Abstract
Wet bubble disease caused by Hypomyces perniciosus (syn: Mycogone perniciosa) is one of the major constraints in the production of button mushroom (Agaricus bisporus) worldwide. Deformation of basidiome and exudation of brown coloured liquid are the characteristic symptoms of wet bubble disease. The objective of this research was to assess the in vitro production of antimicrobial compounds by H. perniciosus. The culture filtrate of H. perniciosus induced leakage of cellular electrolytes from the mycelium of A. bisporus. Thin-layer chromatography analysis of the ethyl acetate extract of the culture filtrate of H. perniciosus revealed the presence of a predominant band at Rf 0.8 reacting to Folin–Ciocalteu reagent. The compounds at Rf 0.8 were capable of inducing electrolyte leakage from A. bisporus mycelium. Ultra-performance liquid chromatography analysis of the purified fraction revealed the presence of syringic, vanillic, cinnamic, 2-hydroxycinnamic, ferulic and p-coumaric acids suggesting that phenolic acids in the culture filtrate might be involved in the mycoparasitic activity of H. perniciosus.
Introduction
Wet bubble disease of Agaricus bisporus (J. Lange) Imbach caused by the mycoparasitic fungus Hypomyces perniciosus Magnus (Mycogone perniciosa (Magnus) Delacroix) is one of the major constraints in the button mushroom (Agaricus bisporus) production worldwide. The parasitic fungus severely affects the morphogenesis of A. bisporus and causes deformation of basidiome into fluffy white growth followed by decay and exudation of amber coloured liquid from the deformed tissues, thereby seriously affecting the yield (Fletcher et al. Citation1995; Fu et al. Citation2016; Zhang et al. Citation2017). In general, vegetative mycelium of A. bisporus is not affected by H. perniciosus (Zhang et al. Citation2017). The morphological, genetic and pathogenic variability in isolates of M. perniciosa have been reported (Fletcher et al. Citation1995; Wen et al. Citation2010; Wang et al. Citation2016; Zhou et al. Citation2016; Li et al. Citation2019b). Li et al. (Citation2019b) reported that brown coloured isolates of M. perniciosa were highly virulent compared to white or yellow coloured isolates. Huang et al. (Citation2014) while studying the interactions between M. perniciosa and A. bisporus reported that the volatile compounds of M. perniciosa inhibited the growth of A. bisporus and caused mycelial knots and breakages. The production of certain toxic metabolites by a few pathogens of A. bisporus has been documented. For instance, Trichoderma aggressivum, the causal agent of green mould disease is known to produce a toxin 3,4-dihydro-8- hydroxy-3-methyl isocoumarin, that inhibits the mycelial growth of A. bisporus (Krupke et al. Citation2003). However, antimicrobial compounds produced by H. perniciosus have not been reported. In this study, the production of fungitoxic metabolites by H. perniciosus was demonstrated and the toxic metabolites were characterized.
Materials and methods
Fungal strain
An isolate of Hypomyces perniciosus (GenBank accession number MZ149255) isolated from wet bubble-diseased Agaricus bisporus, was obtained from the Department of Plant Sciences, Sultan Qaboos University. The culture was maintained on potato dextrose agar (PDA) (Oxoid Ltd., UK).
Preparation of culture filtrate
H. perniciosus was grown in 500 ml conical flasks containing 250 ml of potato dextrose (PD) broth (Oxoid Ltd., UK) under stationary condition at 25°C for 3 weeks. The culture filtrate was collected by filtration through sterile filter paper and evaluated for its toxic activity towards A. bisporus by using electrolyte leakage bioassay.
Electrolyte leakage bioassay
A. bisporus was cultured in 500 ml conical flasks containing 250 ml of PD broth at 25°C for 2 weeks and mycelia were collected under aseptic conditions. Fifty mg of A. bisporus mycelium was transferred to a glass vial containing 10 ml of culture filtrate of H. perniciosus and the electrical conductivity of the solution was measured 3 h after incubation at 25°C using a conductivity meter (Jenway model 4510; Jenway, UK) and the data were recorded as µS cm−1 (Halo et al. Citation2018). The mycelial mat of A. bisporus treated with un-inoculated PD broth served as control. There were three replicates per treatment and control.
Analysis of toxic metabolites
The culture filtrate (500 ml) of H. perniciosus was mixed vigorously with ethyl acetate (1:1, v/v) in a separating funnel. The solvent fraction was collected and evaporated to dryness in vacuo using a rotary evaporator (Buchi Rotavapor R-215; BUCHI Labortechnik AG, Switzerland) at 50°C. The residues were dissolved in 1 ml of methanol followed by analysis using thin layer chromatography.
Thin layer chromatography (TLC)
An aliquot (10 µl) of the ethyl acetate extract was spotted on a pre-coated Silica Gel 60254 plate (Merck KGaA, Germany). The plate was developed in chloroform: ethyl acetate: formic acid (10:8:2, v/v/v) and visualized under UV light (254 nm). The plate was sprayed with Folin–Ciocalteu reagent (Sigma, USA) by using an atomizer and observed for the colour changes, and the retention factor (Rf) of the reacting band(s) was calculated. The silica gel at the Rf 0.8 from a newly developed and un-sprayed preparative TLC plate was scratched out and the compounds were eluted in methanol as described by Al-Rawahi et al. (Citation2018). The purified fraction was diluted in water (1:20, v/v) and evaluated for its ability to induce leakage of electrolytes from the mycelium of A. bisporus as described earlier. The chemical composition of the purified fraction was analyzed by ultra-performance liquid chromatography.
Ultra-performance liquid chromatography
An autosampler (SIL-30A; Shimadzu, Tokyo, Japan) was employed to introduce 20 µL of the sample into the UPLC (Shimadzu LC-30AD; Shimadzu, Japan). The analysis was carried out using a Tracer Excel 120 ODS column (3 µm particle size, 3 mm internal diameter (I.D.), 150 mm length, Teknokroma, Spain) maintained at 30°C using an oven (CTO-30A). The analysis was performed using a gradient program with a two solvent system A: 1% Acetic acid, B: Acetonitrile. Initial condition was 98% A; 0–27 min, changed to 75% A; 27–28 min, to 60% A; 28–35 min, to 56%A; 35–50 min to 90% A. The flow rate of the mobile phase was 0.3 mL/min. The compounds of interest were spectrally scanned (190–800 nm) by using a Diode Array detector (Prominence SPDM20A, Shimadzu, Japan) and 280 nm was selected to monitor the phenolic acids. Gallic acid (97.5%), 3,4-dihyroxybenzoic acid (≥97%), chlorogenic acid (≥97.0%), vanillic acid (≥97%), caffeic acid (≥98%), syringic acid (≥97%), p-coumaric acid (≥98%), ferulic acid (≥98%), 2-hydroxycinnamic acid (97%) and cinnamic acid (≥99%) were purchased from Sigma and Fluka.
Results and discussion
Findings from the electrolyte leakage bioassay showed that the culture filtrate obtained from H. perniciosus induced leakage of cellular electrolytes from the mycelium of A. bisporus and recorded 429 µS cm−1 after 3 h of incubation whereas the control recorded 24 µS cm−1, suggesting the presence of antifungal compounds in the culture filtrate. Li et al. (Citation2019a) identified several secondary metabolites genes including the genes involved in the biosynthesis of toxins, production of antibiotics, anthelminthics and antifungals in the genome of a highly virulent strain of H. perniciosus. The toxic activity of the culture filtrate of H. perniciosus on A. bisporus in this study is attributed mainly to its major compounds.
The electrolyte leakage is commonly used as an indicator of fungal cell membrane damage. This assay is being used to measure the toxic activities of a wide variety of compounds on fungi (Manhas and Kaur Citation2016; Halo et al. Citation2018; Al-Badi et al. Citation2020). Manhas and Kaur (Citation2016) while studying the inhibitory properties of the culture supernatant of Streptomyces hydrogenans against Alternaria brassicicola reported leakage of cellular electrolytes from A. brassicicola. Similarly, Halo et al. (Citation2018) demonstrated that the culture filtrate of Aspergillus terreus induced leakage of electrolytes from the mycelium of Pythium aphanidermatum. Al-Badi et al. (Citation2020) demonstrated that the culture filtrates Nigrospora sphaerica, Subramaniula cristata and Polycephalomyces sinensis caused electrolyte leakage from the mycelium of Monosporascus cannonballus. The leakage of electrolytes from the mycelium of A. bisporus upon treatment with the crude culture filtrate of H. perniciosus in this study indicates a possible cell membrane disruption (Manhas and Kaur Citation2016; Halo et al. Citation2018).
Zhang et al. (Citation2017) reported that H. perniciosus had no pathogenic activity on the mycelium of A. bisporus whereas basidiomes of A. bisporus at various growth stages were infected by H. perniciosus. In the present study, mycelium of A. bisporus was damaged by the culture filtrate of H. perniciosus and electrolytes were leaked out from the cells. Differences in the cell wall surface molecules between vegetative hyphae and fruiting body hyphae of A. bisporus have been documented (Lugones et al. Citation1998). For instance, the surface of vegetative mycelium of A. bisporus is covered by hydrophobin ABH3, whereas the fruiting bodies are lined with hydrophobin ABH1 (Lugones et al. Citation1996, Citation1998). The hyphal surface molecules play a key role in the attachment of mycoparasitic fungi to the hyphae of A. bisporus (Berendsen et al. Citation2010). Bernardo et al. (Citation2004) reported that a glucogalactomannan isolated from Lecanicillium fungicola, the causal agent of dry bubble disease of A. bisporus, failed to bind to the cell walls of A. bisporus vegetative hyphae, but specifically binds to the cell walls of A. bisporus fruiting body hyphae. The leakage of electrolytes from the vegetative mycelium of A. bisporus upon treatment with the culture filtrate of H. perniciosus in this study might be due to the direct contact of A. bisporus hyphal walls with the toxic metabolites of M. perniciosa in the electrolyte leakage bioassay.
The analysis of the ethyl acetate extract of the culture filtrate by TLC revealed the presence of a major band at Rf 0.80 reacting to Folin–Ciocalteu reagent (Figure ) suggesting the presence of phenolic compounds. The compounds at Rf 0.80 eluted from the TLC plate induced leakage of cellular electrolytes from the mycelium of A. bisporus similar to that of the crude culture filtrate and recorded 252 µS cm−1 after 3 h of incubation whereas the control recorded 41 µS cm−1. The electrolyte leakage induced by the partially purified phenolic compounds was lower than that of the crude culture filtrate of H. perniciosus. This may be due to lower concentration of phenolic compounds used in the bioassay as toxic compounds act on the mycelium of susceptible fungi and induce leakage of electrolytes in a dose-dependent manner (Simons et al. Citation2006). UPLC analysis of the active fraction at Rf 0.8 revealed the presence of syringic acid (10.44 ppm), vanillic acid (2.82 ppm), cinnamic acid (2.31 ppm), ferulic acid (0.76 ppm), 2-hydroxycinnamic acid (0.67 ppm) and p-coumaric acid (0.52 ppm) (Table ; Figure ). Several plant species and fungi are known to produce phenolic acids as secondary metabolites (Dey et al. Citation2016; Valanciene et al. Citation2020). Many phenolic acids have been shown to have antifungal (Heleno et al. Citation2013; Reis et al. Citation2013) and antibacterial (Alves et al. Citation2013) activities. Cinnamic and p-hydroxybenzoic acids have been found in extract of Ganoderma lucidum that showed antifungal activity against Aspergillus spp. (Heleno et al. Citation2013). Reis et al. (Citation2013) demonstrated that the extract of Cordiceps militaris that showed antifungal properties contained cinnamic and p-hydroxybenzoic acids. Alves et al. (Citation2013) reported that β-resorcylic and protocatechuic acids were present in extracts of wild mushrooms that exhibited antibacterial activity. It has been reported that the effect of phenolic acids depends on their concentrations (Ferrochio et al. Citation2013; Ferruz et al. Citation2016). For instance, 1 mM ferulic acid induced the growth of Fusarium verticillioides whereas 20 mM ferulic acid suppressed the fungal growth (Ferrochio et al. Citation2013).
Figure 1. TLC analysis of the culture filtrate of Hypomyces perniciosus. (A) under UV light; (B) sprayed with Folin-Ciocalteu reagent.
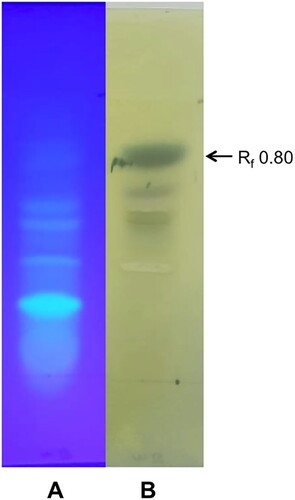
Figure 2. UPLC chromatograms of phenolic acid standards (A) and the compound eluted at Rf 0.8 from the TLC plate (B).
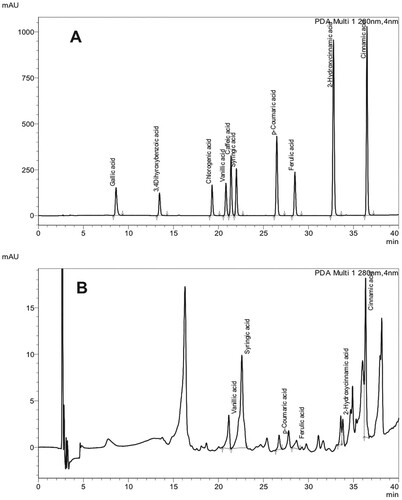
Table 1. Phenolic acids detected in the fraction at Rf 0.8 purified from the culture filtrate of Hypomyces perniciosus.
In this study, syringic acid (SA) was identified as the major constituent in the fraction purified from the culture filtrate of H. perniciosus that induced electrolyte leakage from the mycelium of A. bisporus. SA has been demonstrated to have antimicrobial properties (Chong et al. Citation2011; Srinivasulu et al. Citation2018). Shi et al. (Citation2016) demonstrated that SA inhibited the growth of Cronobacter sakazakii through modifications of its membrane function and alterations in membrane polarization, intracellular pH and ATP levels and morphology of the cells. SA was found to affect the permeability of bacterial cell membrane by inducing proton influx and ion leakage (Campos et al. Citation2009). It could be concluded from the results that SA might have caused permeability changes in the cell membrane of A. bisporus that resulted in the release of cellular electrolytes. Secretion of a few proteins that are essential for mycoparasitism such as protein kinases, carbohydrate-active enzymes, peptidases, cytochrome P450, and secondary metabolites by H. perniciosus has been reported earlier (Li et al. Citation2019a). To our knowledge this is the first report of secretion of antifungal phenolic acids by H. perniciosus. Further studies are required to assess the relationship between the levels of production of phenolic acids and mycoparasitic activities of H. perniciosus isolates.
Disclosure statement
No potential conflict of interest was reported by the author(s).
Data availability statement
The data supporting the findings of this study are available in Mendeley Data, V1, doi: 10.17632/mk5tjfg4j7.1 (https://data.mendeley.com/datasets/mk5tjfg4j7/1).
Additional information
Funding
References
- Al-Badi RS, Karunasinghe TG, Al-Sadi AM, Al-Mahmooli IH, Velazhahan R. 2020. In vitro antagonistic activity of endophytic fungi isolated from Shirazi Thyme (Zataria multiflora Boiss.) against Monosporascus cannonballus. Polish J Microbiol. 9:379–383.
- Al-Rawahi AY, Al-Mahmooli IH, Al-Sadi AM, Al-Sabahi JN, Velazhahan R. 2018. Toxin production by melon root rot fungus, Monosporascus cannonballus. Australas Plant Pathol. 47:543–546.
- Alves MJ, Ferreira IC, Froufe HJ, Abreu RMV, Martins A, Pintado M. 2013. Antimicrobial activity of phenolic compounds identified in wild mushrooms, SAR analysis and docking studies. J Appl Microbiol. 115:346–357.
- Berendsen RL, Baars JJ, Kalkhove SI, Lugones LG, Wosten HAB, Bakker PAHM. 2010. Lecanicillium fungicola: causal agent of dry bubble disease in white button mushroom. Mol Plant Pathol. 11:585–595.
- Bernardo D, Cabo AP, Novaes-Ledieu M, Mendoza CG. 2004. Verticillium disease or” dry bubble” of cultivated mushrooms: the Agaricus bisporus lectin recognizes and binds the Verticillium fungicola cell wall glucogalactomannan. Can J Microbiol. 50:729–735.
- Campos FM, Couto JA, Figueiredo AR, Tóth IV, Rangel AO, Hogg TA. 2009. Cell membrane damage induced by phenolic acids on wine lactic acid bacteria. Int J Food Microbiol. 135:144–151.
- Chong KP, Rossall S, Atong M. 2011. HPC fingerprints and in vitro antimicrobial activity of syringic acid, caffeic acid and 4-hydroxybenzoic acid against Ganoderma boninense. J Appl Sci. 11:2284–2291.
- Dey TB, Chakraborty S, Jain KK, Sharma A, Kuhad RC. 2016. Antioxidant phenolics and their microbial production by submerged and solid state fermentation process: a review. Trends Food Sci Technol. 53:60–74.
- Ferrochio L, Cendoya E, Farnochi MC, Massad W, Ramirez ML. 2013. Evaluation of ability of ferulic acid to control growth and fumonisin production of Fusarium verticillioides and Fusarium proliferatum on maize based media. Int J Food Microbiol. 167:215–220.
- Ferruz E, Atanasova-Penichon V, Bonnin-Verdal MN, Marchegay G, Pinson-Gadais L, Ducos C, Loran S, Arino A, Barreau C, Richard-Forget F. 2016. Effects of phenolic acids on the growth and production of T-2 and HT-2 toxins by Fusarium langsethiae and F. sporotrichioides. Molecules. 21:449.
- Fletcher JT, Jaffe B, Muthumeenakshi S, Brown AE, Wright DM. 1995. Variations in isolates of Mycogone perniciosa and in disease symptoms in Agaricus bisporus. Plant Pathol. 44:130–140.
- Fu Y, Wang X, Li D, Liu Y, Song B, Zhang C, Wang Q, Chen M, Zhang Z, Li Y. 2016. Identification of resistance to wet bubble disease and genetic diversity in wild and cultivated strains of Agaricus bisporus. Int J Mol Sci. 17:1568.
- Halo BA, Al-Yahyai RA, Al-Sadi AM. 2018. Aspergillus terreus inhibits growth and induces morphological abnormalities in Pythium aphanidermatum and suppresses Pythium-induced damping-off of cucumber. Front Microbiol. 9:95.
- Heleno SA, Ferreira IC, Esteves AP, Ciric A, Glamoclija J, Martins A, Sokovic M, Queiroz MJR. 2013. Antimicrobial and demelanizing activity of Ganoderma lucidum extract, p-hydroxybenzoic and cinnamic acids and their synthetic acetylated glucuronide methyl esters. Food Chem Toxicol. 58:95–100.
- Huang Q, Wang S, Zhang Y, Wen Z. 2014. The interactions between Mycogone perniciosa and Agaricus bisporus. Mycosystema. 33:440–448.
- Krupke OA, Castle AJ, Rinker DL. 2003. The North American mushroom competitor, Trichoderma aggressivum f. aggressivum, produces antifungal compounds in mushroom compost that inhibit mycelial growth of the commercial mushroom Agaricus bisporus. Mycol Res. 107:1467–1475.
- Li D, Sossah FL, Sun L, Fu Y, Li Y. 2019a. Genome analysis of Hypomyces perniciosus, the causal agent of wet bubble disease of button mushroom (Agaricus bisporus). Genes. 10:417.
- Li D, Sossah FL, Yang Y, Liu Z, Dai Y, Song B, Fu Y, Li Y. 2019b. Genetic and pathogenic variability of Mycogone perniciosa isolates causing wet bubble disease on Agaricus bisporus in China. Pathogens. 8:179.
- Lugones LG, Bosscher JS, Scholtmeyer K, de Vries OMH, Wessels JGH. 1996. An abundant hydrophobin (ABH1) forms hydrophobic rodlet layers in Agaricus bisporus fruiting bodies. Microbiology. 142:1321–1329.
- Lugones LG, Wosten HAB, Wessels JGH. 1998. A hydrophobin (ABH3) specifically secreted by vegetatively growing hyphae of Agaricus bisporus. Microbiology. 144:2345–2353.
- Manhas RK, Kaur T. 2016. Biocontrol potential of Streptomyces hydrogenans strain DH16 toward Alternaria brassicicola to control damping off and black leaf spot of Raphanus sativus. Front Plant Sci. 7:1869.
- Reis FS, Barros L, Calhelha RC, Ciric A, Van Griensven LJ, Sokovic M, Ferreira IC. 2013. The methanolic extract of Cordyceps militaris (L.) Link fruiting body shows antioxidant, antibacterial, antifungal and antihuman tumor cell lines properties. Food Chem Toxicol. 62:91–98.
- Shi C, Sun YI, Zheng Z, Zhang X, Song K, Jia Z, Chen Y, Yang M, Liu X, Dong R, Xia X. 2016. Antimicrobial activity of syringic acid against Cronobacter sakazakii and its effect on cell membrane. Food Chem. 197:100–106.
- Simons V, Morrissey JP, Latijnhouwers M, Csukai M, Cleaver A, Yarrow C, Osbourn A. 2006. Dual effects of plant steroidal alkaloids on Saccharomyces cerevisiae. Antimicrob Agents Chemother. 50:2732–2740.
- Srinivasulu C, Ramgopal M, Ramanjaneyulu G, Anuradha CM, Kumar CS. 2018. Syringic acid (SA) – a review of its occurrence, biosynthesis, pharmacological and industrial importance. Biomed Pharmacother. 108:547–557.
- Valanciene E, Jonuskiene I, Syrpas M, Augustiniene E, Matulis P, Simonavicius A, Malys N. 2020. Advances and prospects of phenolic acids production, biorefinery and analysis. Biomolecules. 10:874.
- Wang W, Li X, Chen B, Wang S, Li C, Wen Z. 2016. Analysis of genetic diversity and development of SCAR markers in a Mycogone perniciosa population. Curr Microbiol. 73:9–14.
- Wen Z, Wang Y, Liu X, Xie B. 2010. Population differentiation of Mycogone perniciosa in Fujian, China. Mycosystema. 29:329–334.
- Zhang C, Kakishima M, Xu J, Wang Q, Li Y. 2017. The effect of Hypomyces perniciosus on the mycelia and basidiomes of Agaricus bisporus. Microbiology. 163:1273–1282.
- Zhou C, Li D, Chen L, Li Y. 2016. Genetic diversity analysis of Mycogone perniciosa causing wet bubble disease of Agaricus bisporus in China using SRAP. J Phytopathol. 164:271–275.