Abstract
The meiosis-specific recombinase Dmc1 is a member of the Rad51 family that is required for the pairing of homologous chromosomes, formation of crossovers and recombination during meiosis. However, the molecular mechanisms of Dmc1 in cattle spermatogenesis remain unclear. In this study, we measured the methylation status of bDmc1 promoter by bisulfite sequencing, and the results showed that the methylation level of -2012CpG site in bDmc1 promoter was higher in the testes of cattle-yak hybrids (30%, 3/10) with male sterility compared with cattle (0%, 0/10). Moreover, the cattle bDmc1 promoter especially -2012CpG site was highly methylated in bovine mammary epithelial cells (BMECs) in which bDmc1 was low-expressed. Luciferase assays revealed that the promoter activity of bDmc1 was decreased significantly after M.SssI methylase treatment. Real-time PCR showed that bDmc1 transcription was dramatically induced in BMECs after treatment with 5-Aza-CdR. Interestingly, the differentially methylated site -2012CpG was located in the binding site for HSF by bioinformatics predictions. Taken together, these results suggest that the expression of bDmc1 was regulated by its promoter methylation and provide new insight into the molecular mechanism of Dmc1 in bovine spermatogenesis.
Introduction
Meiosis is an essential stage in spermatogenesis, consisting of two continuous rounds of division to produce sperm cells, the first and second meiotic division (meiosis I and II). During the first meiotic prophase, a series of complex molecular events occur, such as homologous chromosome pairing, synapsis, crossing-over at chiasma followed by separation of the sister chromosomes, and these finally result in two daughter cells each having a complete copy of the genome (Handel and Schimenti Citation2010; Chan et al. Citation2014). Proper chromosome segregation, crucial for preventing fertility problems, is dependent upon the formation of physical connections between chromosome pairs through homologous recombination (HR) that is triggered by DNA double-strand breaks (DSBs) (Gerton and Hawley Citation2005; Kagawa and Kurumizaka Citation2010; Lorenz et al. Citation2014). Therefore, HR is essential for the balance between genome diversity and stability, and proper chromosome segregation and formation of eupyrene sperm (Handel and Schimenti Citation2010). HR abnormalities can lead to various degrees of abnormal spermatogenesis and the emergence of infertility (Shin et al. Citation2010; Dowdle et al. Citation2013; Llano et al. Citation2014).
HR is markedly activated during meiotic prophase to play crucial roles in bridging the homologous chromosomes. This process is mediated by the RecA recombinase homologues Rad51 and Dmc1 (Cloud et al. Citation2012; Yamada and Ohta Citation2013; Qi et al. Citation2015). Rad51 is the only protein that acts directly in homologous joint molecules formation during mitotic recombination, whereas Dmc1 is a meiosis-specific protein at prophase I of the first meiotic division (zygotene stage); the latter is required for the pairing of homologous chromosomes and the formation of crossovers and recombination during meiosis (Kagawa and Kurumizaka Citation2010; Bugreev et al. Citation2011; Bugreev et al. Citation2014; Chang et al. Citation2015). Yeast dmc1 mutants are defective in reciprocal recombination and synaptonemal complex formation, and arrest in late prophase of meiosis I (Bishop et al. Citation1992). The targeted mutation of mouse Dmc1 gene results in the hallmarks of poorly repaired DSBs in reproductive cells, meiotic prophase arrest with failure of chromosome synapsis and sterility due to defects in homologous recombination (Pittman et al. Citation1998; Yoshida et al. Citation1998). In humans, two variants DMC1-M200 V and DMC1-I37N were identified; the former is a source of human infertility (Hikiba et al. Citation2008) whereas the latter is a source of improper meiotic recombination and causes meiotic defects (Hikiba et al. Citation2009). Our previous study had suggested that bovine Dmc1 (bDmc1) may be involved in bull spermatogenesis and its testicular low expression associated with male sterility in cattle-yak hybrids (Li et al. Citation2010). However, the epigenetic regulation and the molecular mechanism of Dmc1 remains unclear. In this study, we explored the epigenetic mechanisms of low bDmc1 expression in the testes of male cattle-yak hybrids.
Materials and methods
Sample and tissue collection
Male cattle (n = 8) and cattle-yak hybrids (n = 8) were provided by the Songpan Bovine Breeding Farm, Sichuan Province. The testes tissue of animals were collected through castration or slaughter. Bovine mammary epithelial cells (BMECs) were isolated from the mammary tissues of Holstein dairy cows, and were cultured in Dulbecco’s modified Eagle’s medium (DMEM) containing 4.5 mg/mL glucose and supplemented with 10% fetal bovine serum (Life Technologies, Gaithersburg, MD, USA), 1% antibiotic-antimycotic solution (Sigma-Aldrich, St. Louis, MO, USA), and 2 mmol/L glutamine (Sigma-Aldrich, St. Louis, MO, USA) in a humidified incubator, with 5% CO2 at 37°C. All experiments were undertaken in accordance with the guidelines of the regional Animal Ethics Committee and were approved by the Institutional Animal Care and Use Committee of Shenyang Agricultural University (2021006024).
DNA/RNA extraction
Genomic DNA of testes and BMECs was isolated by standard phenol–chloroform methods. Total RNA from BMECs was extracted by Trizol Total RNA Extract Kit (Invitrogen, CA, USA), and then it was reverse transcribed to cDNA using M-MLV reverse transcriptase and oligo(dT)18 (Promega, Madison, USA). The quality of DNA and total RNA was examined by UV-spectrophotometer after electrophoresis in 0.5% agarose (Promega, Madison, USA).
PCR and sequencing
A 0.5 µL of genomic DNA was used for PCR in a final volume of 10 µL containing 1 U of Ex Taq DNA polymerase (TaKaRa, Dalian, China), 1 µL of 10× PCR buffer, 0.25 mM each dNTP and 0.5 µL each primer (10 mM) (Table S1). PCR was performed: one cycle of 1 min at 94 °C; followed by 35 cycles of 30 s at 94 °C, annealing for 30 s, extension for 30 s at 72 °C; and a final extension for 5 min at 72 °C. PCR products were separated using 1.2% agarose gel (Promega, WI, USA) electrophoresis and purified using a DNA Purification Kit (Axygen, USA). The purified PCR products were cloned into the pMD18-T vector (TaKaRa) and then were transformed into Escherichia coli strain JM109 and sequenced by Shanghai Invitrogen Co. (Shanghai, China).
Bioinformatic analysis
Editing and alignment of the nucleotide sequence was performed by the EditSeq and MegAlign programs of DNASTAR 5.22 software (DNAStar Inc., WI, USA). We searched for candidates CpG islands with the online program (http://www.urogene.org/methprimer/index1.html). Prediction of transcription response elements was performed using JASPAR (http://jaspar.genereg.net/).
Bisulfite genomic sequencing
Primers P5 for bisulphite sequencing PCR (BSP) was designed by using Methyl Primer Express v1.0 software (ABI, Foster City, CA, USA) (Table S1). Sodium bisulfite treatment of genomic DNA from the testes was carried out using the MethylCode™ Bisulfite Conversion Kit (Invitrogen) according to the manufacturer’s instructions. The BSP was performed as previously described (Luo et al. Citation2013). The BSP products were cloned into pMD18-T vector (TaKaRa, Dalian, China). The insert was confirmed by restriction digestion and sanger sequencing. Ten subclones were selected for each DNA sample. The positions and percentages of methylated cytosine residues for all non-CpGs were then compared by aligning the sequenced results with the bisulphite modified DNA sequences converted from Methyl Primer Express v1.0 software (ABI).
Methylase M.SssI treatment
A 316-bp fragment was amplified from -2237/-1922 region of bDmc1 promoter using PCR with the primers P5 (Table S1). The amplified fragment was purified using a DNA Purification Kit (Axygen, USA), and cloned into pGL3 dual-luciferase reporter vector (pbDmc1). In vitro methylation reactions were performed in 100 µL containing 2 µL of pbDmc1 plasmids, 2 µL of CpG methyltransferase M.SssI (New England Biolabs, UK), 0.5 µL of S-adenosyl methionine (SAM) and 10 µL of 10× NEB buffer 2. The reaction was incubated at 37°C for 16 h followed by enzyme inhibition at 65°C for 20 min. The completion of the methylation reaction was confirmed by restriction digestion with methylation sensitive HpaII restriction enzyme (NEB). The restricted product was analyzed on 3% agarose gel. The methylated (mpbDmc1) or unmethylated (pbDmc1) plasmids were transfected into GC-1 and COS7 cell line with Lipofectamine 2000 (Invitrogen), respectively.
Dual-luciferase reporter assay
Cell extracts were prepared 30 h after transfection, and the luciferase activity was measured using the Dual-Luciferase Reporter Assay System (Promega) with a Modulus Single Tube Multimode Reader (Turner Biosystems, Sunnyvale, CA, USA) according to the manufacturer's protocol. Results are expressed as firefly/Renilla luciferase activities.
5′-Aza-CdR treatment
Primary BMECs at passage two were cultured for 72 h at 37°C in the absence 5% CO2, and then were seeded at low density in six-well plates and treated with 5 µM of methylase inhibitor 5-aza-2′-deoxycytidine (5-Aza-CdR) (Sigma, USA) as previously described (Linher et al. Citation2009).
Real-time PCR analysis
The expression level of bDmc1 in BMECs was evaluated by AceQ® qPCR SYBR® Green Master Mix (Vazyme Biotech Co., Ltd, China) using an ABI stepone plus Real-Time PCR system (ABI, USA). Real-time reactions were performed in a total volume of 20 µL containing 10 µL AceQ® qPCR SYBR® Green Master Mix, 0.4 µL each primer, 2 µL template cDNA, 0.4 µL ROX Reference Dye 1 and 6.8 µL water. Cycling conditions consisted of an initial 95 °C for 5 min followed by 40 cycles of 95 °C for 10 s, annealing temperature for 30 s. β-actin was used as internal parameter, with 3 repeats for every sample, and the primers were designed by primer premier 5 software and listed in Table S1. The relative quantification of bDmc1 was calculated by 2-ΔΔCt method.
Statistical analysis
Statistical analysis was performed by Student’s t test using SPSS19.0 (SPSS Inc., IL, USA). p < .05 was considered to be statistically significant.
Results
Cloning and characterization of 5’ regulatory region of bDmc1
To delineate the regulatory mechanisms for low expression of bDmc1 in cattle-yak testes, we cloned a 3-kb region on the 5’ end of the cattle bDmc1 gene. The region contained 5’ transcriptional regulatory region (TRR) sequence (2493 bp), complete exon 1 (51 bp) and partial sequence of intron 1 (452 bp) (Figure ). CpG islands (CGI) were identified using the online program CpG Island Searcher (http://ccnt.hsc.usc.edu/cpgislands2/cpg.aspx). One 759-bp CGI (-2493 nt ∼ -1735 nt) possessing a GC content of 59.3% and an observed CpG/expected CpG ratio of 0.735 was identified (Figure ).
Figure 1. The 5′-flanking sequence of cattle bDmc1 gene. Under line indicates the CpG island prediction. CpG sites in 316-bp sequence for methylation analysis were marked with a gray background. Dashed line indicates exon1. Bold letter indicates intron1. Nucleotide numbering is relative to +1 at the initiating ATG codon.
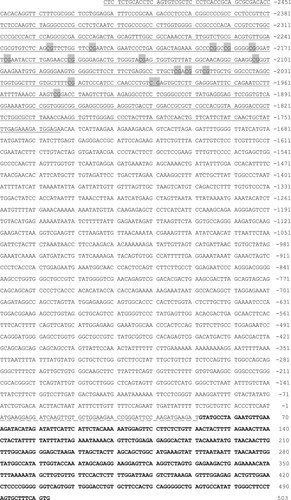
Methylation status of bDmc1 promoter
To assess methylation levels in CGI of bDmc1 promoter, primers P5 for bisulfite sequencing was designed (Table S1). The results revealed that the 316-bp sequence for methylation analysis contained 15 CpG sites, which were located at -2228 nt (CpG1), -2217 nt (CpG2), -2186 nt (CpG3), -2182 nt (CpG4), -2176 nt (CpG5), -2169 nt (CpG6), -2152 nt (CpG7), -2134 nt (CpG8), -2105 nt (CpG9), -2055 nt (CpG10), -2052 nt (CpG11), -2048 nt (CpG12), -2012 nt (CpG13), -1991 nt (CpG14) and -1946 nt (CpG15), respectively (Figure (a and b)). In cattle-yak hybrids with male sterility, we found higher bDmc1 promoter methylation (17.3%, 26/150) compared to that in cattle (12.0%, 18/150) (p < .10) (Figure (c)). In addition, we detected the methylation level of bDmc1 gene in the BMECs where bDmc1 expression was low. We also found that the methylation level of bDmc1 gene promoter in BMECs was significantly higher than in cattle testes (p < .05), however, it is not significantly different from that of cattle-yak hybrids testes (Figure (c)). Interestingly, the methylation level at CpG13 (-2012CpG) were significantly increased in the testes of cattle-yak hybrids and BMECs compared with the testes of cattle (p < .05).
Figure 2. The methylation profile of the CpG island in the bDmc1 promoter. (a) Schematic diagram of the CpG island in the bDmc1 promoter. Nucleotide numbering is relative to +1 at the initiating ATG codon. (b) Schematic diagram of the CpG sites for methylation analysis. The short vertical bars represent the CpG dinucleotides. (c) Promoter methylation statues of bDmc1 in cattle and cattle-yak hybrid testes, and bovine mammary epithelial cells (BMECs). Each line represents an individual bacterial clone which was sequenced. Open circles indicate unmethylated CpG sites, and filled circles indicate methylated CpG sites.
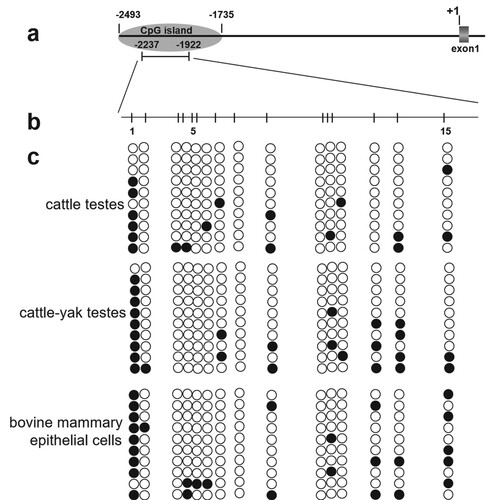
Effect of DNA methylation on bDmc1 promoter activity
To further determine the effects of promoter methylation on transcriptional activity of bDmc1 gene, we used DNA methylase to treat bDmc1 promoter reporter construct in vitro. Luciferase assays showed that the promoter activity of bDmc1 promoter plasmids in both GC-1 (a mouse germ cell line) and COS7 (non germ cell line) cells were significantly decreased after M.SssI methylase treatment (p < .01) (Figure ), suggesting that the promoter activity of bDmc1 was blocked by DNA methylation modification.
Figure 3. Methylase M.SssI represses bDmc1 promoter activity in vitro methylation. The bDmc1 promoter vector was treated with 2μL M.SssI methylase, and named mpbDmc1. The methylated or unmethylated vector was transfected into the GC-1 and COS7 cells. Normalized luciferase activities were expressed as mean ± SEM of at least three independent experiments. ** p < .01.
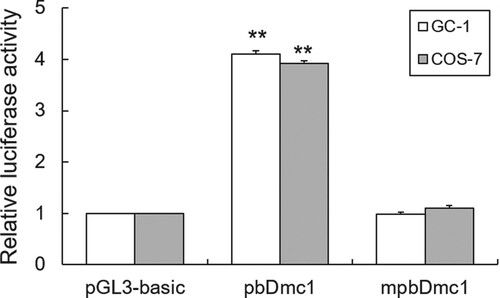
Effect of demethylation on the expression of bDmc1
To further confirm that hypermethylation of the promoter is responsible for the silencing of the bDmc1 gene, a DNA methyltransferase inhibitor 5-Aza-CdR was used to treat BMECs in which bDmc1 is low-expressed. Real-time qPCR revealed that bDmc1 mRNA expression was dramatically induced in BMECs after treatment with 5-Aza-CdR (p < .01, 3.0-fold change) compared to the control group (BMECs without 5-Aza-CdR treatment) (Figure ). This result further indicates that the expression of bDmc1 was regulated by DNA methylation of its promoter.
Discussion
The meiosis-specific recombinase Dmc1 is essential for meiotic recombination, synaptonemal complex formation, spermatogenesis and male reproduction (Sansam and Pezza Citation2015). Dysregulation or mutation of the Dmc1 gene could result in reproductive disorders such as male infertility (Hikiba et al. Citation2008; Li et al. Citation2010), while Dmc1-deficient mice are sterile due to defective meiotic recombination and chromosome synapsis (Yoshida et al. Citation1998; Bannister et al. Citation2007). Our previous study found that bDmc1 was lowly expressed in the testes of cattle-yak hybrids with male sterility (Li et al. Citation2010). Here, we demonstrate that DNA methylation is a novel epigenetic regulator of bDmc1 expression in the testes.
The epigenetic modification such as DNA methylation, histone modification and non-coding RNAs, play a crucial role in bull spermatogenesis, while epigenetic defects result in spermatogenesis failure, subfertility and male sterility (Du et al. Citation2014; Verma et al. Citation2014; Verma et al. Citation2015; Yao et al. Citation2015). Previous data demonstrated that Dmc1, a protein directly required for meiotic recombination of spermatogenesis, is regulated by DNA and histone methylation in mouse (Stielow et al. Citation2010). In this study, we show that the methylation level of bDmc1 promoter in testes of cattle-yak hybrids and BMECs with low bDmc1 expression were higher than that of cattle. Meanwhile, we demonstrated that promoter activity and expression of bDmc1 was regulated by DNA methylation through methyltransferase M.SssI and methyltransferase inhibitor 5-Aza-CdR treatment, respectively. A previous study shows that the Dmc1 promoter in mouse is highly methylated in mouse embryonic fibroblasts (MEFs), but is unmethylated in Sp3-/- MEFs linking SUMOylation of Sp3 to tissue-specific CpG methylation (Shin et al. Citation2010). Our results in the BMECs is also consistent with the above findings. In summary, these data suggest that there is a strong correlation between DNA methylation of bDmc1 promoter and its transcriptional activity.
DNA methylation modification in the gene promoter region can suppress gene transcription by preventing the combination of transcription factors and their CpG dinucleotide recognition sites (Kudo Citation1998; Bergman and Cedar Citation2013). In the present study, a CpG site (CpG13, -2012CpG) was found to be differentially methylated (DM) within bDmc1 promoter region among the testes of cattle, cattle-yak and BMECs. We next identified putative transcription factor binding sites associated with the DM CpG sites using JASPAR software and the results showed that -2012CpG is located in the binding site for the transcription factor HSF (Figure S1). HSF (heat shock factor) is a transcription factor that regulates the heat shock response. HSF regulates the target gene transcription activation or repression by binding to the consensus HSF sequence-binding elements (HSEs) of promoter region in target gene (Sasi et al. Citation2014; Carpenter et al. Citation2015). In males, HSF can regulate the expression of genes related to spermatogenesis, and is associated with apoptosis of germ cells, spermatogenic meiosis and male sterile (Vydra et al. Citation2006; Li et al. Citation2012). Therefore, we speculate that the hypermethylation of the HSF binding site (-2012CpG) in the bDmc1 promoter in the testicular tissues of cattle-yak hybrids is probably responsible for the lower expression of bDmc1.
Conclusions
In the study, we demonstrated that methylation level of bDmc1 gene was higher in cattle-yak testes than in cattle. Moreover, the promoter activity of bDmc1 gene was decreased by DNA methylase, while the expression level of bDmc1 gene was induced by DNA methyltransferase inhibitor 5-Aza-CdR. These results indicate that the methylation level of bDmc1 gene plays an important role in the bovine spermatogenesis. This study provides new insights into the role of bDmc1 gene methylation in spermatogenesis.
Disclosure statement
No potential conflict of interest was reported by the author(s).
Data availability statement
The data that support the findings of this study are available in the figshare repository (https://doi.org/10.6084/m9.figshare.16689553.v1).
Additional information
Funding
References
- Bannister LA, Pezza RJ, Donaldson JR, de Rooij DG, Schimenti KJ, Camerini-Otero RD, Schimenti JC. 2007. A dominant, recombination-defective allele of Dmc1 causing male-specific sterility. PLoS Biol. 5:e105.
- Bergman Y, Cedar H. 2013. DNA methylation dynamics in health and disease. Nat Struct Mol Biol. 20:274–281.
- Bishop DK, Park D, Xu L, Kleckner N. 1992. DMC1: a meiosis-specific yeast homolog of E. coli recA required for recombination, synaptonemal complex formation, and cell cycle progression. Cell. 69:439–456.
- Bugreev DV, Huang F, Mazina OM, Pezza RJ, Voloshin ON, Camerini-Otero RD, Mazin AV. 2014. HOP2-MND1 modulates RAD51 binding to nucleotides and DNA. Nat Commun. 5:4198.
- Bugreev DV, Pezza RJ, Mazina OM, Voloshin ON, Camerini-Otero RD, Mazin AV. 2011. The resistance of DMC1 D-loops to dissociation may account for the DMC1 requirement in meiosis (vol 18, pg 56, 2011). Nat Struct Mol Biol. 18:1084–1084.
- Carpenter RL, Paw I, Dewhirst MW, Lo HW. 2015. Akt phosphorylates and activates HSF-1 independent of heat shock, leading to slug overexpression and epithelial-mesenchymal transition (EMT) of HER2-overexpressing breast cancer cells. Oncogene. 34:546–557.
- Chan YL, Brown MS, Qin D, Handa N, Bishop DK. 2014. The third exon of the budding yeast meiotic recombination gene HOP2 is required for calcium-dependent and recombinase Dmc1-specific stimulation of homologous strand assimilation. J Biol Chem. 289:18076–18086.
- Chang HY, Liao CY, Su GC, Lin SW, Wang HW, Chi P. 2015. Functional relationship of ATP hydrolysis, presynaptic filament stability, and homologous DNA pairing activity of the human meiotic recombinase DMC1. J Biol Chem. 290:19863–19873.
- Cloud V, Chan Y L, Grubb J, Budke B, Bishop D K. 2012. Rad51 Is an accessory factor for Dmc1-mediated joint molecule formation during meiosis. Science. 337:1222–1225.
- Dowdle JA, Mehta M, Kass E M, Vuong B Q, Inagaki A, Egli D, Jasin M, Keeney S. 2013. Mouse BAZ1A (ACF1) Is dispensable for double-strand break repair but Is essential for averting improper gene expression during spermatogenesis. PLoS Genet. 9:e100394.
- Du Y, Wang XG, Wang B, Chen WJ, He RJ, Zhang L, Xing XP, Su JM, Wang YS, Zhang Y. 2014. Deep sequencing analysis of microRNAs in bovine sperm. Mol Reprod Dev. 81:1042–1052.
- Gerton JL, Hawley RS. 2005. Homologous chromosome interactions in meiosis: diversity amidst conservation. Nat Rev Genet. 6:477–487.
- Handel MA, Schimenti JC. 2010. Genetics of mammalian meiosis: regulation, dynamics and impact on fertility. Nat Rev Genet. 11:124–136.
- Hikiba J, Hirota K, Kagawa W, Ikawa S, Kinebuchi T, Sakane I, Takizawa Y, Yokoyama S, Mandon-Pepin B, Nicolas A, et al. 2008. Structural and functional analyses of the DMC1-M200 V polymorphism found in the human population. Nucleic Acids Res. 36:4181–4190.
- Hikiba J, Takizawa Y, Ikawa S, Shibata T, Kurumizaka H. 2009. Biochemical analysis of the human DMC1-I37N polymorphism. FEBS J. 276:457–465.
- Kagawa W, Kurumizaka H. 2010. From meiosis to postmeiotic events: uncovering the molecular roles of the meiosis-specific recombinase Dmc1. FEBS J. 277:590–598.
- Kudo S. 1998. Methyl-CpG-binding protein MeCP2 represses Sp1-activated transcription of the human leukosialin gene when the promoter is methylated. Mol Cell Biol. 18:5492–5499.
- Li D, Lin Y, Liu Z, Zhang Y, Rong Z, Liu X. 2012. Transcriptional regulation of human novel gene SPATA12 promoter by AP-1 and HSF. Gene. 511:18–25.
- Li X, Li Q, Zhao X, Xu H, Gu Y, Zhu X, Xie Z, Liu H. 2010. Cloning and expression level of Dmc1 gene in yak and cattle-yak testis. Scientia Agricultura Sinica. 43:3221–3229.
- Linher K, Cheung Q, Baker P, Bedecarrats G, Shiota K, Li J. 2009. An epigenetic mechanism regulates germ cell-specific expression of the porcine deleted in azoospermia-like (DAZL) gene. Differentiation. 77:335–349.
- Llano E, Gomez L, Garcia-Tunon I, Sanchez-Martin M, Caburet S, Barbero JL, Schimenti JC, Veitia RA, Pendas AM. 2014. STAG3 is a strong candidate gene for male infertility. Hum Mol Genet. 23:3421–3431.
- Lorenz A, Mehats A, Osman F, Whitby MC. 2014. Rad51/Dmc1 paralogs and mediators oppose DNA helicases to limit hybrid DNA formation and promote crossovers during meiotic recombination. Nucleic Acids Res. 42:13723–13735.
- Luo H, Zhou Y, Li YX, Li QF. 2013. Splice variants and promoter methylation status of the Bovine Vasa Homology (Bvh) gene may be involved in bull spermatogenesis. BMC Genet. 14:58.
- Pittman DL, Cobb J, Schimenti KJ, Wilson LA, Cooper DM, Brignull E, Handel MA, Schimenti JC. 1998. Meiotic prophase arrest with failure of chromosome synapsis in mice deficient for Dmc1, a germline-specific RecA homolog. Mol Cell. 1:697–705.
- Qi Z, Redding S, Lee JY, Gibb B, Kwon Y, Niu H, Gaines WA, Sung P, Greene EC. 2015. DNA sequence alignment by microhomology sampling during homologous recombination. Cell. 160:856–869.
- Sansam CL, Pezza RJ. 2015. Connecting by breaking and repairing: mechanisms of DNA strand exchange in meiotic recombination. FEBS J. 282:2431–2444.
- Sasi BK, Sonawane PJ, Gupta V, Sahu BS, Mahapatra NR. 2014. Coordinated transcriptional regulation of Hspa1a gene by multiple transcription factors: crucial roles for HSF-1, NF-Y, NF-kappaB, and CREB. J Mol Biol. 426:116–135.
- Shin YH, Choi Y, Erdin SU, Yatsenko SA, Kloc M, Yang F, Wang PJ, Meistrich ML, Rajkovic A. 2010. Hormad1 mutation disrupts synaptonemal complex formation, recombination, and chromosome segregation in mammalian meiosis. PLoS Genet. 6:e1001190.
- Stielow B, Kruger I, Diezko R, Finkernagel F, Gillemans N, Kong-a-San J, Philipsen S, Suske G. 2010. Epigenetic silencing of spermatocyte-specific and neuronal genes by SUMO modification of the transcription factor Sp3. PLoS Genet. 6:e1001203.
- Verma A, Rajput S, De S, Kumar R, Chakravarty AK, Datta TK. 2014. Genome-wide profiling of sperm DNA methylation in relation to buffalo (bubalus bubalis) bull fertility. Theriogenology. 82:750–759.
- Verma A, Rajput S, Kumar S, De S, Chakravarty AK, Kumar R, Datta TK. 2015. Differential histone modification status of spermatozoa in relation to fertility of buffalo bulls. J Cell Biochem. 116:743–753.
- Vydra N, Malusecka E, Jarzab M, Lisowska K, Glowala-Kosinska M, Benedyk K, Widlak P, Krawczyk Z, Widlak W. 2006. Spermatocyte-specific expression of constitutively active heat shock factor 1 induces HSP70i-resistant apoptosis in male germ cells. Cell Death Differ. 13:212–222.
- Yamada T, Ohta K. 2013. Initiation of meiotic recombination in chromatin structure. J Biochem. 154:107–114.
- Yao W, Li Y, Li B, Luo H, Xu H, Pan Z, Xie Z, Li Q. 2015. Epigenetic regulation of bovine spermatogenic cell-specific gene boule. PloS one. 10:e0128250.
- Yoshida K, Kondoh G, Matsuda Y, Habu T, Nishimune Y, Morita T. 1998. The mouse RecA-like gene Dmc1 is required for homologous chromosome synapsis during meiosis. Mol Cell. 1:707–718.