. Mycelial discs were prepared using sterile cork borer (10 mm diameter) as inoculants.
Fungal treatment and mushroom production
Fungal treatment and mushroom production were performed following the procedure developed by the CTMRD in CLSU.
Sampling of rice straw-based substrate at different harvesting period
A total of 40 fully ramified substrate samples were opened and incubated at the incubation area until flushing. The sampling of substrate samples was done during full ramification up to the 3rd flush of mushroom.
Pre-drying and grinding of samples for laboratory analysis
Untreated and treated rice straw were spread on a tray and dried at 60°C for three days using a drying oven. The oven-dried samples were ground using a cyclone mill to produce a fine sample that could pass through 1 millimeter-mesh. The ground samples were used for different chemical analyses.
Chemical analyses
All samples were oven-dried immediately at 70°C for 48 h followed by drying at 135°C for two hrs to determine the dry matter (DM) content. The content of ash, crude protein (CP), ether extract (EE), and crude fiber (CF) was analyzed according to the official methods of the AOAC (Citation1995). The acid detergent fiber (ADF), neutral detergent fiber (NDF), cellulose, hemicellulose, and acid detergent lignin (ADL) or lignin were evaluated following the procedure of Van Soest (Citation1973) and Van Soest et al. (Citation1991).
In vitro digestibility
The effect of fungal treatment was evaluated using in vitro digestibility. The in vitro incubation was performed according to the standard procedure of Tilley and Terry (Citation1963). Triplicate samples of 0.5 g of each feed were placed in a centrifuge tube of polypropylene (NALGENE) of 100 ml. Three blank tubes (without sample) were used in each run. To each sample, 50 ml of a mixture of Mcdougall’s buffer solution and ruminal fluid was added, then bubbled with CO2 until saturation. The tubes were placed later on in a tube rack and incubated to 39°C for 48 hrs. All the samples were vigorously shaken every 12 h. After the incubation period, 1.5 ml of acid pepsin solution was added to each tube to avoid foam and later, 3.5 ml of the same solution were added and incubated for 48 hrs. Lastly, the samples were filtered (Whatman No. 5) by a vacuum pump, and the residues were weighed and the digestibility was calculated following the conventional procedure.
Morphological structure of rice straw
The morphological changes in the structure of untreated and treated rice straw were observed through a scanning electron microscope (SEM) (JSM-5310; JEOL, Tokyo) following the procedure described by Goldstein et al. (Citation2003) for capturing SEM image.
Statistical analysis
Data of preparation of rice straw were analyzed by t-test, whereas, data of rice straw treated with P. florida were assigned in two-factor factorial design in a completely randomized design (CRD) and analyzed using analysis of variance (ANOVA). Significant differences of means were compared using Tukey’s HSD test at a 5% level of significance using SPSS and R Statistics software.
Results and discussion
Chemical composition and in vitro digestibility of composted and uncomposted rice straw
Table shows the chemical composition of composted and uncomposted rice straw. Significantly higher (P < 0.05) ash, CP, and silica content (23.26 vs 19.92, 5.34 vs 4.40 and 20.01 vs 17.07%, respectively) were observed in composted rice straw than uncomposted rice straw. However, significantly lower (P < 0.05) levels of OM, CF, NDF, and hemicellulose concentrations (76.74, 35.90, 69.27, and 8.76%, respectively) were also observed in composted rice straw. On the other hand, Table shows the in vitro digestibility of composted and uncomposted rice straw. Significantly higher (P < 0.05) in vitro acid detergent fiber (IVADFD) was observed in composted rice straw (50.10%) than uncomposted rice straw (47.63%). However, no significant difference (P > 0.05) was revealed in other nutrient digestibility estimates.
Table 1. Chemical composition of composted and uncomposted rice straw.
Table 2. In vitro digestibility of composted and uncomposted rice straw.
During the composting period as well as the chemical composition of the rice straw changed. In this study, higher CP content was observed in composted rice straw than uncomposted rice straw. The increase in CP content during the composting of rice straw may be due to the presence of microorganisms and extracellular enzymes (Ball and Jackson Citation1995; Khattab et al. Citation2013), which hydrolyzed structural and soluble carbohydrates for microbial biomass synthesis that are rich in protein. The composting process also affects the ash and silica content of the rice straw, which is higher than the rice straw without composting. The increase in ash and silica may be due to the breakdown of the silicates in lignified structures hence, releasing silica in free form (Phutela et al. Citation2012) and therefore increasing overall ash content. Morover, the composting process could decrease the OM and structural carbohydrates such as CF, NDF, and hemicellulose concentrations of rice straw which might be caused by the enzymatic action of microorganisms secreting fiber degrading enzymes (Arora et al. Citation2002) and therefore, improved the nutrient composition of the rice straw. On the other hand, the rigidity of lignin and its complexes is one of the major barriers preventing microbial enzymes digesting the cell wall carbohydrates in rice straw (Huyen et al. Citation2019b) that result in lower digestibility. In addition, composting and hydrolysis of structural carbohydrates in rice straw was relatively beneficial to make it more readily available for the action of rumen microorganisms, hence, improving the digestibility of the ADF in rice straw (Mirzaei et al. Citation2007).
Chemical composition of rice straw treated with P. florida
The chemical composition of rice straw was significantly affected (P < 0.05) by the interaction of composted and uncomposted rice straw treated with P. florida and the substrate harvesting periods (Table ). In this study, results showed that composted and uncomposted rice straw in the 2nd flush had the highest DM content of 96.07 and 95.56%, respectively. The highest CP content (10.57%) was obtained in composted rice straw in the 3rd flush followed by 2nd flush (9.70%). However, the fully ramified uncomposted rice straw had the lowest CP content (6.98%). The highest concentrations of EE were observed in uncomposted rice straw in the 2nd and 3rd flushes (3.39 and 3.53%, respectively). The highest CF content of 28.71% was observed in fully ramified uncomposted rice straw. Whereas, highest NDF and ADF content of 69.26 and 63.04%, respectively were obtained from the fully ramified composted rice straw. Noticeably, the composted and uncomposted rice straw in the 3rd flush had the lowest level of CF, NDF, and ADF (9.26 & 10.83, 55.85 & 56.19, and 52.27 & 49.87%, respectively). It was also noticed that the highest ADL content was obtained in fully ramified composted and uncomposted rice straw (13.16 & 11.81%, respectively). However, the lowest level of ADL was revealed in composted and uncomposted rice straw in the 3rd flush. The highest hemicellulose content (11.02%) was observed in fully ramified uncomposted rice straw. The highest silica content was observed in composted rice straw in the 2nd and 3rd flushes (32.85 and 31.92%, respectively) and uncomposted rice straw in the 3rd flush (33.10%).
Table 3. Interaction of the chemical composition of composted and uncomposted rice straw treated with P. florida and different substrate harvesting periods.
On the other hand, Table shows the main effects of the application of P. florida in composted and uncomposted rice straw and its different substrate harvesting periods. It showed that the OM content of the uncomposted rice straw was significantly higher (68.64%) than the composted rice straw (62.63%). A low level of OM concentration of rice straw was revealed in the 3rd flush (58.92%). Higher ash content was observed in composted rice straw (37.37%) compared to uncomposted rice straw (31.36%). The highest ash content (41.08%) of rice straw was obtained in the 3rd flush. The higher cellulose content was observed in composted rice straw (46.54%) than the uncomposted rice straw (43.25%).
Table 4. Chemical composition of composted and uncomposted rice straw treated with P. florida and its substrate harvesting period.
The chemical composition of composted and uncomposted rice straw treated with P. florida presented in this study showed that fungal growth was rapid when substrates from rice straw were high at the start (full ramification), thus, the increase in DM content. But after successive harvest on the late mushroom flush, substrates were depleted and relied on the recycled substrates and from the extensive mycelia that were formed previously (Mukherjee and Nandi Citation2004; Nasehi et al. Citation2017). Khattab et al. (Citation2013) explained that fungus obtains their requirements from the decaying OM, in particular, the lignocellulolytic constituents, lack of carbon and energy supply from the lignin, would prompt the fungi to utilize substrates such as cellulose or other carbon sources from delignification for their growth; and thus, resulted in the decrease in OM and increase in ash content (Ruggeri and Sassi Citation2003). The fungal treatment and extend the number of flushing also increased the CP, ash, and EE contents of the composted and uncomposted rice straw. Such an apparent increase could be due to the hydrolysis of carbohydrates and uses this carbon source to synthesize fungal biomass that rich in protein (Akinfemi et al. Citation2010). In addition, an increased in CP and EE especially during 3rd flushing may be due to the proliferation of fungi, which capture carbon and nitrogen sources in rice straw resulting in lipid accumulation (Azad et al. Citation2014; Nasehi et al. Citation2017). This agrees with the report published by Zadrazil et al. (Citation1996) and Huyen et al. (Citation2019a), who noted that this was attributed to the proliferation of fungal cells during the degradation of substrates. The degradation of structural carbohydrates such as CF, NDF, ADF, ADL, cellulose, and hemicellulose may be due to the extensive hyphae of the fungus that are capable of penetrating deep into the lignocellulosic matrix of the straw (Akinfemi and Ogunwole Citation2012). This means that P. florida does not only thrive on the surface of the substrate but its hyphae invasive action destroys lignified layers of the substrate. Furthermore, the decrease in the amount of NDF and ADF of rice straw treated with P. florida is indicative that there is a rapid degradation of the cell wall component of lignocellulosic materials of the substrates as a result of extracellular enzymes such as laccase, cellulase, xylanase, and glucosidase of the fungus (Taniguchi et al. Citation2005; Akinfemi et al. Citation2010). The decrease in the concentrations of cellulose was also continuously observed until 3rd flushing. Apparently, white-rot fungi at the primary growth phase degrade a higher proportion of digestible polysaccharides which consume lignin and hemicellulose relatively more than cellulose. Then, in the second growth phase, these fungi intend to degrade cellulose more than hemicellulose and lignin (Zadrazil et al. Citation1996). The fungal treatment also affected the silica content of composted and uncomposted rice straw during the substrate harvesting period. This study conformed with the study of Phutela et al. (Citation2012) who supported that silica continuously increases as the time of incubation passes.
In vitro digestibility of rice straw treated with P. florida
Figure shows the in vitro digestibility of composted and uncomposted rice straw treated with P. florida. The higher IVDMD, IVOMD, IVCPD, IVNDFD, and IVADFD (62.54, 66.14, 61.36, 58.18 and 54.39%, respectively) were observed in composted rice straw than the uncomposted rice straw (61.54, 63.01, 60.21, 56.07, 52.35%, respectively) (P < 0.05). On the other hand, Figure shows the in vitro digestibility of rice straw treated with P. florida at different substrate harvesting periods. An increase in trend on the nutrient digestibility was observed in fully ramified rice straw until 2nd flush but the values decreased during the 3rd flush. It is noted that the highest values of IVDMD, IVOMD, IVCPD, IVNDFD, and IVADFD (64.30, 67.0, 63.42, 60.67 and 56.09%, respectively) of rice straw were found in the 2nd flush; lowest values (59.72, 62.30, 58.60, 53.96 and 50.85%, respectively) were observed in fully ramified rice straw.
Figure 1. The main effects of P. florida on the in vitro digestibility of composted and uncomposted rice straw.
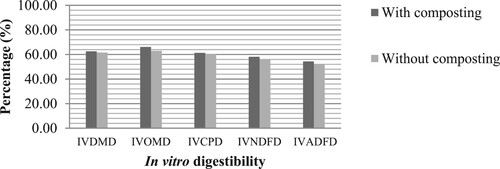
Figure 2. In vitro digestibility of rice straw treated with P. florida during different substrate harvesting periods.
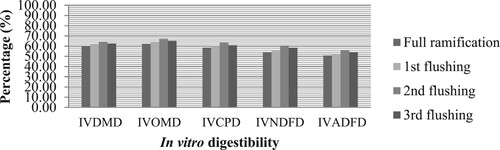
The increase in the nutrient digestibility of rice straw treated with Pleurotus florida may be due to the continuous degradation of lignocellulosic materials or fractions such as cellulose, hemicelluloses, and lignin, which is probably associated with a reduction in cell wall components and an increase in CP content of substrates (Sharma and Arora Citation2010). Lignin binds with hemicellulosic components of the cell wall, and through covalent linkages and physical binding, prevents the biodegradation of straw carbohydrates by microorganisms (Eriksson et al. Citation1990). These findings were supported by some in vitro studies (Zadrazil Citation1997; Fazaeli Citation2007). In addition, Jung and Allen (Citation1995) stated that lignin content is one of the major barriers preventing microorganism enzymes to digest nutrients in rice straw, however, fungi can delignify the rice straw resulting in changes in the cell wall structure, removal of lignin, and a subsequent opening of structural fibers that make it more readily available to rumen microorganism. Therefore, this could lead to an increase in rice straw nutrient digestibility (Arora et al. Citation2002). However, a decrease in nutrient digestibility during 3rd flushing may be possibly related to many factors such as silica content of rice straw and fungus growth stage influence cell wall degradation and their digestibility (Jalc et al. Citation1996). In addition, Khattab et al. (Citation2013) stated that silica has been shown to exert an antimicrobial effect on the rumen microbes, which inhibit cellulolytic microbes and thus reduce the digestion of plant cell walls.
Morphological changes on the structure of rice straw
Figure shows the morphological structure of composted and uncomposted rice straw via a scanning electron microscope. The structure of composted and uncomposted rice straw was seen intact with phytoliths (P showed by arrow). These phytoliths make the straw surface less wettable and add resistance against microbial attack (Kaur and Phutela Citation2018).
Figure 3. Scanning electron micrograph of rice straw (RS). [A] Composted RS (2000X); [B]: Uncomposted RS (1500X); P showed by arrow means phytoliths.
![Figure 3. Scanning electron micrograph of rice straw (RS). [A] Composted RS (2000X); [B]: Uncomposted RS (1500X); P showed by arrow means phytoliths.](/cms/asset/8874ebea-efe9-44d8-9a3e-e0979329ce96/tfls_a_1947392_f0003_oc.jpg)
On the other hand, Figure shows the scanning electron micrograph of composted rice straw treated with P. florida at different substrate harvesting periods. In Figure [A], fungal mycelia (FM shown by arrow) colonized the lignocellulose of the rice straw, whereas, Figure [B]-[D] looked disruptions on the lignocellulose such as cellulose, hemicelluloses, and lignin of the rice straw. Figure [D] shows extensive perforations (Pe showed by arrow) on the various layers of lignocellulose of the rice straw. Similarly, Figure shows the scanning electron micrograph of uncomposted rice straw treated with P. florida at different substrate harvesting periods. In Figure [A], fungal mycelia (FM showed by arrow) colonized the lignocellulose of the rice straw, whereas, Figure [B–D] manifest disruptions on the lignocellulose such as cellulose, hemicelluloses, and lignin of the rice straw. Figure [D] shows several areas of perforations (Pe showed by arrow) on the lignocellulose of the rice straw.
Figure 4. Scanning electron micrograph of composted rice straw treated with P. florida at different substrate harvesting period. [A]: Full ramification (1000X); [B]: 1st flush (1500X); [C]: 2nd flush (1500X); [D]: 3rd flush (1500X); FM-fungal mycelia; Pe-perforations.
![Figure 4. Scanning electron micrograph of composted rice straw treated with P. florida at different substrate harvesting period. [A]: Full ramification (1000X); [B]: 1st flush (1500X); [C]: 2nd flush (1500X); [D]: 3rd flush (1500X); FM-fungal mycelia; Pe-perforations.](/cms/asset/c2b7b298-6081-484d-aed3-0b1a12a6a41f/tfls_a_1947392_f0004_oc.jpg)
Figure 5. Scanning electron micrograph of uncomposted rice straw treated with P. florida at different substrate harvesting periods. [A]: Full ramification (2000X); [B]: 1st flush (2000X); [C]: 2nd flush (1000X); [D]: 3rd flush (1500X); FM-fungal mycelia; Pe-perforations.
![Figure 5. Scanning electron micrograph of uncomposted rice straw treated with P. florida at different substrate harvesting periods. [A]: Full ramification (2000X); [B]: 1st flush (2000X); [C]: 2nd flush (1000X); [D]: 3rd flush (1500X); FM-fungal mycelia; Pe-perforations.](/cms/asset/a43215a1-6dbd-4a6e-ad03-837edb86150c/tfls_a_1947392_f0005_oc.jpg)
The disruptions of lignocellulose of the rice straw may due to the fungal hyphae that penetrate deep into the substrate. The fungal hyphae of P. florida were found penetrating into the cell wall of the rice straw which resulted in the degradation of lignin and enhanced digestibility (Kaur and Phutela Citation2018). The extensive perforations on the lignocellulose of rice straw may be due to the various lignolytic enzymes such as lignin peroxidases, laccases, manganese peroxidases, and hydrogen peroxide-producing enzymes, involved in catalyzing oxidative reaction by Pleurotus species (Fitria Citation2008). These enzymes are of great importance because they degrade the lignin components of lignocellulosic material, which acts as a barrier for any solution or enzyme by linking to both cellulose and hemicellulose, thus prevents penetration of lignolytic enzymes to the interior lignocellulosic structure (Breen and Singleton Citation1999).
Conclusion
Composting process and fungal pretreatment using P. florida of rice straw can increase the decomposition of organic matter which is essential for the degradation of lignocellulose such as cellulose, hemicellulose, and lignin, hence, improve chemical composition and enhance nutrient digestibility. Based on the results generated in this study, it is concluded that the composted rice straw in 3rd flush was found to be the best in terms of sufficient degrading of lignin content, minimum loss in cellulose concentrations, enhanced crude protein content, and nutrient digestibility. In addition, composted rice straw treated with P. florida, can have extensive disruptions on the morphological structure of the lignocellulosic component, especially during 3rd flush. Therefore, it is suggested to develop spent mushroom substrate feed supplements for possible use in yielding ruminants.
Acknowledgement
The authors would like to extend their sincerest gratitude to the Research for Development Division and Production Systems and Nutrition Section staff of the Philippine Carabao Center, and to the to the technical staff of the Center for Tropical Mushroom Research and Development (CTMRD) in Central Luzon State University for their unrelenting support during the conduct of this study.
Data availability statement
The data that support the findings of this study are openly available in figshare.com at https://doi.org/10.6084/m9.figshare.14740422.v1.
Disclosure statement
No potential conflict of interest was reported by the author(s).
Additional information
Funding
References
- Akinfemi A, Adu OA, Doherty F. 2010. Conversion of sorghum stover into animal feed with white-rot fungi: Pleurotus ostreatus and Pleurotus pulmunarius. Afr J Biotechnol. 9(11):1706–1712.
- Akinfemi A, Ogunwole OA. 2012. Chemical composition and in vitro digestibility of rice straw treated with Pleurotus ostreatus, Pleurotus Pulmonarius and Pleurotus tuber-regium. Slovak J Anim Sci. 45:14–20.
- Andberg M, Penttila M, Saloheimo M. 2015. Swollen in from Trichoderma reesei exhibits hydrolytic activity against cellulosic substrates with features of both endoglucanases and cellobiohydrolases. Bioresour Technol. 181:105–113.
- AOAC. 1995. Official methods of analysis. 16th ed. Arlington, VA: Association of Official Analytical Chemists.
- Arora DS, Chander M, Gill PK. 2002. Involvement of lignin peroxidase, manganese peroxidase and laccase in degradation and selective lignolysis of wheat straw. Int Biodeterioration Biodegradation. 50(2):115–120.
- Azad AK, Yousuf A, Ferdoush A, Hasan MM, Karim MR, Jahan A. 2014. Production of microbial lipids from rice straw hydrolysates by Lipomyces staryi for biodiesel synthesis. J Microb Biochem Technol. 6(7):1–6.
- Baldrian T, Gabriel J. 2003. Lignocellulose degradation by Pleurotus ostreatus in the present of cadmium. FEMS Microbiol Lett. 220(2):235–240.
- Ball AS, Jackson AM. 1995. The recovery of lignocellulose degrading enzymes from spent mushroom compost. Bioresour Technol. 54(3):311–314.
- Binod P, Janu KU, Sindhu R, Pandey A. 2011. Hydrolysis of lignocellulosic biomass for bioethanol production. Biofuels Altern Feed Convers Process. 229–250.
- Breen A, Singleton FL. 1999. Fungi in lignocellulose breakdown and biopulping. Curr Opin Biotechnol. 10(3):252–258.
- Dashtban M, Schraft H, Qin WS. 2009. Fungal bioconversion of lignocellulosic residues: opportunities and perspectives. Int J Biol Sci. 5(6):578–595.
- Eriksson KE, Blanchette RA, Ander P. 1990. Microbial and enzymatic degradation of wood and wood components. Berlin: Springer-Verlag; p. 407.
- Fazaeli H. 2007. Nutritive value index of treated wheat straw with Pleurotus fungi. Biotechnol Anim Husbandry. 23(5-6):169–180.
- Fitria F. 2008. Processing of Lignocellulosic biomass for pulp making for pulp making: a review. J Teknologi Pertanian. 9(12):69–74.
- Goldstein J, Newbury D, Michael JR, Ritchie NW, Scott JHJ, Joy DC. 2003. Scanning electron microscopy and X-ray microanalysis. 3rd ed. New York, NY: Springer.
- Huyen NT, Le NT, Tuan BQ. 2019a. Fermenting rice straw with the fungus Pleurotus eryngii increased the content of crude and the digestibility of the straw. Livestock Res Rural Devel. 31(2).
- Huyen NT, Tuan BQ, Nghien NX, Thuy NT, Le T. 2019b. Effect of using fungal treated rice straw in sheep diet on nutrients digestibility and microbial protein synthesis. Asian J Anim Sci. 13:1–7.
- Jafari MA, Nikkhah A, Sadeghi AA, Chamani M. 2007. The effect of Pleurotus spp. fungi on chemical composition and in vitro digestibility of rice straw. Pak J Biol Sci. 10(15):2460–2464.
- Jalc D, Nerud F, Erbanová P, Siroka P. 1996. Effect of white-rot basidiomycetes-treated wheat straw on rumen fermentation in the artificial rumen. Reprod Nutr Dev. 36:263–270.
- Jung HG, Allen MS. 1995. Characteristics of plant cell walls affecting the intake and digestibility of forages by ruminants. J Anim Sci. 73(9):2774–2790.
- Kaur K, Phutela UG. 2018. Morphological and structural changes in paddy straw influenced by alkali and microbial pretreatment. Detritus. 1(3):30–36.
- Khattab HM, Gado HM, Salem AZM, Camacho LM, El-Sayed MM, Kholif AM, El-Shewy AA, Kholif AE. 2013. Chemical composition and in vitro digestibility of Pleurotus ostreatus spent rice straw. Anim Nutr Feed Technol. 13:507–516.
- Kumar R, Mago G, Balan V, Wyman CE. 2009. Physical and chemical characterizations of corn stover and poplar solids resulting from leading pretreatment technologies. Bioresour Technol. 100(17):3948–3962.
- Machado AD, Ferraz A. 2017. Biological pretreatment of sugarcane bagasse with basidiomycetes producing varied patterns of biodegradation. Bioresour Technol. 225:17–22.
- Madadi M, Abbas A. 2017. Lignin degradation by fungal treatment: a review. J Plant Pathol Microbiol. 8(2):1–6.
- Malherbe S, Cloete TE. 2002. Lignocellulose biodegradation: fundamentals and applications: a review. Environ Sci Biotechnol. 1:105–114.
- Mirzaei J, Tabari M, Daroodi H. 2007. The effect of Pleurotus spp. fungi on chemical composition and in vitro digestibility of rice straw. Pak J Biol Sci. 10(115):2460–2464.
- Mondal SR, Rehana MJ, Noman MS, Adhikary SK. 2011. Comparative study on growth and yield performance of oyster mushroom (Pleurotus florida) on different substrates. J Bangladesh Agric Univ. 8(2):213–220.
- Mukherjee R, Nandi B. 2004. Improvement of in vitro digestibility through the biological treatment of water hyacinth biomass by two Plerotus species. Int Biodeterioration Biodegradation. 53(1):7–12.
- Nasehi M, Torbatinejad NM, Zerehdaran S, Safaie AR. 2017. Effects of solid-state fermentation by oyster mushroom (Pleurotus florida) on the nutritive value of some agro by-products. J Anim Res. 45(1):221–226.
- Phutela UG, Kaur K, Gangwar M, Khullar NK. 2012. Effect of Plerotus florida on paddy straw digestibility and biogas production. Int J Life Sci. 6(1):14–19.
- Ruggeri B, Sassi G. 2003. Experimental sensitivity analysis of trickle bed bioreactor for lignin peroxidases production by Phanerochaete chrysosporium. Process Biochem. 38:1669–1676.
- Sarnklong C, Cone JW, Pellikaan W, Hendriks WH. 2010. Utilization of rice straw and different treatments to improve its feed value for ruminants: a review. Asian-Australas J Anim Sci. 23(5):680–692.
- Sharma RK, Arora DS. 2010. Changes in biochemical constituents of paddy straw during degradation by white-rot fungi and its impact on in vitro digestibility. J Appl Microbiol. 109(2):679–686.
- Taniguchi M, Suzuki H, Hoshino K, Watanabe D, Sakai K, Tanaka T. 2005. Evaluation of pretreatment with Pleurotus ostreatus for enzymatic hydrolysis of rice straw. J Biosci Bioeng. 100(6):637–643.
- Tilley JMA, Terry RA. 1963. A two-stage technique for the in vitro digestion of forage crops. Grass Forage Sci. 18:104–111.
- Tuyen DV, Phuong HN, Cone JW, Baars JJ, Sonnenberg AS, Hendriks WH. 2013. Effect of fungal treatments of fibrous agricultural by-products on chemical composition and in vitro rumen fermentation and methane production. Bioresour Technol. 129:256–263.
- Van Soest PJ. 1973. Collaborative study of acid detergent fiber and lignin. J Assoc Anal Chem. 56:781–784.
- Van Soest PJ, Robertson JB, Lewis BA. 1991. Methods of dietary fiber, neutral detergent fiber, and non-starch carbohydrates in relation to animal nutrition. J Dairy Sci. 74:3583–3597.
- Zadrazil F. 1997. Changes in in vitro digestibility of wheat straw during fungal growth and after harvest of oyster mushrooms (Pleurotus spp.) on laboratory and industrial scale. J Anim Res. 11:37–48.
- Zadrazil F, Kamra ND, Isikhuemhen OS, Schuchardt F, Flachowsky G. 1996. Bioconversion of lignocellulose into ruminal feed with white-rot fungi. J Appl Anim Res. 10:105–124.