Abstract
Novel COVID-19 is the most considerable health threat the humanity has faced in decades, with global impact also in the social and economic scopes. Moreover, SARS-CoV-2 involves an unprecedented exciting scientific challenge that has focused all efforts on defeating the new coronavirus. Research results are continuously increasing and updating knowledge about the virus and the disease, and understanding the virus characteristics proves essential in order to identify and attack its weak points, as well as uncovering the host reactions to search for treatments. Through this survey, we will offer the reader a thorough exposition on how SARS-CoV-2 infects and affects the human organism, the wide set of risk factors that impact the susceptibility to and the course of the disease, related biomarkers, and potential drugs and treatments against the virus-host entry, the infection and its consequences. What has been learned over one and a half year is expected to help in facing future global health threats.
Introduction
If you were an external observer of the Earth, you would perceive that the power of nature and the human mind rule the world. The current COVID-19 pandemic implies a fascinating time and health pressing complex challenge for both and some imbalance that favors nature. At least up to now.
SARS-CoV-2 is a novel Betacoronavirus (Romano et al. Citation2020) from the Coronaviridae family, responsible of the COVID-19 disease, that was first informed by the WHO in early 2020 and is currently active representing a global health threat in the form of pandemic. The sudden appearance of SARS-CoV-2, its incredibly fast spread across the planet, and the lack of specific treatments for COVID-19 or vaccines to prevent the disease along 2020 has originated a huge worldwide impact (COVID-19 Dashboard by the Center for Systems Science and Engineering (CSSE) at Johns Hopkins University (JHU) Citation2021) in terms of human lives loss – which by August 2021 exceeds a dreadful four million – of people affected by the disease, and, consequently, of an unprecedented international economic crisis that is shaking the foundation of our civilization in the twenty-first century.
Only several months after the COVID-19 pandemic appearance, there are some issues that we certainly know, many that are continuously being discovered, but still others yet to be uncovered that prevent us from definitely knocking down this nightmare. We know for sure we are facing a virus which is a close relative of former severe acute respiratory syndrome coronavirus (SARS-CoV), that infects us through our respiratory tract, entering the organism via our mouth or nose, and that spreads quickly and easily with a relatively low lethality rate. We also know that many infected never develop any symptom (asymptomatic), that elderly people are more severely affected, males to a large extent, and that rarely children are seriously impacted. We have discovered that SARS-CoV-2 is able to infect through the air, by droplets but also aerosols expelled when breathing, talking and principally shouting and singing, mainly in poorly ventilated indoor environments. That some previous pathologies are associated with the worst prognosis, such as cardiovascular diseases (mostly hypertension), obesity or diabetes, as well as some biomarkers and genetic factors. That the majority of deaths are caused by an inadequate overreacted immune response to the pathogen. And not only the lungs but many other organs are affected and suffer from inflammation and severe vascular problems, not to mention COVID-19 persistent symptoms. That old well-known elementary physical measures such as hands washing, face masks, and social distance do help in avoiding infection or spreading of the virus when strictly implemented. That though diagnostic tests are rapidly evolving, they have room for improvement in sensitivity, in auto diagnosis capabilities and price, so that they can constitute a pivotal role in early isolating the infected and stopping the spread of the disease. That the non-adoption of security measures pursuing to reach a herd or population immunity, at the cost of many lives in some kind of natural selection process is not scientifically supported and has proven to be a resounding failure in countries like Sweden.
Non-pharmaceutical interventions (NPIs) have been implemented worldwide trying to narrow down the spread of the SARS-CoV-2 transmission reflected on the time-varying reproduction number Rt, which is defined as the expected number of secondary cases appearing from a primary case infected at time t. If Rt value exceeds the unity we face a rising outbreak, otherwise the outbreak contracts. Several studies have assessed the effectiveness of a variety of NPIs, frequently adopted in parallel, against the pandemic expansion. Data throw evidence on the advantage of school/university and workplace closure, internal movement restrictions, and gathering control (especially when limited to a maximum of ten people). Closing catering industries seems to show a moderate effect. International travel restrictions have limited effect if not strictly implemented. The impact of other NPIs such as public transport closure, testing, and contact tracing has not been found conclusive. In any case, the effectiveness of any NPI is tightly dependent on its relative moment of adoption with respect to Rt (Haug et al. Citation2020; Askitas et al. Citation2021; Brauner et al. Citation2021; Li et al. Citation2021c; Liu et al. Citation2021b).
And there is still no silver bullet treatment against COVID-19, but the long-awaited vaccine is here (Baden et al. Citation2020; Flanagan et al. Citation2020; Krammer Citation2020; Polack et al. Citation2020; Logunov et al. Citation2021; Novavax COVID-19 Vaccine Demonstrates 89.3% Efficacy in UK Phase 3 Trial January 28 Citation2021; Sadoff et al. Citation2021; Voysey et al. Citation2021). Early in the pandemic, several possible antiviral treatments were expected to avoid severe COVID-19 complications and even fatal outcomes in the short term, what could have saved thousands of lives. Nevertheless, none of them has demonstrated significant efficacy up to now; moreover, they would have neither been able to stop the course of the pandemic (contagions) nor avoid the unexpected subsequent complications that may appear even in mild or asymptomatic patients. Therefore, the vaccine against COVID-19 is indispensable. As of December 2020, several pharmaceutical companies had already developed their vaccines in record time of less than one year. This is a really impressive achievement and will surely constitute a scientific milestone that will trigger a new era for the development of vaccines to fight other critical diseases. However, the end is near but not so straightforward (aside from phase IV clinical trial results): most of the world population needs to be vaccinated – with a percentage that depends on the contagiousness of the dominant variants – to theoretically reach the herd immunity and successfully finish the pandemic (with all the broad implications this requirement poses), but also to avoid potentially dangerous SARS-CoV-2 mutations that may arise if the virus is widely spread, and that could even affect the effectivity of the vaccine. This herd immunity would only be achieved with sterilizing vaccines that protect the vaccinated from infection and therefore also the non-vaccinated: transmission of the infection might not be avoided with an intramuscular vaccine since mucosal immunity is not ensured in all cases (Krammer Citation2020); moreover, sterilization may be jeopardized due to decreased immune response and viral evolution over time. Reinfected vaccinated (with a non-sterilizing solution) individuals could still transmit the infection but to a lower extent, during a narrow transmission window that spans from infection until neutralization by a quick immune response that has previously faced the antigen. In the end, it is a matter of probability: of contagion, of transmission, of severity, of vaccine side effects, of vaccine effectiveness, of virus mutations, etc. and this game of probabilities has a direct impact on people’s behavior and in turn again on the pandemic evolution.
Knowledge about the virus and the disease is continuously growing and evolving, with some studies and trials even offering contradictory results, so our vision of the problem should not be strict but open to a new hypothesis, suggestions, and heterodox discussion. In this paper, we will try to dissect the challenging problem that SARS-CoV-2 constitutes from a scientific point of view, offering an extensive and referenced review with the aim of shedding light over questions we all have in mind, and also over some deeper queries. With the hope that what we are learning during this extreme situation helps to better confront future global health problems.
The problem statement
Elderly affected by a more severe COVID-19 fall within what could be expected. But why do male patients present higher rates of critical illness and lethality than females? Why do children providentially barely suffer severe COVID-19 unlike what happens with other common cold coronaviruses or even influenza? What triggers the cytokine storm? What is the cross-reactivity and how can it affect the disease? What kind of drugs are being tested against the viral infection and the overreacted immune response? Are virus mutations and their effects predictable? Is there a reason for the counterintuitive low rate of asthmatic patients, or smokers? Do host genetics have any influence on the course of the disease? And our microbiota? Are antibodies essential for fighting the infection, or could they even act against the organism? Do food supplements like vitamin D or zinc show any benefit? … Many questions arise, and we will try to approach valid answers keeping in mind that biology is not (yet) infallible mathematics.
The paper is structured as follows. We can contain the SARS-CoV-2 threat in several ways, first of all, by avoiding infection and consequently, spreading of the disease. This is an essential strategy and involves hygienic, social, and pharmacological measures to tackle the problem at source. Second, by treating the disease when already infected. Many factors may intervene and influence the course of the infection and therefore multiple treatment candidates arise. We will mark several lines of defense for reaching both objectives. Following, we will analyze relevant COVID-19 risk factors including biomarkers, patient’s sex and age, genetic factors, autoimmunity, and influence of the microbiota. Next, implications of SARS-CoV-2 mutations will be analyzed, and finally, emergent potential treatments will be exposed. The conclusions section will summarize what we have learned and will identify open issues.
Avoiding the infection
First line of defense: physical barriers
Though SARS-CoV-2 is a novel virus, it presents several characteristics shared with other known coronaviruses like SARS(-CoV) or MERS. Also, the understanding of the virus has grown quickly since its appearance. However, as knowledge expands it becomes more evident that too many aspects related to the SARS-CoV-2 biology and impact on human health still remain unknown. Here we can humbly adopt Socrates saying, ‘I know that I know nothing’.
The main entry of the SARS-CoV-2 in our organism is via the respiratory airways through our nose and/or mouth. Probably, the eye mucous membrane is another point of entry; however, there is currently no strong evidence but the intuitive logical affection when droplets directly impact the eye. Also, it is already proven that, apart from droplets and fomites, aerosols are one, if not the most relevant SARS-CoV-2 transmission factor (Scientific Brief: SARS-CoV-2 and Potential Airborne Transmission Citation2020; Coronavirus is in the air – there’s too much focus on surfaces Citation2021). We could even think of this way of contagion as fortunate since protective measures are possible and even straightforward. Therefore, a physical barrier covering nose and mouth is the first solution to think of, and this is not new: this was already the case early in the twentieth century when the erroneously called Spanish influenza in 1918 devastated the world causing millions of deaths. Hands washing and social distance complement the current–old measures to keep the virus away. However, face masks used by general population are not perfect due to loose fit, overuse and fabrics with variable permeability to virus penetration. Only respirators or personal protective equipment prevent the wearer from inhaling aerosols (including the aerosol transported SARS-CoV-2) and gases. In Europe, they must meet the European standard EN 149: 2001 that consists of three classes of disposable particulate respirators: FFP1, FFP2, and FFP3, depending on the percentage of filtration. FFP3, with a minimum filtration percentage of 99, is not usually available for consumers and is restricted to sanitary personnel dealing with patients. Since a physical barrier is an easiest and safest solution, we believe efforts should have been put on providing FFP3-like protective face masks to the population at an affordable price. New generation electronic masks are beginning to appear. Think that if every person in the world wore a really protective face mask for several weeks each time out of home or in any potentially infectable situation, SARS-CoV-2 would have soon been history (skipping other issues like social eating or even pets possibility of transmission (Kiros et al. Citation2020; Munnink BB et al. Citation2021)).
Second line of defense: preventing the virus from entering the cells
Viruses need the (transcription and) translation host cell machinery to replicate. SARS-CoV-2 would not cause infection in humans if binding to the ACE2 receptor was avoided. Several choices co-opt to reach this aim.
The human innate immune system
Even tiny bacteria have an immune system. The human immune system exhibits a very high complexity and is still not fully understood. The immune system consists of innate and adaptive branches that interact together. The innate part attacks first and may handle up to 99% of infections. It consists mainly of physical and chemical barriers, phagocytic cells such as neutrophils and macrophages, dendritic cells, natural killer cells, and the complement system. If it fails, the adaptive branch is called, and specific T lymphocytes (or T cells) and antibody-producing B lymphocytes (or B cells), among other cells and mechanisms, enter into action (de Vrieze Citation2020).
Many pathogens exhibit glycoproteins and glycolipids on their surface, named pathogen-associated molecular patterns or PAMPS that enable some innate cells such as monocytes, macrophages, and dendritic cells to recognize them as foreign. Pattern recognition receptors (PRRs) located inside and outside the cell, like toll-like receptors (TLRs like intracellular TLR7 involved in detecting SARS-CoV-2) bind to the PAMPs, and start processing the exogenous microorganism. Consequent activation of downstream transcription factors like interferon regulator factors (IRFs) and nuclear factor κB (NF-κB) occurs, inducing type I and III interferons (IFN-I and IFN-III) and IFN-stimulated genes (ISGs) activity, as well as chemokine secretion to recruit leukocytes (Blanco-Melo and Nilsson-Payant Citation2020) such as macrophages and dendritic cells, that bridge both the innate and adaptive systems, and release cytokines that enroll other macrophages and dendritic cells to destroy the microbes through the inflammatory response (Figure ). T cells and B cells from the adaptive system can also be activated (Iwasaki and Medzhitov Citation2015; Tortora et al. Citation2019). IFNs are one of the main weapons that the innate immune system raises, warning the neighboring cells of the presence of the pathogen in order to stop their own protein synthesis, and therefore the virion (the complete extracellular infectious viral particle) replication. IFNs signal through the JAK-STAT pathway and induce the transcription of ISGs, which generate antiviral functions and reinforce the immune system by further inducing IFNs or ISGs; however, some genes may facilitate viral replication. Type I (IFN-α/β) and type III (IL-28A, IL-28B, IL-29) IFNs are antiviral, though type II IFN (IFN-γ) has antiviral properties too. Each IFN induces a unique set of ISGs (Schoggins and Rice Citation2011).
Figure 1. In innate immunity, sensors outside and inside a cell detect unusual molecules (e.g. from pathogens like viruses) through pattern recognition receptors (PRR), triggering immediate alarm through the secretion of cytokines and interferons. (Reprinted from ‘Innate Immunity', by BioRender, June 2020, retrieved from https://app.biorender.com/biorender-templates/ Copyright 2021 by BioRender.)
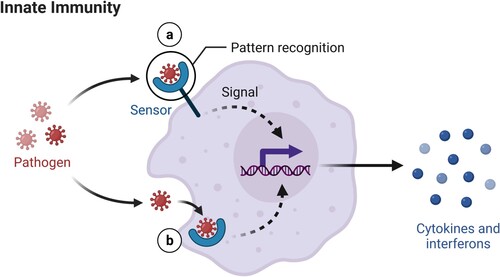
Cells from the immune system communicate with each other and coordinate by releasing cytokines (Iwasaki and Medzhitov Citation2015; Testar Citation2021; James MacLachlan and Dubovi Citation2017; McComb et al. Citation2019) and chemical substances that are captured by specific surface cell receptors. Cytokines are small signaling proteins or glycoproteins that modulate both innate and adaptive immune responses, inflammation, and hematopoietic cell proliferation and differentiation. The two principal producers of cytokines are a kind of T cells (helper T cells) and macrophages, although any nucleated cell may act as secretor. Cytokines can be proinflammatory or anti-inflammatory. Proinflammatory cytokines induce fever and inflammation responding to infection or tissue injury, examples include interleukins (IL) 1, 6, 8, 12, and 18; interferons (INF); and tumor necrosis factor (TNF). Anti-inflammatory cytokines such as IL-4, IL-10, IL-11, and IL-13; and transforming growth factor-beta (TGF-β) suppress the immune system. Receptor engagement triggers intracellular signaling cascades that alter gene expression in the target cell, causing biological effects like differentiation, proliferation, or activation.
SARS-CoV-2 has the ability to escape the host immune surveillance, avoiding initial recognition by PRRs, dysregulating IFN responses by some IFN-antagonizing non-structural proteins (nsps, components of the viral replication/transcription complex, expressed in infected cells but not conforming the virion particle), or directly activating the NF-κB pathway by the N protein. Very low amounts of IFNs have been found in the peripheral blood or lungs of severe COVID-19 patients, though IFN activity has been found in alveoli of some patients (Acharya et al. Citation2020).
Therefore, IFN administration has been proposed for antiviral treatment, also against SARS-CoV-2. Some studies point to ACE2 as a human ISG, suggesting that SARS-CoV-2 could exploit species-specific IFN-α-driven upregulation of ACE2 in human upper airway epithelial cells (IFN-γ would be responsible in the case of enterocytes in the gastrointestinal mucosa), that induces tissue protection during lung injury, to enhance infection (Taylor and Waghray Citation2020; Heuberger et al. Citation2021). However, recent research shows that IFN I, II, and III actually induce a short transcript of ACE2, an isoform – proteins encoded by the same gene that may exert different biological roles – unable to bind SARS-CoV-2 spike protein that might have antiviral effects. In that respect, cytokines could be responsible for long ACE2 induction (Blume et al. Citation2021).
Therapies administering IFN should consider the correct dose and time of infection, as well as the specific patient features, to avoid any unwanted outcome. On the other side, severe and critical patients show a unique phenotype consisting of the absence of IFN-β and few IFN-α with low activity, along with a lingering blood viral load and a disproportionate inflammatory response, to some extent lead by the transcriptional factor NF-κB and characterized by augmented TNF-α and IL-6 production and signaling (Hadjadj et al. Citation2020). Also, and strikingly, high titers of neutralizing autoantibodies against type I IFN have been discovered in around 10% of severe COVID-19 pneumonia patients, mostly aged men (Zhang et al. Citation2020b). Type III IFNs signal through a receptor complex whose expression is limited to epithelial cells and a group of immune cells like neutrophils, so a therapy relying on type III IFN administration would induce ISGs restricted to epithelial cells, thus lowering side effects and inflammation linked with the systemic action of type I IFNs (Prokunina-Olsson et al. Citation2020).
It is worth mentioning the possible neutrophils’ role in COVID-19. These innate immune cells kill pathogens by oxidative burst, phagocytosis, and NETs (Neutrophil Extracellular Traps), complexes of DNA and proteins forming a web released by the neutrophil. Under a cytokine storm where inflammation factors are widely present, NETs may induce macrophages to secrete IL-1β, which in turn enhances NET formation, forming a noxious loop that might promote microthrombi and aberrant immune responses (Barnes et al. Citation2020).
Cellular virus entry blockade
Our complex immune system includes B cells that produce antibodies which target antigens present in pathogens like viruses. An epitope is a fragment of an antigen (a foreign or mutated self-protein able to induce an immune response) that is bound by antibodies or B/T cells receptors. Many of the designed vaccines against SARS-CoV-2 are focused on stimulating the immune system to generate neutralizing antibodies that bind epitopes present in the receptor-binding domain (RBD) of the virus spike protein, therefore avoiding the spike binding to the ACE2 receptor. However, among the SARS-CoV-2 strategies trying to evade the immune system monitoring, RBD hiding or folding is found, in a way that RBD stochastically swings upwards to offer the ACE2 binding site (Zhou et al. Citation2020a). Also, pre-activation of the spike via the furin enzyme enhances SARS-CoV-2 entering the host cell. Additionally, cell surface protease TMPRSS2 and lysosomal cathepsins activate SARS-CoV-2 entry and they both help furin in gaining access to the cell (Shang et al. Citation2020). Therefore, drugs capable of blocking furin, the ACE2 receptor, the TMPRSS2, or the lysosomal cathepsins should be contemplated (Jamshaid et al. Citation2020; Jeong et al. Citation2020; Li et al. Citation2020a; Rehman et al. Citation2020); always with caution since these host cell elements may be essential for other activities. For example, ACE2, present in cells of many organs such as the lungs, heart, kidney, testis, large conduit arteries, or intestines (Igase et al. Citation2008), is critical in lowering blood pressure by reducing the amount of angiotensin-II (vasoconstrictor) and increasing angiotensin (1–7) (vasodilator). Besides, patients suffering from diabetes or hypertension have ACE2 expression increased, and they are treated with angiotensin-converting enzyme (ACE) inhibitors and angiotensin II type-I receptor blockers (ARBs) that increase ACE2 expression and therefore may be also a virus infection; however, further research on this possibility is required (Fang et al. Citation2020; Gupta et al. Citation2020; Sadria et al. Citation2020; Shibata et al. Citation2020). The same is applicable to the host cell factor TMPRSS2, associated with physiological and pathological processes including digestion, tissue remodeling, fertility, blood coagulation, inflammatory responses, tumor cell invasion, and apoptosis.
Despite the virus entry through the ACE2 receptor is undoubted, it is worth mentioning that other receptors might be helping SARS-CoV-2 entering the host cell. On the one hand, the expression pattern of ACE2 does not exactly match tissue tropism of SARS-CoV-2. On the other hand, there exist numerous human neutralizing antibodies that bind the spike but not the RBD. A recent study suggests that the tyrosine-protein kinase receptor UFO (encoded by the AXL gene) is essential in facilitating the respiratory system infection, what would point to other potential treatments against SARS-CoV-2 (Wang et al. Citation2021b).
Potential treatments against virus entry (Depfenhart et al. Citation2020; Jamshaid et al. Citation2020; Jeong et al. Citation2020; Li et al. Citation2020a; Rehman et al. Citation2020) include hrsACE2, a recombinant (genetically modified) soluble form of ACE2, and arbidol targeting ACE2; camostat mesilate, type 1 plasminogen activator inhibitor (PAI1) and nafamostat, serine protease inhibitors, and bromhexine hydrochloride, a mucolytic drug, targeting TMPRSS2; and monoclonal and recombinant antibodies targeting furin protease (Gene cards Citation2021). Arbidol, in fact, may interact with several steps of the virus life cycle (Blaising et al. Citation2014), showing a broad-spectrum efficacy (Blaising et al. Citation2014; Gao et al. Citation2020; Nojomi et al. Citation2020). Also worth mentioning, hrsACE2 is able to neutralize the SARS-CoV-2 by binding the spike protein mimicking ACE2, and also to reduce noxious angiotensin II concentrations (Zoufaly et al. Citation2020).
Antibodies and aptamers
An antibody is a type of immunoglobulin, a protein made by B cells (Alberts et al. Citation2015). When an antigen binds to the B-cell surface, the B cell, with the help of a type of T cell (helper T cell) undergoes clonal proliferation and differentiation. The differentiated B plasma cells secrete millions of antibodies into the bloodstream and lymphatic system, where they may permeate the other body fluids, and bind to and only to the specific antigen that stimulated their production. With this binding, the antibody helps destroying the antigen, either directly (neutralizing antibody) or indirectly, marking the antigen to help other white blood cells and activate the complement system (a circulating protein complex that acts in cascade and helps in eliminating pathogens).
Regarding antibodies response to SARS-CoV-2, there are still many open issues. Some studies have shown that antibody responses seem to wane after infection with some coronaviruses including SARS-CoV-2, though the latest studies suggest long-lasting antibody immunity (Ibarrondo et al. Citation2020; Long et al. Citation2020; Gaebler et al. Citation2021). Concretely, it has been proven that over 90% of more than 30,000 mild COVID-19 patients develop robust immunoglobulin G (IgG) antibodies against the spike protein (neutralizing antibodies), that last for at least five months (Wajnberg et al. Citation2020). Also, mucosal IgA shows potent neutralizing activity in early stages of the infection (Sterlin et al. Citation2021).
Besides, it has been demonstrated that in mild convalescent COVID-19 patients, serum IgG antibodies against the spike exhibit a falling slope that smooths from month four to eleven after infection, when these IgG are still detected. This is compatible with antibodies being initial and profusely generated by short-lived plasmablasts, and subsequently by long-lived bone marrow plasma cells (BMPCs). BMPCs together with memory B-cells (MBCs), that quickly differentiate into antibody-secreting cells and form new germinal centers if second exposure to antigen occurs, then contribute to the humoral immune response against SARS-CoV-2. However, the neutralizing profile of the measured antibodies in this study is not known (Turner et al. Citation2021a).
In symptomatic and, to a slightly lesser extent, asymptomatic COVID-19 patients, antibodies exhibit not only neutralizing capabilities, hindering the SARS-CoV-2 entrance in the host cells, but are also able to activate complement through the complement-dependent cytotoxicity (CDC), and kill infected cells via the antibody-dependent cellular cytotoxicity (ADCC) that turn on natural killer (NK) cells. It is possible that asymptomatic patients exert a quick IgA response to control the virus that, in turn, impacts a posterior lower antibody response (Dufloo et al. Citation2021).
Monoclonal antibodies
Monoclonal antibodies (mAb) are identical immunoglobulins, generated from a single B-cell clone (Monoclonal Antibodies Overview Citation2021). These antibodies recognize and bind unique epitopes on a single antigen. The epitope is a specific part of the antigen (which has several different epitopes) recognized by antibodies and T cells. Therefore, we can think of identifying the main epitopes of the antigens present in the virus (the spike and the nucleocapsid are the most immunogenic proteins in SARS-CoV-2 (Dutta et al. Citation2020)) and develop monoclonal antibodies against them.
Many tools currently exist for predicting B cell and T cell epitopes (Sanchez-Trincado et al. Citation2017; Immune Epitope Database (IEDB) Citation2021). For example, EpiVax has computational tools to predict epitopes, then manufactures synthetic amino acid peptides that mimic the epitopes of the virus, and finally tests the reactivity of these synthetic peptides in blood samples from COVID-19 recovered patients, since their blood – especially if recently recovered – most likely contains immune cells and antibodies that recognize the antigenic epitopes of the virus (Medeiros Citation2020). Also, some neutralizing monoclonal antibodies binding to specific epitopes have already been identified, such as 47D11 that binds a conserved epitope on the spike RBD, and therefore is able to cross-neutralize both SARS-CoV and SARS-CoV-2 (Wang et al. Citation2020). Or (the double discovery of) two human mAbs cloned from RBD-specific memory B cells isolated from recovered COVID-19 patients that are able to block the interaction between SARS-CoV-2 RBD and ACE2 receptor, therefore neutralizing SARS-CoV-2 infection (Chen et al. Citation2020c; Wu et al. Citation2020b). Also, the AstraZeneca’s AZD7442 that is under trial (COVID-Citation19 Long-Acting AntiBody (LAAB) combination AZD7442 rapidly advances into Phase III clinical trials Citation2020), or Lilly’s LY-CoV555 mAb (a.k.a. bamlanivimab) alone or in combination with LY-CoV016 mAb, which bind different epitopes in the SARS-CoV-2 spike. A trial is testing the ability of this antibody cocktail to reduce the number of patients with persistently high viral load and the hospitalizations (Lilly announces proof of concept data for neutralizing antibody LY-CoV555 in the COVID-19 outpatient setting Citation2020), and recently bamlanivimab has been authorized by the FDA as treatment for COVID-19 (Coronavirus (COVID-19) Update: FDA Authorizes Monoclonal Antibody for Treatment of COVID-19 Citation2020). Also, in late phase the trials for the Regeneron REGN-COV2, a combination of two neutralizing mAbs (REGN10933 and REGN10987), that bind non-competitively to the RBD of the virus’ spike protein, decreasing the probability that mutant viruses or spike variants evade treatment. With the commercial names of casirivimab and imdevimab, both mAbs together have already been authorized by the FDA as treatment for COVID-19 (Coronavirus (COVID-19) Update: FDA Authorizes Monoclonal Antibodies for Treatment of COVID-19 Citation2020). Eli Lilly and AbCellera Biologics have developed LY-CoV1404 (or LY3853113) monoclonal antibody that has demonstrated resistance to new variants since it binds to a region in the RBD little susceptible to mutations (a reasonably conserved epitope) (Westendorf et al. Citation2021).
Besides monoclonal antibodies, broadly neutralizing antibodies (bnAbs) that target several coronavirus types (also known as cross-neutralizing antibodies) can be synthesized to overcome their low binding affinity. The engineered bnAb ADG-2 is able to approach a highly conserved epitope in the RBD with a different angle, effectively neutralizing SARS-CoV-2 (Rappazzo et al. Citation2021).
The Antibody Society offers data from anti-SARS-CoV-2 antibodies in clinical studies (COVID-19 Biologics Tracker Citation2021).
Aptamers
Aptamers are synthetic short, stable single-stranded DNA or RNA oligonucleotides that bind to specific targets including toxins, non-immunogenic targets, metabolites, or live cells. Their tertiary structure (3D folding) is responsible for the aptamers’ high affinity binding properties. Unlike antibodies, aptamers’ smaller size allows for tissue penetration, they are non-immunogenic (do not elicit an immune response, as non-human antibodies do), and they can be more easily stored, produced, and delivered (Kalra et al. Citation2018). A DNA aptamer has shown to inhibit viral infection differently from antibodies, without binding the spike RBD but once the virus has bound the host cell. However, the exact mechanism by which this inhibition is performed is still undiscovered (Schmitz et al. Citation2021).
Nasal cavity: virus entry, ACE2 presence, and nasal administration
Since the virus penetrates the organism through our respiratory tract, we can easily think of a drug administered with the help of a nasal inhaler. An early blockade of the virus is a crucial strategy since it avoids the invasion of the lower respiratory tract, the spread of the virus throughout the organism and the potentially fatal consequences in the lungs and other organs.
Aptamers and monoclonal antibodies could be potential candidates for a nasally administered treatment; however, the latter are large and not very stable, therefore not convenient for an intranasal application. Computer-designed miniproteins, which are easier to produce, more stable and avoid the need for refrigeration, have shown to block SARS-CoV-2 infection as well as monoclonal antibodies. These high-affinity miniproteins compete with ACE2 in binding the SARS-CoV-2 spike RBD, showing potent neutralization of SARS-Cov-2 (Cao et al. Citation2020). A similar alternative is bivalent VH-Fc Ab8 that binds to the three S protomers competing with ACE2 and potently neutralizes SARS-CoV-2 (Li et al. Citation2020b). Ty1, a single domain antibody fragment derived from an animal binds the RBD with high affinity, avoiding spike to ACE2 attachment (Hanke et al. Citation2020). Other solutions include the nasal administration of the naturally occurring protein with antiviral properties INF-β that may protect the virus-infected lung cells. A study administering an inhaled nebulized recombinant INF-β-1a (SNG001) to COVID-19 hospitalized patients showed greater than one odds ratio and faster recovery (Monk et al. Citation2020). Also, stable lipopeptide membrane fusion inhibitors that are inhaled daily have shown fully effective prophylactic properties in ferrets cohabiting with infected individuals, avoiding SARS-CoV-2 transmission (de Vries et al. Citation2021). Currently, Taffix nasal spray is available on the market, and offers a simpler solution based on a muco-adhesive gel (hydroxypropyl methyl cellulose) that covers the epithelial cells and avoids the virus binding to cell receptors, also creating an acidic microenvironment of pH 3.5 on the nasal mucosa that hinder respiratory viruses, including SARS-CoV-2, from infecting cells. Taffix achieves an odds ratio of 0.22 for SARS-CoV-2 infection (Klang et al. Citation2021). Besides, the well-known anticoagulant heparin and its variants exhibit exceptional binding affinity to the spike protein and show promising antiviral activity and low cytotoxicity (Kwon et al. Citation2020). Of note, it is proven that heparin can also act in dismantling NETs (Crimi et al. Citation2020).
Though vaccines are out of the scope of this article, there are also projects proposing intranasal administration, such as the single-dose Codagenix COVI-VAC that has shown efficacy and safety in preclinical studies and is currently in clinical development. This vaccine is based on a live attenuated version of SARS-CoV-2, and as such would induce a robust and long-lasting immune response. This interesting approach processes the SARS-CoV-2 genome and inserts silent mutations in order to use codon pairs underrepresented in human cells (but keeping the aminoacids), and as a result the protein translation is deoptimized and slowed down, yielding a great decrease in pathogenesis (Wang et al. Citation2021c).
We should not forget the mouth cavity as a means of spreading the virus when exhaling air, talking or singing. Oral mouthwashes exhibit antiviral properties against influenza and several coronaviruses such as SARS-CoV-2 (Meister et al. Citation2020; O’Donnell et al. Citation2020; Statkute et al. Citation2020), and their usual constituent CetylPyridinium Chloride (CPC) has demonstrated its ability to block viral entry by breaking the integrity of the SARS-CoV-2 membrane, hence offering a decrease in infectivity of over one thousand times. Additionally, this would give grounds for developing CPC-based prophylactic nasal sprays (Muñoz-Basagoiti et al. Citation2021).
ACE2 is particularly expressed in nasal epithelial cells, besides alveolar epithelial type II cells in the parenchyma (Sungnak et al. Citation2020). Moreover, the majority of ACE2 is localized in the olfactory neuroepithelium (but not present in olfactory neurons), which may explain the anosmia suffered by COVID-19 patients. This picture may also justify nasally administered therapies (Chen et al. Citation2020a). Neuropilins (NRP) are essential cell surface receptors involved in multiple cellular signaling cascades, also related to many pathological processes, that are profusely expressed in the respiratory and olfactory epithelium, showing the highest expression in endothelial cells and in the epithelial cells of the nasal cavity. Besides, NRP-1 give assistance to carrying their binding molecules inside the cell and among cells, penetrating tissues. It has been found that the SARS-CoV-2 spike binds NRP-1, remarkably potentiating SARS-CoV-2 infectivity (Cantuti-Castelvetri et al. Citation2020). This would explain the enhanced spreading of SARS-CoV-2 and its infection in cells with low ACE2 expression (in fact, the majority), and could also clarify the COVID-19 anosmia symptom, since both the S protein and the vascular endothelial growth factor-A (VEGF-A, a pro-nociceptive and angiogenic factor) bind NRP-1, therefore the spike is able to block the VEGF-A-triggered sensory neuronal firing and so silences pain (Moutal et al. Citation2021). Interestingly, therapies for blocking NRP-1 currently exist (Ding et al. Citation2018).
Also, SARS-CoV-2 genetic material and proteins have been found in different parts of the nasopharynx and brain, what would support virus entry via the neural–mucosal interface in olfactory mucosa previous to a possible transport through the olfactory tract towards the central nervous system. In fact, it has been proven that the spike protein S1 subunit is able to cross the blood-brain barrier in mice (Rhea et al. Citation2020). Moreover, virus found in the cerebellum and the brainstem would suggest additional virus ingress ways, and the latter location could further impact disturbance of the cardiovascular and respiratory systems (Meinhardt et al. Citation2020).
Conclusions
Face masks are a simple and effective way of blocking SARS-CoV-2 entry into our organism through our respiratory airways. On the other hand, monoclonal (synthetic) antibodies bind epitopes on virus antigens and may exert neutralizing capabilities, but their size has some disadvantages tackled by aptamers, single-stranded DNA or RNA oligonucleotides that could constitute a solution by inhibiting viral infection. Miniproteins, smaller in size than monoclonal antibodies, could be applied intranasally, as well as some vaccines, in a straightforward means of preventing the virus natural entrance.
Within the complex human innate immune system, interferons are key players. SARS-CoV-2 is able to dysregulate the IFN responses; also, critical COVID-19 patients exhibit a distinctive IFN phenotype, and some even show autoantibodies against type I IFN. Therapies administering IFN are being considered. To prevent the virus entry into the cell, drugs able to obstruct furin, ACE2, the TMPRSS2 or the lysosomal cathepsins are the main candidates.
Treating the disease
Third line of defense: inside the human host cell
SARS-CoV-2 structure and life cycle
SARS-CoV-2 is an enveloped, positive-sense, single-stranded RNA virus with a large genome comprising 31.3 kilobases, protected by a lipid membrane containing the spike (S), envelope (E), and membrane (M) proteins. The nucleocapsid (N) protein tightly coils and coats the virus genetic code (RNA) (Lee et al. Citation2020).
The virus enters the host cell through endocytosis by binding the Receptor Binding Domain (RBD) of its S protein to the Angiotensin-Converting Enzyme 2 (ACE2) receptor, which is present on the surface of several types of human cells such as the membrane of secretory and ciliated cells in nasal mucosa, bronchus, kidney, intestinal tract, pancreas or gallbladder, and in endothelial cells and pericytes in many tissues (The human protein atlas Citation2021).
Once inside the cell, uncoating undergoes and the genetic code of the virus is released. The cell’s translation machinery (the ribosomes) confuses the virus RNA with own messenger RNA (mRNA) and begins translating the main gene of the genome, the replicase, that contains the open reading frames (ORFs) 1a and 1b, which express polyproteins pp1a and pp1ab. The polyproteins are proteolytically cleaved and give rise to 16 non-structural proteins (nsp1–16) needed for viral replication and transcription, starting from the autocleaved main protease Mpro (nsp5, also known as 3CLpro). Some relevant nsps include the papain-like proteinase PLpro (nsp3), the RNA-dependent RNA polymerase RdRp (nsp12), or the helicase (nsp13), that also have other relevant functions to evade the immune system (Wu et al. Citation2020a). These nsps form the replication-transcription complex that will synthesize a negative RNA strand, from which more positive RNA will be replicated to conform the genetic code of the newly synthesized virions, and subgenomic positive mRNAs will be generated and then translated into the new virion proteins. Budding and assembly of the new virions occur at the rough endoplasmic reticulum and the Golgi, and the virions finally exit the cell via exocytosis (Manirul Haque et al. Citation2020) (Figures and ).
Figure 2. SARS-CoV-2 genome including ORF1a, ORF1b; spike (S), envelope (E), membrane (M), and nucleocapsid (N) proteins. ORF1ab, the largest gene, includes overlapping ORFs that encode polyproteins pp1a and pp1ab. Non-structural proteins (nsp) PLpro, 3CLpro, endoribonuclease, helicase, and RdRp are shown together with the structural proteins and some 3D protein structures. (Reprinted from ‘Genomic Organization of SARS-CoV-2', by BioRender, March 2020, retrieved from https://app.biorender.com/biorender-templates/ Copyright 2021 by BioRender.)
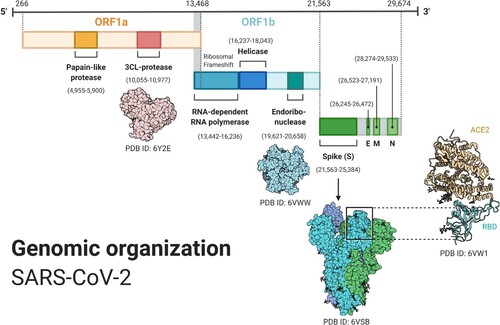
Figure 3. SARS-CoV-2 life cycle. (Adapted from ‘Life Cycle of Coronavirus', by BioRender, August 2020, retrieved from https://app.biorender.com/biorender-templates/ Copyright 2021 by BioRender.)
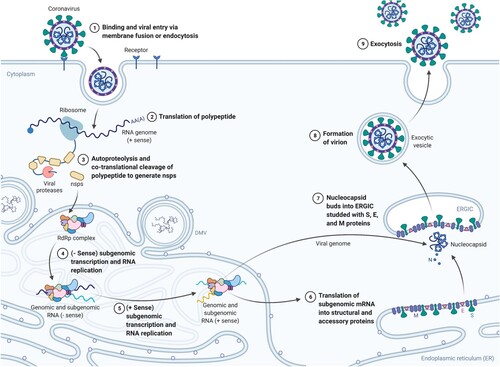
Antivirals
All the proteins and processes involved in the virion replication process constitute potential drug targets by (antivirals) disrupting the SARS-CoV-2 life cycle, being the most relevant the proteins Mpro and RdRp. Among these direct-acting antiviral candidate drugs we can find (hydroxi)chloroquine and arbidol against endocytosis; lopinavir/ritonavir (also together with INF-β), darunavir/covicistat, ASC09F/oseltamivir, α-ketoamide or disulfiram against Mpro; and remdesivir (the only drug approved by the FDA against COVID-19 up to January 2021), ribavirin, favipiravir or tenofovir disoproxil against RdRp (Horby et al. Citation2020; Jamshaid et al. Citation2020; Jeong et al. Citation2020; Li et al. Citation2020a; Rehman et al. Citation2020).
SARS-CoV-2 employs a smart triple strategy to obstruct the infected host cell gene expression process. First, it reduces the cell’s translation capacity; it also degrades host mRNA; and, finally, it inhibits the translation of the cell’s mRNA by avoiding its nuclear export. Altogether, these facts could lie behind the IFN response deletion appeared in some COVID-19 patients (Finkel et al. Citation2021).
The identification of 332 human proteins that interact with SARS-CoV-2’s has helped in uncovering druggable host targets (Gordon et al. Citation2020). Besides, some of the proposed treatments against COVID-19 have already been tested in patients formerly affected from SARS and MERS (Zumla et al. Citation2016). Drug repurposing or reuse of medicines from other diseases such as cancer (aplidin), HIV (lopinavir, ritonavir), rheumatoid arthritis (baricitinib, tofacitinib), hepatitis C HCV (ribavirin), ebola (remdesivir), or even gastric ulcer (famotidine) is being investigated in many studies (Bairi et al. Citation2020; Janowitz et al. Citation2020; Kumar et al. Citation2020; Li and De Clercq Citation2020; Mahdi et al. Citation2020; Sang et al. Citation2020; Guimarães et al. Citation2021). Artificial intelligence is playing a pivotal role in repurposing and discovery of new drugs with platforms like Broad Institute’s Clue drug repurposing hub (Broad Institute Citation2021; Hoagland et al. Citation2020) or the IBM Functional Genomics Platform (IMB Citation2021). A tracing of COVID-19 treatments, drugs and vaccines can be found at (Milken Institute Citation2021; Wu et al. Citation2021).
It is worth remarking the high error rates that affect the replication process of positive-sense RNA virus genomes, generating viral clouds of mutants able to adapt to the environment and eventually evade antivirus treatments or vaccines. But the proofreading mechanism exerted by the non-structural protein nsp14 of large genome coronaviruses possibly including SARS-CoV-2, is capable to increase replication fidelity. New generation nucleotide/nucleoside analogue inhibitors (NIs) may overcome this correction barrier. Administered as prodrugs, they are metabolized and activated inside the cell, and their mis-incorporation or mispairing with natural nucleotides in the replication process may lead to chain termination or mutations that reduce replication fidelity and end in lethal mutagenesis. Remdesivir, ribavirin and favipiravir, and anti-HCV drugs daclatasvir and sofosbuvir are examples of common antiviral nucleoside analogues, but unfortunately have not been able to show clinical benefits up to now maybe due to the action of nsp14 (Pruijssers and Denison Citation2019; Robson et al. Citation2020; Sacramento et al. Citation2020), though studies continue and they still cannot be definitely discarded (Buckland et al. Citation2020; Kokic et al. Citation2021). Molnupiravir, an oral pro-drug of the nucleoside analogue N4-hydroxycytidine (NHC) also known as MK-4482 or EIDD-2801, and initially purposed to treat influenza, has shown therapeutic and prophylactic success from inhibiting SARS-CoV-2 replication in vivo (Wahl et al. Citation2021). Updated information about COVID-19 antiviral therapies can be found in Stanford University (Citation2021). On the other hand, antimicrobials such as azithromycin (Oldenburg and Doan Citation2020; Blanco et al. Citation2021) and antiparasitics like ivermictin (a nuclear transport inhibitor) (Caly et al. Citation2020; Portmann-Baracco et al. Citation2020; Chaccour et al. Citation2021) have shown antiviral effects in COVID-19 cases. In fact, macrolide bacteriostatic antibiotics like azithromycin, and to a higher extent clarithromycin and lexithromycin, are able to in vitro inhibit SARS-CoV-2 viral entry and could be applied to fight COVID-19 early infection (Galvez et al. Citation2021).
We can also think of inhibiting the de novo biosynthesis of pyrimidine-based nucleotides, which basically compose the RNA and DNA, facilitated by the human dihydroorotate dehydrogenase enzyme (DHODH). Inhibition of DHODH induces pyrimidine nucleosides depletion that essentially affects RNA virus replication, moreover, the decrease in pyrimidine pools triggers host antiviral genes expression and promotes innate immune responses (Coelho and Oliveira Citation2020; Xu and Jiang Citation2020). PTC Therapeutics oral small molecule PTC299 trial for inhibition of DHODH activity is underway (PTC Therapeutics Announces the Initiation of a Phase 2/3 Clinical Trial to Evaluate PTC299 for the Treatment of COVID-19 Citation2020). Or the blocking of the eukaryotic translation elongation factor 1 alpha (EEF1A), responsible for the enzymatic delivery of aminoacyl tRNAs to the ribosome (NCBI Resources Citation2021). PharmaMar’s bet Aplidin (plitidepsin), an anticancer agent of marine origin with ongoing trials, exerts its action by effectively blocking EEF1A, already successfully tested in vivo (Onco’Zine Citation2020; White et al. Citation2021). Targeting host factors avoids the potential immune response evasion occasioned by virus mutations.
However, prophylactic oral drugs are still missing, that would be an essential weapon against SARS-CoV-2 and its complex set of variants. Viruses depend on host metabolism pathways to replicate, so these could become new early targets. It has been shown that SARS-CoV-2 infected cells suffer from exhausted stores of glucose and folate, which are supposed to be deviated to viral biosynthesis. Of interest, inhibitors of folate metabolism like methotrexate are already employed to treat inflammatory types of arthritis (Zhang et al. Citation2021b). Another option comes from the well-known anti-leprosy drug clofazimine, that exerts its antiviral activity by inhibiting the viral membrane fusion and the helicase nsp action, minimizing the viral load and the subsequent inflammation. For hospitalized COVID-19 patients, clofazimine could be combined with remdesivir or with interferon beta-1b. Clinical trials are underway. Above all, this inexpensive oral drug also exhibits pan-coronavirus activity (Yuan et al. Citation2021). Pfizer is also running trials for its oral (pan-coronavirus) antiviral drug PF-07321332, a 3CLpro protease inhibitor intended to fight the initial infection. For COVID-19 hospitalized patients, another protease inhibitor intravenously administered, PF-07304814, is under clinical trial (Pfizer initiates Phase 1 study of novel oral antiviral therapeutic agent against SARS-CoV-2 Citation2021).
EXO-CD24, an inhaled drug employs exosomes, the extracellular vesicles involved in intercellular communication to transport CD24 – a glycophosphatidylinositol-anchored protein able to regulate inflammation and hence repress the cytokine storm – to the lungs. The success of Phase I trial, in which all 35 patients recovered within few days has been confirmed with another 90 patients in Phase II, and will be followed by Phase III trials (Evaluation of the Safety of CD24-Exosomes in Patients With COVID-19 Infection Citation2021; CD24Fc as a Non-antiviral Immunomodulator in COVID-19 Treatment (SAC-COVID) Citation2021).
Epigenomics
Epigenomics refers to epigenetic modifications involving external factors that impact gene activity (the degree of expression or even turning genes on/off) without changing the underlying DNA sequence. DNA methylation (DNAm), histone – proteins that pack the DNA – modifications (acetylation, citrullination, or phosphorylation) and nucleosome remodeling, or regulation by non-coding RNAs such as miRNAs (microRNAs, short RNAs that repress target gene expression’s mRNA after transcription) and siRNAs (small interfering RNAs that cleaves target mRNA and avoids translation) are typical epigenetic mechanisms. Regarding COVID-19, viral entry and immune responses like NETosis and TGF-β pathway seem to be associated with epigenetic regulation. Also, DNAm could be related to higher susceptibility of the lung tissue to the disease (Shirvaliloo Citation2021).
On the one hand, SARS-CoV-2 is able to use epigenetic-regulated mechanisms during host–pathogen interaction in order to interfere with innate and adaptive immunity and inflammatory response. For example, by downregulating the MDA5 gene, involved in the antigen presentation, or by handling host miRNA machinery for its own benefit. Some drugs may try to counteract these epigenetic manipulations of the virus: for example, heparin can avoid histone-induced platelet aggregation; curcumin (a histone deacetylase inhibitor) is a possible inhibitor of Mpro and might lower proinflammatory cytokines and expression of the NF-kB gene in macrophages; and tocilizumab, the IL-6 antagonist monoclonal antibody, may impact two epigenetic-sensitive mechanisms: the reduction of NETosis (neutrophil death after NET extrusion) and the upregulation of miRNA-23, miRNA-146, and miRNA-223 – related to coronary and immune diseases – serum levels (Crimi et al. Citation2020). Also, drugs like vafidemstat, a lysine-specific histone demethylase 1A (KDM1A) inhibitor, are already being tested (Oryzon Citation2021).
On the other hand, we can think of targeting the virus mRNA, especially in highly conserved regions of the genome, with the help of siRNAs, a powerful and recent tool whose side effects would still need a deep evaluation. Previous studies applying siRNA over SARS and MERS have been published (Ghosh et al. Citation2020; Uludağ et al. Citation2020). The open reading frames (ORFs) themselves, the genes encoding the structural proteins and the non-structural proteins (nsps) are evident targets, but also others like the untranslated region 5′ UTR, involved in post-transcriptional regulation of gene expression (Baldassarre et al. Citation2020). Current studies are being developed regarding siRNAs (Chen et al. Citation2020b; Gu et al. Citation2020b; Zhang and Lu Citation2020). Nasal administration is a natural option for potential siRNA drugs.
Fourth line of defense: the war: the adaptive immune system
The human adaptive immune system
Adaptive immunity is activated when a pathogen manages to evade the innate immune system. An antigen-presenting cell (APC), such as a dendritic cell or macrophage, phagocytoses the antigen and presents it through its major histocompatibility complex (MHC) class II to immature helper T cells. Then helper T cells maturate and proliferate into several types of helper T cells, which either produce cytokines to guide cytotoxic T cells to pathogens and activate macrophages, or present antigens to B cells. Infected cells present antigens through their MHC class I to activated cytotoxic T cells that secrete toxins to destroy the cell (Figure ). Finally, memory T cells remember the antigen and might evolve to either helper or cytotoxic T cells if the antigen reappears. Other T cells, so-called suppressor T cells, have immunosuppressive capacities.
Figure 4. A dendritic cell presents antigens from the virus to a CD8+ T cell, which becomes activated and is able to recognize the same antigen on the surface of an infected cell, releasing perforin and granzymes that destroy the infected cell. (Reprinted from ‘Acute Immune Responses to Coronaviruses', by BioRender, August 2020, retrieved from https://app.biorender.com/biorender-templates/ Copyright 2021 by BioRender.)
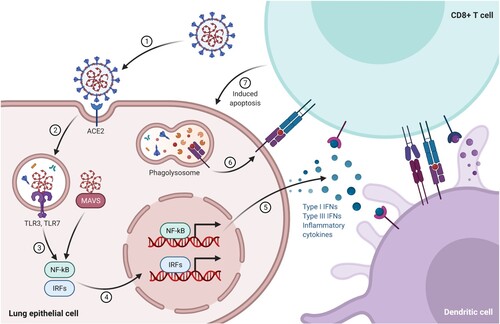
The mature helper T cells activate naïve B cells that have previously recognized the antigen. These B cells then proliferate and differentiate into plasma cells, which generate antibodies; plasmablasts, short-lived produced early in the infection that also produce (weaker) antibodies; B reg immunosuppresive cells; and memory B cells, that remember the antigen and quickly secrete antigen-specific antibodies if the antigen is newly detected (Figure ). Both B and T memory cells are formed when the infection ends (Alberts Citation2020).
Figure 5. (Left) Once they reach the target tissue, cytotoxic T cells detect antigens of the virus on the surface of infected cells and destroy them, eliminating virus factories. (Right) Antibodies secreted by B cells bind to the surface of the virus and block host entry (neutralizing antibodies). (Reprinted from ‘Recruitment of T and B Cells by Antigen-presenting Cells (APCs)', by BioRender, August 2020, retrieved from https://app.biorender.com/biorender-templates/ Copyright 2021 by BioRender.)
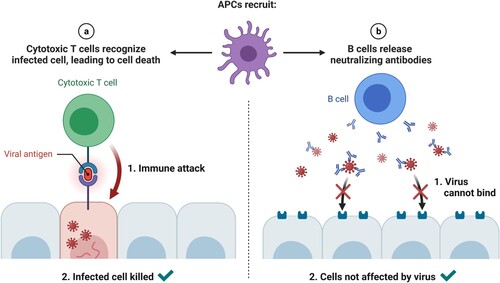
Ideally, the complex network that conforms our immune system and involves immune cells and the cytokines that communicate them, should be enough for blocking virus infections (Zhang and An Citation2007). However, strange things like trans-infection may happen. C-type Lectin Receptors (CLRs) and PRRs present in APCs could help in transporting infectious SARS-CoV-2 virions to target cells, specifically DC/L-SIGN CLRs. Interestingly, glycomimetic antagonists of DC-SIGN like PM26 are capable of inhibiting the interaction of the viral spike protein with the CLR and therefore prevent the SARS-CoV-2 trans-infection process (Thépaut et al. Citation2021).
Besides, studies carried out on SARS-CoV-2 affected patients show impairments of the immune system performance, and also responses differ among individuals depending on age, sex, comorbidities, possible cross-reactivities, genetic alterations, and other factors. The following section will delve into these facts.
The cytokine storm
When fighting the virus, the immune system makes use of inflammation and fever. However, overreaction might occur, and if signals to lower inflammation are lost, prolonged cell-to-cell (involving both innate and adaptive immune cells) interactions and amplification of a pro-inflammatory cytokine cascade may lead to a cytokine storm (Crayne et al. Citation2019; Barnes et al. Citation2020; Ledford Citation2020). It is still unsure what triggers and propagates the cytokine storm. One possible factor could be the aberrant activation of neutrophils, the most abundant leukocyte in peripheral blood, and the expelling of NETs. Exorbitant NETs may precipitate inflammatory reactions that damage nearby tissues, promotes micro thrombosis, and results in permanent organ damage in lungs, kidneys, and cardiovascular system. In fact, neutrophilia is a predictor of poor outcomes in COVID-19 patients. Related to this, host DNA damage promoted by SARS-CoV-2, and the virus components themselves can bind several cytosolic PRRs ending in pyroptosis, which causes cytokine and DAMP release. As a consequence, dendritic cells, macrophages, natural killer cells, neutrophils, and B and T cells may be damaged or activated and provoke inflammation, coagulation, and immunity dysfunction. In the course of it, transcription factors like NF-κB and IRF3 are activated, and if not quickly and properly controlled may culminate in septic shock, multiple organ failure, and finally death (Tang et al. Citation2020a).
Many cytokines are involved in the cytokine storm shown in severe COVID-19 cases, where increased plasma concentrations of IL-1β, IL-2, IL-6, IL-7, IL-8, IL-10, IL-17, IFN-γ, IFN-γ-inducible protein 10, monocyte chemoattractant protein 1 (MCP1), G-CSF, macrophage inflammatory protein 1α, and TNF-α are present. Besides general immunosuppressive therapies, novel treatments specifically targeting cytokines are being investigated to temper the cytokine storm. Among them, IL-6 has emerged as the main target for COVID-19 treatment. Many anti-IL-6 drugs are being tested (Hodgson Citation2020), such as IL-6 antagonists sarilumab and tocilizumab Ab that bind to both the membrane (mIL-6R) and soluble (sIL-6R) IL-6 receptors (Avci et al. Citation2018), or baricitinib, a selective inhibitor of the JAK1/JAK2 kinases through which IL-6 signals when binding its receptor (Mogul et al. Citation2019; Favalli et al. Citation2020).
A meta-analysis carried out by the WHO Rapid Evidence Appraisal for COVID-19 Therapies (REACT) Working Group over more than 10,000 COVID-19 patients shows that treatment with IL-6 antagonists like tocilizumab and sarilumab, correlated with lower 28-day all-cause death in hospitalized patients (working group Citation2021). Other studies also show benefits in treating severe COVID-19 patients with tocilizumab or sarilumab for both primary and secondary outcomes (Gordon et al. Citation2021). The RECOVERY trial has demonstrated that a combined treatment of a systemic corticosteroid like dexamethasone and tocilizumab notably lessens mortality in COVID-19 patients with hypoxia and serious inflammation, with a reduction of one-third for patients assisted with oxygen and of one half for those with invasive mechanical ventilation (Horby and Pessoa-Amorim Citation2021b). As of June 2021, the FDA has authorized emergency use of tocilizumab – Actemra/RoActemra – as medication of hospitalized COVID-19 patients treated with systemic corticosteroids and oxygen ( Coronavirus (COVID-19) Update: FDA Authorizes Drug for Treatment of COVID-19 Citation2021).
Apart from attacking the inflammatory molecules for blocking their action, other strategies can be observed, such as targeting the pathways that lead to the cytokines secretion. The nuclear factor kappa-light-chain-enhancer of activated B cells (NF-κB) and the nuclear factor of activated T cells (NFAT) are families of transcription factors that influence the immune response (Fisher et al. Citation2006; Liu et al. Citation2017). Both NFAT and NF-κB may bind to promoter regions of DNA and together with other transcription factors, allow the expression of genes related to inflammation. Specifically, NF-κB promotes the release of cytokines and chemokines by inducing the expression of pro-inflammatory genes, intervenes in inflammasome (intracellular proteins that respond to PAMPs and DAMPs, and induce the secretion of pro-inflammatory cytokines) regulation and regulates the survival, activation, and differentiation of immune system cells (innate and inflammatory T cells). The NF-κB pathway may be activated by responding to stimuli such as ligands of cytokine receptors, pattern-recognition receptors (PRRs) or T cell receptors (TCR) and B cell receptors (BCR). Cytokines such as tumor necrosis factor alpha (TNF-α) or pathogen-associated molecular patterns (PAMPs) such as lipopolysaccharide (LPS) are examples of activators of NF-κB. We can think of inhibiting NF-κB to lessen inflammation; however, NF-κB is essential for normal immune responses and cell survival and therefore its inhibition might originate severe side effects, so the specific mechanism that triggers pathological activation of NF-κB in SARS-CoV-2 should be known for precise attack.
Moreover, the occupation of ACE2 receptors by SARS-CoV-2 and the consequent increase in Angiotensin II is able to trigger inflammation through NF-κB and IL-6-STAT3 activation, initiating the IL-6 amplifier, a positive feedback cycle involved in the hyperactivation of NF-κB finally causing the cytokine storm. This inflammation pathway is strengthened with age, and IL-6 is a marker of cellular senescence (cell growth arrest due to stress factors that alter its gene expression (Campisi and d'Adda di Fagagna Citation2007)), which could explain the positive correlation between old age and COVID-19 severity, therefore the relevance of targeting the molecules contributing to the IL-6 AMP (Hirano and Murakami Citation2020).
Cyclophilin A (CyPA) (Nigro et al. Citation2013) is an intracellular protein that can also be secreted by cells in response to inflammatory stimuli. CyPA regulates protein folding and trafficking, as well as the infection and replication of several human viruses. High CyPA expression is a marker of poor outcome of patients with inflammatory diseases. Cyclosporine A (CsA) is a widely used immunosuppressant in transplantation and rheumatic drug (Osman et al. Citation2011). CsA together with CypA can sequester and inhibit the calcineurin phosphatase, which would prevent the nuclear translocation of NFAT and the dephosphorylation of IkB-α, avoiding the translocation of NF-κB (Lim et al. Citation2016). Recent work has shown this CsA-CypA complex could reduce the hyperinflammatory phase in COVID-19 (Guisado-Vasco et al. Citation2020).
The mammalian target of rapamycin (mTOR) signaling pathway is involved in regulating the cellular growth and proliferation in response to nutrients. mTOR clinically approved inhibitors extend lifespan in model organisms and protect against a wide list of age-derived pathologies including cancer, metabolic, and cardiovascular disease; for example, rapamycin and derivatives are already employed for cancer treatment (Johnson et al. Citation2013; Schreiber et al. Citation2016). One possible cause for the cytokine storm in COVID-19 is the antibody-dependent enhancement (ADE), which would imply suboptimal antibody responses from sub-neutralizing titers of antiviral host antibodies including non-neutralizing antibodies and cross-reactive antibodies against SARS-CoV-2 produced by activated memory B cells (from a previous infection). These antibodies bind the virus inefficiently or with weak affinity, accomplishing virus entry into immune cells through phagocytosis, followed by a breakdown of the fragile bound of the complex and the consequent virus escape from immunity and replication. mTOR inhibitors may limit the proliferation of memory B cells, therefore reducing early cross-reactive antibody production and thus antibody-dependent enhancement (Arvin et al. Citation2020; Ramaiah Citation2020; Zheng et al. Citation2020). Clinical trials show that selective low-dose TOR complex 1 TORC1 inhibition decreases the incidence of infections of all types, improves influenza vaccination responses, and up-regulates antiviral immunity in the elder population, results that could be extrapolated to the COVID-19 disease (Mannick et al. Citation2018). Also, the key tumor-suppressor p53 protein activators or microRNAs (miRNAs) that mimic p53 and can target mTOR are candidates to inhibit SARS-CoV-2 replication (Ramaiah Citation2020).
Cross-reactivity
Antibodies serological testing is easy to perform and therefore is usually accomplished to examine the immunological state and response of an individual against SARS-CoV-2. Nevertheless, since antibodies evolution is not yet fully characterized in COVID-19, it seems clear that immunity cannot be assessed by antibodies presence or even absence. Besides, antibody-dependent enhancement of disease, which enables viral entry into cells bearing Fcγ receptors and might amplify infection if the virus is not degraded but replicated inside the cell, has already been presented, and cross-reactive antibodies presence may be one cause (Arvin et al. Citation2020).
On the other hand, some studies suggest that a relatively high proportion of SARS-CoV-2 non-exposed individuals present T cells that react to SARS-CoV-2, probably from seasonal coronaviruses responsible for common colds, what could confer some degree of immune protection against COVID-19 (Grifoni et al. Citation2020; Sagar et al. Citation2021). Specifically, mild COVID-19 convalescent patients have been found to exhibit robust memory T cell responses against both internal – the nucleocapsid – and surface – the membrane and the spike – virus proteins months after infection, even when no circulating SARS-CoV-2 specific antibodies are detected. Moreover, cross-reactive T cell responses against SARS-CoV-2 surface proteins appear in over one-fourth of unexposed healthy blood donors (Sekine et al. Citation2020). Also, high levels of SARS-CoV-2 responsive T cells are associated with the absence of symptomatic SARS-CoV-2 disease, and the number of individuals showing high T cell responses is inversely proportional to age, what could relate to higher incidence and severity of COVID-19 in the elderly (Wyllie et al. Citation2021). As already introduced, T cell epitope identification is a helping tool for detecting both cross-reactive and specific SARS-CoV-2 T cell immunity (Nelde et al. Citation2020).
Effective adaptive immunity creates immunological memory that reacts to previously exposed pathogens and interestingly can also affect the immune response and the course of infection of unassociated pathogens, leading to either protective immunity or immunopathology. This process is called heterologous immunity and may be induced by vaccination through trained immunity by reprogramming innate cells such as natural killer cells (NK), natural killer T cells (NKT), and monocytes. Other procedures such as modulation of helper, regulatory and memory T cells, cytokine responses, the concentration of antibodies, and even cross-reactive antibodies and cross-reactive epitopes may also be considered as mechanisms originating heterologous immunity (Sharma and Thomas Citation2014; Agrawal Citation2019). For example, BCG (Bacille Calmette Guerin) vaccine to prevent tuberculosis is known to confer heterologous protective immunity by inducing trained immunity (Arts et al. Citation2016; Moorlag et al. Citation2019), and a correlation between BCG vaccinated population and less susceptibility to COVID-19 has been observed (Curtis et al. Citation2020; O’Neill and Netea Citation2020). Besides, DTP (diphtheria, tetanus and pertussis) vaccine has been found to exhibit cross-reactive epitopes with SARS-CoV-2, including many cytotoxic and helper T cell epitopes and potentially neutralizing B cell epitopes in conserved antigenic domains of the virus spike protein. Since the DTP vaccine is widely administered in children worldwide, DTP cross-reactivity could be one contributor to the youngest population protection against SARS-CoV-2 (Reche Citation2020). Also, mumps titers related to the Merck MMR II vaccine (licensed in 1979) has been found inversely associated with COVID-19 severity (Gold and Baumgartl Citation2020), and a high rate of pneumococcal vaccination inversely correlates with population morbidity and mortality due to COVID-19 (Root-Bernstein Citation2020).
Previous exposure to other unrelated pathogens and vaccines such as influenza is being considered as a source of cross-reactivity (Salem and El-Hennawy Citation2020; Marín-Hernández et al. Citation2021; Zanettini et al. Citation2021), for example, it has been demonstrated in-vitro that the quadrivalent inactivated influenza vaccine can induce a trained immunity response positively impacting SARS-CoV-2 infection improving some cytokine responses (Debisarun et al. Citation2020).
Convalescent plasma
It seems obvious to think about using hyperimmune plasma from recovered COVID-19 patients with sick individuals that may be in an acute phase of the disease but still have not developed an effective immune response. This plasma will likely contain SARS-CoV-2 specific antibodies that would help in defeating the virus. This hypothesis is being demonstrated in a clinical essay carried out by a group of over 30 hospitals in Spain, with preliminary results showing that administering hyperimmune plasma may reduce the probability of disease progression to mechanical ventilation, ICU admission or death, for patients with less-advanced clinical presentations, but no benefit in critically ill patients admitted to ICU (Avendano-Sola et al. Citation2020). Another study at the Mayo Clinic with over 35,000 patients revealed that mortality rate was reduced in patients transfused within three days of COVID-19 diagnosis compared with patients transfused four or more days after diagnosis. Also, convalescent plasma with higher antibody levels was associated with reduced mortality (Joyner and Senefeld Citation2020). A recent study confirms that early administration of high-titer convalescent plasma is a key in decreasing the disease advance in mild COVID-19 old patients (Libster et al. Citation2021).
In addition, it is surprising to find how long-lasting immunoglobulin G (IgG) antibodies against SARS-CoV-2 disappear in a relatively short time span of several months, in a proportion of recovered patients. It has been discovered an absence of germinal centers in lymphoid organs of severe COVID-19 patients, which causes that long-lived antibody-secreting plasma cells and memory B cells are not being produced, so a dysregulated humoral immune is presented. The abnormal absence of germinal centers correlates with an impaired follicular helper T (TFH) cell differentiation and aberrant lymphoid TNF-α production (Kaneko et al. Citation2020).
(Tests, tests, tests …)
COVID-19 asymptomatic and presymptomatic patients may be responsible for over one-half of all the contagions, with all the implications this proportion presents (Moghadas et al. Citation2020; Johansson et al. Citation2021). Besides, presymptomatic cases are more infectious than after symptom onset (Li et al. Citation2021a). The genetic material of SARS-CoV-2 can be detected several weeks or even months after infection; however, live virus culture has not been fruitfully achieved after nine days since symptoms onset, which marks a theoretical deadline for a patient’s infectious state, with asymptomatic patients showing faster viral clearance. Therefore, quarantines or isolation periods of ten days without repeating confirmation RNA tests could be justified, though this time span could vary depending on the SARS-CoV-2 variant (Cevik et al. Citation2021).
Diagnostic evaluations like reverse transcription polymerase chain reaction (RT–PCR) and antigen tests are carried out searching for the presence of fragments of the SARS-CoV-2 RNA and specific virus proteins, respectively, usually via nasopharyngeal swabs, showing active virus infection. Besides, serology tests look for antibodies that imply past (IgG) or active (IgM) infection (Figure ). However, observation of a viable virus, capable of replicating itself, is the most trustworthy benchmark of contagiousness.
Figure 6. SARS-CoV-2 PCR and serological tests processes. (Reprinted from ‘COVID-19 Fact Sheet', by BioRender, August 2020, retrieved from https://app.biorender.com/biorender-templates/ Copyright 2021 by BioRender.)
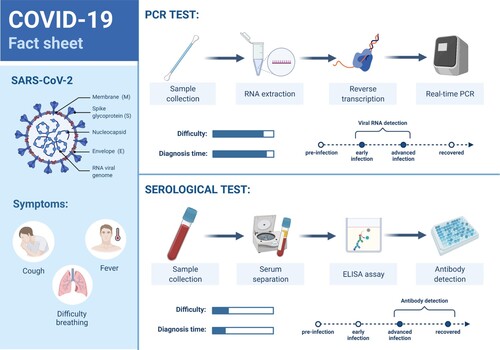
RT–PCR tests have high specificity but moderate sensitivity, and their results depend on the patient viral load, therefore the possibility of a false negative increases around the day of symptoms onset (usually about five days after infection) and when symptomatology diminishes at the final course of the infection, so an RT–PCR result initially negative might become positive after some days, and so the need for preventive quarantine of straight contacts of a COVID-19 positive, even if asymptomatic. Antigen tests are less accurate and could give false-negative results due to a low or a variable viral load and the variability in the sampling process, but are faster and simpler to process. On the other side, antibodies can be detected several days or even weeks after infection and so their false negative window spans larger than PCR. Antibody tests must be designed to check for antibodies targeting specific SARS-CoV-2 antigens (avoiding cross-reactivity). Though most tests detect antibodies against the spike or the nucleocapsid proteins, it is possible to point to other immunogenic proteins like Mpro, which implies cell infection and therefore might correspond with tissue damage. Also, it is interesting to detect antibodies from saliva, and include mucosal IgA that would infer protection of the upper respiratory tract (Martínez-Fleta et al. Citation2020). Serology tests should not be employed for COVID-19 diagnosis but to check whether an individual has had the infection, as well as for seroprevalence studies and to monitor the evolution of the immune response, included that from vaccination (Jessica et al. Citation2020). Depending on the patient symptomatologic state and initial test results, both diagnostic and serology tests may be complementary. New faster diagnostic tests are being commercialized, which despite a somewhat higher false-negative rate help to quickly isolate infected persons, therefore, cutting the transmission chain of the virus (La Marca et al. Citation2020; Alemany et al. Citation2021). Quick, frequently performed affordable autotests like saliva tests will surely help in ending with the pandemic bad dream. Of note, saliva load has been discovered as a potential biomarker of COVID-19 severity and mortality, and a notably higher saliva viral load is detected in COVID-19 patients with risk factors (Silva et al. Citation2021). Also of interest, neutralizing antibodies can be detected with serology tests, being authorized by the U.S. Food and Drug Administration (FDA) for the first time in November 2020 (Coronavirus (COVID-19) Update Citation2020).
Last line of defense: fighting the consequences of the infection
COVID-19 phases
COVID-19 starts with viral replication after infection by SARS-CoV-2. Initially, an incubation period of several days – which vary among virus variants – includes a first lapse in which infection goes undetected, followed by a virus detectable (by PCR) time correlated with infectiousness, even before symptoms – if any – appear. Around 80% of COVID-19 patients exhibit a non-robust viral replication and early interferon response, and are able to solve the infection in about two weeks’ time. If the immune system does not respond properly, the COVID-19 pathogenesis progresses through three phases, namely pulmonary, proinflammatory, and prothrombic. In the pulmonary phase, the infection may lead to pneumonia and Acute Respiratory Distress Syndrome (ARDS). In the pro-inflammatory phase, the cytokine storm and subsequent systemic inflammation may conduct to acute lung injury. Finally, the prothrombic phase causes platelet aggregation and thrombosis, ending in coagulopathy and multi-organ failure that can lead to fatal outcome (Khadke et al. Citation2020; Griffin et al. Citation2021; Lee and Choi Citation2021).
Respiratory and vascular problems
Lung damage and blood clots are one of the main severe consequences of the COVID-19. Actually, COVID-19 is recognized as a vascular disease. Consequently, the virus targets many human organs and systems and may cause heart and renal failure, cerebrovascular accident, and Acute Respiratory Distress Syndrome (ARDS) (Lee et al. Citation2020). Moreover, COVID-19 patients might undergo long-term sequalae affecting health beyond respiratory and cardiovascular disorders, including neurological and mental, metabolic and gastrointestinal complications, musculoskeletal pain and fatigue or anemia, in direct proportion to COVID-19 severity but also reaching mildly affected individuals (Al-Aly et al. Citation2021).
ARDS implies hypoxemic respiratory failure and may be produced by a SARS-CoV-2 derived pneumonia. ARDS is one of the most common causes of death in the inflammatory phase of COVID-19, especially affecting the older and also among patients admitted to intensive care units (ICU) (Figure ) (Torres Acosta and Singer Citation2020).
Figure 7. A healthy alveolus (left) and a damaged alveolus (right) in the acute phase of ARDS. An excess of cytokines increases the permeability of the capillaries leading to pulmonary edema (in light brown) that hinders gas exchange in the alveoli, ending in dyspnea and ARDS. (Reprinted from ‘Acute Respiratory Distress Syndrome (ARDS)', by BioRender, July 2020, retrieved from https://app.biorender.com/biorender-templates/ Copyright 2021 by BioRender.)
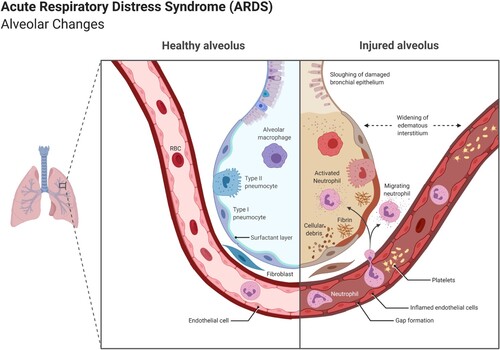
On the cardiovascular side, angiotensin II and angiotensin (1-7) control blood pressure. ACE2 keeps the balance between both hormones; however, it seems that the way SARS-CoV-2 enters the host cell through the ACE2 receptor provokes an imbalance with an excess of angiotensin II that makes blood more viscous, leading to clots. Heparin has already been pointed out as an effective anticoagulant drug. The TRV027 molecule, an AT1 receptor-selective agonist that disrupts overactivation caused by SARS-CoV-2, presents a dual-action blocking angiotensin II activity and mimicking angiotensin (1–7), and is currently under test. The type 1 angiotensin receptor AT1 is used by angiotensin II to increase blood pressure by inducing vasoconstriction and to promote inflammation and fibrosis (London researchers to test promising coronavirus treatment Citation2020). Studies show that severely ill COVID-19 patients exhibit an elevated risk for venous thromboembolism even when treated with prophylactic anticoagulation drugs (Bellmunt et al. Citation2020). Besides, a retrospective study involving over 11,000 hospitalized COVID-19 patients treated with angiotensin-converting enzyme inhibitor (ACEI) and angiotensin II receptor blocker (ARB) exhibits a decrease in mortality rate, especially among hypertensive patients and for ARB treatment. As a consequence, ACEI/ARB should not be commonly removed for this specific group of patients (Roy-Vallejo et al. Citation2021).
The ACE2 receptor virus gateway is also broadly expressed in endothelial cells that traverse multiple organs. Recruited immune cells can originate widespread endothelial dysfunction after a SARS-CoV-2 infection, implying microvascular dysfunction with vasoconstriction that cause organ ischemia, inflammation with tissue edema and a pro-coagulant state. Endothelial cells death containing viral elements, and inflammatory dead cells accumulation would suggest that SARS-CoV-2 infection induces endotheliitis in several organs, which would explain the systemic impaired microcirculatory function (Figure ). Also, induction of apoptosis and pyroptosis could have a crucial function in endothelial cell injury in COVID-19 patients. Therapies to stabilize the endothelium while coping with viral replication seem appropriate, especially with anti-inflammatory cytokine drugs, ACE inhibitors and statins (Varga et al. Citation2020). In fact, statins, a common drug for decreasing blood cholesterol, are showing lower SARS-CoV-2 infection-related mortality when administered before hospitalization (Masana et al. Citation2020).
Figure 8. ACE2 receptors facilitating SARS-CoV-2 entry, causing, among others, vascular effects like endothelial dysfunction, vascular inflammation, micro and macrovascular thrombosis and vasospasm. (Reprinted from ‘Expression of ACE2 Receptor in Human Host Tissues', by BioRender, August 2020, retrieved from https://app.biorender.com/biorender-templates/ Copyright 2021 by BioRender.)
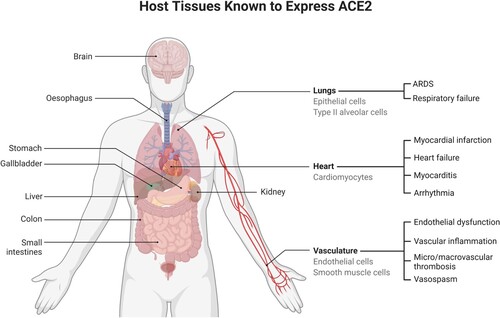
The von Willebrand factor (vWF) is a multimeric protein released by the endothelial cells that operates in conjunction with other components of the coagulation system and helps platelets adhere for blood clotting. In severe COVID-19 patients, an evident increment in plasma vWF levels, together with a decline in the plasma activity of ADAMTS13, the vWF-cleaving protease has been found, which is consequent with the microvascular thrombosis caused by SARS-CoV-2 (Ward et al. Citation2021).
On the other side, the presence of antiphospholipid (aPL) antibodies – able to promote a potentially fatal thrombosis – has been found in the serum of half of the hospitalized COVID-19 patients within a recent study. aPL antibodies titers positively correlate with neutrophil overactivity and NETs, platelet counts and severity of respiratory disease. The existence of aPL antibodies in COVID-19 patients may have implications for instance in treatments with convalescent plasma (Zuo et al. Citation2020b).
Melatonin is a bioactive molecule synthesized from tryptophan in the pineal gland, and actually in any cell since it is synthesized in mitochondria. It effectively points to an immense span of health targets ranging from sleep disorders (chronobiotic), delirium, atherosclerosis, respiratory diseases including acute respiratory stress, and viral infections (as anti-inflammatory, antiapoptotic, immunomodulatory, and potent antioxidant). Immune cells such as macrophages/monocytes in an inflammatory environment cannot synthesize melatonin and this could lie behind their inability to prevent the cytokine storm and neutralize free radicals inducing lung damage. Importantly, melatonin moderates the removal of the angiogenic factor VEGF in vascular endothelial cells, reducing edema and the leakage of immune cells from blood vessels contributing to the integrity of the vascular endothelial barrier, essential for immunoregulation within alveoli. Melatonin may also prevent sepsis injury and positively impact heart diseases including hypertension. Moreover, it exerts neurological protection by reducing the cerebral inflammation, cerebral edema and brain–blood barrier permeability. Melatonin synthesis sensibly decreases with age and children have a much higher melatonin level. All the aforementioned positive impacts on symptoms associated with COVID-19, together with its high safety profile makes melatonin an excellent candidate to be evaluated in treating the disease (Reiter et al. Citation2020a, Citation2020b; Zhang et al. Citation2020c).
Inflammation
Inflammation is a natural defensive response of the organism to infection or tissue damage, that through inflammatory mediators dilates blood vessels to boost the blood flow and permit the infiltration of lymphocytes, fluid and proteins to the site of infection. However, if this process gets out of control it may lead to a cytokine storm, related to poor clinical prognosis (Mahmudpour et al. Citation2020; Pamukçu Citation2020). The inflammatory phase in COVID-19 follows the infection and the pulmonary phases, and may culminate in a fatal prothrombotic final stage (Taboada et al. Citation2020; Lee and Choi Citation2021a). Intriguingly, the SARS-CoV-2 S protein by itself is able to damage the endothelium by downregulating ACE2, what would lessen the virus infectivity but also impair the renin-angiotensin system (RAS), finally resulting in endothelial injury and inflammation or endotheliitis (Lei et al. Citation2021). Apparently, this would not have impact on spike-producing vaccines against COVID-19, since the generated spike would be anchored to the host cell surface, unable to bind ACE2 receptors.
Corticosteroids are common drugs treating chronic inflammatory pathologies, that bind to glucocorticoid receptors and reverse histone acetylation of activated inflammatory genes, switching them off (Barnes Citation2006). Corticosteroids may modulate immune-mediated lung injury; however, there is uncertainty about their effectiveness in COVID-19, which depend on the right dose, at the right time, in the right patient, despite their frequent use in syndromes related to COVID-19, such as SARS, MERS, influenza, or community-acquired pneumonia. Dexamethasone, a low cost, safe and widely available corticosteroid, has shown benefits in COVID-19 patients that receive oxygen by reducing mortality, especially with those at a later stage of the disease where active virus replication has given way to immunopathology and is actually being widely used. However, no benefit arises for patients not assisted with oxygen; moreover, they might suffer possible harm from the treatment (Horby et al. Citation2021a). Recently, it has been reported the benefits of colchicine, an alkaloid used against gout, viral pericarditis and coronary diseases with anti-inflammatory properties, for orally treating non-hospitalized COVID-19 patients, in inhibiting the cytokine storm associated with SARS-CoV-2. Statistically significant results show colchicine notable impact in reducing hospitalizations by 25%, mechanical ventilation need by 50%, and deaths by 44% (Tardif et al. Citation2021).
Neurological effects
Among the wide variety of consequences on the human organism caused by the SARS-CoV-2, there is still place for fearsome neurological complications that arise with high frequency, even in the long term. Most common neurologic manifestations include myalgia, headache, encephalopathy, dizziness, dysgeusia, and anosmia. Other symptoms such as strokes, movement disorders, motor and sensory deficits, ataxia, and seizures may also appear but are less common. Clinical studies show that severe COVID-19 patients are in higher risk of developing neurologic manifestations, and that encephalopathy is associated with augmented morbidity and mortality, unrelated to respiratory disease severity (Liotta et al. Citation2020). Cognitive failures such as loss of memory, disorientation or concentration problems (‘brain fog’), as well as fatigue, dyspnea, and sleep disorders have also been reported as a consequence of this hyperactive virus (Garrigues et al. Citation2020). People recovered from COVID-19 perform worse than expected on cognitive tests, exhibiting deficits on higher cognitive functions such as reasoning, planning or selective attention, also with slower response time latencies, which is in accordance with the long-COVID symptoms just previously mentioned (Hampshire et al. Citation2021). EEG analyses of COVID-19 patients suffering from neurologic disorders like encephalopathy, seizures and status epilepticus have been performed, showing abnormalities like slowing, periodic discharges, or epileptiform discharges, revealing localized dysfunction, nonspecific encephalopathy, and cortical irritability. Interestingly, the frontal lobe, in charge of voluntary movements, and cognitive skills such as language, memory, and emotional expression registered the most common EEG abnormalities. In fact, EEGs may act as a useful tool to evaluate early cerebral impact in the COVID-19 disease. These neurological malfunctions might be caused by an extensive brain inflammation, by poor blood flow or by inflammation of the brain blood vessels, and/or by hypoxia, not discarding other reasons like possible virus ingress in the central nervous system as already mentioned in a former section (Antony and Haneef Citation2020; Cecchetti et al. Citation2020; Meinhardt et al. Citation2020; Rhea et al. Citation2020; Song et al. Citation2021). In fact, as has been mentioned previously the spike protein itself, and more specifically the S1 subunit, could lie behind endothelial damage with neurological implications, and particularly on brain endothelial cells might also provoke a pro-inflammatory response that negatively impact the blood–brain barrier function (Buzhdygan et al. Citation2020; Nuovo et al. Citation2021). Moreover, SARS-CoV-2 infection could represent a risk factor triggering neurodegenerative disorders such as Parkinson, multiple sclerosis, and narcolepsy in susceptible individuals (Schirinzi et al. Citation2020). A study on eight patients who died of COVID-19 revealed brain barrier inflammation and anomalously activated microglia – the central nervous system (CNS) immune cells – which resembles that of Alzheimer’s disease. Dysfunction found in upper-layer cortical circuitry could associate with autism spectrum disorders and cognitive deficits. Neurons exhibited distinctive perturbations but glial cells’ – the white matter that gives support to neurons – were similar to those in chronic CNS diseases such as Alzheimer, multiple sclerosis, or autism. Also, enrichment of differentially expressed genes of neurodisorders and traits, particularly in cognition, schizophrenia, and depression were identified. Despite all the above, no trace of SARS-CoV-2 was found in the brain (Yang et al. Citation2021).
Conclusions
Antivirals may target any protein and process associated with the virion replication. Also, the host proteins that work alongside the virus may become drug targets. Repurposing drugs from other disorders is being assessed by artificial intelligence against COVID-19. Unluckily, prophylactic oral remedies are still to be discovered. Besides, SARS-CoV-2 is able to jam the host immunity by handling epigenetic-controlled mechanisms that could be neutralized by specific drugs. Other epigenetic tools like siRNA could point to the virus mRNA.
The cytokine storm is the core of the immune overreaction. Approaches to strike the inflammatory molecules, especially IL-6 as well as to interfere the pathways associated with the cytokine secretion are being observed. Though the vast majority of COVID-19 patients progresses to a satisfactory resolution of the infection, some of them will traverse through pulmonary, proinflammatory, and prothrombic phases. Corticosteroid dexamethasone is by now one of the few drugs that successfully copes with inflammation.
Initially thought of as a respiratory disease, COVID-19 real face is vascular, with a wide tropism affecting almost any organ, including the neurological system with a bunch of symptoms that could extend to long-duration covid.
Exposure to different pathogens as well as vaccination programs may confer heterologous immunity that could somewhat be protective against SARS-CoV-2 infection; however, cross-reactive antibodies could also contribute to the antibody-dependent enhancement of COVID-19.
COVID-19 risk factors
Thanks to the many clinical and genetic analyses that are being carried out in the quite elevated number of COVID-19 patients, the majority of the population is already aware of the most common risk factors to SARS-CoV-2 susceptibility and severity such as age, sex (male), obesity or previous pathologies including diabetes and cardiovascular diseases, especially hypertension. In this section, we will review the main known determinants that influence COVID-19 susceptibility, severity, and prediction of the course of the disease.
Symptoms and clinical biomarkers
In hospitalized patients, clinical trials show that the worst prognosis is associated with the isolated set of symptoms consisting of fever, cough, and dyspnea (which frequently also accompanied the following other symptomatology); predominant diarrhea, vomiting and/or abdominal pain also present bad prognosis; on the contrary, ageusia and/or anosmia, followed by arthromyalgia, headache and/or sore throat symptoms seem to offer better prognosis (Rubio-Rivas et al. Citation2020).
Asymptomatic and severely affected COVID-19 patients show different immune profiles. In a cohort of 130 individuals, single-cell multi-omics analysis on almost 800,000 peripheral blood mononuclear cells (PBMC) has been performed, including single-cell sequencing, surface proteome, and lymphocyte antigen receptor analyses. Asymptomatic patients show augmented levels of B cells in nasal mucosa, related to higher number of defensive IgA antibodies. B cells and helper T-cells are depleted in patients suffering severe COVID-19, in contrast to patients with mild to moderate symptoms who show raised levels of both immune cells. Finally, in hospitalized COVID-19 patients the shot up of monocytes and killer T-cells could end in lung inflammation, and also high levels of megakaryocytes, the platelet-producing cells are present (Stephenson et al. Citation2021).
Clinical analyses clearly show D-dimer, C-reactive protein (CRP), ferritin, lactate dehydrogenase (LDH), IL-6, TNFα, IL-1β, red cell distribution width (RDW), or procalcitonin (PCT) biomarkers of inflammation and coagulopathy associated with poor clinical outcomes, among others (Ayanian et al. Citation2020; Foy et al. Citation2020; Hu et al. Citation2020; Huang et al. Citation2020; Lippi and Plebani Citation2020; Tang et al. Citation2020b; Zhou et al. Citation2020b; Abers et al. Citation2021). Predictive scores have been developed to allow for enhanced prognosis in hospitalized COVID-19 patients, comprehending factors such as age, sex, dyspnea, oxygen saturation, neutrophil-to-lymphocyte ratio, and Chronic Kidney Disease Epidemiology Collaboration (CKD-EPI) equation (estimated glomerular filtration rate, related to renal failure) (Berenguer et al. Citation2021). Also, phase angle (PhA), together with standardized PhA (sPhA), a parameter that senses cell status (hydration) and is a biomarker for malnutrition and inflammation, is an accurate predictor of mortality risk in COVID-19 patients (Cornejo-Pareja et al. Citation2021). Hyperglycemia is a strong predictor of mortality in non-critical and even non-diabetic hospitalized patients. It may be directly induced by the SARS-CoV-2 that infects the pancreas cells through their ACE2 receptors, originating a transient diabetes, and by stress hyperglycemia. Patients suffering from diabetes or obesity present high levels of glucose in their blood that may impact the normal macrophages and monocytes behavior. Hence patient hyperglycemia should be monitored and controlled since it stimulates the infection and may worsen the inflammatory response (Carrasco-Sánchez et al. Citation2021). Even periodontitis, related to systemic inflammation, has been associated with poor disease outcomes including death in COVID-19 patients (Marouf et al. Citation2021). Inflammatory mitochondrial DNA (mtDNA) is released by injured organs, and circulating mtDNA has also been found a potential biomarker of poor COVID-19 outcomes ranging from ICU admission and intubation to mortality (Scozzi et al. Citation2021). Eosinophil count is a prognostic biomarker too, as well as lymphopenia (Liu et al. Citation2020; Tan et al. Citation2020). Artificial intelligence techniques may help in predicting the COVID-19 phases and even the so-called long-haul COVID so as to more efficiently adopt treatments (Patterson et al. Citation2021).
Obesity is characterized by an excessive fat accumulation that may negatively impact metabolism, the respiratory and the renal systems, also inducing a chronic low-inflammatory state that stimulates the development of cardiovascular diseases like coagulation or atherosclerosis, and diabetes mellitus or cancer. A dysregulated immune response may arise in individuals with obesity. Many studies show an increased mortality rate of obese COVID-19 patients, that present augmented values of parameters such as blood lymphocytes, IL-6, CRP, triglycerides (heart and metabolic function), cystatin C (renal function), alanine aminotransferase (ALT, liver function), and erythrocyte sedimentation rate (ESR, inflammation) (Kang et al. Citation2020; Kwok et al. Citation2020). However, a meta-analysis published in mid-2021 over more than 44,000 COVID-19 patients did not determine any association between male sex or obesity with mortality, but confirmed older age, smoking antecedents, and comorbidities such as hypertension, diabetes, cardiovascular, respiratory and renal diseases, and malignancy as mortality risk factors (Taylor et al. Citation2021). Also related to an individual physical condition, muscular strength lies behind the physical exercise’s preservative effect against COVID-19 in older people (Maltagliati et al. Citation2021).
Additional risk factors such as sociodemographic issues, comorbid conditions, and symptoms are also being studied (Ioannou et al. Citation2020).
Patient’s sex and age
Males vs. females
It is well known that general infectious disease incidence is often male-biased (Guerra-Silveira et al. Citation2013), COVID-19 is not an exception and men suffer from higher COVID-19 severity and lethality than women, though their infection rate is similar (Peckham et al. Citation2020; The COVID-19 Sex-Disaggregated Data Tracker Citation2021). Apart from lifestyle and comorbidities that affect men to a greater extent, other factors underlay this imbalance. Men with heart failure show more elevated plasma concentrations of ACE2 than women, what could extrapolate to a higher expression of ACE2 that would enhance SARS-CoV-2 cell entry (Sama et al. Citation2020). Also, there seems to be a clear difference in the construction of the immune response between men and women, with factors like the X-linked essence of many immune genes (genes on the X chromosome), such as the toll-like receptor 7 TLR7 – the endosomal innate immune sensor that detects single-stranded RNA as SARS-CoV-2’s and induces type I interferon and inflammatory cytokine production –, and the feminine reproductive hormones estrogen and progesterone and its metabolite allopregnanolone, that have anti-inflammatory effects, improve the performance of immune cells, stimulate antibody production, and reinforce respiratory epithelial cells (Klein and Flanagan Citation2016; Pinna Citation2021).
A study involving patients with moderate COVID-19 show men having higher plasma levels of innate immune cytokines like IL-8 and IL-18, together with a stronger induction of non-classical monocytes, and women with more robust CD8 T cell activation. Interestingly, a poor T cell response was associated with worse disease outcome in men, but not in women, and higher levels of innate immune cytokines like TNF SuperFamily member 10 (TNFSF10) and IL-15 were associated with worse disease progression in women, but not in men (Takahashi et al. Citation2020). Also, as already mentioned autoantibodies against type I interferons were found in around 10% of patients with severe COVID-19, and these patients were almost all men (Bastard et al. Citation2020). Another study examining host response gene expression show higher expression of B cell transcripts in females (since women present higher levels of B cells, and also tend to an increased inflammation responding to viral infections), and reduced B cell-specific and NK cell-specific transcripts and increased inhibitors of NF-κB signaling in males, perhaps not adequately lowering antiviral responses trying to reduce deleterious inflammation (Lieberman et al. Citation2020). Besides, the National Health Service in England has found among plasma donors that men produce higher levels of COVID-19 plasma antibodies than women (NHS Citation2020).
Testosterone seems to play a pivotal role in this male COVID-biased affection. Male COVID-19 patients show very low levels of circulating testosterone at hospitalization – compatible with secondary hypogonadism – which inversely correlate with poorer clinical outcomes, including ICU admission and death. Probably, as a consequence of the absence of the testosterone anti-inflammatory activity (Çayan et al. Citation2020; Salonia et al. Citation2021). Moreover, genetic polymorphism in the androgen receptor diminishing the receptor functionality would also have a relevant influence, in fact, normal testosterone levels could mask a low androgenicity problem. Severe COVID-19 patients with defective androgen signaling together with reduced levels of circulating androgens could potentially benefit from testosterone as adjuvant therapy (Baldassarri et al. Citation2021). Additionally, an altered lipid metabolism reflected in triglycerides depletion, along with high estradiol levels (shared with females), positively associated with IFN-γ (in charge of macrophage activation), negatively impact male COVID-19 patients’ prognosis (Schroeder et al. Citation2020).
Children and older people
Fortunately, children and young adults mostly suffer a milder version of COVID-19 compared to adults, although they are exposed to develop a life-threatening multisystem inflammatory syndrome (MIS-C) (Ahmed et al. Citation2020; Feldstein et al. Citation2020). Much has been speculated about the reasons, including heterologous immunity from past exposures to similar or even unrelated pathogens and from vaccination, and lower affinity of ACE2 receptors for SARS-CoV-2 binding (Nogrady Citation2020; Zimmermann and Curtis Citation2020). Another explanation implies a strong response from the innate immunity, involving interferon release and macrophages that may be delayed in adults. Studies carried out with pediatric COVID-19 patients show different antibody responses compared to adults, who present IgG, IgM and IgA antibodies against spike (S) protein, and IgG antibody against nucleocapsid (N) protein. By contrast, children generate IgG antibodies against the S protein but not for the N, a protein only visible for the immune system after cells are infected, what would suggest a limited propagation of the infection. Also, serum concentrations of interleukin-17A and interferon-γ appear inversely related to age, but not TNF-α or IL-6. Moreover, children present reduced neutralizing activity, what is compatible with a powerful innate response and a consequent quick virus elimination (Pierce et al. Citation2020; Weisberg et al. Citation2021). Innate cell profile in patients with mild infection show an augmented activation of neutrophils and a declined circulation of all monocyte types, dendritic cells and natural killer cells in children, while adults only exhibit depletion in the non-classical monocyte type (Neeland et al. Citation2021). A study involving a family infected with COVID, formed by the parents and three children gave intriguing results, with the youngsters presenting SARS-CoV-2 antibodies and even undergoing mild symptoms but repeatedly testing negative for RT–PCR. This would suggest a very effective immune response in children able to halt virus replication before it reached the swab diagnostic test detection threshold, also with implications in the detection strategies of infected children and consequent spread of the disease (Tosif et al. Citation2020; Hippich et al. Citation2021). Besides, cross-reactive (non-specific) and polyreactive secretory antibodies SIgA/IgA and SIgM/IgM against SARS-CoV-2 spike protein subunits S1 and S2 and nucleocapsid protein have been abundantly detected in human milk, as well as lactoferrin antiviral proteins, what might passively protect breastfed infants (Demers-Mathieu et al. Citation2020; Root-Bernstein Citation2020). However, as time goes by in the pandemic era, new consequences appear, and children have been found to develop neurological symptoms in the course of the disease and even once recovered (Lindan et al. Citation2020). Also, in children – as in adults – endothelial cells of small blood vessels may be damaged by SARS-CoV-2, leading to thrombotic microangiopathy (TMA) (Diorio et al. Citation2020). Results from autopsies in children with COVID-19 show the virus capacity of infecting several organs including brain and intestine, that is suggested to have a direct impact on the pathogenesis of MIS-C (Duarte-Neto et al. Citation2021).
A seroepidemiological survey in Spain arbitrarily recruited over 61,000 individuals during its first pandemic wave, collecting information on COVID-19 related symptoms as well as blood samples, with the aim of outlining asymptomatic profiles. Results show an overall of almost 30% of asymptomatic infections, especially among males, younger and older people, and smokers (see Nicotine section) (Pérez-Gómez et al. Citation2021).
In any case, children do seem to get infected with a similar susceptibility than adults, and they can also act as transmitters of the infection like adults, however, due to their high asymptomatic profile they are frequently undetected and therefore might constitute a relevant infection focus, which specially materializes at school when insufficient anti-COVID measures are taken (Hallal et al. Citation2020; Hippich et al. Citation2020; Laxminarayan et al. Citation2020; Li et al. Citation2021a). The latter is also extendible to young adults and university campuses (Losina et al. Citation2020; Lu et al. Citation2021).
On the other hand, it is natural to think that factors associated with aging relate to infectious disease severity. In the case of COVID-19 we can point to comorbidities, cardiovascular malfunction, higher density and affinity of ACE2, antibody-dependent enhancement, immunosenescence (decreased immunological competence) and inflammaging (low-grade proinflammatory state), lower levels of vitamin D and shorter telomeres length (Pietrobon et al. Citation2020; Zimmermann and Curtis Citation2020; Sanchez-Vazquez et al. Citation2021). Cellular senescence, which stands for cell proliferation arrest due to stress factors like DNA damage or dysfunctional telomeres, protects the organism from cancer but also causes loss of regenerative capacity and therefore ageing. With their gene expression altered, senescent cells secrete pro-inflammatory cytokines and chemokines, that direct and negatively influence the course of COVID-19. Immune cells senescence or immunosenescence intensify age-related immunity vanishing facing novel pathogens and vaccines. Geroprotector drugs like mTOR inhibitors, already introduced, suppress the excess of inflammation related to senescence and enhances innate immunity, contributing to revert the effects of ageing (Mannick et al. Citation2018; Cox et al. Citation2020; Di Micco et al. Citation2021).
Genetic factors
Since the genome codes for the operation of the whole organism, it is palpable that genetic variations must be analyzed regarding response to virus infections.
Genetic analyses would show a relation between ABO blood groups and Covid-19 susceptibility, with a slight advantage from blood group O versus group A, however, any blood group appears appreciably associated with mortality risk (Barnkob et al. Citation2020; The Severe Covid-19 GWAS Group Citation2020; Badedi et al. Citation2021; Lehrer and Rheinstein Citation2021). With respect to coagulopathies, correlated with COVID-19 severity, altered platelet gene expression and increased platelet aggregation have been found in COVID-19 patients, which may contribute to the disease pathophysiology (Manne et al. Citation2020). Moreover, conventional clinical assays can be outperformed by plasma proteomic signatures, able to identify potential biomarkers differentially expressed according to the severity level of COVID-19 patients, among them inflammation modulators, complement factors, the coagulation system, and pro-inflammatory factors like IL-6 can be found (Messner et al. Citation2020).
More examples, a mutation in an interferon-stimulated gene (ISG) might severely interfere in the interferon’s activity (forgive the redundancy). Following this, a deficiency found on the TLR7 of some severe COVID-19 patients cause defects in the production of type I and II interferons that might lead to impaired viral clearance (van der Made et al. Citation2020; Solanich et al. Citation2021). Additionally, preexisting dementia is known to be a risk factor for COVID-19 severity. The apolipoprotein E (ApoE) genotype is a genetic risk factor for dementia, Alzheimer and cardiovascular diseases (especially allele e4). It has been discovered that the ApoE e4e4 allele results on a higher risk of developing severe COVID-19, and ApoE e4 not only affects lipoprotein function related to cardiopathies but also mediates macrophage pro-inflammatory and anti-inflammatory phenotypes. Interestingly, ApoE gene is highly co-expressed in type II alveolar cells in the lungs, as well as ACE2 (Kuo et al. Citation2020). On the other hand, telomere length in peripheral blood lymphocytes has been observed inversely associated with severity in COVID-19, which in turn usually correlates to age (Sanchez-Vazquez et al. Citation2021).
In another dimension, genome-wide association studies (GWAS) show that the risk for respiratory failure in SARS-CoV-2 is increased in people carrying a genetic variant on chromosome three, inherited from Neanderthals, associated with an odds ratio for needing hospitalization of 1.6. This risk locus has an irregular geographical distribution, being carried by about half people in south Asia, one of six people in Europe, absent in east Asia and South America and with low presence in North America (The Severe Covid-19 GWAS Group Citation2020; Zeberg and Pääbo Citation2020). Other GWAS studies have discovered relevant genetic associations with severe COVID-19, such as the protective role of interferon receptor subunit IFNAR1/2 or the association between the TYK2 expression, a gene that codes for a JAK protein and is involved in interferon signaling, and life-threatening illness. Other genes like OAS1, DPP9, CCR2, FOXP4 and SLC6A20 may also contribute to explain some severe cases (Pairo-Castineira et al. Citation2020; COVID-19 Host Genetics Initiative Citation2021). Furthermore, a very relevant factor, the human leukocyte antigen (HLA) system, which codes for the major histocompatibility complex (MHC) proteins that present pathogen antigens to immune B and T cells, is essential for a correct immune response. The HLA system is polymorphic, therefore genetic variability across the three MHC I genes (HLA A, B, and C) that present antigens from a virus inside the cell, may affect susceptibility to and severity of SARS-CoV-2. In silico analysis has been performed to predict the best combination of HLAs for binding SARS-CoV-2 proteins, considering that the highest proportion and the relevance of these proteins for the virus replication have an impact on immune response effectivity (Nguyen et al. Citation2020). Another GWAS study relates the DNA methylation status of several cytosine–guanine (CpG) sites to COVID-19 severity, specifically respiratory failure. These loci are situated inside genes linked to the interferon response pathway, such as the Absent in Melanoma 2 (AIM2) and HLA-C genes. AIM2 detects dsDNA from both damaged host cells or pathogens and triggers the assembly of the inflammasome complex, the caspase-1 activation, a sharp innate immune response with release of cytokines IL-1β and IL-18 and finally pyroptosis. Both hyperactivated HLA-C and AIM2 may imply autoimmune reactions and overproduction of cytokines, with a consequent aggravated immune response (de Moura et al. Citation2021).
Autoimmunity
Autoimmunity (previously introduced with neutralizing autoantibodies against type I interferon) also appears to intervene in the course of the COVID-19 disease. In this respect, prothrombotic antiphospholipid autoantibodies that promote NETs release have been found in serum from COVID-19 hospitalized patients (Zuo et al. Citation2020b). Also, a clinical test has detected probable de novo autoantibodies on severe COVID-19 patients, some of them associated with coagulation abnormalities. If these autoreactive antibodies happened to be long lasting they could lie behind long-haul COVID, which shows a wide and diverse bunch of symptoms (Woodruff et al. Citation2020a; Woodruff et al. Citation2020b; Huang et al. Citation2021). The possibility of SARS-CoV-2 RNA integration into the infected cell genome following reverse-transcription, and consequent expression as chimeric transcripts has been exposed, and might contribute to explain the viral RNA presence in COVID-19 patients weeks after initial detection of infection by PCR (Zhang et al Citation2021a).
Chronic airway diseases: asthma
Apparently, there is a proportionally low number of patients with asthma suffering from an acute respiratory disease like COVID-19. Some studies have suggested that treatment with high-dose inhaled corticosteroids (ICSs) might increase the risk of death among severe asthmatic patients, but low-dose ICS would have no impact on the course of the disease (Schultze et al. Citation2020; Smith et al. Citation2020; Yue et al. Citation2020; Heffler et al. Citation2021; Kow et al. Citation2021). Other studies point that allergic asthma (Th2 phenotype) could even be protective from SARS-CoV-2 infection, and this might be due to a lower expression of ACE2 because of exposure to allergens and the effect of IL-13 (Branco et al. Citation2020; Muñoz et al. Citation2020; Wark et al. Citation2020; Yao et al. Citation2020). The complexity of chronic inflammatory airway diseases like asthma and their relation to ACE2 expression levels, drives the need for further research to be conducted around asthmatic population and their real risk of contracting and suffering from severe COVID-19.
Nicotine
Nicotinic acetylcholine receptors, specifically α7nAchR, constitute an anti-inflammatory pathway and are profusely expressed in immune cells like B cells, T cells and macrophages. Nicotine is an exogenous α7nAchR agonist already used to treat some inflammatory diseases, since it is able to inhibit pro-inflammatory cytokines like TNF-α, IL-1 and IL-6, and is even employed in some neuropsychiatric and neurodegenerative disorders. Additionally, α7nAChRs activation on macrophages might be involved in the stimulation of the vagus nerve, which has been demonstrated to have a positive impact on certain diseases associated with high levels of proinflammatory cytokines such as sepsis. Together with the fact that smokers are proportionally less likely to be hospitalized, a possible therapeutic use of nicotine to inhibit the COVID-19 cytokine storm would be suggested (Gonzalez-Rubio et al. Citation2020; Tizabi et al. Citation2020).
Influence of the microbiota and relevant nutrients
Microbiota and gut-brain axis
The evidence of the microbiome essential role in an organism state of health increases every day mainly due to its relevant impact on the host’s innate and adaptive immune system, so imbalances in microbiota-immunity may contribute to the pathogenesis of many immune-mediated disorders (Zheng et al. Citation2020). In COVID-19 disease, it should be noted that ACE2 receptors are also expressed in the intestinal epithelial cells, that a fraction of patients experience gastrointestinal symptoms usually associated with a poorer clinical outcome, and that SARS-CoV-2 RNA is present in fecal samples of infected patients.
An interesting investigation has applied machine learning to effectively predict progression to severe COVID-19 disease from expression levels of a set of known serum protein and metabolite viral infections biomarkers, including the activated acute phase proteins amyloid A-1 (SAA1), SAA2 and C-reactive protein (a factor of systemic inflammation) (Shen et al. Citation2020). This identified set of biomarkers could also be an indicator of unbalanced host immune system among older adults. In fact, elderly with preexistent cardiovascular, pulmonary, renal, and metabolic disorders present worse COVID-19 prognostic and are also more likely to present gut microbiota dysbiosis. Recent studies suggest that COVID-19 patients exhibit gut microbiota dysbiosis, and therefore microbiota eubiosis should be pursued. Additionally, gut microbial features (operational taxonomic units, OTUs, groups of similar bacteria) can be particularly predictive of the blood proteomic biomarkers of COVID-19 severity among non-infected healthy individuals, since disruption of gut microbiome may induce abnormal inflammation, a key factor in COVID-19 susceptibility and severity. Moreover, a group of microbial OTUs have been found notably correlated with the host inflammatory cytokines IL-1β, IL-2, IL-4, IL-6, IL-8, IL-10, IL-12p70, IL-13, TNF-α and IFN-γ (Gou et al. Citation2021). Multiple studies support the microbiome direct influence on the COVID-19 course (Ferreira et al. Citation2020; Gu et al. Citation2020a; Zuo et al. Citation2020a; Zuo et al. Citation2021).
Furthermore, both gastrointestinal and neurological symptoms related to COVID-19 lead us to the fascinating gut-(lung)-brain axis. The enteric nervous system (ENS), which belongs to the autonomic nervous system (ANS) and is also known as the second brain, regulates the complex enteric performance with independence of the central nervous system (CNS). Despite this neural independence, the gut and the brain constitute a thrilling axis that bidirectionally relates diseases among them through the vagus nerve, the interaction with short-chain fatty acids, immunoregulatory elements and tryptophan (the serotonin neurotransmitter) metabolism, and the secretion of neurotransmitters by certain microbes (Figure ). On the one hand, neurological disorders such as autism, Parkinson or Alzheimer correlate with gastrointestinal abnormalities (Boehme et al. Citation2021); also, psychotropic drugs can mold the microbiome; on the other hand, psychiatric disorders like depression or anxiety are affected by the microbiota, and inflammatory bowel disease changes in stress-mediated intestinal microbiota may alter neural activity in stress-sensitive areas of the brain (Rao and Gershon Citation2016; Bastiaanssen et al. Citation2019; Sinagra et al. Citation2020). Related to this, a retrospective study carried out over a cohort of patients with severe mental disorders and under antipsychotics treatment, suggests a potential role of antipsychotics on preventing both SARS-CoV-2 infection and poor COVID-19 outcomes (Canal-Rivero et al. Citation2021). Specifically, the FDA approved phenylpiperazine aripiprazole has been shown to revert the expression of a bunch of genes related to the immune response and hyperinflammation that are disturbed in COVID-19 patients (Crespo-Facorro et al. Citation2021). To conclude, the gut-brain connection deserves further exploration, possibly targeting the vagus nerve, that transmits information about the microbiota metabolites to the central nervous system and is also probably able to modulate the microbiota composition. Vagus nerve stimulation achieves anti-inflammatory attributes and indirectly modulates the immune activity of the spleen, what could significantly impact the course of the COVID-19 disease (Bonaz et al. Citation2018; Breit et al. Citation2018).
Figure 9. The gut-brain axis bidirectionally relates diseases through the vagus nerve, the interaction with short-chain fatty acids, immunoregulatory elements and tryptophan metabolism, and the secretion of neurotransmitters by certain microbes. (Reprinted from ‘Gut-Brain-Axis', by BioRender, December 2020, retrieved from https://app.biorender.com/biorender-templates/ Copyright 2021 by BioRender.)
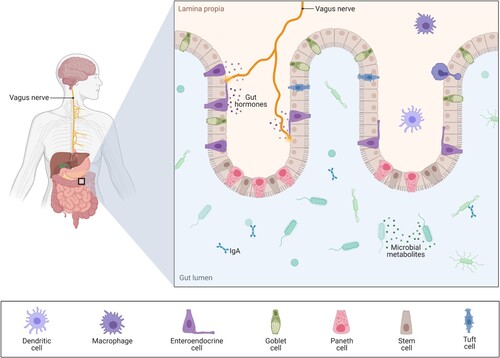
Vitamin D and C
Much has been speculated about the role of vitamin D in protecting from SARS-CoV-2 infection. Vitamin D is a secosteroid hormone that is mainly synthesized in the skin by the action of ultraviolet light radiation (vitamin D3) and can also be obtained in a lesser extent from the diet (vitamin D2 from animals, vitamin D3 from plants). By binding to vitamin D-binding protein (VDBP) in the blood flow, vitamin D reaches the liver and is metabolized into 25(OH) vitamin D or calcidiol, the most common circulating form of vitamin D in serum. 25(OH)D is then metabolized in the kidney to 1α,25-dihydroxyvitamin D, 1α,25(OH)2D or calcitriol, the most biologically active form of vitamin D, that binds the vitamin D receptor (VDR) in the nucleus of target tissues where it takes control of regulation of calcium and phosphate metabolism. Interestingly, vitamin D also impacts the regulation of the immune system: in the innate branch, it induces the secretion of antimicrobial peptides, (calcitriol) shows virus suppressive effects and reduces the release of TNF-α and interferon-γ proinflammatory cytokines. In the adaptive immune branch, calcitriol stimulates T regulatory lymphocytes and suppresses cytokine release from Th1 and Th2 lymphocytes (Jeon and Shin Citation2018; Charoenngam and Holick Citation2020). Importantly, some studies have shown the inverse relation between low serum levels of 25(OH)D and COVID-19 severity, with an association between COVID-19 increasing severity and 25(OH)D level decrease (Merzon et al. Citation2020). Also, 25(OH)D level positively correlates with serum C-reactive protein (CRP) levels and negatively correlates with neutrophil and lymphocyte counts, what points to inflammation. Therefore, serum calcidiol appears as an independent predictor of COVID-19 mortality, and may constitute another factor explaining elderly outcomes since vitamin D deficiency is inversely related to age (Karahan and Katkat Citation2020; Kaufman et al. Citation2020). However, recent studies show that it is actually the active form of vitamin D (calcitriol or 1α,25(OH)2D) which is directly associated with bacteria that produce butyrate and improve gut microbial health, and therefore ratios of vitamin D activation and catabolism may be more accurate biomarkers than serum 25(OH)D (Thomas et al. Citation2020). It seems evident that further research on vitamin D, general vitamin supplementation and even the impact of diet in the COVID-19 course of disease should be conducted.
Vitamin C or ascorbic acid is a pleiotropic stress hormone that also exerts interesting properties to fight COVID-19. As anti-inflammatory, vitamin C is able to interfere the NF-κB triggering and reduce TNFα and IL-1β, to suppress NETosis and also to induce cortisol production; as immunomodulator, it rises the production of type I interferons; as antioxidant, it upregulates antioxidant proteins; and as antiviral, vitamin C might inhibit the Mpro viral main protease. Multiple studies support vitamin C properties, and interestingly it has been shown that vitamin C levels are imperceptible in most COVID-19 patients suffering from acute respiratory distress syndrome (ARDS) (Chiscano-Camón et al. Citation2020; Holford et al. Citation2020).
Zinc and selenium
Zinc is a mineral present in low concentrations in cells that supports essential chemical reactions. It is involved in maintaining the respiratory epithelium barriers that help avoiding pathogens entry into the organism, in boosting the immune system, and it also shows anti-inflammatory and direct antiviral properties (inhibiting viral replication). It seems clear that zinc deficiency might negatively impact the SARS-CoV-2 infection course. A study with hospitalized COVID-19 patients shows that zinc administered together with a zinc ionophore (a fat-soluble transporter that helps zinc cross the cell membrane) correlated with a decrease of 24% in COVID-19 mortality, what evidences the need for further studies on a straightforward oral zinc supplementation against the disease (Frontera et al. Citation2020; Wessels et al. Citation2020; Rahman and Idid Citation2021). Selenium, a trace element that exists in organic and inorganic forms, is known to prevent exacerbated cytokine activation in infectious and inflammatory diseases, lessening the incidence and severity of viral infections including COVID-19. It is hypothesized that redox-active selenium compounds would be able to inhibit some SARS-CoV-2 proteases (Zhang et al. Citation2020a). Other nutrients may have positive influence on the course of COVID-19, such as folic acid, a water-soluble B vitamin that could inhibit the activity of the furin protease (Sheybani et al. Citation2020).
Omega-3 fatty acids
Omega-3 are unsaturated fatty acids mainly present in fish oils, with very-long-chain Eicosapentaenoic acid (EPA) and docosahexaenoic acid (DHA) being and of especial relevance. Both EPA and DHA exert a bunch of interesting anti-inflammatory properties that have shown benefit on animal models of rheumatoid arthritis, inflammatory bowel disease and asthma (Calder Citation2015). A recent study on a small cohort of COVID-19 patients has established a strong inverse relationship between Omega-3 tissue levels measured by the Omega-3 Index (O3I) and decease, finding an odds ratio for death in patients with higher O3I levels (first quartile) of 0.25 compared to O3I less than 5.7% (Asher et al. Citation2021).
Influence of environmental factors on SARS-CoV-2 spread
Many studies have been carried out to assess the impact of environmental factors in the pandemic spread, nonetheless not always conclusive. Some environmental factors are known to decrease the SARS-CoV-2 transmission, such as high temperature, humidity and solar radiation (able to inactivate the virus by degrading its lipid envelop). However, the impact of human patterns, for instance, high population density particularly indoors, and mobility, overshadows the natural factors’ effects. Besides, both human and natural elements are interrelated, with temperature (and, in general, seasonality) influencing human behavior, which hinders the identification of each parameter’s independent effect. On the other hand, though SARS-CoV-2 survival on inert surfaces may last several days, especially under low-temperature conditions, this means of contagion is not considered relevant (Damette et al. Citation2021; Smith et al. Citation2021). Air pollution positively affects COVID-19 severity and lethality mainly indirectly, by affecting previous chronic pathologies with impact on the disease course, or by impairing vitamin D synthesis (Bourdrel et al. Citation2021). Also, high airborne pollen concentrations have shown a positive impact on the increase of infection rates (Damialis et al.. Citation2021).
Since COVID-19 patients exhibit the presence of SARS-CoV-2 RNA in their feces, analysis of sewage water is a simple and non-expensive way to search for fragments of the virus genetic material and observe the incidence of the infection by population areas. This presence of the virus correlates with subsequent PCR results and even accounts for asymptomatic cases; it may also point at the reappearance of the infection in a sector with few detected cases. Data collected from wastewater treatment plants allows for monitoring infection trends at a town level, whilst sewer pipes data may be used for identifying hot spots or for observing potential sites of infection spread (e.g. schools or work places), assessing health public actions like the closure of spaces, testing or vaccination. Interestingly, sewage water analysis has allowed to discover SARS-CoV-2 presence months before the official declaration of the pandemic. Many countries have implemented wastewater surveillance programs, and global collaborative initiatives exist to support wastewater-based epidemiology of SARS-CoV-2 (Fuschi et al.. Citation2021; Prado et al.. Citation2021).
Conclusions
Many clinical biomarkers are related to poor clinical outcomes, and predictive scores have been set to help in COVID-19 prognosis. Females are underrepresented in severe COVID-19 cases maybe due to genes on the X chromosome and feminine sexual hormones. The disease severity in children is milder though their susceptibility to infection seems similar to that in adults, and they are not preserved from complications like multisystem inflammatory syndrome (MIS-C) or long covid. Genetic studies have unveiled some genetic variants associated with increased susceptibility and /or severity of COVID-19, with the HLA system standing out due to its inherent high variability.
It is remarkable the potentially relevant influence of the microbiota on the course of COVID-19, with associations between microbiota dysbiosis and microbial features with unfavorable outcomes. Some main nutrients like vitamins, minerals and fatty acids may also affect the progress of the disease.
Finally, though many environmental factors may influence the spread of the disease, human behavior linked to aerosol transmission is revealed as the main driving agent. Wastewater-based epidemiology constitutes a valuable help in monitoring infection trends.
SARS-CoV-2 mutations and variants
Almost from the beginning of the official COVID-19 outbreak in January 2020, the SARS-CoV-2 genome is continuously being sequenced and published in repositories like the GISAID initiative, that as of August 2021 holds the official hCoV-19 reference sequence along with almost three million genetic sequences in exponential growth (GISAID Citation2021). This allows for tracking the pandemic spread and for monitoring mutations that may impact the transmissibility, pathogenicity or even the effectivity of vaccines or other treatments like therapeutic antibodies targeting the antigens’ epitopes. The (evolutionary) mutation rate of SARS-CoV-2 is said to be relatively low, showing one mutation per two or three weeks (Rausch et al.. Citation2020), which is not incompatible with the thousands of detected mutations that can be consulted at GISAID. For example, the long-time dominant D614G mutation (replacement of aspartic acid D amino acid by glycine G) of the spike protein is associated with an increase in viral loads in the upper respiratory tract and a younger patient profile, and it may also suggest a selective advantage and increase in virus transmission, though in principle this should not affect the effectivity of the designed vaccines targeting the spike (Korber et al.. Citation2020; Plante et al.. Citation2020; Wesley Long et al. Citation2020; Volz et al. Citation2021). Mutations can affect not only the spike but also other proteins such as the immunogenic nucleocapsid (N) (Tomaszewski et al. Citation2020) or the open reading frames (ORFs). Variant Δ382 presents a 382-nucleotide deletion in the strongly immunogenic ORF8, that might be involved in the virus immune evasion, so Δ382 would presumably cause a less severe infection (Young et al. Citation2020; Zhang et al. Citation2020d). Other variant called 20A.EU1 found in summer 2020 probably in Spain was spread across Europe as a consequence of the summer mobility (Hodcroft et al. Citation2020).
Variants comprising many mutations may have been originated in immunosuppressed COVID-19 patients where virus replication is increased (Kemp et al. Citation2021), or even as a consequence of reverse-zoonosis that create new animal reservoirs and can revert back to humans (Aydillo et al. Citation2020; Bazykin et al. Citation2021). The United Kingdom has communicated in late December 2020 the new SARS-CoV-2 B.1.1.7 lineage, also known as 20I/501Y.V1 Variant of Concern (VOC) 202012/01, with surprising multiple mutations affecting the spike protein (deletion 69-70, deletion 144, N501Y, A570D, D614G, P681H, T716I, S982A, D1118H), ORF1ab, ORF8 and the nucleocapsid protein (European Centre for Disease Prevention and Control Citation2020), with an overall figure of 14 non-synonymous mutations and 3 deletions. Some of the mutations affecting the spike are of special interest since they could exert biological effects: mutation N501Y (501 is one of six key contact residues within the receptor-binding domain (RBD)) might increase binding affinity to ACE2 (Santos and Passos Citation2021); 69-70del might be related to evasion of the immune system; and mutation P681H is adjoining to the furin cleavage site between subunits S1 and S2 (that promotes host cells viral entry) (Rambaut et al. Citation2021). Preliminary studies show higher transmissibility than other lineages and a notable increased affectation among the young population, which could be caused by a longer duration of the acute infection (Volz et al. Citation2020; Kissler et al. Citation2021). An increased risk of death has been detected in this variant as compared to preceding variants, estimated at 64% (Challen et al. Citation2021). However, subsequent studies contradict this and do not detect greater severity in hospitalized patients nor even an increment in the reinfection rate (Frampton et al. Citation2021; Graham et al. Citation2021).
Also, B.1.351 lineage, a.k.a. 20H/501Y.V2, detected in South Africa shows several mutations affecting the spike protein, among them K417N, E484 K and N501Y (this one in common with the B.1.1.7 lineage) located in the RBD. Regarding binding affinity, both E484K and K417N mutations seem to exert a minimum effect. However, K417N and N501Y mutations, and especially E484K, could escape neutralizing antibodies binding, since the RBD is immunodominant (provokes the majority of the generated antibodies) and also the target of 90% of neutralizing antibodies (Piccoli et al. Citation2020; Tegally et al. Citation2020). Studies show that possible RBD escape mutations coincide in or near the contact surface between antibodies and the RBD, particularly in the epitope positioned around the 443–450 loop, precisely targeted by the Regeneron antibody cocktail REGEN-COV2 (Greaney et al. Citation2021a, Citation2021b). In fact, many monoclonal antibodies against both the RBD and the N-terminal domain (NTD) region within the spike do fail to neutralize variants carrying the E484K mutation (Wang et al. Citation2021a). B.1.351 lineage offers a substantially increased resistance to neutralization by wild convalescent plasma and from the Pfizer/BioNTech BNT162b2 and the Moderna mRNA-1273 vaccines (Wang et al. Citation2021a; Liu et al. Citation2021a). No altered neutralizing activity of antibodies generated by the Pfizer/BioNTech BNT162b2 vaccine against N501Y mutation has been found (Xie et al. Citation2021). Observational results from Israel, the first country to immunize its population with the Pfizer/BioNTech BNT162b2 vaccine are aligned with the clinical trial outcomes. The two-dose schedule is extremely effective in impeding symptomatic and asymptomatic SARS-CoV-2 infections in adults older than 16, including hospitalizations and death, even for the B.1.1.7 variant. COVID-19 incidence descent ignores the reopening of society and directly associates with higher vaccination coverage (Dagan et al. Citation2021; Haas et al. Citation2021). Some embolic and thrombotic events affecting AstraZeneca ChAdOx1-S and COVID-19 Vaccine Janssen recipients have been reported. In the so-called vaccine induced prothrombotic immune thrombocytopenia (VIPIT) disorder, the vaccine apparently induces platelet-activating (auto)antibodies against platelet factor 4 (PF4), a cytokine released by activated platelets when aggregating, that may lead to serious thrombocytopenia and thrombotic complications at rare sites like the brain or abdomen (Greinacher et al. Citation2021). In a complementary mechanism, the DNA encoding for the spike protein in these adenovirus vaccines could suffer from undesired splicing events in the transcription process carried out in the cell nucleus, leading to shorter, soluble variants of the spike protein. These mutants, unable to be anchored to the cell membrane, may bind ACE2 expressing endothelial cells in blood vessels, and be also targeted by newly created antibodies, leading to strong inflammation and even NETs formation. The solution would imply the redesign of the spike open reading frames in DNA-based vaccines to prevent unwanted splice incidents (Kowarz et al. Citation2021). A large study comprising over 280,000 ChAdOx1-S vaccinees aged 18–65 years in Norway and Denmark confirmed a higher rate of venous thromboembolic events, especially of cerebral venous thrombosis emerging in one in 40,000 individuals (Pottegård et al. Citation2021). Though arterial events were not significant in that study, several leading to ischaemic stroke have been reported in young people (Al-Mayhani et al. Citation2021).
P.1 lineage (a.k.a. 20J/501Y.V3) noticed in Brazil exhibit a bunch of amino acid changes and deletions, including K417T, E484K, and N501Y. A reinfection case has been reported carrying this variant (Resende et al. Citation2021).
Additionally, N439K mutation in the receptor-binding motif (RBM) of the spike appears in several variants, and has shown increased binding affinity to ACE2 and also evasion of several monoclonal antibodies neutralization and of polyclonal sera responses, while maintaining disease severity unaltered (Thomson et al. Citation2021). Maps of mutations in the RBD of SARS-CoV-2 that evade antibody binding are being uncovered, with the aim of predicting potential viral escape from human sera or monoclonal antibodies in emerging variants (Greaney et al. Citation2021b; Greaney et al. Citation2021a; Hie et al. Citation2021; Starr et al. Citation2021).
B.1.617 lineage, along with its three subtypes B.1.617.1, B.1.617.2 (especially this one) and B.1.617. 3 was initially identified in India. It shows an increase in transmissibility of around 50%, and has quickly displaced B.1.1.7 variant in the United Kingdom. It carries mutations like L452R, T478K (B.1.617.2) and E484Q (B.1.617.1), among others, that are related to vaccine escape (Adam Citation2021). In a study comprising over 200 B.1.617 infected individuals, including 71 mRNA fully vaccinated and 130 non-vaccinated, it was found that mRNA vaccines were highly protective against symptomatic infection and severe disease caused by this (a.k.a. Delta) variant. Although initial viral loads were alike (measured in PCR Ct (cycle threshold) values) in both vaccinated and non-vaccinated patients, those with mRNA vaccine experienced a faster viral load drop, which would relate to memory immunity against COVID-19, and would also imply a shortening in secondary transmission (Chia et al. Citation2021).
C.37 lineage, first detected in Perú, adds to the in crescendo bunch of variants with mutations G75V, F490S, D614G, T859N, and especially T76I and L452Q, both associated to higher infectivity, and the 7-amino-acid deletion mutation in the NTD of the spike RSYLTPGD246-253N, that along with L452Q (curiously with a different amino acid change than L452R in B.1.617) and F490S are associated to evasion from neutralizing antibodies. On the other hand, mutations in the NTD are associated with escape neutralization; and antibodies responsible for antibody-dependent enhancement (ADE), detected in severe COVID-19 patients, target the NTD. Intriguingly, T76I and L452Q mutations could contribute to an enhanced viral infectivity or ADE (Kimura et al. Citation2021).
Centers for Disease Control and Prevention like the CDC from the U.S.A. and the European eCDC offer updated information related to SARS-CoV-2 variants of interest, variants of concern and variants of high consequence (SARS-CoV-2 Variant Classifications and Definitions Citation2021; SARS-CoV-2 variants dashboard Citation2021).
Back to immunity, and importantly CD4+ and CD8+ T cell responses in both COVID-19 patients and mRNA vaccinated individuals are likely not significantly affected by mutations present in the aforementioned variants B.1.1.7, B.1.351, P.1, and also in lineage B.1.429 (a.k.a. CAL.20C), nor are the sequences of most SARS-CoV-2 T cell epitopes (Tarke et al. Citation2021a; Tarke et al. Citation2021b). This could be related to the polyclonal character of the T cell response, with T cell epitopes distributed along numerous regions of the spike. Also, for SARS-CoV-2 mRNA-based vaccines, S-binding germinal centers B cells and plasmablasts are found in draining lymph nodes four months after the complete vaccination series, suggesting induction of long-lived plasma cell responses and therefore long-lasting robust humoral immunity (Turner et al. Citation2021b).
New generation vaccines are to be developed in order to face novel arising variants, that usually share similarities since they are the natural consequence of the virus evolution and adaptation to the human host and the environment (e.g. antibodies presence).
Finally, genome analyses together with proteomics also shed light on the implications of the virus, for instance, the non-structural proteins nsp9 and nsp10 seem to behave like virulence factors that interact with the NF-kB transcriptional repressor factor NKRF, which may contribute to the induction of IL-8 and IL-6 in lung epithelial cells and the consequent inflammatory overreaction (Li et al. Citation2021b). However, the SARS-CoV-2 genome remains incompletely uncovered since for example overlapping genes, able to code for proteins and /or exert other functions in the virus lifecycle, such as ORF3d are still appearing (Nelson et al. Citation2020).
Some innovative treatments against COVID-19
Cell-based therapies
Cell-based therapies employ living cells with biological effect. Among them, Mesenchymal Stem Cells (MSCs), natural killer (NK) cells, dendritic cells and SARS-CoV2-specific T cells are being evaluated as potential COVID-19 treatments (Golchin Citation2020). For instance, a study has carried out an allogeneic adipose-tissue derived MSC (AT-MSC) treatment, that was intravenously administered to a small group of 13 COVID-19 patients under invasive mechanical ventilation. As a result, most patients showed clinical improvement with a decrease in inflammatory parameters (CRP, IL-6, ferritin, LDH and d-dimer) as well as an increase in lymphocytes (Sánchez-Guijo et al. Citation2020). A similar strategy is being developed by a Bonus BioGroup drug based on activated mesenchymal cells, leading to the fast cure of pneumonia symptoms by reducing the immune cells burden in the injured pulmonary alveoli (Safety and the Efficacy of MesenCure for the Treatment of Pulmonary Manifestations of COVID-19 Citation2021). Also, SARS-CoV-2-specific memory T cells extracted from recovered patients might be infused into severe COVID-19 patients, enabling them to create their own immunological memory. A study has demonstrated the easiness of isolating SARS-CoV-2-specific T cells from selected donors conditioned to the expression of IFN-γ and HLA (Ferreras et al. Citation2021). In this regard, a clinical trial to infuse NK cells and memory T cells to severe COVID-19 patients suffering from pneumonia and/or lymphopenia is ongoing (Safety Infusion of NatuRal KillEr celLs or MEmory T Cells as Adoptive Therapy in COVID-19 pnEumonia or Lymphopenia (RELEASE) Citation2020).
CRISPR
Clustered Regularly Interspaced Short Palindromic Repeats or CRISPR, and more specifically, CRISPR/Cas9, is an eminent genome editing tool composed of a Cas9 endonuclease that cuts the DNA at a user-defined specific sequence, determined by a bound short single-stranded guide RNA (gRNA), in order to add or remove DNA target nucleotide pairs. The cell itself is able to repair its damaged DNA yielding a loss-of-function mutation on the target gene. This DNA modification usually presents non-desired side effects, however CRISPR-mediated gene activation or repression without modifying the DNA can also be performed (Dance Citation2015; McDade Citation2020).
New CRISPR techniques are also able to target RNA instead of DNA with novel endonucleases like Cas13, even exerting higher-level specificity than other knockdown techniques such as RNA interference (RNAi). A CRISPR system using a Cas13d protein and CRISPR-associated RNAs (crRNAs) that guide the Cas13d to specific RNA molecules has already been designed, to effectively target and degrade the positive-sense viral genome used as template RNA and also its viral gene expression (mRNA), successfully restricting viral replication (with pan-coronavirus protection) (Abbott et al. Citation2020). This astounding technology can also be used to perform genome-wide CRISPR loss-of-function screening to uncover host genes essential for SARS-CoV-2 to successfully infect the cells. Along with known host factors such as ACE2 receptor and the protease Cathepsin L, genes like HMGB1 and SIGMAR, and many others involved in the virus replication process, including those related to endosome regulation, have been identified (Daniloski et al. Citation2021; Wei et al. Citation2021).
Also worth mentioning, CRISPR can be included as a sequence detector in tests for detecting SARS-CoV-2, in two-steps methods like SHERLOCK (Specific High-sensitivity Enzymatic Reporter unLOCKing), following viral RNA extraction and amplification, showing results quicker than PCR (Joung et al. Citation2020).
Conclusions
The COVID-19 occurrence has affected health and economy in a worldwide scale with severe consequences on daily life of every human being. This novel disease dares our medical and biological knowledge due to its intrinsic complexity and its fast and easy spread across the globe. The wide bunch of symptoms including respiratory, digestive, neurological, cardiovascular, dermatological, inflammatory and even immunologic dysfunctions; or heterogeneous immune responses, severity profiles – including asymptomatic and long-haulers or persistent covid – and risk factors, hinder the patient prognosis and the development of an effective and personalized treatment. In fact, any (especially, non-vaccinated) person has a potential risk of developing severe COVID-19.
The resolution of the pandemic involves many factors, from simple prophylactic measures like perfectly efficient face masks to mass vaccination, going through treatments including repurposed and newly designed drugs like antivirals that block the virus replication cycle (e.g. nucleoside analogues), monoclonal antibodies and nanobodies (e.g. neutralizing antibodies targeting the receptor-binding domain RBD of the spike; or IL-6 antagonists), immune modulators (e.g. convalescent hyperimmune plasma and derivates; or interferons), anti-inflammatories (e.g. dexamethasone), anticoagulants and other small molecules. Also, massive, quick, accurate and affordable tests must be frequently performed, since one of the main reasons for the success of the COVID-19 widespread has been the undetected asymptomatic but infectious individuals. Distinct biomarkers to make a precise prognosis in order to adjust treatment should be uncovered. In addition, research on alternative effective ways of neutralizing the SARS-CoV-2 capacity of infection also contributes, for instance destroying the virus lipidic membrane in the air or inside our mouth and nasal cavities. High-Efficiency Particulate Arresting HEPA mechanical filters, ozone generators, ultra-violet UV germicidal irradiation or hydrogen peroxide-based air purifiers are currently available.
Research has undoubtedly been the key. Research, and the associated funding, have made possible the historic scientific milestone of having a notable number of highly effective vaccines against COVID-19 in record time of only several months. Technology and especially artificial intelligence have provided invaluable assistance in accelerating processes and analyzing huge amounts of data, helping with the discovery of new drugs or repurposing existing ones, with the prediction of the COVID-19 phases or the spread of the infection. However, the core work remains within the biological and medical scopes, inside the labs (research, genomic virus surveillance, genetic analyses …) and with the clinical trials, and so scientific effort and investment must keep on them their main focus. Besides, social and administrative issues have markedly impacted the development of the COVID-19 crisis. General deficiencies within the sanitary, governance, or logistic structures, not prepared for facing such a global health threat and such a disruption in the ordinary course of life, have been uncovered all over the world. The ability to implement fast and effective measures, offering accurate information to the general public, taking care and attention of elder people but also children show room for improvement. Besides, more individual responsibilities like maintaining high air quality indoors, a correct state of nutrition or physical fitness should now be of general awareness.
Many challenges and hazards are still watching. Maybe the most relevant is the appearance of SARS-CoV-2 mutations that might evade or diminish the immune response from vaccinated, or the efficacy of therapies targeting parts of the virus like monoclonal antibodies and antivirals. Or the emergence of variants that increase transmissibility, susceptibility and lethality. Or new strains. Or virus recombinations with other strains or other viruses (Gribble et al. Citation2021). Even different viruses reactivation in immunodepressed patients. Also, reverse zoonosis is a significant threat. Hence the urgency of a global, fast, flexible and massive vaccination program complemented with effective treatments (easily administered in early stages of the disease, or else for severely ill), along with a permanent tracking of vaccination immunity response, duration and side effects, potential reinfections and coinfections (Sabino et al. Citation2021), population immunity and virus genome sequencing. We have unfailingly just started a new era where research and monitoring must be uninterrupted not only for ensuring the ending of the COVID-19 pandemic but also to better face foreseeable future pandemics.
Disclosure statement
No potential conflict of interest was reported by the author(s).
Data availability statement
Data sharing is not applicable to this article as no new data were created or analyzed in this study.
References
- Abbott TR, Dhamdhere G, Liu Y, Lin X, Goudy L, Zeng L, Chemparathy A, Chmura S, Heaton NS, Debs R, et al. 2020. Development of CRISPR as an antiviral strategy to combat SARS-CoV-2 and influenza. Cell. 181(4):865–876.e12. https://doi.org/10.1016/j.cell.2020.04.020.
- Abers MS, Delmonte OM, Ricotta EE, Fintzi J, Fink DL, Almeida de Jesus AA, Zarember KA, Alehashemi S, Oikonomou V, Desai JV, et al. 2021. An immune-based biomarker signature is associated with mortality in COVID-19 patients. JCI Insight. 6(1):e144455. https://doi.org/10.1172/jci.insight.144455.
- Acharya D, Liu G, Gack MU. 2020. Dysregulation of type I interferon responses in COVID-19. Nat Rev Immunol. 20:397–398. https://doi.org/10.1038/s41577-020-0346-x.
- Adam D. 2021. What scientists know about new, fast-spreading coronavirus variants. Nature. https://doi.org/10.1038/d41586-021-01390-4.
- Agrawal B. 2019. Heterologous immunity: role in natural and vaccine-induced resistance to infections. Front Immunol. 10:2631. https://doi.org/10.3389/fimmu.2019.02631.
- Ahmed M, Advani S, Moreira A, Zoretic S, Martinez J, Chorath K, Acosta S, Naqvi R, Burmeister-Morton F, Burmeister F, et al. 2020. Multisystem inflammatory syndrome in children: a systematic review. Clin Med. 26:100527. https://doi.org/10.1016/j.eclinm.2020.100527.
- Al-Aly Z, Xie Y, Bowe B. 2021. High-dimensional characterization of post-acute sequalae of COVID-19. Nature. https://doi.org/10.1038/s41586-021-03553-9.
- Alberts B. 2020. Molecular biology of the cell. 6th ed. Garland Science: Taylor & Francis Group.
- Alberts B, Johnson A, Lewis J, Raff M, Roberts K, Walter P. 2015. Molecular biology of the cell. 6th ed. New York: Garland Science.
- Alemany A, Baró B, Ouchi D, Rodó P, Ubals M, Corbacho-Monné M, Vergara-Alert J, Rodon J, Segalés J, Esteban C, et al. 2021. Analytical and clinical performance of the panbio COVID-19 antigen-detecting rapid diagnostic test. J Infect. https://doi.org/10.1016/j.jinf.2020.12.033.
- Al-Mayhani T, Saber S, Stubbs MJ, Losseff NA, Perry RJ, Simister RJ, Gull D, Jäger HR, Scully MA, Werring DJ. 2021. Ischaemic stroke as a presenting feature of ChAdOx1 nCoV-19 vaccine-induced immune thrombotic thrombocytopaenia. Neurosurg Psychiatry. https://doi.org/10.1136/jnnp-2021-326984.
- Antony AR, Haneef Z. 2020. Systematic review of EEG findings in 617 patients diagnosed with COVID-19. Seizure. 83:234–241 https://doi.org/10.1016/j.seizure.2020.10.014.
- Arts RJW, Carvalho A, La Rocca C, Palma C, Rodrigues F, Silvestre R, Kleinnijenhuis J, Lachmandas E, Gonçalves LG, Belinha A, et al. 2016. Immunometabolic pathways in BCG-induced trained immunity. Cell Rep. 17. https://doi.org/10.1016/j.celrep.2016.11.011.
- Arvin AM, Fink K, Schmid MA, Cathcart A, Spreafico R, Havenar-Daughton C, Lanzavecchia A, Corti D, Virgin HW. 2020. A perspective on potential antibody-dependent enhancement of SARS-CoV-2. Nature. 584:353–363. https://doi.org/10.1038/s41586-020-2538-8.
- Asher A, Tintle NL, Myers M, Lockshon L, Bacareza H, Harris WS. 2021. Blood omega-3 fatty acids and death from COVID-19: a pilot study. Prostaglandins, Leukotrienes Essent Fatty Acids. 166:102250. https://doi.org/10.1016/j.plefa.2021.102250.
- Askitas N, Tatsiramos K, Verheyden B. 2021. Estimating worldwide effects of non-pharmaceutical interventions on COVID-19 incidence and population mobility patterns using a multiple-event study. Sci Rep. 11:1972. https://doi.org/10.1038/s41598-021-81442-x.
- Avci AB, Feist E, Burmester GR. 2018. Targeting IL-6 or IL-6 receptor in rheumatoid arthritis: What’s the difference? BioDrugs. 32:531–546. https://doi.org/10.1007/s40259-018-0320-3.
- Avendano-Sola C, Ramos-Martinez A, Munez-Rubio E, Ruiz-Antorán B, de Molina RM, Torres F, Fernández-Cruz A, Callejas-Díaz A, Calderón J, Payares-Herrera C, et al. 2020. Convalescent plasma for COVID-19: a multicenter, randomized clinical trial. medRxiv. https://doi.org/10.1101/2020.08.26.20182444.
- Ayanian S, Reyes J, Lynn L, Teufel K. 2020. The association between biomarkers and clinical outcomes in novel coronavirus pneumonia in a US cohort. Biomark Med. 14(12): 1091–1097. https://doi.org/10.2217/bmm-2020-0309.
- Aydillo T, Gonzalez-Reiche AS, Aslam S, van de Guchte A, Khan Z, Obla A, Dutta J, van Bakel H, Aberg J, García-Sastre A, et al. 2020. Shedding of viable SARS-CoV-2 after immunosuppressive therapy for cancer. N Engl J Med. 383:2586–2588. https://doi.org/10.1056/NEJMc2031670.
- Badedi M, Makrami A, Alnami A. 2021. Co-morbidity and blood group type risk in coronavirus disease 2019 patients: a case–control study. J Infect Public Health. 14(4):550–554. https://doi.org/10.1016/j.jiph.2020.12.035.
- Baden LR, El Sahly HM, Essink B, Kotloff K, Frey S, Novak R, Diemert D, Spector SA, Rouphael N, Creech CB, et al. 2020. Efficacy and Safety of the mRNA-1273 SARS-CoV-2 vaccine. N Engl J Med. https://doi.org/10.1056/NEJMoa2035389.
- Bairi KE, Trapani D, Petrillo A, Le Page C, Zbakh H, Daniele B, Belbaraka R, Curigliano G, Afqir S. 2020. Repurposing anticancer drugs for the management of COVID-19. Eur J Cancer. 141:40–61. https://doi.org/10.1016/j.ejca.2020.09.014.
- Baldassarre A, Paolini A, Bruno SP, Felli C, Tozzi AE, Masotti A. 2020. Potential use of noncoding RNAs and innovative therapeutic strategies to target the 5’UTR of SARS-CoV-2. Epigenomics. 12(15):1349–1361. https://doi.org/10.2217/epi-2020-0162.
- Baldassarri M, Picchiotti N, Fava F, Fallerini C, Benetti E, Daga S, Valentino F, Doddato G, Furini S, Giliberti A, et al. 2021. Shorter androgen receptor polyQ alleles protect against life-threatening COVID-19 disease in European males. EBioMedicine. 65:103246. https://doi.org/10.1016/j.ebiom.2021.103246.
- Barnes BJ, Adrover JM, Baxter-Stoltzfus A, Borczuk A, Cools-Lartigue J, Crawford JM, Daßler-Plenker J, Guerci P, Huynh C, Knight JS, et al. 2020, June 1. Targeting potential drivers of COVID-19: neutrophil extracellular traps. J Exp Med. 217(6):e20200652. https://doi.org/10.1084/jem.20200652.
- Barnes PJ. 2006. How corticosteroids control inflammation: quintiles prize lecture 2005. Br J Pharmacol. 148(3):245–254. https://doi.org/10.1038/sj.bjp.0706736.
- Barnkob MB, Pottegård A, Støvring H, Haunstrup TM, Homburg K, Larsen R, Hansen MB, Titlestad K, Aagaard B, Møller BK, et al. 2020. Reduced prevalence of SARS-CoV-2 infection in ABO blood group O. Blood Adv. 4(20):4990–4993. https://doi.org/10.1182/bloodadvances.2020002657.
- Bastard P, Rosen LB, Zhang Q, Michailidis E, Hoffmann HH, Zhang Y, Dorgham K, Philippot Q, Rosain J, Béziat V, et al. 2020. Autoantibodies against type I IFNs in patients with life-threatening COVID-19. Science. 370(6515):eabd4585. https://doi.org/10.1126/science.abd4585.
- Bastiaanssen TFS, Cowan CSM, Claesson MJ, Dinan TG, Cryan JF. 2019. Making sense of … the microbiome in psychiatry. Int J Neuropsuchopharmacolog. 22(1):37–52. https://doi.org/10.1093/ijnp/pyy067.
- Bazykin GA, Stanevich O, Danilenko D, Fadeev A, Komissarova K, Ivanova A, Sergeeva M, Safina K, Nabieva E, Klink G, et al. Emergence of Y453F and Δ69-70HV mutations in a lymphoma patient with long-term COVID-19. Virological. [accessed 2021 Aug 17]. https://virological.org/t/emergence-of-y453f-and-69-70hv-mutations-in-a-lymphoma-patient-with-long-term-covid-19/580.
- Bellmunt S, Riera C, Gil D, Rodríguez M, García-Reyes M, Martínez-Carnovale L, Marrero C, Gil M, Ruiz-Rodríguez JC, Ferrer R, et al. 2020. COVID-19 infection in critically Ill patients carries a high risk for venous thromboembolism. Eur J Vasc Endovasc Surg. https://doi.org/10.1016/j.ejvs.2020.12.015.
- Berenguer J, Borobia AM, Ryan P, Rodríguez-Baño J, Bellón JM, Jarrín I, Carratalà J, Pachón J, Carcas AJ, Yllescas M, et al. 2021. Development and validation of a prediction model for 30-day mortality in hospitalised patients with COVID-19: the COVID-19 SEIMC score. Thorax. https://doi.org/10.1136/thoraxjnl-2020-216001.
- Blaising J, Polyak SJ, Pécheur E-I. 2014. Arbidol as a broad-spectrum antiviral: an update. Antiviral Res. 107:84–94. https://doi.org/10.1016/j.antiviral.2014.04.006.
- Blanco JIM, Alvarenga Bonilla JA, Homma S, Suzuki K, Fremont-Smith P, de las Heras KVG. 2021. Antihistamines and azithromycin as a treatment for COVID-19 on primary health care – a retrospective observational study in elderly patients. Pulm Pharmacol Ther. 67:101989. https://doi.org/10.1016/j.pupt.2021.101989.
- Blanco-Melo D, Nilsson-Payant BE, Uhl S, Hoagland D, Møller R, Jordan TX, Oishi K, Panis M, Sachs D, Wang TT, et al. 2020. Imbalanced host response to SARS-CoV-2 drives development of COVID-19. Cell. 181:1036–1045.e9. https://doi.org/10.1016/j.cell.2020.04.026.
- Blume C, Jackson CL, Spalluto CM, Legebeke J, Nazlamova L, Conforti F, Perotin JM, Frank M, Butler J, Crispin M, et al. 2021. A novel ACE2 isoform is expressed in human respiratory epithelia and is upregulated in response to interferons and RNA respiratory virus infection. Nat Genet. https://doi.org/10.1038/s41588-020-00759-x.
- Boehme M, Guzzetta KE, Bastiaanssen TFS, van de Wouw M, Moloney GM, Gual-Grau A, Spichak S, Olavarría-Ramírez L, Fitzgerald P, Morillas E, et al. 2021. Microbiota from young mice counteracts selective age-associated behavioral deficits. Nat Aging. 1:666–676. https://doi.org/10.1038/s43587-021-00093-9.
- Bonaz B, Bazin T, Pellissier S. 2018. The vagus nerve at the interface of the microbiota-gut-brain axis. Front Neurosci. 12:49. https://doi.org/10.3389/fnins.2018.00049.
- Bourdrel T, Annesi-Maesano I, Alahmad B, Bind M-A, Maesano CN, Bind MA. 2021. The impact of outdoor air pollution on COVID-19: a review of evidence from in vitro, animal, and human studies. Eur Respir Rev. 30:200242. https://doi.org/10.1183/16000617.0242-2020.
- Branco ACCC, Sato MN, Alberca RW. 2020. The possible dual role of the ACE2 receptor in asthma and coronavirus (SARS-CoV2) infection. Front Cell Infect Microbiol. 10:550571. https://doi.org/10.3389/fcimb.2020.550571.
- Brauner JM, Mindermann S, Sharma M, Johnston D, Salvatier J, Gavenčiak T, Stephenson AB, Leech G, Altman G, Mikulik V, et al. 2021. Inferring the effectiveness of government interventions against COVID-19. Science. https://doi.org/10.1126/science.abd9338.
- Breit S, Kupferberg A, Rogler G, Hasler G. 2018. Vagus nerve as modulator of the brain–Gut axis in psychiatric and inflammatory disorders. Front Psychiatry. 9:44. https://doi.org/10.3389/fpsyt.2018.00044.
- Broad Institute. Drug repurposing hub. [accessed 2021 Aug 17]. https://clue.io/repurposing.
- Buckland MS, Galloway JB, Fhogartaigh CN, Meredith L, Provine NM, Bloor S, Ogbe A, Zelek WM, Smielewska A, Yakovleva A, et al. 2020. Treatment of COVID-19 with remdesivir in the absence of humoral immunity: a case report. Nat Commun. 11:6385. https://doi.org/10.1038/s41467-020-19761-2.
- Buzhdygan TP, DeOre BJ, Baldwin-Leclair A, Bullock TA, McGary HM, Khan JA, Razmpour R, Hale JF, Galie PA, Potula R, et al. 2020. The SARS-CoV-2 spike protein alters barrier function in 2D static and 3D microfluidic in-vitro models of the human blood–brain barrier. Neurobiol Dis. 146:105131. https://doi.org/10.1016/j.nbd.2020.105131.
- Calder Philip C. 2015. Marine omega-3 fatty acids and inflammatory processes: Effects, mechanisms and clinical relevance. Biochimica et Biophysica Acta (BBA) - Molecular and Cell Biology of Lipids. 1851(4):469–484. http://dx.doi.org/10.1016/j.bbalip.2014.08.010.
- Caly L, Druce JD, Catton MG, Jans DA, Wagstaff KM. 2020. The FDA-approved drug ivermectin inhibits the replication of SARS-CoV-2 in vitro. Antiviral Res. 178:104787. https://doi.org/10.1016/j.antiviral.2020.104787.
- Campisi J, d'Adda di Fagagna F. 2007. Cellular senescence: when bad things happen to good cells. Nat Rev Mol Cell Biol. 8:729–740. https://doi.org/10.1038/nrm2233.
- Canal-Rivero M, Catalán-Barragán R, Rubio-García A, Garrido-Torres N, Crespo-Facorro B, Ruiz-Veguilla M. 2021. Lower risk of SARS-CoV2 infection in individuals with severe mental disorders on antipsychotic treatment: a retrospective epidemiological study in a representative Spanish population. Schizophr Res. 229:53–54. https://doi.org/10.1016/j.schres.2021.02.002.
- Cantuti-Castelvetri L, Ojha R, Pedro LD, Djannatian M, Franz J, Kuivanen S, van der Meer F, Kallio K, Kaya T, Anastasina M, et al. 2020, Nov 13. Neuropilin-1 facilitates SARS-CoV-2 cell entry and infectivity. Science. 370(6518):856–860. https://doi.org/10.1126/science.abd2985. Epub 2020 Oct 20. PMID: 33082293.
- Cao L, Goreshnik I, Coventry B, Case James B, Miller L, Kozodoy L, Chen RE, Carter L, Walls AC, Park YJ, et al. 2020, Oct 23. De novo design of picomolar SARS-CoV-2 miniprotein inhibitors. Science. 370(6515):426–431. https://doi.org/10.1126/science.abd9909. Epub 2020 Sep 9. PMID: 32907861.
- Carrasco-Sánchez FJ, López-Carmona MD, Martínez-Marcos FJ, Pérez-Belmonte LM, Hidalgo-Jiménez A, Buonaiuto V, Suárez Fernández C, Freire Castro SJ, Luordo D, Pesqueira Fontan PM, et al. 2021. Admission hyperglycemia as a predictor of mortality in patients hospitalized with COVID-19 regardless of diabetes status: data from the Spanish SEMI-COVID-19 registry. Ann Med. 53(1):103–116. https://doi.org/10.1080/07853890.2020.1836566.
- Çayan S, Uğuz M, Saylam B, Akbay E. 2020. Effect of serum total testosterone and its relationship with other laboratory parameters on the prognosis of coronavirus disease 2019 (COVID-19) in SARS-CoV-2 infected male patients: a cohort study. Aging Male. https://doi.org/10.1080/13685538.2020.1807930.
- CD24Fc as a Non-antiviral Immunomodulator in COVID-19 Treatment (SAC-COVID). [accessed 2021 Aug 17]. https://clinicaltrials.gov/ct2/show/NCT04317040.
- Cecchetti G, Vabanesi M, Chieffo R, Fanelli G, Minicucci F, Agosta F, Tresoldi M, Zangrillo A, Filippi M. 2020. Cerebral involvement in COVID-19 is associated with metabolic and coagulation derangements: an EEG study. J Neurol. 267:3130–3134. https://doi.org/10.1007/s00415-020-09958-2.
- Cevik M, Tate M, Lloyd O, Maraolo AE, Schafers J, Ho A. 2021. SARS-CoV-2, SARS-CoV, and MERS-CoV viral load dynamics, duration of viral shedding, and infectiousness: a systematic review and meta-analysis. Lancet Microbe. 2:e13–e22. https://doi.org/10.1016/S2666-5247(20)30172-5.
- Chaccour C, Casellas A, Matteo AB-D, Pineda I, Fernandez-Montero A, Ruiz-Castillo P, Richardson MA, Rodríguez-Mateos M, Jordán-Iborra C, Brew J, et al. 2021. The effect of early treatment with ivermectin on viral load, symptoms and humoral response in patients with non-severe COVID-19: a pilot, double-blind, placebo-controlled, randomized clinical trial. EClinicalMedicine. 32:100720. https://doi.org/10.1016/j.eclinm.2020.100720.
- Challen R, Brooks-Pollock E, Read J M, Dyson L, Tsaneva-Atanasova K, Danon L. 2021. Risk of mortality in patients infected with SARS-CoV-2 variant of concern 202012/1: matched cohort study. Br Med J. 372:n579. https://doi.org/10.1136/bmj.n579.
- Charoenngam N, Holick MF. 2020. Immunologic effects of vitamin D on human health and disease. Nutrients. 12:2097. https://doi.org/10.3390/nu12072097.
- Chen M, Shen W, Rowan NR, Kulaga H, Hillel A, Ramanathan M Jr, Lane AP. 2020a, Sep 24. Elevated ACE-2 expression in the olfactory neuroepithelium: implications for anosmia and upper respiratory SARS-CoV-2 entry and replication. Eur Respir J. 56(3):2001948. https://doi.org/10.1183/13993003.01948-2020.
- Chen W, Feng P, Liu K, Wu M, Lin H. 2020b. Computational Identification of Small Interfering RNA Targets in SARS-CoV-2. Virologica Sinica. 35(3):359–361. http://dx.doi.org/10.1007/s12250-020-00221-6.
- Chen X, Li R, Pan Z, Qian C, Yang Y, You R, Zhao J, Liu P, Gao L, Li Z, et al. 2020c. Human monoclonal antibodies block the binding of SARS-CoV-2 spike protein to angiotensin converting enzyme 2 receptor. Cellular & Molecular Immunology. 17(6):647–649. http://dx.doi.org/10.1038/s41423-020-0426-7.
- Chia PY, Xiang Ong SW, Chiew CJ, Ang LW, Chavatte JM, Mak TM, Cui L, Kalimuddin S, Chia WN, Tan CW, et al. 2021. Virological and serological kinetics of SARS-CoV-2 delta variant vaccine-breakthrough infections: a multi-center cohort study. Medrxiv. https://doi.org/10.1101/2021.07.28.21261295.
- Chiscano-Camón L, Ruiz-Rodriguez J, Ruiz-Sanmartin A, Roca O, Ferrer R. 2020. Vitamin C levels in patients with SARS-CoV-2-associated acute respiratory distress syndrome. Crit Care. 24:522. https://doi.org/10.1186/s13054-020-03249-y.
- Coelho AR, Oliveira PJ. 2020. Dihydroorotate dehydrogenase inhibitors in SARS-CoV-2 infection. Eur J Clin Investig. 50:e13366. https://doi.org/10.1111/eci.13366.
- Cornejo-Pareja I, Vegas-Aguilar IM, García-Almeida JM, Bellido-Guerrero D, Talluri A, Lukaski H, Tinahones FJ. 2021. Phase angle and standardized phase angle from bioelectrical impedance measurements as a prognostic factor for mortality at 90 days in patients with COVID-19: a longitudinal cohort study. Clin Nutr. https://doi.org/10.1016/j.clnu.2021.02.017.
- Coronavirus (COVID-19) Update: FDA authorizes first test that detects neutralizing antibodies from recent or prior SARS-CoV-2 infection. November 06, 2020. https://www.fda.gov/news-events/press-announcements/coronavirus-covid-19-update-fda-authorizes-first-test-detects-neutralizing-antibodies-recent-or.
- Coronavirus (COVID-19) Update: FDA Authorizes Drug for Treatment of COVID-19. 2021. [accessed 2021 Aug 17]. https://www.fda.gov/news-events/press-announcements/coronavirus-covid-19-update-fda-authorizes-drug-treatment-covid-19.
- Coronavirus (COVID-19) Update: FDA Authorizes Monoclonal Antibodies for Treatment of COVID-19. November 21, 2020. https://www.fda.gov/news-events/press-announcements/coronavirus-covid-19-update-fda-authorizes-monoclonal-antibodies-treatment-covid-19.
- Coronavirus (COVID-19) Update: FDA Authorizes Monoclonal Antibody for Treatment of COVID-19. November 09, 2020. https://www.fda.gov/news-events/press-announcements/coronavirus-covid-19-update-fda-authorizes-monoclonal-antibody-treatment-covid-19.
- Coronavirus is in the air — there’s too much focus on surfaces. Editorial, 02 February 2021. Nature. Available at https://www.nature.com/articles/d41586-021-00277-8.
- COVID-19 Biologics Tracker. [accessed 2021 Aug 17]. https://www.antibodysociety.org/covid-19-biologics-tracker/.
- COVID-19 Dashboard by the Center for Systems Science and Engineering (CSSE) at Johns Hopkins University (JHU). [accessed 2021 Aug 17]. https://gisanddata.maps.arcgis.com/apps/opsdashboard/.
- COVID-19 Host Genetics Initiative. 2021. Mapping the human genetic architecture of COVID-19. Nature. https://doi.org/10.1038/s41586-021-03767-x.
- COVID-19 Long-Acting AntiBody (LAAB) combination AZD7442 rapidly advances into Phase III clinical trials. 9 October 2020. https://www.astrazeneca.com/media-centre/press-releases/2020/covid-19-long-acting-antibody-laab-combination-azd7442-rapidly-advances-into-phase-iii-clinical-trials.html.
- Cox LS, Bellantuono I, Lord JM, Sapey E, Mannick JB, Partridge L, Gordon AL, Steves CJ, Witham MD. 2020. Tackling immunosenescence to improve COVID-19 outcomes and vaccine response in older adults. Lancet Healthy Longevity. 1(2). https://doi.org/10.1016/S2666-7568(20)30011-8.
- Crayne CB, Albeituni S, Nichols KE, Cron RQ. 2019. The immunology of macrophage activation syndrome. Front Immunol. 10:119. https://doi.org/10.3389/fimmu.2019.00119.
- Crespo-Facorro B, Ruiz-Veguilla M, Vázquez-Bourgon J, Sánchez-Hidalgo AC, Garrido-Torres N, Cisneros JM, Prieto C, Sainz J. 2021. Aripiprazole as a candidate treatment of COVID-19 identified through genomic analysis. Front Pharmacol. 12:646701. https://doi.org/10.3389/fphar.2021.646701.
- Crimi E, Benincasa G, Figueroa-Marrero N, Galdiero M, Napoli C. 2020. Epigenetic susceptibility to severe respiratory viral infections and its therapeutic implications: a narrative review. Br J Anaesth. 125:1002–1017. https://doi.org/10.1016/j.bja.2020.06.060.
- Curtis N, Sparrow A, Ghebreyesus TA, Netea MG. 2020. Considering BCG vaccination to reduce the impact of COVID-19. Lancet. 395:1545–1546. https://doi.org/10.1016/S0140-6736(20)31025-4.
- Dagan N, Barda N, Kepten E, Miron O, Perchik S, Katz MA, Hernán MA, Lipsitch M, Reis B, Balicer RD. 2021. BNT162b2 mRNA covid-19 vaccine in a nationwide mass vaccination setting. N Engl J Med. 384:1412–1423. https://doi.org/10.1056/NEJMoa2101765.
- Damette O, Mathonnat C, Goutte S, Troccoli A. 2021. Meteorological factors against COVID-19 and the role of human mobility. PLOS ONE. 16(6):e0252405. https://doi.org/10.1371/journal.pone.0252405.
- Damialis A, Gilles S, Sofiev M, Sofieva V, Kolek F, Bayr D, Plaza MP, Leier-Wirtz V, Kaschuba S, Ziska LH, et al. 2021, March. Higher airborne pollen concentrations correlated with increased SARS-CoV-2 infection rates, as evidenced from 31 countries across the globe. Proc Natl Acad Sci USA. 118(12):e2019034118. https://doi.org/10.1073/pnas.2019034118.
- Dance A. 2015, May 19. Core concept: CRISPR gene editing. Proc Natl Acad Sci U S A. 112(20):6245–6246. https://doi.org/10.1073/pnas.1503840112. PMID: 25991847; PMCID: PMC4443382.
- Daniloski Z, Jordan TX, Wessels H-H, Hoagland DA, Kasela S, Legut M, Maniatis S, Mimitou EP, Lu L, Geller E, et al. 2021. Identification of required host factors for SARS-CoV-2 infection in human cells. Cell. 184(1):92–105.e16. https://doi.org/10.1016/j.cell.2020.10.030.
- Debisarun PA, Struycken P, Domínguez-Andrés J, Moorlag SJCFM, Taks E, Gössling KL, Ostermann PN, Müller L, Schaal H, Oever JT, et al. 2020. The effect of influenza vaccination on trained immunity: impact on COVID-19. medRxiv. https://doi.org/10.1101/2020.10.14.20212498.
- Demers-Mathieu V, Do DM, Mathijssen GB, Sela DA, Seppo A, Järvinen KM, Medo E. 2020. Difference in levels of SARS-CoV-2 S1 and S2 subunits – and nucleocapsid protein-reactive SIgM/IgM, IgG and SIgA/IgA antibodies in human milk. J Perinatol. https://doi.org/10.1038/s41372-020-00805-w.
- de Moura MC, Davalos V, Planas-Serra L, Alvarez-Errico D, Arribas C, Ruiz M, Aguilera-Albesa S, Troya J, Valencia-Ramos J, Vélez-Santamaria V, et al. 2021. Epigenome-wide association study of COVID-19 severity with respiratory failure. EBioMedicine. 66:103339. https://doi.org/10.1016/j.ebiom.2021.103339.
- Depfenhart M, de Villiers D, Lemperle G, Meyer M, Di Somma S. 2020. Potential new treatment strategies for COVID-19: Is there a role for bromhexine as add-on therapy? Intern Emerg Med. 15(5):801–812. https://doi.org/10.1007/s11739-020-02383-3.
- de Vries RD, Schmitz KS, Bovier FT, Predella C, Khao J, Noack D, Haagmans BL, Herfst S, Stearns KL, Drew-Bear J, et al. 2021, Feb 17. Intranasal fusion inhibitory lipopeptide prevents direct-contact SARS-CoV-2 transmission in ferrets. Science. eabf4896. https://doi.org/10.1126/science.abf4896. Epub ahead of print. PMID: 33597220.
- de Vrieze J. 2020, March 23. Can a century-old TB vaccine steel the immune system against the new coronavirus?. https://www.sciencemag.org/news/2020/03/can-century-old-tb-vaccine-steel-immune-system-against-new-coronavirus.
- Di Micco R, Krizhanovsky V, Baker D, d’Adda di Fagagna F. 2021. Cellular senescence in ageing: from mechanisms to therapeutic opportunities. Nat Rev Mol Cell Biol. 22:75–95. https://doi.org/10.1038/s41580-020-00314-w.
- Ding Y, Zhou J, Wang S, Li W, Mi Y, Gao S, Xu Y, Chen Y, Yan J. 2018. Anti-neuropilin-1 monoclonal antibody suppresses the migration and invasion of human gastric cancer cells via Akt dephosphorylation. Exp Ther Med. 16(2):537–546. https://doi.org/10.3892/etm.2018.6234.
- Diorio C, McNerney KO, Lambert M, Paessler M, Anderson EM, Henrickson SE, Chase J, Liebling EJ, Burudpakdee C, Lee JH, et al. 2020. Evidence of thrombotic microangiopathy in children with SARS-CoV-2 across the spectrum of clinical presentations. Blood Adv. 4(23):6051–6063. https://doi.org/10.1182/bloodadvances.2020003471.
- Duarte-Neto AN, Caldini EG, Gomes-Gouvêa MS, Kanamura CT, de Almeida Monteiro RA, Ferranti JF, Ventura AMC, Regalio FA, Fiorenzano DM, Gibelli MABC, et al. 2021. An autopsy study of the spectrum of severe COVID-19 in children: from SARS to different phenotypes of MIS-C. EClinicalMedicine. 35. https://doi.org/10.1016/j.eclinm.2021.100850.
- Dufloo J, Grzelak L, Staropoli I, Madec Y, Tondeur L, Anna F, Pelleau S, Wiedemann A, Planchais C, Buchrieser J, et al. 2021. Asymptomatic and symptomatic SARS-CoV-2 infections elicit polyfunctional antibodies. Cell Reports Med. https://doi.org/10.1016/j.xcrm.2021.100275.
- Dutta NK, Mazumdar K, Gordy JT, Dutch RE. 2020. The nucleocapsid protein of SARS-CoV-2: a target for vaccine development. J Virol. 94(13):e00647–20. https://doi.org/10.1128/JVI.00647-20.
- European Centre for Disease Prevention and Control. Threat assessment brief: rapid increase of a SARS-CoV-2 variant with multiple spike protein mutations observed in the United Kingdom. 20 Dec 2020. https://www.ecdc.europa.eu/en/publications-data/threat-assessment-brief-rapid-increase-sars-cov-2-variant-united-kingdom.
- Evaluation of the Safety of CD24-Exosomes in Patients With COVID-19 Infection. [accessed 2021 Aug 17]. https://clinicaltrials.gov/ct2/show/NCT04747574.
- Fang L, Karakiulakis G, Roth M. 2020. Are patients with hypertension and diabetes mellitus at increased risk for COVID-19 infection? Lancet Respir Med. 8. https://doi.org/10.1016/S2213-2600(20)30116-8.
- Favalli EG, Biggioggero M, Maioli G, Caporali R. 2020. Baricitinib for COVID-19: a suitable treatment? Lancet Infect Dis. 20(9):1012–1013. https://doi.org/10.1016/S1473-3099(20)30262-0.
- Feldstein LR, Rose EB, Horwitz SM, Collins JP, Newhams MM, Son MBF, Newburger JW, Kleinman LC, Heidemann SM, Martin AA, et al. 2020. Multisystem inflammatory syndrome in U. S. children and adolescents. New England J Med. 383:334–346. https://doi.org/10.1056/NEJMoa2021680.
- Ferreira C, Viana SD, Reis F. 2020. Gut microbiota dysbiosis–immune hyperresponse–inflammation triad in coronavirus disease 2019 (COVID-19): impact of pharmacological and nutraceutical approaches. Microorganisms. 8:1514. https://doi.org/10.3390/microorganisms8101514.
- Ferreras C, Pascual-Miguel B, Mestre-Durán C, Navarro-Zapata A, Clares-Villa L, Martín-Cortázar C, De Paz R, Marcos A, Vicario JL, Balas A, et al. 2021. SARS-CoV-2 specific memory T lymphocytes from COVID-19 convalescent donors: identification, biobanking and large-scale production for adoptive cell therapy. Front Cell Dev Biol. 9:620730. https://doi.org/10.3389/fcell.2021.620730.
- Finkel Y, Gluck A, Nachshon A, Winkler R, Fisher T, Rozman B, Mizrahi O, Lubelsky Y, Zuckerman B, Slobodin B, et al. 2021. SARS-CoV-2 uses a multipronged strategy to impede host protein synthesis. Nature. https://doi.org/10.1038/s41586-021-03610-3.
- Fisher WG, Yang PC, Medikonduri RK, Jafri MS. 2006. NFAT and NFκB activation in T lymphocytes: a model of differential activation of gene expression. Ann Biomed Eng. 34(11):1712–1728. https://doi.org/10.1007/s10439-006-9179-4.
- Flanagan KL, Best E, Crawford NW, Giles M, Koirala A, Macartney K, Russell F, Teh BW, Wen SCH. 2020. Progress and pitfalls in the quest for effective SARS-CoV-2 (COVID-19) vaccines. Front Immunol. 11. https://doi.org/10.3389/fimmu.2020.579250.
- Foy BH, Carlson JCT, Reinertsen E, Padros I. Valls R, Pallares Lopez R, Palanques-Tost E, Mow C, Westover MB, Aguirre AD, Higgins JM. 2020. Association of red blood cell distribution width with mortality risk in hospitalized adults with SARS-CoV-2 infection. JAMA Network Open. 3(9):e2022058. https://doi.org/10.1001/jamanetworkopen.2020.22058.
- Frampton D, Rampling T, Cross A, Bailey H, Heaney J, Byott M, Scott R, Sconza R, Price J, Margaritis M, et al. 2021. Genomic characteristics and clinical effect of the emergent SARS-CoV-2 B.1.1.7 lineage in London, UK: a whole-genome sequencing and hospital-based cohort study. Lancet Infect Dis. https://doi.org/10.1016/S1473-3099(21)00170-5.
- Frontera JA, Rahimian JO, Yaghi S, Liu M, Lewis A, de Havenon A, Mainali S, Huang J, Scher E, Wisniewski T, et al. 2020, October 26. Treatment with zinc is associated with reduced in-hospital mortality among COVID-19 patients: a multi-center cohort study, PREPRINT (Version 1) available at Research Square [https://doi.org/10.21203/rs.3.rs-94509/v1].
- Fuschi C, Pu H, Negri M, Colwell R, Chen J. 2021. Wastewater-based epidemiology for managing the COVID-19 pandemic. ACS ES&T Water. https://doi.org/10.1021/acsestwater.1c00050.
- Gaebler C, Wang Z, Lorenzi JCC, Muecksch F, Finkin S, Tokuyama M, Cho A, Jankovic M, Schaefer-Babajew D, Oliveira TY, et al. 2021. Evolution of antibody immunity to SARS-CoV-2. Nature. 591:639–644. https://doi.org/10.1038/s41586-021-03207-w.
- Galvez J, Zanni R, Galvez-Llompart M, Benlloch JM. 2021. Macrolides may prevent severe acute respiratory syndrome coronavirus 2 entry into cells: a quantitative structure activity relationship study and Experimental validation. J Chem Inf Model. 61(4):2016–2025. https://doi.org/10.1021/acs.jcim.0c01394.
- Gao W, Chen S, Wang K, Chen R, Guo Q, Lu J, Wu X, He Y, Yan Q, Wang S, et al. 2020. Clinical features and efficacy of antiviral drug, arbidol in 220 nonemergency COVID-19 patients from east-west-lake shelter hospital in Wuhan: a retrospective case series. Virol J. 17:162. https://doi.org/10.1186/s12985-020-01428-5.
- Garrigues E, Janvier P, Kherabi Y, Le Bot A, Hamon A, Gouze H, Doucet L, Berkani S, Oliosi E, Mallart E, et al. 2020. Post-discharge persistent symptoms and health-related quality of life after hospitalization for COVID-19. J Infect. 81(6):e4–e6. https://doi.org/10.1016/j.jinf.2020.08.029.
- Gene cards. Furin gene. [accessed 2021 Aug 17]. https://www.genecards.org/cgi-bin/carddisp.pl?gene=FURIN.
- Ghosh S, Firdous SM, Nath A. 2020. siRNA could be a potential therapy for COVID-19. EXCLI J. 19:528–531. Published 2020 Apr 22. https://doi.org/10.17179/excli2020-1328.
- GISAID. [accessed 2021 Aug 17]. https://www.gisaid.org/.
- Golchin A. 2020. Cell-based therapy for severe COVID-19 patients: clinical trials and cost-utility. Stem Cell Reviews Reports. https://doi.org/10.1007/s12015-020-10046-1.
- Gold JE, Baumgartl WH, Okyay RA, Licht WE, Fidel PL, Noverr MC, Tilley LP, Hurley DJ, Rada B, Ashford JW, et al. Nov 2020. Analysis of measles-mumps-rubella (MMR) titers of recovered COVID-19 patients. mBio. 11(6):e02628–20. https://doi.org/10.1128/mBio.02628-20.
- Gonzalez-Rubio J, Navarro-Lopez C, Lopez-Najera E, Lopez-Najera A, Jimenez-Diaz L, Navarro-Lopez JD, Najera A. 2020. Cytokine release syndrome (CRS) and nicotine in COVID-19 patients: trying to calm the storm. Front Immunol. 11:1359. https://doi.org/10.3389/fimmu.2020.01359.
- Gordon AC, Mouncey PR, Al-Beidh F, Rowan KM, Nichol AD, Arabi YM, Annane D, Beane A, van Bentum-Puijk W, Berry LR, et al. 2021. Interleukin-6 receptor antagonists in critically Ill patients with covid-19 – preliminary report. The REMAP-CAP investigators. medRxiv; https://doi.org/10.1101/2021.01.07.21249390.
- Gordon DE, Jang GM, Bouhaddou M, Xu J, Obernier K, White KM, O’Meara MJ, Rezelj VV, Guo JZ, Swaney DL, et al. 2020. A SARS-CoV-2 protein interaction map reveals targets for drug repurposing. Nature. 583:459–468. https://doi.org/10.1038/s41586-020-2286-9.
- Gou W, Fu Y, Yue L, Chen GD, Cai X, Shuai M, Xu F, Yi X, Chen H, Zhu Y, et al. 2021. Gut microbiota, inflammation, and molecular signatures of host response to infection. J Genet Genomics. https://doi.org/10.1016/j.jgg.2021.04.002.
- Graham MS, Sudre CH, May A, Antonelli M, Murray B, Varsavsky T, Kläser K, Canas LS, Molteni E, Modat M, et al. 2021. Changes in symptomatology, reinfection, and transmissibility associated with the SARS-CoV-2 variant B.1.1.7: an ecological study. Lancet Public Health. https://doi.org/10.1016/S2468-2667(21)00055-4.
- Greaney AJ, Loes AN, Crawford KHD, Starr TN, Malone KD, Chu HY, Bloom JD. 2021a. Comprehensive mapping of mutations in the SARS-CoV-2 receptor-binding domain that affect recognition by polyclonal human plasma antibodies. Cell Host Microbe. 29(3):463–476.e6. https://doi.org/10.1016/j.chom.2021.02.003.
- Greaney AJ, Starr TN, Gilchuk P, Zost SJ, Binshtein E, Loes AN, Hilton SK, Huddleston J, Eguia R, Crawford KHD, et al. 2021b. Complete mapping of mutations to the SARS-CoV-2 spike receptor-binding domain that escape antibody recognition. Cell Host Microbe. 29(1):44–57.e9. https://doi.org/10.1016/j.chom.2020.11.007.
- Greinacher A, Thiele T, Warkentin TE, Weisser K, Kyrle PA, Eichinger S. 2021. Thrombotic thrombocytopenia after ChAdOx1 nCov-19 vaccination. N Engl J Med. https://doi.org/10.1056/NEJMoa2104840.
- Gribble J, Stevens LJ, Agostini ML, Anderson-Daniels J, Chappell JD, Lu X, Pruijssers AJ, Routh AL, Denison MR. 2021. The coronavirus proofreading exoribonuclease mediates extensive viral recombination. PLoS Pathog. 17(1): e1009226. https://doi.org/10.1371/journal.ppat.1009226.
- Griffin DO, Brennan-Rieder D, Ngo B, Kory P, Confalonieri M, Shapiro L, Iglesias J, Dube M, Nanda N, In GK, et al. 2021. The importance of understanding the stages of COVID-19 in treatment and trials. AIDS Rev. https://doi.org/10.24875/AIDSRev.200001261.
- Grifoni A, Weiskopf D, Ramirez SI, Mateus J, Dan JM, Moderbacher CR, Rawlings SA, Sutherland A, Premkumar L, Jadi RS, et al. 2020. Targets of T cell responses to SARS-CoV-2 coronavirus in humans with COVID-19 disease and unexposed individuals. Cell. 181:1489–1501.e15. https://doi.org/10.1016/j.cell.2020.05.015.
- Gu S, Chen Y, Wu Z, Chen Y, Gao H, Lv L, Guo F, Zhang X, Luo R, Huang C, et al. 2020a. Alterations of the Gut Microbiota in Patients With Coronavirus Disease 2019 or H1N1 Influenza. Clinical Infectious Diseases. 71(10):2669–2678. http://dx.doi.org/10.1093/cid/ciaa709.
- Gu SH, Yu CH, Song Y, Kim NY, Sim E, Choi JY, Song DH, Hur GH, Shin YK, Jeong ST. 2020b. A small interfering RNA lead targeting RNA-dependent RNA-polymerase effectively inhibit the SARS-CoV-2 infection in golden Syrian hamster and rhesus macaque. bioRxiv. https://doi.org/10.1101/2020.07.07.190967.
- Guerra-Silveira F, Abad-Franch F, Nishiura H. 2013. Sex bias in infectious disease epidemiology: patterns and processes. PLoS ONE. 8:e62390. https://doi.org/10.1371/journal.pone.0062390.
- Guimarães PO, Quirk D, Furtado RH, Maia LN, Saraiva JF, Antunes MO, Kalil Filho R, Junior VM, Soeiro AM, Tognon AP, et al. 2021. Tofacitinib in patients hospitalized with covid-19 pneumonia. N Engl J Med. https://doi.org/10.1056/NEJMoa2101643.
- Guisado-Vasco P, Valderas-Ortega S, Carralón-González MM, Roda-Santacruz A, González-Cortijo L, Sotres-Fernández G, Martí-Ballesteros EM, Luque-Pinilla JM, Almagro-Casado E, La Coma-Lanuza FJ, et al. 2020. Clinical characteristics and outcomes among hospitalized adults with severe COVID-19 admitted to a tertiary medical center and receiving antiviral, antimalarials, glucocorticoids, or immunomodulation with tocilizumab or cyclosporine: a retrospective observational study (COQUIMA cohort). EClinicalMedicine. 28:100591. https://doi.org/10.1016/j.eclinm.2020.100591.
- Gupta R, Hussain A, Misra A. 2020. Diabetes and COVID-19: evidence, current status and unanswered research questions. Eur J Clin Nutr. 74:864–870. https://doi.org/10.1038/s41430-020-0652-1.
- Haas EJ, Angulo FJ, McLaughlin JM, Anis E, Singer SR, Khan F, Brooks N, Smaja M, Mircus G, Pan K, et al. 2021. Impact and effectiveness of mRNA BNT162b2 vaccine against SARS-CoV-2 infections and COVID-19 cases, hospitalisations, and deaths following a nationwide vaccination campaign in Israel: an observational study using national surveillance data. Lancet. https://doi.org/10.1016/S0140-6736(21)00947-8.
- Hadjadj J, Yatim N, Barnabei L, Corneau A, Boussier J, Smith N, Péré H, Charbit B, Bondet V, Chenevier-Gobeaux C, et al. 2020. Impaired type I interferon activity and inflammatory responses in severe COVID-19 patients. Science. 369:718–724. http://doi.org/10.1126/science.abc6027.
- Hallal PC, Hartwig FP, Horta BL, Silveira MF, Struchiner CJ, Vidaletti LP, Neumann NA, Pellanda LC, Dellagostin OA, Burattini MN, et al. 2020. SARS-CoV-2 antibody prevalence in Brazil: results from two successive nationwide serological household surveys. Lancet Global Health. 8. https://doi.org/10.1016/S2214-109X(20)30387-9.
- Hampshire A, Trendera W, Chamberlain SR, Jolly AE, Grant JE, Patrick F, Mazibuko N, Williams SCR, Barnby JM, Hellyer P, et al. 2021. Cognitive deficits in people who have recovered from COVID-19. EClinicalMedicine. https://doi.org/10.1016/j.eclinm.2021.101044.
- Hanke L, Vidakovics Perez L, Sheward DJ, Das H, Schulte T, Moliner-Morro A, Corcoran M, Achour A, Karlsson Hedestam GB, Hällberg BM, et al. 2020. An alpaca nanobody neutralizes SARS-CoV-2 by blocking receptor interaction. Nat Commun. 11:4420. https://doi.org/10.1038/s41467-020-18174-5.
- Haug N, Geyrhofer L, Londei A, Dervic E, Desvars-Larrive A, Loreto V, Pinior B, Thurner S, Klimek P. 2020. Ranking the effectiveness of worldwide COVID-19 government interventions. Nat Hum Behav. 4:1303–1312. https://doi.org/10.1038/s41562-020-01009-0.
- Heffler E, Detoraki A, Contoli M, Papi A, Paoletti G, Malipiero G, Brussino L, Crimi C, Morrone D, Padovani M, et al. 2021. COVID-19 in severe asthma network in Italy (SANI) patients: clinical features, impact of comorbidities and treatments. Allergy. https://doi.org/10.1111/all.14532.
- Heuberger J, Trimpert J, Vladimirova D, Goosmann C, Lin M, Schmuck R, Mollenkopf HJ, Brinkmann V, Tacke F, Osterrieder N, et al. 2021. Epithelial response to IFN-γ promotes SARS-CoV-2 infection. EMBO Mol Med. 0:e13191. https://doi.org/10.15252/emmm.202013191.
- Hie B, Zhong ED, Berger B, Bryson B. 2021, Jan 15. Learning the language of viral evolution and escape. Science. 371(6526):284–288. https://doi.org/10.1126/science.abd7331. PMID: 33446556.
- Hippich M, Holthaus L, Assfalg R, Zapardiel-Gonzalo J, Kapfelsperger H, Heigermoser M, Haupt F, Ewald DA, Welzhofer TC, Marcus BA, et al. 2020. A public health antibody screening indicates a 6-fold higher SARS-CoV-2 exposure rate than reported cases in children. Med. https://doi.org/10.1016/j.medj.2020.10.003.
- Hippich M, Sifft P, Zapardiel-Gonzalo J, Böhmer MM, Lampasona V, Bonifacio E, Ziegler AG. 2021. A public health antibody screening indicates a marked increase of SARS-CoV-2 exposure rate in children during the second wave, Med, https://doi.org/10.1016/j.medj.2021.03.019.
- Hirano T, Murakami M. 2020. COVID-19: a new virus, but a familiar receptor and cytokine release syndrome. Immunity. 52:731–733. https://doi.org/10.1016/j.immuni.2020.04.003.
- Hoagland DA, Clarke DJB, Møller R, Han Y, Yang L, Wojciechowicz ML, Lachmann A, Oguntuyo KY, Stevens C, Lee B, et al. 2020. Modulating the transcriptional landscape of SARS-CoV-2 as an effective method for developing antiviral compounds. bioRxiv. https://doi.org/10.1101/2020.07.12.199687.
- Hodcroft EB, Zuber M, Nadeau S, Vaughan TG, Crawford KHD, Althaus CL, Reichmuth ML, Bowen JE, Walls AC, Corti D, et al. 2020. Emergence and spread of a SARS-CoV-2 variant through Europe in the summer of 2020. SeqCOVID-SPAIN consortium. Tanja Stadler, Richard A Neher MedRxiv. https://doi.org/10.1101/2020.10.25.20219063.
- Hodgson J. 2020. The pandemic pipeline. Nat Biotechnol. 38:523–532. https://doi.org/10.1038/d41587-020-00005-z.
- Holford P, Carr AC, Jovic TH, Ali SR, Whitaker IS, Marik PE, Smith AD. 2020. Vitamin C—an adjunctive therapy for respiratory infection, sepsis and COVID-19. Nutrients. 12:3760. https://doi.org/10.3390/nu12123760.
- Horby P, Lim WS, Emberson J, Mafham M, Bell JL, Linsell L, Staplin N, Brightling C, Ustianowski A, Elmahi E, et al. 2021a. RECOVERY collaborative group. Dexamethasone in hospitalized patients with covid-19. N Engl J Med. 384:693–704. https://doi.org/10.1056/NEJMoa2021436.
- Horby PW, Mafham M, Bell JL, Linsell L, Staplin N, Emberson J, Palfreeman A, Raw J, Elmahi E, Prudon B, et al. 2020. Lopinavir–ritonavir in patients admitted to hospital with COVID-19 (RECOVERY): a randomised, controlled, open-label, platform trial. Lancet. 396:1345–1352. https://doi.org/10.1016/S0140-6736(20)32013-4.
- Hu R, Han C, Pei S, Yin M, Chen X. 2020. Procalcitonin levels in COVID-19 patients. Int J Antimicrob Agents. 56. https://doi.org/10.1016/j.ijantimicag.2020.106051.
- Huang C, Huang L, Wang Y, Li X, Ren L, Gu X, Kang L, Guo L, Liu M, Zhou X, et al. 2021. 6-month consequences of COVID-19 in patients discharged from hospital: a cohort study. Lancet. 397:10270. https://doi.org/10.1016/S0140-6736(20)32656-8.
- Huang C, Wang Y, Li X, Ren L, Zhao J, Hu Y, Zhang L, Fan G, Xu J, Gu X, et al. 2020. Clinical features of patients infected with 2019 novel coronavirus in Wuhan, China. Lancet. 395:497–506. https://doi.org/10.1016/S0140-6736(20)30183-5.
- Ibarrondo FJ, Fulcher JA, Goodman-Meza D, Elliott J, Hofmann C, Hausner MA, Ferbas KG, Tobin NH, Aldrovand GM, Yang OO. 2020. Rapid decay of anti–SARS-CoV-2 antibodies in persons with mild covid-19. N Engl J Med. 383:1085–1087. https://doi.org/10.1056/NEJMc2025179.
- Igase M, Kohara K, Nagai T, Miki T, Ferrario CM. 2008. Increased expression of angiotensin converting enzyme 2 in conjunction with reduction of neointima by angiotensin II type 1 receptor blockade. Hypertens Res. 31:553–559. https://doi.org/10.1291/hypres.31.553.
- IMB. Functional genomics platform. [accessed 2021 Aug 17]. https://s2s-omxware.us-south.containers.appdomain.cloud/home, https://covid19-mol.mybluemix.net/.
- Immune Epitope Database (IEDB). [accessed 2021 Aug 17]. http://www.iedb.org/.
- Ioannou GN, Locke E, Green P, Berry K, O’Hare AM, Shah JA, Crothers K, Eastment MC, Dominitz JA, Fan VS. 2020. Risk factors for hospitalization, mechanical ventilation, or death among 10 131 US veterans with SARS-CoV-2 infection. JAMA Network Open. 3(9):e2022310. https://doi.org/10.1001/jamanetworkopen.2020.22310.
- Iwasaki A, Medzhitov R. 2015. Control of adaptive immunity by the innate immune system. Nat Immunol. 16:343–353. https://doi.org/10.1038/ni.3123.
- James MacLachlan N., and Dubovi Edward J. 2017. Chapter 4 – Antiviral immunity and virus vaccines. In: James MacLachlan N., and Dubovi Edward J., editors. Fenner's veterinary virology. Fifth ed. Academic Press; p. 79–104.
- Jamshaid H, Zahid F, Din IU, Zeb A, Choi HG, Khan GM, Din F. 2020. Diagnostic and treatment strategies for COVID-19. AAPS PharmSciTech. 21:222. https://doi.org/10.1208/s12249-020-01756-3.
- Janowitz T, Gablenz E, Pattinson D, Wang TC, Conigliaro J, Tracey K, Tuveson D. 2020. Famotidine use and quantitative symptom tracking for COVID-19 in non-hospitalised patients: a case series. Gut. 69:1592–1597. http://doi.org/10.1136/gutjnl-2020-321852.
- Jeon SM, Shin EA. 2018. Exploring vitamin D metabolism and function in cancer. Exp Mol Med. 50(20). https://doi.org/10.1038/s12276-018-0038-9.
- Jeong GU, Song H, Yoon GY, Kim D, Kwon Y-C. 2020. Therapeutic strategies against COVID-19 and structural characterization of SARS-CoV-2: a review. Front Microbiol. 11:1723. https://doi.org/10.3389/fmicb.2020.01723.
- Jessica W, Alex R, Jonathan D. 2020. Testing for SARS-CoV-2 antibodies. Br Med J. 370:m3325. https://doi.org/10.1136/bmj.m3325.
- Johansson MA, Quandelacy TM, Kada S, Prasad PV, Steele M, Brooks JT, Slayton RB, Biggerstaff M, Butler JC. 2021. SARS-CoV-2 transmission from people without COVID-19 symptoms. JAMA Network Open. 4(1):e2035057. https://doi.org/10.1001/jamanetworkopen.2020.35057.
- Johnson SC, Rabinovitch PS, Kaeberlein M. 2013. mTOR is a key modulator of ageing and age-related disease. Nature. 493(7432):338–345. https://doi.org/10.1038/nature11861.
- Joung J, Ladha A, Saito M, Kim NG, Woolley AE, Segel M, Barretto RPJ, Ranu A, Macrae RK, Faure G, et al. 2020. Detection of SARS-CoV-2 with SHERLOCK one-pot testing. N Engl J Med. 383:1492–1494. https://doi.org/10.1056/NEJMc2026172.
- Joyner MJ, Senefeld JW, Mills JR, Johnson PW, Theel ES, Wiggins CC, Bruno KA, Klompas AM, Lesser ER, Kunze KL, et al. 2020. Effect of convalescent plasma on mortality among hospitalized patients with COVID-19: initial three-month experience. medRxiv. https://doi.org/10.1101/2020.08.12.20169359.
- Kalra P, Dhiman A, Cho WC, Bruno JG, Sharma TK. 2018. Simple methods and rational design for enhancing aptamer sensitivity and specificity. Front Mol Biosci. 5:41. https://doi.org/10.3389/fmolb.2018.00041.
- Kaneko N, Kuo H-H, Boucau J, Farmer JR, Allard-Chamard H, Mahajan VS, Piechocka-Trocha A, Lefteri K, Osborn M, Bals J, et al. 2020. Loss of Bcl-6-expressing T follicular helper cells and germinal centers in COVID-19. Cell. 183:143–157.e13. https://doi.org/10.1016/j.cell.2020.08.025.
- Kang Z, Luo S, Gui Y, Zhou H, Zhang Z, Tian C, Zhou Q, Wang Q, Hu Y, Fan H, et al. 2020. Obesity is a potential risk factor contributing to clinical manifestations of COVID-19. Int J Obes. 44:2479–2485. https://doi.org/10.1038/s41366-020-00677-2.
- Karahan S, Katkat F. 2020. Impact of serum 25(OH) vitamin D level on mortality in patients with COVID-19 in Turkey. J Nutr Health Aging. https://doi.org/10.1007/s12603-020-1479-0.
- Kaufman HW, Niles JK, Kroll MH, Bi C, Holick MF, Reddy SV. 2020. SARS-CoV-2 positivity rates associated with circulating 25-hydroxyvitamin D levels. PLOS ONE. 15(9):e0239252. https://doi.org/10.1371/journal.pone.0239252.
- Kemp SA, Collier DA, Datir RP, Ferreira IATM, Gayed S, Jahun A, Hosmillo M, Rees-Spear C, Mlcochova P, Lumb IU, et al. 2021. SARS-CoV-2 evolution during treatment of chronic infection. Nature. https://doi.org/10.1038/s41586-021-03291-y.
- Khadke S, Ahmed N, Ahmed N, Ratts R, Raju S, Gallogly M, de Lima M, Sohail MR. 2020. Harnessing the immune system to overcome cytokine storm and reduce viral load in COVID-19: a review of the phases of illness and therapeutic agents. Virol J. 17:154. https://doi.org/10.1186/s12985-020-01415-w.
- Kimura I, Kosugi Y, Wu J, Yamasoba D, Butlertanaka EP, Tanaka YL, Liu Y, Shirakawa K, Kazuma Y, Nomura R, et al. 2021. SARS-CoV-2 lambda variant exhibits higher infectivity and immune resistance. Biorxiv. https://doi.org/10.1101/2021.07.28.454085.
- Kiros M, Andualem H, Kiros T, Hailemichael W, Getu S, Geteneh A, Alemu D, Abegaz WE. 2020. COVID-19 pandemic: current knowledge about the role of pets and other animals in disease transmission. Virol J. 17:143. https://doi.org/10.1186/s12985-020-01416-9.
- Kissler S, Fauver JR, Mack C, Tai CG, Breban MI, Watkins AE, Samant RM, Anderson DJ, Ho DD, Grubaugh ND, et al. 2021. Densely sampled viral trajectories suggest longer duration of acute infection with B.1.1.7 variant relative to non-B.1.1.7 SARS-CoV-2. Preprint. https://nrs.harvard.edu/URN-3:HUL.INSTREPOS:37366884.
- Klang S, Mann B, Meggido D, Tair L, Naparstek Y. 2021, January 20. Low pH Hypromellose (Taffix™) nasal powder spray reduced SARS-CoV-2 infection rate post mass-gathering event at a highly endemic community: An observational prospective open label user survey, PREPRINT (Version 2) available at Research Square. https://doi.org/10.21203/rs.3.rs-100328/v2.
- Klein S, Flanagan K. 2016. Sex differences in immune responses. Nat Rev Immunol. 16:626–638. https://doi.org/10.1038/nri.2016.90.
- Kokic G, Hillen HS, Tegunov D, Dienemann C, Seitz F, Schmitzova J, Farnung L, Siewert A, Höbartner C, Cramer P. 2021. Mechanism of SARS-CoV-2 polymerase stalling by remdesivir. Nat Commun. 12:279. https://doi.org/10.1038/s41467-020-20542-0.
- Korber B, Fischer WM, Gnanakaran S, Yoon H, Theiler J, Abfalterer W, Hengartner N, Giorgi EE, Bhattacharya T, Foley B, et al. 2020. Tracking changes in SARS-CoV-2 spike: evidence that D614G increases infectivity of the COVID-19 virus. Cell. 182(4):812–827.e19. https://doi.org/10.1016/j.cell.2020.06.043.
- Kow CS, Capstick T, Hasan SS. 2021. Are severe asthma patients at higher risk of developing severe outcomes from COVID-19? Allergy. https://doi.org/10.1111/all.14589.
- Kowarz E, Krutzke L, Reis J, Bracharz S, Kochanek S, Marschalek R. 2021, May 26. “Vaccine-Induced Covid-19 Mimicry” Syndrome: Splice reactions within the SARS-CoV-2 Spike open reading frame result in Spike protein variants that may cause thromboembolic events in patients immunized with vector-based vaccines. PREPRINT (Version 1) available at Research Square, https://doi.org/10.21203/rs.3.rs-558954/v1.
- Krammer F. 2020. SARS-CoV-2 vaccines in development. Nature. 586:516–527. https://doi.org/10.1038/s41586-020-2798-3.
- Kumar S, Zhi K, Mukherji A, Gerth K. 2020. Repurposing antiviral protease inhibitors using extracellular vesicles for potential Therapy of COVID-19. Viruses. 12(5):486. Published 2020 Apr 26. https://doi.org/10.3390/v12050486.
- Kuo C-L, Pilling LC, Atkins JL, Masoli JAH, Delgado J, Kuchel GA, Melzer D. 2020, November. APOE e4 genotype predicts severe COVID-19 in the UK biobank community cohort. J Gerontol: Ser A. 75(11):2231–2232. https://doi.org/10.1093/gerona/glaa131.
- Kwok S, Adam S, Ho JH, Iqbal Z, Turkington P, Razvi S, Le Roux CW, Soran H, Syed AA. 2020. Obesity: a critical risk factor in the COVID-19 pandemic. Clin Obes. 10. https://doi.org/10.1111/cob.12403.
- Kwon PS, Oh H, Kwon SJ, Jin W, Zhang F, Fraser K, Hong JJ, Linhardt RJ, Dordick JS. 2020. Sulfated polysaccharides effectively inhibit SARS-CoV-2 in vitro. Cell Discov. 6:50. https://doi.org/10.1038/s41421-020-00192-8.
- La Marca A, Capuzzo M, Paglia T, Roli L, Trenti T, Nelson SM. Testing for SARS-CoV-2 (COVID-19): a systematic review and clinical guide to molecular and serological in-vitro diagnostic assays, Reproductive BioMedicine Online 41, 2020, https://doi.org/10.1016/j.rbmo.2020.06.001.
- Laxminarayan R, Wahl B, Dudala SR, Gopal K, Mohan BC, Neelima S, Jawahar RKS, Radhakrishnan J, Lewnard JA. 2020, Nov 6. Epidemiology and transmission dynamics of COVID-19 in two Indian states. Science. 370(6517):691–697. https://doi.org/10.1126/science.abd7672. Epub 2020 Sep 30. PMID: 33154136.
- Ledford H. Nature. How does COVID-19 kill? Uncertainty is hampering doctors’ ability to choose treatments. 9 April 2020. https://www.nature.com/articles/d41586-020-01056-7.
- Lee C, Choi WJ. 2021. Overview of COVID-19 inflammatory pathogenesis from the therapeutic perspective. Arch Pharmacal Res. 44. https://doi.org/10.1007/s12272-020-01301-7.
- Lee S, Channappanavar R, Kanneganti T-D. 2020. Coronaviruses: innate immunity, inflammasome activation, inflammatory cell death, and cytokines. Trends Immunol. 41. https://doi.org/10.1016/j.it.2020.10.005.
- Lehrer S, Rheinstein PH. 2021. ABO blood groups, COVID-19 infection and mortality. Blood Cells, Molecules, Diseases. 89:102571. https://doi.org/10.1016/j.bcmd.2021.102571.
- Lei Y, Zhang J, Schiavon CR, He M, Chen L, Shen H, Zhang Y, Yin Q, Cho Y, Andrade L, et al. 2021. SARS-CoV-2 spike protein impairs endothelial function via downregulation of ACE 2. Circ Res. 128(9):1323–1326. https://doi.org/10.1161/CIRCRESAHA.121.318902.
- Li F, Li Y-Y, Liu M-J, Fang L-Q, Dean NE, Wong QWK, Yang X-B, Longini I, Halloran ME, Wang H-J, et al. 2021a. Household transmission of SARS-CoV-2 and risk factors for susceptibility and infectivity in Wuhan: a retrospective observational study. Lancet Infect Dis. https://doi.org/10.1016/S1473-3099(20)30981-6.
- Li G, De Clercq E. 2020. Therapeutic options for the 2019 novel coronavirus (2019-nCoV). Nat Rev Drug Discovery. 19:149–150. https://doi.org/10.1038/d41573-020-00016-0.
- Li H, Zhou Y, Zhang M, Wang H, Zhao Q, Liu J. 2020a. Updated approaches against SARS-CoV-2. Antimicrob Agents Chemother. 64:e00483–20. https://doi.org/10.1128/AAC.00483-20.
- Li J, Guo M, Tian X, Wang X, Yang X, Wu P, Liu C, Xiao Z, Qu Y, Yin Y, et al. 2021b. Virus-host interactome and proteomic survey reveal potential virulence factors influencing SARS-CoV-2 pathogenesis. Med. 2(1):99–112.E7. https://doi.org/10.1016/j.medj.2020.07.002.
- Li W, Schäfer A, Kulkarni SS, Liu X, Martinez DR, Chen C, Sun Z, Leist SR, Drelich A, Zhang L, et al. 2020b. High potency of a bivalent human VH domain in SARS-CoV-2 animal models. Cell. 183. https://doi.org/10.1016/j.cell.2020.09.007.
- Li Y, Campbell H, Kulkarni D, Harpur A, Nundy M, Wang X, Nair H. 2021c. The temporal association of introducing and lifting non-pharmaceutical interventions with the time-varying reproduction number (R) of SARS-CoV-2: a modelling study across 131 countries. The Lancet Infectious Diseases. 21(2):193–202. http://dx.doi.org/10.1016/S1473-3099(20)30785-4.
- Libster R, Marc GP, Wappner D, Coviello S, Bianchi A, Braem V, Esteban I, Caballero MT, Wood C, Berrueta M, et al. 2021. Early high-titer plasma therapy to prevent severe Covid-19 in older adults. N Engl J Med. https://doi.org/10.1056/NEJMoa2033700.
- Lieberman NAP, Peddu V, Xie H, Shrestha L, Huang ML, Mears MC, Cajimat MN, Bente BA, Shi P-Y, Bovier F, et al. 2020. In vivo antiviral host transcriptional response to SARS-CoV-2 by viral load, sex, and age. PLoS Biol. 18(9):e3000849. https://doi.org/10.1371/journal.pbio.3000849.
- Lilly announces proof of concept data for neutralizing antibody LY-CoV555 in the COVID-19 outpatient setting. 2020, September 16. https://investor.lilly.com/news-releases/news-release-details/lilly-announces-proof-concept-data-neutralizing-antibody-ly.
- Lim D, Rocchio F, Mapelli L, Moccia F. 2016. From pathology to physiology of calcineurin signalling in astrocytes. Opera Medica et Physiologica. 2(2):122–140. https://doi.org/10.20388/OMP2016.002.0029.
- Lindan CE, Mankad K, Ram D, Kociolek LK, Silvera VM, Boddaert N, Stivaros SM, Palasis S, Akhtar S, Alden D, et al. 2020. Neuroimaging manifestations in children with SARS-CoV-2 infection: a multinational, multicentre collaborative study. Lancet Child Adolesc Health. https://doi.org/10.1016/S2352-4642(20)30362-X.
- Liotta EM, Batra A, Clark JR, Shlobin NA, Hoffman SC, Orban ZS, Koralnik IJ 2020. Frequent neurologic manifestations and encephalopathy-associated morbidity in covid-19 patients. Ann Clin Transl Neurol. 7:2221–2230. https://doi.org/10.1002/acn3.51210.
- Lippi G, Plebani M. 2020. Laboratory abnormalities in patients with COVID-2019 infection. Clin Chem Lab Med (CCLM). 58(7):1131–1134. https://doi.org/10.1515/cclm-2020-0198.
- Liu F, Xu A, Zhang Y, Xuan W, Yan T, Pan K, Yu W, Zhang J 2020. Patients of COVID-19 may benefit from sustained lopinavir-combined regimen and the increase of Eosinophil may predict the outcome of COVID-19 progression. Int J Infect Dis. 95. https://doi.org/10.1016/j.ijid.2020.03.013.
- Liu T, Zhang L, Joo D, Sun S-C, 2017. NF-κB signaling in inflammation. Signal Transduction Targeted Ther. 2:17023. https://doi.org/10.1038/sigtrans.2017.23.
- Liu Y, Liu J, Xia H, Zhang X, Fontes-Garfias CR, Swanson KA, Cai H, Sarkar R, Chen W, Cutler M, et al. 2021a. Neutralizing Activity of BNT162b2-Elicited Serum. New England Journal of Medicine. 384(15):1466–1468. http://dx.doi.org/10.1056/NEJMc2102017.
- Liu Y, Morgenstern C, Kelly J, Lowe R, Jit M. 2021b. The impact of non-pharmaceutical interventions on SARS-CoV-2 transmission across 130 countries and territories. BMC Medicine. 19(1):553. http://dx.doi.org/10.1186/s12916-020-01872-8.
- Logunov DY, Dolzhikova IV, Shcheblyakov DV, Tukhvatulin AI, Zubkova OV, Dzharullaeva AS, Kovyrshina AV, Lubenets NL, Grousova DM, Erokhova AS, et al. 2021. Safety and efficacy of an rAd26 and rAd5 vector-based heterologous prime-boost COVID-19 vaccine: an interim analysis of a randomised controlled phase 3 trial in Russia. Lancet. https://doi.org/10.1016/S0140-6736(21)00234-8.
- London researchers to test promising coronavirus treatment. Julia Bakker. 15 June 2020. https://www.bhf.org.uk/what-we-do/news-from-the-bhf/news-archive/2020/june/london-researchers-to-test-promising-coronavirus-treatment.
- Long QX, Tang XJ, Shi QL, Li Q, Deng HJ, Yuan J, Hu JL, Xu W, Zhang Y, Lv FJ, et al. 2020. Clinical and immunological assessment of asymptomatic SARS-CoV-2 infections. Nat Med. 26:1200–1204. https://doi.org/10.1038/s41591-020-0965-6.
- Losina E, Leifer V, Millham L, Panella C, Hyle EP, Mohareb AM, Neilan AM, Ciaranello AL, Kazemian P, Freedberg KA. College campuses and COVID-19 mitigation: clinical and economic value. Ann Intern Med. Epub ahead of print 2020, December 21. https://doi.org/10.7326/M20-6558.
- Lu H, Weintz C, Pace J, Indana D, Linka K, Kuhl E. 2021. Are college campuses superspreaders? A data-driven modeling study. Comput Methods Biomech Biomed Engin. https://doi.org/10.1080/10255842.2020.1869221.
- Mahdi M, Mótyán JA, Szojka ZI, Golda M, Miczi M, Tőzsér J. 2020. Analysis of the efficacy of HIV protease inhibitors against SARS-CoV-2′s main protease. Virol J. 17:190. https://doi.org/10.1186/s12985-020-01457-0.
- Mahmudpour M, Roozbeh J, Keshavarz M, Farrokhi S, Nabipour I. 2020 Sep. COVID-19 cytokine storm: the anger of inflammation. Cytokine. 133:155151. https://doi.org/10.1016/j.cyto.2020.155151. Epub 2020 May 30. PMID: 32544563; PMCID: PMC7260598.
- Maltagliati S, Sieber S, Sarrazin P, Cullati S, Chalabaev A, Millet GP, Boisgontier MP, Cheval B. 2021. Muscle strength explains the protective effect of physical activity against COVID-19 hospitalization among adults aged 50 years and older. medRxiv. https://doi.org/10.1101/2021.02.25.21252451.
- Manirul Haque SK, Ashwaq O, Sarief A, Azad John Mohamed AK. 2020. A comprehensive review about SARS-CoV-2. Future Virol. 15(9):625–648. https://doi.org/10.2217/fvl-2020-0124.
- Manne BK, Denorme F, Middleton EA, Portier I, Rowley JW, Stubben C, Petrey AC, Tolley ND, Guo L, Cody M, et al. 2020. Platelet gene expression and function in patients with COVID-19. Blood. 136(11):1317–1329. https://doi.org/10.1182/blood.2020007214.
- Mannick JB, Morris M, Hockey HP, Roma G, Beibel M, Kulmatycki K, Watkins M, Shavlakadze T, Zhou W, Quinn D, et al. 2018, July 11. TORC1 inhibition enhances immune function and reduces infections in the elderly. Sci Transl Med. 10(449):eaaq1564. https://doi.org/10.1126/scitranslmed.aaq1564. PMID: 29997249.
- Marín-Hernández D, Schwartz RE, Nixon DF. 2021. Epidemiological evidence for association between higher influenza vaccine uptake in the elderly and lower COVID-19 deaths in Italy. J Med Virol. 93:64–65. https://doi.org/10.1002/jmv.26120.
- Marouf N, Cai W, Said KN, Daas H, Diab H, Chinta VR, Hssain AA, Nicolau B, Sanz M, Tamimi F. 2021. Association between periodontitis and severity of COVID-19 infection: a case-control study. J Clin Periodontol. Accepted Author Manuscript. https://doi.org/10.1111/jcpe.13435.
- Martínez-Fleta P, Alfranca A, González-Álvaro I, Casasnovas JM, Fernández-Soto D, Esteso G, Cáceres-Martell Y, Gardeta S, López-Sanz C, Prat S, et al. 2020, December 1. SARS-CoV-2 cysteine-like protease antibodies can be detected in serum and saliva of COVID-19–seropositive individuals. J Immunol. 205(11):3130–3140. https://doi.org/10.4049/jimmunol.2000842.
- Masana L, Correig E, Rodríguez-Borjabad C, Anoro E, Arroyo JA, Jericó C, Pedragosa A, Miret M, Näf S, Pardo A, et al. 2020. On behalf of the STACOV-XULA research group. Effect of statin therapy on SARS-CoV-2 infection-related mortality in hospitalized patients, European Heart Journal – Cardiovascular Pharmacotherapy, pvaa128, https://doi.org/10.1093/ehjcvp/pvaa128.
- McComb S, Thiriot A, Akache B, Krishnan L, Stark F. 2019. Introduction to the immune system. In: Fulton K., Twine S, editor. Immunoproteomics. Methods in molecular biology, vol 2024. New York, NY: Humana; https://doi.org/10.1007/978-1-4939-9597-4_1.
- McDade J. Genome-wide screening using CRISPR. Updated on Aug 20, 2020. https://blog.addgene.org/genome-wide-screening-using-crispr/cas9.
- Medeiros D. Mar 4, 2020. Press release: generex signs contract with EpiVax to develop Ii-key peptide vaccines to address the coronavirus pandemic. https://epivax.com/news/press-release-generex-signs-contract-with-epivax-to-develop-ii-key-peptide-vaccines-to-address-the-coronavirus-pandemic.
- Meinhardt J, Radke J, Dittmayer C, Franz J, Thomas C, Mothes R, Laue M, Schneider J, Brünink S, Greuel S, et al. 2020. Olfactory transmucosal SARS-CoV-2 invasion as a port of central nervous system entry in individuals with COVID-19. Nat Neurosci. https://doi.org/10.1038/s41593-020-00758-5.
- Meister TL, Brüggemann Y, Todt D, Conzelmann C, Müller JA, Groß R, Münch J, Krawczyk A, Steinmann J, Steinmann J, et al. 2020, October 15. Virucidal efficacy of different oral rinses against severe acute respiratory syndrome coronavirus 2. J Infect Dis. 222(8):1289–1292. https://doi.org/10.1093/infdis/jiaa471.
- Merzon E, Tworowski D, Gorohovski A, Vinker S, Golan Cohen A, Green I, Frenkel-Morgenstern M. 2020. Low plasma 25(OH) vitamin D level is associated with increased risk of COVID-19 infection: an Israeli population-based study. FEBS J. 287:3693–3702. https://doi.org/10.1111/febs.15495.
- Messner CB, Demichev V, Wendisch D, Michalick L, White M, Freiwald A, Textoris-Taube K, Vernardis SI, Egger AS, Kreidl M, et al. 2020. Ultra-high-throughput clinical proteomics reveals classifiers of COVID-19 infection. Cell Syst. 11:11–24.e4. https://doi.org/10.1016/j.cels.2020.05.012.
- Milken Institute. COVID-19 treatment and vaccine tracker. Last updated: February 2, 2021. https://covid-19tracker.milkeninstitute.org/.
- Moghadas SM, Fitzpatrick MC, Sah P, Pandey A, Shoukat A, Singer BH, Galvani AP. 2020, July. The implications of silent transmission for the control of COVID-19 outbreaks. Proc Natl Acad Sci USA. 117(30):17513–17515. https://doi.org/10.1073/pnas.2008373117.
- Mogul A, Corsi K, McAuliffe L. 2019 Sep. Baricitinib: the second FDA-approved JAK inhibitor for the treatment of rheumatoid arthritis. Ann Pharmacother. 53(9):947–953. https://doi.org/10.1177/1060028019839650. Epub 2019 Mar 24. PMID: 30907116.
- Monk PD, Marsden RJ, Tear VJ, Brookes J, Batten TN, Mankowski M, Gabbay FJ, Davies DE, Holgate ST, Ho LP, et al. 2020. Safety and efficacy of inhaled nebulised interferon beta-1a (SNG001) for treatment of SARS-CoV-2 infection: a randomised, double-blind, placebo-controlled, phase 2 trial. Lancet Respir Med. https://doi.org/10.1016/S2213-2600(20)30511-7.
- Monoclonal Antibodies Overview. [accessed 2021 Aug 17]. https://www.genscript.com/how-to-make-monoclonal-antibodies.html.
- Moorlag SJCFM, Arts RJW, van Crevel R, Netea MG. 2019. Non-specific effects of BCG vaccine on viral infections. Clin Microbiol Infect. 25. https://doi.org/10.1016/j.cmi.2019.04.020.
- Moutal A, Martin L, Boinon L, Gomez K, Ran D, Zhou Y, Stratton HJ, Cai S, Luo S, Gonzalez KB, et al. 2021, January. SARS-CoV-2 spike protein co-opts VEGF-A/neuropilin-1 receptor signaling to induce analgesia. PAIN. 162(1):243–252. https://doi.org/10.1097/j.pain.0000000000002097.
- Munnink BB O, Sikkema RS, Nieuwenhuijse DF, Molenaar RJ, Munger E, Molenkamp R, van der Spek A, Tolsma P, Rietveld A, Brouwer M, et al. 2021. Transmission of SARS-CoV-2 on mink farms between humans and mink and back to humans. Science. http://doi.org/10.1126/science.abe5901.
- Muñoz X, Pilia F, Ojanguren I, Romero-Mesones C, Cruz MJ. 2020. Is asthma a risk factor for COVID-19? Are phenotypes important? ERJ Open Res. https://doi.org/10.1183/23120541.00216-2020.
- Muñoz-Basagoiti J, Perez-Zsolt D, León R, Blanc V, Raïch-Regué D, Cano-Sarabia M, Trinité B, Pradenas E, Blanco J, Gispert J, et al. 2021, July. Mouthwashes with CPC reduce the infectivity of SARS-CoV-2 variants in vitro. J Dent Res. https://doi.org/10.1177/00220345211029269.
- NCBI Resources. Gene. EEF1A1 eukaryotic translation elongation factor 1 alpha 1. Updated on 2021, Jan 29. https://www.ncbi.nlm.nih.gov/gene/1915.
- Neeland MR, Bannister S, Clifford V, Dohle K, Mulholland K, Sutton P, Curtis N, Steer AC, Burgner DP, Crawford NW, et al. 2021. Innate cell profiles during the acute and convalescent phase of SARS-CoV-2 infection in children. Nat Commun. 12:1084. https://doi.org/10.1038/s41467-021-21414-x.
- Nelde A, Bilich T, Heitmann JS, Maringer Y, Salih HR, Roerden M, Lübke M, Bauer J, Rieth J, Wacker M, et al. SARS-CoV-2 T-cell epitopes define heterologous and COVID-19-induced T-cell recognition, 2020, June 16. PREPRINT available at Research Square. https://doi.org/10.21203/rs.3.rs-35331/v1.
- Nelson CW, Ardern Z, Goldberg TL, Meng C, Kuo CH, Ludwig C, Kolokotronis SO, Wei X. 2020. Dynamically evolving novel overlapping gene as a factor in the SARS-CoV-2 pandemic. eLife. 9:e59633. https://doi.org/10.7554/eLife.59633.
- Nguyen A, David JK, Maden SK, Wood MA, Weeder BR, Nellore A, Thompson RF, Gallagher T. 2020, June. Human leukocyte antigen susceptibility map for severe acute respiratory syndrome coronavirus 2. J Virol. 94(13):e00510–20. https://doi.org/10.1128/JVI.00510-20.
- NHS. Men make more coronavirus antibodies than women, making them better plasma donors. 2020, June 23. https://www.nhsbt.nhs.uk/news/men-make-more-coronavirus-antibodies-than-women/.
- Nigro P, Pompilio G, Capogrossi M. 2013. Cyclophilin A: a key player for human disease. Cell Death Disease. 4:e888. https://doi.org/10.1038/cddis.2013.410.
- Nogrady B. 2020. How kids’ immune systems can evade COVID. Nature. 588:382. https://doi.org/10.1038/d41586-020-03496-7.
- Nojomi M, Yassin Z, Keyvani H, Makiani MJ, Roham M, Laali A, Dehghan N, Navaei M, Ranjbar M. 2020. Effect of arbidol (umifenovir) on COVID-19: a randomized controlled trial. BMC Infect Dis. 20:954. https://doi.org/10.1186/s12879-020-05698-w.
- Novavax COVID-19 Vaccine Demonstrates 89.3% Efficacy in UK Phase 3 Trial. 2021, January 28. https://ir.novavax.com/node/15506/pdf.
- Nuovo GJ, Magro C, Shaffer T, Awad H, Suster D, Mikhail S, He B, Michaille JJ, Liechty B, Tili E. 2021. Endothelial cell damage is the central part of COVID-19 and a mouse model induced by injection of the S1 subunit of the spike protein. Ann Diagn Pathol. 51:151682. https://doi.org/10.1016/j.anndiagpath.2020.151682.
- O’Donnell VB, Thomas D, Stanton R, Maillard JY, Murphy RC, Jones SA, Humphreys I, Wakelam MJO, Fegan C, Wise MP, et al. 2020. Potential role of oral rinses targeting the viral lipid envelope in SARS-CoV-2 infection. Function. 1(1):zqaa002. https://doi.org/10.1093/function/zqaa002.
- Oldenburg CE, Doan T. 2020. Azithromycin for severe COVID-19. Lancet. 396:936–937. https://doi.org/10.1016/S0140-6736(20)31863-8.
- Onco’Zine. Plitidepsin – a novel Anti-cancer agent possibly active against COVID19. Peter Hofland. March 16, 2020. https://www.oncozine.com/plitidepsin-a-novel-anti-cancer-agent-possibly-active-against-covid18/.
- O’Neill LAJ, Netea MG. 2020. BCG-induced trained immunity: Can it offer protection against COVID-19? Nat Rev Immunol. 20:335–337. https://doi.org/10.1038/s41577-020-0337-y.
- Oryzon. 2021. Vafidemstat. [accessed 2021 Aug 17]. https://www.oryzon.com/es/programas/vafidemstat.
- Osman MM, Lulic D, Glover L, Stahl CE, Lau T, van Loveren H, Borlongan CV. 2011. Cyclosporine-A as a neuroprotective agent against stroke: its translation from laboratory research to clinical application. Neuropeptides. 45:359–368. https://doi.org/10.1016/j.npep.2011.04.002.
- Pairo-Castineira E, Clohisey S, Klaric L, Bretherick AD, Rawlik K, Pasko D, Walker S, Parkinson N, Fourman MH, Russell CD, et al. 2020. Genetic mechanisms of critical illness in covid-19. Nature. https://doi.org/10.1038/s41586-020-03065-y.
- Pamukçu B. 2020. Inflammation and thrombosis in patients with COVID-19: a prothrombotic and inflammatory disease caused by SARS coronavirus-2. Anatolian J Cardiol. 24(4):224–234. https://doi.org/10.14744/AnatolJCardiol.2020.56727.
- Patterson BK, Guevara-Coto J, Yogendra R, Francisco EB, Long E, Pise A, Rodrigues H, Parikh P, Mora J, Mora-Rodríguez RA. 2021. Immune-based prediction of COVID-19 severity and chronicity decoded using machine learning. Front Immunol. 12:700782. https://doi.org/10.3389/fimmu.2021.700782.
- Peckham H, de Gruijter NM, Raine C, Radziszewska A, Ciurtin C, Wedderburn LR, Rosser EC, Webb K, Deakin CT. 2020. Male sex identified by global COVID-19 meta-analysis as a risk factor for death and ITU admission. Nat Commun. 11:6317. https://doi.org/10.1038/s41467-020-19741-6.
- Pérez-Gómez B, Pastor-Barriuso R, Pérez-Olmeda M, Hernán MA, Oteo-Iglesias J, Fernández de Larrea N, Fernández-García A, Martín M, Fernández-Navarro P, Cruz I, et al. 2021. ENE-COVID nationwide serosurvey served to characterize asymptomatic infections and to develop a symptom-based risk score to predict COVID-19. J Clin Epidemiol. https://doi.org/10.1016/j.jclinepi.2021.06.005.
- Pfizer initiates Phase 1 study of novel oral antiviral therapeutic agent against SARS-CoV-2. 2021. [accessed 2021 Aug 17]. https://www.pfizer.com/news/press-release/press-release-detail/pfizer-initiates-phase-1-study-novel-oral-antiviral.
- Piccoli L, Park Y-J, Alejandra Tortorici M, Czudnochowski N, Walls AC, Beltramello M, Silacci-Fregni C, Pinto D, Rosen LE, Bowen JE, et al. 2020. Mapping neutralizing and immunodominant sites on the SARS-CoV-2 spike receptor-binding domain by structure-guided high-resolution serology. Cell. 183(4):1024–1042.e21. https://doi.org/10.1016/j.cell.2020.09.037.
- Pierce CA, Preston-Hurlburt P, Dai Y, Aschner CB, Cheshenko N, Galen B, Garforth SJ, Herrera NG, Jangra RK, Morano NC, et al. 2020, Oct 7. Immune responses to SARS-CoV-2 infection in hospitalized pediatric and adult patients. Sci Transl Med. 12(564):eabd5487. https://doi.org/10.1126/scitranslmed.abd5487. Epub 2020 Sep 21. PMID: 32958614; PMCID: PMC7658796.
- Pietrobon AJ, Teixeira FME, Sato MN. 2020. Immunosenescence and inflammaging: risk factors of severe COVID-19 in older people. Front Immunol. 11:579220. https://doi.org/10.3389/fimmu.2020.579220.
- Pinna G. 2021. Sex and COVID-19: a protective role for reproductive steroids. Trends Endocrinol Metab. 32:3–6. https://doi.org/10.1016/j.tem.2020.11.004.
- Plante JA, Liu Y, Liu J, Xia H, Johnson BA, Lokugamage KG, Zhang X, Muruato AE, Zou J, Fontes-Garfias CR, et al. 2020. Spike mutation D614G alters SARS-CoV-2 fitness. Nature. https://doi.org/10.1038/s41586-020-2895-3.
- Polack FP, Thomas SJ, Kitchin N, Absalon J, Gurtman A, Lockhart S, Perez JL, Pérez Marc G, Moreira ED, Zerbini C, et al. 2020. Safety and efficacy of the BNT162b2 mRNA covid-19 vaccine. N Engl J Med. 383:2603–2615. https://doi.org/10.1056/NEJMoa2034577.
- Portmann-Baracco A, Bryce-Alberti M, Accinelli RA. 2020. Propiedades antivirales y antiinflamatorias de ivermectina y su potencial uso en COVID-19. Archivos de Bronconeumología. 56:831. https://doi.org/10.1016/j.arbres.2020.06.011.
- Pottegård A, Lund LC, Karlstad Ø, Dahl J, Andersen M, Hallas J, Lidegaard Ø, Tapia C, Gulseth HL, Ruiz PLD, et al. 2021. Arterial events, venous thromboembolism, thrombocytopenia, and bleeding after vaccination with Oxford-AstraZeneca ChAdOx1-S in Denmark and Norway: population based cohort study. Br Med J. 373:n1114. https://doi.org/10.1136/bmj.n1114.
- Prado T, Fumian TM, Mannarino CF, Resende PC, Motta FC, Eppinghaus ALF, Chagas do Vale VH, Braz RMS, Andrade JSR, Maranhão AG, et al. 2021. Wastewater-based epidemiology as a useful tool to track SARS-CoV-2 and support public health policies at municipal level in Brazil. Water Res. https://doi.org/10.1016/j.watres.2021.116810.
- Prokunina-Olsson L, Alphonse N, Dickenson RE, Durbin JE, Glenn JS, Hartmann R, Kotenko SV, Lazear HM, O’Brien TR, Odendall C, et al. 2020, May 4. COVID-19 and emerging viral infections: the case for interferon lambda. J Exp Med. 217(5):e20200653. https://doi.org/10.1084/jem.20200653.
- Pruijssers AJ, Denison MR. 2019. Nucleoside analogues for the treatment of coronavirus infections. Curr Opin Virol. 35:57–62. https://doi.org/10.1016/j.coviro.2019.04.002.
- PTC Therapeutics Announces the Initiation of a Phase 2/3 Clinical Trial to Evaluate PTC299 for the Treatment of COVID-19. June 17, 2020. https://ir.ptcbio.com/node/12966/pdf.
- Rahman MT, Idid SZ. 2021. Can Zn be a critical element in COVID-19 treatment? Biol Trace Elem Res. 199:550–558. https://doi.org/10.1007/s12011-020-02194-9.
- Ramaiah MJ. 2020. mTOR inhibition and p53 activation, microRNAs: The possible therapy against pandemic COVID-19, Gene Reports, 20. https://doi.org/10.1016/j.genrep.2020.100765.
- Rambaut A, Loman N, Pybus O, Barclay W, Barrett J, Carabelli A, Connor T, Peacock T, Robertson DL, Volz E. 2021. On behalf of COVID-19 Genomics Consortium UK (CoG-UK). Preliminary genomic characterisation of an emergent SARS-CoV-2 lineage in the UK defined by a novel set of spike mutations. Virological. [accessed 2021 Aug 17]. https://virological.org/t/preliminary-genomic-characterisation-of-an-emergent-sars-cov-2-lineage-in-the-uk-defined-by-a-novel-set-of-spike-mutations/563.
- Rao M, Gershon M. 2016. The bowel and beyond: the enteric nervous system in neurological disorders. Nat Rev Gastroenterol Hepatol. 13:517–528. https://doi.org/10.1038/nrgastro.2016.107.
- Rappazzo CG, Tse LV, Kaku CI, Wrapp D, Sakharkar M, Huang D, Deveau LM, Yockachonis TJ, Herbert AS, Battles MB, et al. 2021, Jan 25. Broad and potent activity against SARS-like viruses by an engineered human monoclonal antibody. Science. eabf4830. https://doi.org/10.1126/science.abf4830. Epub ahead of print. PMID: 33495307.
- Rausch JW, Capoferri AA, Katusiime MG, Patro SC, Kearney MF. 2020, Oct. Low genetic diversity may be an achilles heel of SARS-CoV-2. Proc Natl Acad Sci USA. 117(40):24614–24616. https://doi.org/10.1073/pnas.2017726117.
- REACT working group. 2021. Association between administration of IL-6 antagonists and mortality among patients hospitalized for COVID-19. A meta-analysis. JAMA. https://doi.org/10.1001/jama.2021.11330.
- Reche PA. 2020. Potential cross-reactive immunity to SARS-CoV-2 from common human pathogens and vaccines. Front Immunol. 11:586984. https://doi.org/10.3389/fimmu.2020.586984.
- RECOVERY Collaborative Group. 2021b. Tocilizumab in patients admitted to hospital with COVID-19 (RECOVERY): a randomised, controlled, open-label, platform trial. Lancet. https://doi.org/10.1016/S0140-6736(21)00676-0.
- Rehman M, Tauseef I, Aalia B, Shah SH, Junaid M, Haleem KS. 2020. Therapeutic and vaccine strategies against SARS-CoV-2: past, present and future. Future Virol. https://doi.org/10.2217/fvl-2020-0137.
- Reiter RJ, Abreu-Gonzalez P, Marik PE, Dominguez-Rodriguez A. 2020a. Therapeutic algorithm for use of melatonin in patients With COVID-19. Front Med (Lausanne). 7:226. https://doi.org/10.3389/fmed.2020.00226.
- Reiter RJ, Sharma R, Ma Q, Dominquez-Rodriguez A, Marik PE, Abreu-Gonzalez P. 2020b. Melatonin inhibits COVID-19-induced cytokine storm by reversing aerobic glycolysis in immune cells: a mechanistic analysis. Med Drug Discovery. 6:100044. https://doi.org/10.1016/j.medidd.2020.100044.
- Resende PC, Bezerra JF, de Vasconcelos RHT, Arantes I, Appolinario L, Mendonça AC, Paixao AC, Rodrigues ACD, Silva T, Rocha AS, et al.. 2021. Spike E484 K mutation in the first SARS-CoV-2 reinfection case confirmed in Brazil. Virological. [accessed 2021 Aug 17]. https://virological.org/t/spike-e484k-mutation-in-the-first-sars-cov-2-reinfection-case-confirmed-in-brazil-2020/584.
- Rhea EM, Logsdon AF, Hansen KM, Williams LM, Reed MJ, Baumann KK, Holden SJ, Raber J, Banks WA, Erickson MA. 2020. The S1 protein of SARS-CoV-2 crosses the blood–brain barrier in mice. Nat Neurosci. https://doi.org/10.1038/s41593-020-00771-8.
- Robson F, Khan KS, Le TK, Paris C, Demirbag S, Barfuss P, Rocchi P, Ng W-L, et al. 2020. Coronavirus RNA proofreading: molecular basis and therapeutic targeting. Mol Cell. 79:710–727. https://doi.org/10.1016/j.molcel.2020.07.027.
- Romano M, Ruggiero A, Squeglia F, Maga G, Berisio R. 2020. A structural view of SARS-CoV-2 RNA replication machinery: RNA synthesis, proofreading and final capping. Cells. 9:1267. https://doi.org/10.3390/cells9051267.
- Root-Bernstein R. 2020. Age and location in severity of COVID-19 pathology: Do lactoferrin and pneumococcal vaccination explain Low infant mortality and regional differences? BioEssays. 42:2000076. https://doi.org/10.1002/bies.202000076.
- Roy-Vallejo E, Purificación AS, Torres JD, Sánchez Moreno B, Arnalich F, García Blanco MJ, López Miranda J, Romero-Cabrera JL, Herrero Gil CR, Bascunana J, et al. 2021. Angiotensin-converting enzyme inhibitors and angiotensin receptor blockers withdrawal is associated with higher mortality in hospitalized patients with COVID-19. J Clin Med. 10(12):2642. https://doi.org/10.3390/jcm10122642.
- Rubio-Rivas M, Corbella X, Mora-Luján JM, Loureiro-Amigo J, López Sampalo A, Yera Bergua C, Esteve Atiénzar PJ, Díez García LF, Gonzalez Ferrer R, Plaza Canteli S, et al. 2020. Predicting clinical outcome with phenotypic clusters in COVID-19 pneumonia: an analysis of 12,066 hospitalized patients from the Spanish Registry SEMI-COVID-19. J Clin Med. 9:3488. https://doi.org/10.3390/jcm9113488.
- Sabino EC, Buss LF, Carvalho MPS, Prete CA, Crispim MAE, Fraiji NA, Pereira RHM, Parag KV, da Silva Peixoto P, Kraemer MUG, et al. 2021. Resurgence of COVID-19 in manaus, Brazil, despite high seroprevalence. Lancet. https://doi.org/10.1016/S0140-6736(21)00183-5.
- Sacramento CQ, Fintelman-Rodrigues N, Temerozo JR, de Paula Dias Da Silva A, da Silva Gomes Dias S, dos Santos da Silva C, Ferreira Ferreira, Mattos M, Pão CRR, de Freitas CS, et al. 2020. The in vitro antiviral activity of the anti-hepatitis C virus (HCV) drugs daclatasvir and sofosbuvir against SARS-CoV-2. bioRxiv. https://doi.org/10.1101/2020.06.15.153411.
- Sadoff J, Le Gars M, Shukarev G, Heerwegh D, Truyers C, de Groot AM, Stoop J, Tete S, Van Damme W, Leroux-Roels I, et al. 2021. Interim results of a phase 1–2a trial of Ad26.COV2.S covid-19 vaccine. N Engl J Med. https://doi.org/10.1056/NEJMoa2034201.
- Sadria M, Layton AT, Beard DA. 2020. Use of angiotensin-converting enzyme inhibitors and angiotensin II receptor blockers during the COVID-19 pandemic: a modeling analysis. PLoS Comput Biol. 16:e1008235. https://doi.org/10.1371/journal.pcbi.1008235.
- Safety and the Efficacy of MesenCure for the Treatment of Pulmonary Manifestations of COVID-19. ClinicalTrials.gov Identifier: NCT04716998. Last Update Posted: 2021, January 22. https://clinicaltrials.gov/ct2/show/NCT04716998
- Safety Infusion of NatuRal KillEr celLs or MEmory T Cells as Adoptive Therapy in COVID-19 pnEumonia or Lymphopenia (RELEASE). ClinicalTrials.gov Identifier: NCT04578210. Last Update Posted: 2020, October 19. https://clinicaltrials.gov/ct2/show/NCT04578210.
- Sagar M, Reifler K, Rossi M, Miller NS, Sinha P, White LF, Mizgerd JP. 2021. Recent endemic coronavirus infection is associated with less-severe COVID-19. J Clin Invest. 131(1):e143380. https://doi.org/10.1172/JCI143380.
- Salem ML, El-Hennawy D. 2020. The possible beneficial adjuvant effect of influenza vaccine to minimize the severity of COVID-19. Med Hypotheses. 140:109752. https://doi.org/10.1016/j.mehy.2020.109752.
- Salonia A, Pontillo M, Capogrosso P, Gregori S, Tassara M, Boeri L, Carenzi C, Abbate C, Cignoli D, Ferrara AM, et al. 2021, Feb 26. Severely low testosterone in males with COVID-19: a case-control study. Andrology. https://doi.org/10.1111/andr.12993.
- Sama IE, Ravera A, Santema BT, van Goor H, ter Maaten JM, Cleland JGF, Rienstra M, Friedrich AW, Samani NJ, Ng LL, et al. 2020, May 14. Circulating plasma concentrations of angiotensin-converting enzyme 2 in men and women with heart failure and effects of renin–angiotensin–aldosterone inhibitors. Eur Heart J. 41(19):1810–1817. https://doi.org/10.1093/eurheartj/ehaa373.
- Sánchez-Guijo F, García-Arranz M, López-Parra M, Monedero P, Mata-Martínez C, Santos A, Sagredo V, Álvarez-Avello JM, Guerrero JE, Pérez-Calvo C, et al. 2020. Adipose-derived mesenchymal stromal cells for the treatment of patients with severe SARS-CoV-2 pneumonia requiring mechanical ventilation. A proof of concept study. EClinicalMedicine. 25:100454. https://doi.org/10.1016/j.eclinm.2020.100454.
- Sanchez-Trincado JL, Gomez-Perosanz M, Reche PA. 2017. Fundamentals and methods for T- and B-cell epitope prediction. J Immunol Res. 2017:1–14. Article ID 2680160, https://doi.org/10.1155/2017/2680160.
- Sanchez-Vazquez R, Guio-Carrion A, Zapatero-Gaviria A, Martinez P, Blasco MA. 2021. Shorter telomere lengths in patients with severe COVID-19 disease. Aging (Albany NY). 13:1–15. https://doi.org/10.18632/aging.202463.
- Sang P, Tian S-H, Meng Z-H, Yang L-Q. 2020. Anti-HIV drug repurposing against SARS-CoV-2. RSC Adv. 27. https://doi.org/10.1039/D0RA01899F.
- Santos JC, Passos GA. 2021. The high infectivity of SARS-CoV-2 B.1.1.7 is associated with increased interaction force between spike-ACE2 caused by the viral N501Y mutation. bioRxiv. https://doi.org/10.1101/2020.12.29.424708.
- SARS-CoV-2 Variant Classifications and Definitions. 2021. Center for Disease Control and Prevention, CDC. [accessed 2021 Aug 17]. https://www.cdc.gov/coronavirus/2019-ncov/variants/variant-info.html.
- SARS-CoV-2 variants dashboard. 2021. European Center for Disease Prevention and Control, eCDC. [accessed 2021 Aug 17]. Available at: https://www.ecdc.europa.eu/en/covid-19/situation-updates/variants-dashboard.
- Schirinzi T, Landi D, Liguori C. 2020. COVID-19: dealing with a potential risk factor for chronic neurological disorders. J Neurol. https://doi.org/10.1007/s00415-020-10131-y.
- Schmitz A, Weber A, Bayin M, Breuers S, Fieberg V, Famulok M, Mayer G. 2021. A SARS-CoV-2 spike binding DNA aptamer that inhibits pseudovirus infection by an RBD-independent mechanism. Angew Chem, Int Ed. 60:10279–10285. https://doi.org/10.1002/anie.202100316.
- Schoggins JW, Rice CM. 2011. Interferon-stimulated genes and their antiviral effector functions. Curr Opin Virol. 1:519–525. https://doi.org/10.1016/j.coviro.2011.10.008.
- Schreiber KH, O’Leary MN, Kennedy BK. 2016. Chapter 2 – The mTOR pathway and aging. In: Matt R. Kaeberlein, George M. Martin, editor. Handbook of the biology of aging. 8th ed. Academic Press; https://doi.org/10.1016/B978-0-12-411596-5.00002-2.
- Schroeder M, Schaumburg B, Müller Z, Parplys A, Jarczak D, Nierhaus A, Kloetgen A, Schneider B, Peschka M, Stoll F, et al. 2020. Sex hormone and metabolic dysregulations are associated with critical illness in male covid-19 patients. medRxiv. https://doi.org/10.1101/2020.05.07.20073817.
- Schultze A, Walker AJ, MacKenna B, Morton CE, Bhaskaran K, Brown JP, Rentsch CT, Williamson E, Drysdale H, Croker R, et al. 2020. Risk of COVID-19-related death among patients with chronic obstructive pulmonary disease or asthma prescribed inhaled corticosteroids: an observational cohort study using the OpenSAFELY platform. Lancet Respir Med. 8(11):1106–1120. https://doi.org/10.1016/S2213-2600(20)30415-X.
- Scientific Brief: SARS-CoV-2 and Potential Airborne Transmission. Updated 2020, Oct. 5. https://www.cdc.gov/coronavirus/2019-ncov/more/scientific-brief-sars-cov-2.html
- Scozzi D, Cano M, Ma L, Zhou D, Zhu JH, O’Halloran JA, Goss C, Rauseo AM, Liu Z, Sahu SK, et al. 2021. Circulating mitochondrial DNA is an early indicator of severe illness and mortality from COVID-19. JCI Insight. https://doi.org/10.1172/jci.insight.143299.
- Sekine T, Perez-Potti A, Rivera-Ballesteros O, Strålin K, Gorin JB, Olsson A, Llewellyn-Lacey S, Kamal H, Bogdanovic G, Muschiol S, et al. 2020. Robust T cell immunity in convalescent individuals with asymptomatic or mild COVID-19. Cell. 183:158–168.e14. https://doi.org/10.1016/j.cell.2020.08.017.
- Shang J, Wan Y, Luo C, Ye G, Geng Q, Auerbach A, Li F, et al. 2020, May. Cell entry mechanisms of SARS-CoV-2. Proc Natl Acad Sci USA. 117(21):11727–11734. http://doi.org/10.1073/pnas.2003138117.
- Sharma S, Thomas PG. 2014. The two faces of heterologous immunity: protection or immunopathology. J Leukocyte Biol. 95:405–416. https://doi.org/10.1189/jlb.0713386.
- Shen B, Yi X, Sun Y, Bi X, Du J, Zhang C, Quan S, Zhang F, Sun R, Qian L, et al. 2020. Proteomic and metabolomic characterization of COVID-19 patient sera. Cell. 182(1):59–72.e15. https://doi.org/10.1016/j.cell.2020.05.032.
- Sheybani Z, Dokoohaki MH, Negahdaripour M, Dehdashti M, Zolghadr H, Moghadami M, Masoompour SM, Zolghadr AR. 2020. The role of folic acid in the management of respiratory disease caused by COVID-19. ChemRxiv. https://doi.org/10.26434/chemrxiv.12034980.v1.
- Shibata S, Arima H, Asayama K, Hoshide S, Ichihara A, Ishimitsu T, Kario K, Kishi T, Mogi M, Nishiyama A, et al. 2020. Hypertension and related diseases in the era of COVID-19: a report from the Japanese society of hypertension task force on COVID-19. Hypertens Res. 43:1028–1046. https://doi.org/10.1038/s41440-020-0515-0.
- Shirvaliloo M. 2021. Epigenomics in COVID-19; the link between DNA methylation, histone modifications and SARS-CoV-2 infection. Epigenomics. https://doi.org/10.2217/epi-2021-0057.
- Silva J, Lucas C, Sundaram M, Israelow B, Wong P, Klein J, Tokuyama M, Lu P, Venkataraman A, Liu F, et al. 2021. Saliva viral load is a dynamic unifying correlate of COVID-19 severity and mortality. medRxiv. https://doi.org/10.1101/2021.01.04.21249236.
- Sinagra E, Utzeri E, Morreale GC, Fabbri C, Pace F, Anderloni A. 2020. Microbiota-gut-brain axis and its affect inflammatory bowel disease: pathophysiological concepts and insights for clinicians. World J Clin Cases. 8(6):1013–1025. https://doi.org/10.12998/wjcc.v8.i6.1013.
- Smith SJ, Busby J, Heaney LG, Pfeffer PE, Jackson DJ, Yang F, Fowler SJ, Menzies-Gow A, Idris E, Brown T, et al. 2020. The impact of the first COVID19 surge on severe asthma patients in the UK. which is worse: the virus or the lockdown? ERJ Open Res. https://doi.org/10.1183/23120541.00768-2020.
- Smith TP, Flaxman S, Gallinat AS, Kinosian SP, Stemkovski M, Unwin HJT, Watson OJ, Whittaker C, Cattarino L, Dorigatti I, et al. 2021, June. Temperature and population density influence SARS-CoV-2 transmission in the absence of nonpharmaceutical interventions. Proc Natl Acad Sci USA. 118(25):e2019284118. https://doi.org/10.1073/pnas.2019284118.
- Solanich X, Vargas-Parra G, van der Made CI, Simons A, Schuurs-Hoeijmakers J, Antolí A, del Valle J, Rocamora-Blanch G, Setién F, Esteller M, et al. 2021. Genetic screening for TLR7 variants in young and previously healthy men with severe COVID-19. Front Immunol. 12:719115. https://doi.org/10.3389/fimmu.2021.719115.
- Song E, Zhang C, Israelow B, Lu-Culligan A, Prado AV, Skriabine S, Lu P, Weizman OE, Liu F, Dai Y, et al. 2021, March 1. Neuroinvasion of SARS-CoV-2 in human and mouse brain. J Exp Med. 218(3):e20202135. https://doi.org/10.1084/jem.20202135.
- Stanford University. 2021. SARS-COV-2 antiviral therapy. [accessed 2021 Aug 17]. https://covdb.stanford.edu/page/covid-review/.
- Starr TN, Greaney AJ, Addetia A, Hannon WW, Choudhary MC, Dingens AS, Li JZ, Bloom JD. 2021, Jan 25. Prospective mapping of viral mutations that escape antibodies used to treat COVID-19. Science. eabf9302. https://doi.org/10.1126/science.abf9302. Epub ahead of print. PMID: 33495308.
- Statkute E, Rubina A, O’Donnell VB, Thomas DW, Stanton RJ. 2020. Brief report: the virucidal efficacy of oral rinse components against SARS-CoV-2 in vitro. bioRxiv. https://doi.org/10.1101/2020.11.13.381079.
- Stephenson E, Reynolds G, Botting RA, Calero-Nieto FJ, Morgan MD, Tuong ZK, Bach K, Sungnak W, Worlock KB, Yoshida M, et al. 2021. Single-cell multi-omics analysis of the immune response in COVID-19. Nat Med. https://doi.org/10.1038/s41591-021-01329-2.
- Sterlin D, Mathian A, Miyara M, Mohr A, Anna F, Claër L, Quentric P, Fadlallah J, Devilliers H, Ghillani P, et al. 2021, Jan 20. Iga dominates the early neutralizing antibody response to SARS-CoV-2. Sci Transl Med. 13(577):eabd2223. https://doi.org/10.1126/scitranslmed.abd2223. Epub 2020 Dec 7. PMID: 33288662.
- Sungnak W, Huang N, Bécavin C, Berg M, Queen R, Litvinukova M, Talavera-López C, Maatz H, Reichart D, Sampaziotis F, et al. 2020. SARS-CoV-2 entry factors are highly expressed in nasal epithelial cells together with innate immune genes. Nat Med. 26:681–687. https://doi.org/10.1038/s41591-020-0868-6.
- Taboada M, Caruezo V, Naveira A, Atanassoff PG. 2020. Corticosteroids and the hyper-inflammatory phase of the COVID-19 disease. J Clin Anesth. 66:109926. https://doi.org/10.1016/j.jclinane.2020.109926.
- Takahashi T, Ellingson MK, Wong P, Israelow B, Lucas C, Klein J, Silva J, Mao T, Oh JE, Tokuyama M, et al. 2020. Sex differences in immune responses that underlie COVID-19 disease outcomes. Nature. 588:315–320. https://doi.org/10.1038/s41586-020-2700-3.
- Tan L, Wang Q, Zhang D, Ding J, Huang Q, Tang Y-Q, Wang Q, Miao H. 2020. Lymphopenia predicts disease severity of COVID-19: a descriptive and predictive study. Signal Transduction Targeted Ther. 5:33. https://doi.org/10.1038/s41392-020-0148-4.
- Tang D, Comish P, Kang R, Hobman TC. 2020a. The hallmarks of COVID-19 disease. PLOS Pathogens. 16(5):e1008536. http://dx.doi.org/10.1371/journal.ppat.1008536.
- Tang N, Li D, Wang X, Sun Z. 2020b. Abnormal coagulation parameters are associated with poor prognosis in patients with novel coronavirus pneumonia. J Thromb Haemostasis. 18:844–847. https://doi.org/10.1111/jth.14768.
- Tardif J-C, Bouabdallaoui N, L'Allier PL, Gaudet D, Shah B, Pillinger MH, Lopez-Sendon J, da Luz P, Verret L, Audet S, et al. 2021. Efficacy of colchicine in Non-hospitalized patients with COVID-19. medRxiv. https://doi.org/10.1101/2021.01.26.21250494.
- Tarke A, Sidney J, Kidd CK, Dan JM, Ramirez SI, Yu ED, Mateus J, da Silva Antunes R, Moore E, Rubiro P, et al. 2021a. Comprehensive analysis of T cell immunodominance and immunoprevalence of SARS-CoV-2 epitopes in COVID-19 cases. Cell Reports Med. 2(2):100204. https://doi.org/10.1016/j.xcrm.2021.100204.
- Tarke A, Sidney J, Methot N, Yu ED, Zhang Y, Dan JM, Goodwin B, Rubiro P, Sutherland A, Wang E, et al. 2021b. Impact of SARS-CoV-2 variants on the total CD4+ and CD8+ T cell reactivity in infected or vaccinated individuals. Cell Reports Med. https://doi.org/10.1016/j.xcrm.2021.100355.
- Taylor BL, Waghray A, Mbano IM, Miao VN, Tzouanas CN, Cao Y, Yousif AS, Bals J, Hauser BM, Feldman J, et al. 2020. SARS-CoV-2 receptor ACE2 is an interferon-stimulated gene in human airway epithelial cells and Is detected in specific cell subsets across tissues. Cell. 181. https://doi.org/10.1016/j.cell.2020.04.035.
- Taylor EH, Marson EJ, Elhadi M, Macleod KDM, Yu YC, Davids R, Boden R, Overmeyer RC, Ramakrishnan R, Thomson DA, et al. 2021. Factors associated with mortality in patients with COVID-19 admitted to intensive care: a systematic review and meta-analysis. Anaesthesia. https://doi.org/10.1111/anae.15532.
- Tegally H, Wilkinson E, Giovanetti M, Iranzadeh A, Fonseca V, Giandhari J, Doolabh D, Pillay S, San EJ, Msomi N, et al. 2020. Emergence and rapid spread of a new severe acute respiratory syndrome-related coronavirus 2 (SARS-CoV-2) lineage with multiple spike mutations in South Africa. medRxiv. https://doi.org/10.1101/2020.12.21.20248640.
- Testar J. 2021. Cytokines: Introduction. UK: Imperial College London. [accessed 2021 Aug 17]. https://www.immunology.org/public-information/bitesized-immunology/receptors-and-molecules/cytokines-introduction.
- The COVID-19 Sex-Disaggregated Data Tracker. 2021. [accessed 2021 Aug 17]. https://globalhealth5050.org/the-sex-gender-and-covid-19-project/.
- The human protein atlas. 2021. ACE2. [accessed 2021 Aug 17]. https://www.proteinatlas.org/ENSG00000130234-ACE2/tissue.
- Thépaut M, Luczkowiak J, Vivès C, Labiod N, Bally I, Lasala F, Grimoire Y, Fenel D, Sattin S, Thielens N, et al. 2021. DC/L-SIGN recognition of spike glycoprotein promotes SARS-CoV-2 trans-infection and can be inhibited by a glycomimetic antagonist. PLoS Pathog. 17(5):e1009576. https://doi.org/10.1371/journal.ppat.1009576.
- The Severe Covid-19 GWAS Group. 2020. Genomewide association study of severe covid-19 with respiratory failure. N Engl J Med. 383:1522–1534. https://doi.org/10.1056/NEJMoa2020283.
- Thomas RL, Jiang L, Adams JS, Xu ZZ, Shen J, Janssen S, Ackermann G, Vanderschueren D, Pauwels S, Knight R, et al. 2020. Vitamin D metabolites and the gut microbiome in older men. Nat Commun. 11:5997. https://doi.org/10.1038/s41467-020-19793-8.
- Thomson EC, Rosen LE, Shepherd JG, Spreafico R, da Silva Filipe A, Wojcechowskyj JA, Davis C, Piccoli L, Pascall DJ, Dillen J, et al. 2021. Circulating SARS-CoV-2 spike N439 K variants maintain fitness while evading antibody-mediated immunity. Cell. https://doi.org/10.1016/j.cell.2021.01.037.
- Tizabi Y, Getachew B, Copeland RL, Aschner M. 2020. Nicotine and the nicotinic cholinergic system in COVID-19. FEBS J. 287:3656–3663. https://doi.org/10.1111/febs.15521.
- Tomaszewski T, DeVries RS, Dong M, Bhatia G, Norsworthy MD, Zheng X, Caetano-Anollés G. 2020, Oct 23. New pathways of mutational change in SARS-CoV-2 proteomes involve regions of intrinsic disorder Important for virus replication and release. Evol Bioinform Online. 16:1176934320965149. https://doi.org/10.1177/1176934320965149. PMID: 33149541; PMCID: PMC7586267.
- Torres Acosta MA, Singer BD. 2020, Sep 24. Pathogenesis of COVID-19-induced ARDS: implications for an ageing population. Eur Respir J. 56(3):2002049. https://doi.org/10.1183/13993003.02049-2020. PMID: 32747391; PMCID: PMC7397945.
- Tortora G, Funke B, Case C. 2019. Microbiology: An introduction, 13th. Pearson. ISBN: 9780134709260.
- Tosif S, Neeland MR, Sutton P, Licciardi PV, Sarkar S, Selva KJ, Do LAH, Donato C, Quan Toh Z, Higgins R, et al. 2020. Immune responses to SARS-CoV-2 in three children of parents with symptomatic COVID-19. Nat Commun. 11:5703. https://doi.org/10.1038/s41467-020-19545-8.
- Turner JS, Kim W, Kalaidina E, Goss CW, Rauseo AM, Schmitz AJ, Hansen L, Haile A, Klebert MK, Pusic I, et al. 2021a. SARS-CoV-2 infection induces long-lived bone marrow plasma cells in humans. Nature. https://doi.org/10.1038/s41586-021-03647-4.
- Turner JS, O’Halloran JA, Kalaidina E, Kim W, Schmitz AJ, Zhou JQ, Lei T, Thapa M, Chen RE, Case JB, et al. 2021b. SARS-CoV-2 mRNA vaccines induce persistent human germinal centre responses. Nature. https://doi.org/10.1038/s41586-021-03738-2.
- Uludağ H, Parent K, Aliabadi HM, Haddadi A. 2020. Prospects for RNAi therapy of COVID-19. Front Bioeng Biotechnol. 8:916. https://doi.org/10.3389/fbioe.2020.00916.
- van der Made CI, Simons A, Schuurs-Hoeijmakers J, van den Heuvel G, Mantere T, Kersten S, van Deuren RC, Steehouwer M, van Reijmersdal SV, Jaeger M, et al. 2020. Presence of genetic variants among young Men With severe COVID-19. JAMA. 324(7):663–673. https://doi.org/10.1001/jama.2020.13719.
- Varga Z, Flammer AJ, Steiger P, Haberecker M, Andermatt R, Zinkernagel AS, Mehra MR, Schuepbach RA, Ruschitzka F, Moch H. 2020. Endothelial cell infection and endotheliitis in COVID-19. Lancet. 395:1417–1418. https://doi.org/10.1016/S0140-6736(20)30937-5.
- Volz E, Hill V, McCrone JT, Price A, Jorgensen D, O’Toole A, Southgate J, Johnson R, Jackson B, Nascimento FF, et al. 2021. Evaluating the effects of SARS-CoV-2 spike mutation D614G on transmissibility and pathogenicity. Cell. 184(1):64–75.e11. https://doi.org/10.1016/j.cell.2020.11.020.
- Volz E, Mishra S, Chand M, Barrett JC, Johnson R, Geidelberg L, Hinsley WR, Laydon DJ, Dabrera G, O’Toole A, et al. 2020. The COVID-19 genomics UK (COG-UK) consortium, Seth Flaxman, Oliver Ratmann, Samir Bhatt, Susan Hopkins, Axel Gandy, Andrew Rambaut, Neil M Ferguson. medRxiv. https://doi.org/10.1101/2020.12.30.20249034.
- Voysey M, Clemens SAC, Madhi SA, Weckx LY, Folegatti PM, Aley PK, Angus B, Baillie VL, Barnabas SL, Bhorat QE, et al. 2021. Safety and efficacy of the ChAdOx1 nCoV-19 vaccine (AZD1222) against SARS-CoV-2: an interim analysis of four randomised controlled trials in Brazil, South Africa, and the UK. Lancet J. 397:0. https://doi.org/10.1016/S0140-6736(20)32661-1.
- Wahl A, Gralinski LE, Johnson CE, Yao W, Kovarova M, Dinnon KH, Liu H, Madden VJ, Krzystek HM, De C, et al. 2021. SARS-CoV-2 infection is effectively treated and prevented by EIDD-2801. Nature. https://doi.org/10.1038/s41586-021-03312-w.
- Wajnberg A, Amanat F, Firpo A, Altman DR, Bailey MJ, Mansour M, McMahon M, Meade P, Mendu DR, Muellers K, et al. 2020. Robust neutralizing antibodies to SARS-CoV-2 infection persist for months. Science. 370(6521):1227–1230. https://doi.org/10.1126/science.abd7728.
- Wang C, Li W, Drabek D, Okba NMA, van Haperen R, Osterhaus ADME, van Kuppeveld FJM, Haagmans BL, Grosveld F, Bosch BJ. 2020. A human monoclonal antibody blocking SARS-CoV-2 infection. Nat Commun. 11:2251. https://doi.org/10.1038/s41467-020-16256-y.
- Wang P, Nair MS, Liu L, Iketani S, Luo Y, Guo Y, Wang M, Yu J, Zhang B, Kwong PD, et al. 2021a. Antibody resistance of SARS-CoV-2 variants B.1.351 and B.1.1.7. Nature. 593(7857):130–135. http://dx.doi.org/10.1038/s41586-021-03398-2.
- Wang S, Qiu Z, Hou Y, Deng X, Xu W, Zheng T, Wu P, Xie S, Bian W, Zhang C, et al. 2021b. AXL is a candidate receptor for SARS-CoV-2 that promotes infection of pulmonary and bronchial epithelial cells. Cell Research. 31(2):126–140. http://dx.doi.org/10.1038/s41422-020-00460-y.
- Wang Y, Yang C, Song Y, Coleman JR, Stawowczyk M, Tafrova J, Tasker S, Boltz D, Baker R, Garcia L, et al. 2021c. Scalable live-attenuated SARS-CoV-2 vaccine candidate demonstrates preclinical safety and efficacy. Proc Nat Acad Sci (PNAS). 118(29):e2102775118. https://doi.org/10.1073/pnas.2102775118.
- Ward SE, Fogarty H, Karampini E, Lavin M, Lavin M, Schneppenheim S, Dittmer R, Morrin H, Glavey S, Ni Cheallaigh C, et al. 2021. ADAMTS13 regulation of VWF multimer distribution in severe COVID-19. J Thromb Haemostasis. https://doi.org/10.1111/jth.15409.
- Wark PA, Pathinayake PS, Kaiko G, Nichol K, Ali A, Chen L, Sutanto EN, Garratt LW, Sohal SS, Lu W, et al. 2020. ACE2 expression is elevated in Airway Epithelial Cells from aged and male donors but reduced in asthma. medRxiv. https://doi.org/10.1101/2020.07.26.20162248.
- Wei J, Alfajaro MM, DeWeirdt PC, Hanna RE, Lu-Culligan WJ, Cai WL, Strine MS, Zhang SM, Graziano VR, Schmitz CO, et al. 2021. Genome-wide CRISPR screens reveal host factors critical for SARS-CoV-2 infection. Cell. 184(1):76–91.e13. https://doi.org/10.1016/j.cell.2020.10.028.
- Weisberg SP, Connors TJ, Zhu Y, Baldwin MR, Lin WH, Wontakal S, Szabo PA, Wells SB, Dogra P, Gray J, et al. 2021. Distinct antibody responses to SARS-CoV-2 in children and adults across the COVID-19 clinical spectrum. Nat Immunol. 22:25–31. https://doi.org/10.1038/s41590-020-00826-9.
- Wesley Long S, Olsen RJ, Christensen PA, Bernard DW, Davis JJ, Shukla M, Nguyen M, Saavedra MO, Yerramilli P, Pruitt L, et al. 2020, Oct. Molecular architecture of early dissemination and massive second wave of the SARS-CoV-2 virus in a major metropolitan area. mBio. 11(6):e02707–20. https://doi.org/10.1128/mBio.02707-20.
- Wessels I, Rolles B, Rink L. 2020. The potential impact of zinc supplementation on COVID-19 pathogenesis. Front Immunol. 11:1712. https://doi.org/10.3389/fimmu.2020.01712.
- Westendorf K, Žentelis S, Foster D, Vaillancourt P, Wiggin M, Lovett E, van der Lee R, Hendle J, Pustilnik A, Sauder JM, et al. 2021. LY-CoV1404 potently neutralizes SARS-CoV-2 variants. bioRxiv. https://doi.org/10.1101/2021.04.30.442182.
- White KM, Rosales R, Yildiz S, Kehrer T, Miorin L, Moreno E, Jangra S, Uccellini MB, Rathnasinghe R, Coughlan L, et al. 2021. Plitidepsin has potent preclinical efficacy against SARS-CoV-2 by targeting the host protein eEF1A. Science. https://doi.org/10.1126/Science.Abf4058.
- Woodruff MC, Ramonell RP, Eun-Hyung Lee F, Sanz I. 2020a. Clinically identifiable autoreactivity is common in severe SARS-CoV-2 infection. medRxiv. https://doi.org/10.1101/2020.10.21.20216192.
- Woodruff MC, Ramonell RP, Nguyen DC, Cashman KS, Saini AS, Haddad NS, Ley AM, Kyu S, Howell JC, Ozturk T, et al. 2020b. Extrafollicular B cell responses correlate with neutralizing antibodies and morbidity in COVID-19. Nat Immunol. 21:1506–1516. https://doi.org/10.1038/s41590-020-00814-z.
- Wu C, Liu Y, Yang Y, Zhang P, Zhong W, Wang Y, Wang Q, Xu Y, Li M, Li X, et al. 2020a. Analysis of therapeutic targets for SARS-CoV-2 and discovery of potential drugs by computational methods. Acta Pharmaceutica Sinica. B 10. https://doi.org/10.1016/j.apsb.2020.02.008.
- Wu KJ, Zimmer C, Corum J. Coronavirus Drug and Treatment Tracker. Updated 2021, Feb 2. https://www.nytimes.com/interactive/2020/science/coronavirus-drugs-treatments.html.
- Wu Y, Wang F, Shen C, Peng W, Li D, Zhao C, Li Z, Li S, Bi Y, Yang Y, et al. 2020b. A noncompeting pair of human neutralizing antibodies block COVID-19 virus binding to its receptor ACE2. Science. 368(6496):1274–1278. http://dx.doi.org/10.1126/science.abc2241.
- Wyllie D, Mulchandani R, Jones HE, Trickey A, Taylor-Phillips S, Brooks T, Charlett A, Ades AE, Moore P, Boyes J, et al. 2021. SARS-CoV-2 responsive T cell numbers are associated with protection. from COVID-19: a prospective cohort study in keyworkers. medRxiv. https://doi.org/10.1101/2020.11.02.20222778.
- Xie X, Zou J, Fontes-Garfias CR, et al. 2021. Neutralization of N501Y mutant SARS-CoV-2 by BNT162b2 vaccine-elicited sera. bioRxiv. https://doi.org/10.1101/2021.01.07.425740.
- Xu Y, Jiang H. 2020. Potential treatment of COVID-19 by inhibitors of human dihydroorotate dehydrogenase. Protein Cell. 11:699–702. https://doi.org/10.1007/s13238-020-00769-9.
- Yang AC, Kern F, Losada PM, Agam MR, Maat CA, Schmartz GP, Fehlmann T, Stein JA, Schaum N, Lee DP, et al. 2021. Dysregulation of brain and choroid plexus cell types in severe COVID-19. Nature. https://doi.org/10.1038/s41586-021-03710-0.
- Yao Y, Wang H, Liu Z. 2020. Expression of ACE2 in airways: implication for COVID-19 risk and disease management in patients with chronic inflammatory respiratory diseases. Clin Exp Allergy. 50:1313–1324. https://doi.org/10.1111/cea.13746.
- Young BE, Fong S-W, Chan Y-H, Mak T-M, Ang LW, Anderson DE, Lee CY-P, Amrun SN, Lee B, Goh YS, et al. 2020. Effects of a major deletion in the SARS-CoV-2 genome on the severity of infection and the inflammatory response: an observational cohort study. Lancet. 396(10251):603–611. https://doi.org/10.1016/S0140-6736(20)31757-8.
- Yuan S, Yin X, Meng X, Chan JF-W, Ye Z-W, Riva L, Pache L, Chan CC-Y, Lai P-M, Chan CC-S, et al. 2021. Clofazimine broadly inhibits coronaviruses including SARS-CoV-2. Nature. https://doi.org/10.1038/s41586-021-03431-4.
- Yue J, Qin L, Zhang C, Xie M. 2020. Reply. J Allergy Clin Immunol. 146. https://doi.org/10.1016/j.jaci.2020.06.002.
- Zanettini C, Omar M, Dinalankara W, Imada EL, Colantuoni E, Parmigiani G, Marchionni L. 2021. Influenza vaccination and COVID-19 mortality in the USA: an ecological study. Vaccines (Basel). 9(5):427. https://doi.org/10.3390/vaccines9050427.
- Zeberg H, Pääbo S. 2020. The major genetic risk factor for severe COVID-19 is inherited from neanderthals. Nature. 587:610–612. https://doi.org/10.1038/s41586-020-2818-3.
- Zhang J, Saad R, Taylor EW, Rayman MP. 2020a. Selenium and selenoproteins in viral infection with potential relevance to COVID-19. Redox Biol. 37:101715. https://doi.org/10.1016/j.redox.2020.101715.
- Zhang J-M, An J. 2007. Cytokines, inflammation, and pain. Int Anesthesiol Clin. 45(2):27–37. https://doi.org/10.1097/AIA.0b013e318034194e.
- Zhang L, Richards A, Barrasa MI, Hughes SH, Young RA, Jaenisch R. 2021a. Reverse-transcribed SARS-CoV-2 RNA can integrate into the genome of cultured human cells and can be expressed in patient-derived tissues. Proceedings of the National Academy of Sciences. 118(21):e2105968118. http://dx.doi.org/10.1073/pnas.2105968118.
- Zhang Q, Bastard P, Liu Z, Le Pen J, Moncada-Velez M, Chen J, Ogishi M, Sabli IKD, Hodeib S, Korol C, et al. 2020b. Inborn errors of type I IFN immunity in patients with life-threatening COVID-19. Science. 370. http://doi.org/10.1126/science.abd4570.
- Zhang R, Wang X, Ni L, Di X, Ma B, Niu S, Liu C, Reiter RJ. 2020c. COVID-19: melatonin as a potential adjuvant treatment. Life Sci. 250:117583. https://doi.org/10.1016/j.lfs.2020.117583.
- Zhang SD, Lu J. 2020. In silico design of siRNAs targeting existing and future respiratory viruses with virus. bioRxiv. https://doi.org/10.1101/2020.08.13.250076.
- Zhang Y, Guo R, Kim SH, Shah H, Zhang S, Liang JH, Fang Y, Gentili M, Leary CNO’, Leary SJ, et al. 2021b. SARS-CoV-2 hijacks folate and one-carbon metabolism for viral replication. Nat Commun. 12:1676. https://doi.org/10.1038/s41467-021-21903-z.
- Zhang Y, Zhang J, Chen Y, Luo B, Yuan Y, Huang F, Yang T, Yu F, Liu J, Liu B, et al. 2020d. The ORF8 protein of SARS-CoV-2 mediates immune evasion through potently downregulating MHC-I. bioRxiv. https://doi.org/10.1101/2020.05.24.111823.
- Zheng D, Liwinski T, Elinav E. 2020a. Interaction between microbiota and immunity in health and disease. Cell Res. 30:492–506. https://doi.org/10.1038/s41422-020-0332-7.
- Zheng Y, Li R, Liu S. 2020b. Immunoregulation with mTOR inhibitors to prevent COVID-19 severity: a novel intervention strategy beyond vaccines and specific antiviral medicines. J Med Virol. 92:1495–1500. https://doi.org/10.1002/jmv.26009.
- Zhou D, Duyvesteyn HME, Chen CP, Huang C-G, Chen T-H, Shih S-R, Lin Y-C, Cheng C-Y, Cheng S-H, Huang Y-C, et al. 2020a. Structural basis for the neutralization of SARS-CoV-2 by an antibody from a convalescent patient. Nat Struct Mol Biol. 27:950–958. https://doi.org/10.1038/s41594-020-0480-y.
- Zhou F, Yu T, Du R, Fan G, Liu Y, Liu Z, Xiang J, Wang Y, Song B, Gu X, et al. 2020b. Clinical course and risk factors for mortality of adult inpatients with COVID-19 in Wuhan, China: a retrospective cohort study. Lancet. 395. https://doi.org/10.1016/S0140-6736(20)30566-3.
- Zimmermann P, Curtis N. 2020. Why is COVID-19 less severe in children? A review of the proposed mechanisms underlying the age-related difference in severity of SARS-CoV-2 infections. Arch Dis Child. https://doi.org/10.1136/archdischild-2020-320338.
- Zoufaly A, Poglitsch M, Aberle JH, Hoepler W, Seitz T, Traugott M, Grieb A, Pawelka E, Laferl H, Wenisch C, et al. 2020. Human recombinant soluble ACE2 in severe COVID-19. Lancet Respir Med. 8:1154–1158. https://doi.org/10.1016/S2213-2600(20)30418-5.
- Zumla A, Chan J, Azhar E, Hui DSC, Yuen K-Y. 2016. Coronaviruses — drug discovery and therapeutic options. Nat Rev Drug Discovery. 15:327–347. https://doi.org/10.1038/nrd.2015.37.
- Zuo T, Liu Q, Zhang F, Lui GC-Y, Tso EYK, Yeoh YK, Chen Z, Boon SS, Chan FKL, Chan PKS, et al. 2021. Depicting SARS-CoV-2 faecal viral activity in association with gut microbiota composition in patients with COVID-19. Gut. 70:276–284. https://doi.org/10.1136/gutjnl-2020-322294.
- Zuo T, Zhan H, Zhang F, Liu Q, Tso EYK, Lui GCY, Chen N, Li A, Lu W, Chan FKL, et al. 2020a. Alterations in fecal fungal microbiome of patients With COVID-19 during time of hospitalization until discharge. Gastroenterology. 159(4). https://doi.org/10.1053/j.gastro.2020.06.048.
- Zuo Y, Estes SK, Ali RA, et al. 2020b. Prothrombotic autoantibodies in serum from patients hospitalized with COVID-19. Sci Transl Med. 12(570):eabd3876. https://doi.org/10.1126/scitranslmed.abd3876.