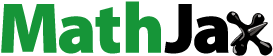
Abstract
In this study, effects of deficit irrigations and leonardite treatments on water use efficiency (WUE), water consumption and agricultural productivity of Lactuva Sativa Var. Crispa were examined in a pot experiment under greenhouse conditions. Experimental treatments included: L0: (Control), L5: (5% leonardite + 95% soil), L10: (10% leonardite + 90% soil) and L20: (20% leonardite + 80% soil) with I100 (full irrigation, control) and I80 and I60 (deficit irrigation). Plant water depletions were determined by weighing the pots. The highest total amount of irrigation water (9.74 × 10−3 m3/pots) was observed in L0I100 control treatment and the lowest value (5.52 × 10−3 m3/pots) was obtained from L20I60 treatment. The highest WUE (41.63 kg/m3) was obtained from L10I80 treatment. The greatest yield (139 g), plant length (20.14 cm) and number of leaves per plant (28) were respectively obtained from L0I100 treatments. The greatest plant diameter (37.05 cm) was obtained from L10I100 treatment. Present findings revealed that L0I100 treatments were prominent for yield and L10I80 treatments for WUE and plant nutrient uptake. Lactuva Sativa Var. Crispa exhibited a strong dependence on water; yield and growth parameters were adversely influenced from I60 deficit irrigation treatments. Additionally, plant water consumptions decreased with Leonardite treatments.
Introduction
Climate change and ever-increasing world population exert serious pressure on scarce environmental resources, notably on water (Bae and Dall'erba Citation2018). The annual precipitations are below 400 mm in the dryland farming areas of Turkey (Oğuz et al. Citation2016). Even with the use of entire renewable water resources, it is not possible to irrigate the entire irrigable lands. Limited water resources and climate change in arid and semi-arid regions have negative impacts on food and water security. Management of irrigation and compost may be used to tackle this issue (Ali et al. Citation2020; Ding et al. Citation2021). By optimizing yield and water productivity (Brempong et al. Citation2019; Ding et al. Citation2020), integrated management of irrigation and organic amendments may help farmers and decision-makers cope with water scarcity in drought and water-limited areas. In addition, increasing demand for food and water requires more efficient use of water in agriculture. Therefore, efficient use of water resources and improved yields with less water should be well analyzed. Deficit irrigation aims to increase the efficiency of irrigation water, to generate water stress at a level without excessive yield loss in the production period of the plant and consequently, to obtain the highest yield corresponding to each unit of water (Kirda Citation2002). Therefore, deficit irrigation should be taken into consideration as an adaptation strategy for regions with limited water resources or prone to drought (Afzal et al. Citation2016).
Crops will experience some level of water stress under deficit irrigation and often have lower yields than fully-irrigated plants (Lopez et al. Citation2017). Deficit irrigation can allow irrigation water savings of up to 20–40% at yield reductions below 10% and has been widely investigated in dry regions (Kögler and Söffker Citation2017). Many vegetable species are shallow-rooted and sensitive to mild water stress. Crop response to different water applications was used to determine irrigation strategies for optimal yield and maximum efficiency of water use for many crops. Meanwhile, crop sensitivity to water deficit can be affected by many factors, including climate conditions, crop species and cultivars and agronomic practices. In previous studies, significant yield losses were not encountered in deficit irrigations and high irrigation water use efficiencies (Patanè et al. Citation2011) or water savings were achieved without significant yield losses (Yang et al. Citation2015; Abd El-Wahed et al. Citation2017).
Irrigation water management is a difficult issue in some vegetables like lettuce due to the crop’s sensitivity to water shortage (Moreira et al. Citation2014). Lettuce is also one of the most widely cultivated vegetables in the world (Křístková et al. Citation2008). According to 2019 statistics, Turkey with an annual production of 30.1 × 106 Mg has fourth place in world vegetable production. According to 2018 statistics, the total lettuce and chicory production of Turkey was 487,543 tons (FAOSTAT Citation2020). However, semi-arid conditions are dominant over the majority of the vegetable fields of Turkey. Kuslu et al. (Citation2008) emphasized that lettuce grown in semi-arid regions should be irrigation without water shortage. In lettuce, where the harvested part of plant is the photosynthetic leaf area, it is especially important to maintain optimal growth through the application of water and nitrogen (Gallardo et al. Citation1996). Karam et al. (Citation2002) indicated that water stress caused by inadequate irrigation in lettuce plants significantly reduced the number of leaves, leaf area index and dry matter accumulation. Moreover, lettuce growth was accurately verified with the use of AquaCrop model simulations and concluded that deficit irrigations yielded 20–60% water savings as compared to full irrigation scenarios (Ket et al. Citation2018).
Water stress is directly related to the amount of water available in the soil. Soils with sandy texture retain and store less water for plants than those with medium or clayey texture, a situation that is aggravated if the soil is poor in organic matter (Stevenson Citation1994). Humic substances are one of the main components of organic matter in the soil. In recent years, many commercial products containing humic substances had been introduced. These products are generally extracted from peat or Leonardite, sometimes enriched with nutrients. Leonardite is a concentrated form of humic and fulvic acids and has the appearance of coal but it does not reach the compactness of coal (Erkoç Citation2009). In addition, Leonardite is rich in organic matter (50–75%) and humic acid content varies between 30% and 80% (Akinremi et al. Citation2000). Effects of humic acid-containing fertilizers on plant yield and nutrient uptake largely depend on humic acid source, concentration, application type, plant species and cultivars (Chen and Aviad Citation1990).
Many benefits of Leonardite have been investigated. These benefits include regulation of soil physical properties, increasing aggregate stability, increasing water holding capacity (Hassanpanah and Khodadadi Citation2009) and increasing aeration (Zandonadi et al. Citation2010). Leonardite also improves the structure of heavy clay soils and prevents salt accumulation in the soil (Yılmaz et al. Citation2008). In addition, an increase in plant root development with the acceleration of mycorrhizal activities was also reported (Moghadam et al. Citation2014). According to Garcia et al. (Citation2008), humic substances increase nutrient uptake due to higher permeability of the cell membrane, chelation and nutrient loading, besides reducing the damaging effects provoked by abiotic stress and promoting an auxin hormone effect. Some studies conducted in recent years do not agree with the above statements. According to Kolay et al. (Citation2016), Leonardite applications at 6 different doses (0, 50, 100, 150, 200 and 250 kg/da) had no effect on soil organic matter, soil moisture and bulk density, except for penetration resistance. Demir et al. (Citation2012) presented that the use of leonardite did not increase the soil organic matter in one year.
Although many studies have shown the positive effects of these products, the agricultural use of some other commercial humic substances was reported to have no effect on plant development. The best outcomes were achieved in soils poor in organic matter when the plants were cultivated under stress conditions (Hartz and Bottoms Citation2010). However, studies on the potential of HA to enhance drought tolerance are still in progress, and more investigation is required (Calvo et al. Citation2014). Gyttja and Leonardite used as organic material are abundantly available in Turkey. The use of domestic resources as fertilizer raw material and direct soil conditioner is economically important issue. Until now, less attention has been paid to the investigation of the combined impacts of irrigation and organic amendments (Leonardite) interactions on crop and water productivity. This study was conducted to determine the effects of leonardite treatments on water use efficiency, water consumption, yield and growth parameters of Lactuva Sativa Var. Crispa under deficit irrigation conditions.
Materials and methods
Experimental site
The research was carried out in a glass greenhouse compartment of Kahramanmaraş Sütçü İmam (KSU) University Faculty of Agriculture, located in the latitude and longitudes of 41° 33′ 25" North and 32° 12′ 01" East in the spring growing period of 2017.
Plant and organic material
Lactuva Sativa Var. Crispa, which is a variety of Iceberg, used as the plant material (Lactuca Sativa Var. Crispa is used as Lettuce for the remaining of the article). Leonardite mixed into potted soil at 0%, 5%, 10% and 20% on a weight basis. Characteristics related to Leonardite can be given as follows: grain size (0–3 mm), total organic matter of 51.2%, total humic + fulvic acid content of 46.2%, maximum humidity of 12.8% and pH of 5.5.
Chemical and physical analysis of soil
Prior to the experiment, some soil properties of top-soil layer of 30 cm, which was considered as effective root depth of lettuce, were determined using standard procedures described by Klute (Citation1986). The pH, electrical conductivity, soil texture, bulk density, field capacity and permanent wilting point values were determined. Soil texture was clay-loam. Analyzes were also conducted to determine the differences in post-harvest soil physical properties with the leonardite treatments. The results are given in Table .
Table 1. Soil properties at the end of the experiments.
Experimental design and treatments
Experiments were conducted in split-plots design with six replications (3 plants in each repetition) in total of 72 pots. Main plots included leonardite levels and the sub-plots included irrigation levels. In the experiment, three different irrigation levels and four leonardite levels were tested as treatments. The irrigation levels were full irrigation (I100), 80% of full irrigation (I80; 20% deficit), 60% of full irrigation (I60; 40% deficit) treatments. The Leonardite treatments were L0: (Control), L5: (5% leonardite + 95% soil), L10: (10% leonardite + 90% soil) and L20: (20% leonardite + 80% soil). Experimental treatments are given in detail in Table .
Table 2. Experimental treatments.
Irrigations in control treatments (I100) were practiced when approximately 30% of the available soil moisture within the effective root depth (30 cm) was depleted. The measured soil moisture in I100 treatment was used to initiate irrigation during the growing season. In I80 and I60 treatments, irrigations were applied at the rates of 80 and 60% of the control treatment on the same day, respectively. The irrigation water requirements were calculated according to the field capacity (L0 = 11.25 kg, L5 = 11.07 kg, L10 = 10.92 kg and L20 = 10.58 kg) of each treatment and applied to the pots by using a drip irrigation system. For the field capacity calculation, the following protocol was undertaken: a sample of 100 g (weight P1) of each leonardite level (control treatment for L0, L5, L10 and L20) was placed in a pot and then watered abundantly until water leaching (saturation phase). The pot is kept in a dry place for 24 h to drain the excess water, then reweighed (weight P2). It is expressed as a percentage (gram of water retained in 100 g of dry soil). The samples show field retention capacities for L0, L5, L10 and L20. Since the weight of pots is known (dry soil) then, field retention capacities are multiplied by the weight of pots. The founding water is added to the dry soil + pot weight, and the pot weight in the field capacity is determined. Water consumption was regularly determined by weighing the pots as it was in lysimeters and irrigation was applied as to bring the soil moisture back to field capacity. The irrigation interval was determined as 3–4 d.
The amount of irrigation water to be applied in each irrigation was calculated with the following Equation (1) (Kurunc et al. Citation2011):
(1)
(1) where I is the amount of irrigation water to be applied in each treatment (l); WFC is the pot weight at the field capacity (kg), Wa is the pot weight before irrigation, ρw is water density (1000 kg/m3) and P is the water application level (%).
Evapotranspiration was determined by using the following Equation (2) (Ünlükara et al. Citation2010).
(2)
(2) where Wb and Ws are the pot weights (kg) at the beginning of the experiment and harvest respectively, ρw is the water density (1000 kg/m3), I is the total amount of irrigation water applied to the pots during the growing season (m3) and D is the total drainage from the bottom of the pot (m3).
The water use efficiency (WUE) was calculated with Equation (3) given below (Erice et al. Citation2011).
(3)
(3) where WUE is the water use efficiency, (kg/m3); Y is the yield, (kg/pot); ET is the evapotranspiration, (m3/pot).
The irrigation water use efficiency (IWUE) was calculated with the Equation (4) given below (Erice et al. Citation2011).
(4)
(4) where IWUE is the irrigation water use efficiency, (kg/m3); Y is the yield, (kg/pot); I is the amount of irrigation water, (m3/pot).
Irrigation water was applied to the plants by the drip irrigation method. The drippers were inline type and spaced 0.20 m apart from each other and had 8 × 10−3 m3/h discharge rate.
Plants received six uniform rate applications varying from 0.4 × 10−3 m3/pots to 0.8 × 10−3 m3/pots were made during the plant establishment period (3-24/4/2017). Treatment irrigations were started on April 28, and terminated on May 19. The total amount of irrigation water is given Table . As shown in this table the amount of irrigation water applied varied from 9.74 × 10−3 m3/pots to 5.52 × 10−3 m3/pots.
Table 3. Amounts of applied irrigation water.
Measured traits
The following measurements and observations were made to evaluate the effects of different leonardite mixture ratios and irrigation water levels on investigated parameters: Yield, plant height, plant diameter, core diameter, root fresh weight and number of leaves, water consumption, water use efficiencies (WUE, IWUE). The plant height, plant diameter, core diameter and number of leaves were determined just before and after the harvest by using standard procedures. The head fresh weight (yield) and root fresh weight were determined using an electronic balance (± 0.1 g). In addition, plant nitrogen content was determined with the use of Kjeldahl method and phosphorus content with the use of an ICP-AES (Varian, Vista) device (Kaçar Citation2014).
Cultural practices
Plastic pots of 12-liter in volume, 50 cm in length, 15 cm in height and 17 cm width were used. Soil taken from the experimental fields was laid on the ground in the greenhouse (Figure ) and placed into the pots according to weight basis. While the leonardite should be kept as mixed with the soil for about 60 days in order to provide organic matter (leonardite) interaction, it could be kept for 30 days due to the climate conditions and the shortage of time in cultivating the plant variety. Irrigation water used in the study was obtained from pipe Networks of KSU University Campus. Generally, irrigations were started at 10.00 in the morning depending on the air temperature and ventilation in the greenhouse. As a maintenance process, manual weed control was practiced. NPK (20-20-0) was given to the plants as fertilizer. All pots were applied twice by 2 g/pot of fertilizers. Fertilizations were practiced twice on 25th and 40th day after planting. Manual harvest was carried out on 25 May 2017 (52 days after transplanting). The plants were removed from the soil by cutting with a knife over the root collar.
Statistical analysis
Experimental findings were analyzed with the use of SPSS software. Multivariate analysis of variance (MANOVA) was carried out to evaluate the effects of different leonardite and irrigation water levels on investigated parameters of lettuce. All parameters were subjected then to a one-way analysis of variance and significant means were compared with the use of Tukey’s test at p < 0.05.
Results and discussion
Multivariate analysis of variance (MANOVA) revealed that leonardite levels (L), irrigation levels (I) and interactions (L x I) had highly significant effects on investigated parameters (p = 0.000 at α < 0.05) indicating variability of plant response to deficit irrigations based on different leonardite levels (Table ). ANOVA results revealed that leonardite and irrigation levels had highly significant effects on all measured parameters (yield, plant dimeter, plant height, leaf number, core diameter) except for root fresh weight. The effects of interactions (L x I) were also found to be significant for all parameters, except for plant height, number of leaves and root fresh weight (Table ).
Table 4. Significant multivariate effects (at p < 0.05).
Table 5. Effects of irrigation and leonardite levels on different variables.
Changes in soil properties
Soil texture was clay-loam (SCL) initially, then turned into clay texture with the addition of leonardite. Soil density increased with increasing leonardite levels. There was an increase in organic matter with leonardite additions. Similarly, there was an increase in FC levels of L20 treatments. FC values varied between 32% (L0) and 37% (L20). Such changes are indicative of regulatory effects of leonardite on soil properties as expected. When the amount of leonardite increased from L0 to L20, soil bulk density decreased from 1.38 to 1.27 g/cm3 (Table ), thus, water holding capacity increased accordingly. Torun (Citation2009) reported that organic material (gittja, leonardite) supplementations improved soil chemical (especially pH) and physical (aggregation, pore distribution, permeability, and water holding capacity) properties and improvements were more dominant over time.
Irrigation water and evapotranspiration (ET)
Amount of applied irrigation water quantities generally decreased with increasing leonardite levels (Table ). The highest irrigation water quantity (9.74 × 10−3 m3/pots) was measured in L0I100 (control) treatment and the lowest (7.20 × 10−3 m3/pots) in L20I100 treatment. Such a case was attributed to increasing water holding capacity with leonardite treatments. Hassanpanah and Khodadadi (Citation2009) indicated more efficient water use and increasing resistance to water stress with leonardite treatments. Humic substances support the form of soil structure that enables water absorption and helps to retain water within the rootzone.
Table 6. The amount of irrigation water and evapotranspiration (ET), water use efficiencies and yield of lettuce.
Among all treatments in the experiments, the greatest ET (11.14 × 10−3 m3/pots) was obtained from L0I100 treatment and the lowest ET (6.48 × 10−3 m3/pots) from L10I60 treatment (Table ). The lowest water consumption in I60 treatments could be related to lack of soil water content resulting from deficit irrigation. Increasing amount of irrigation water also increased the water availability for evapotranspiration.
In order to clarify the effects of irrigation on ET, regression analysis was performed. There was a significant linear relationship (R2 = 0.99 in L0, R2 = 1 in L5, R2 = 0.99 in L10 and R2 = 0.99 in L20 treatments) between the irrigation water applied and crop evapotranspiration as shown in Figure . Here, ET values for full irrigation (control) treatments in all leonardite levels were greater than the ET values of deficit irrigation treatments. Generally, the highest ET values were calculated for L0 treatments. The relationship between seasonal evapotranspiration and marketable yield was linear (Gallardo et al. Citation1996; Kırnak et al. Citation2002). In this study, lettuce showed different yield responses to leonardite levels in each irrigation level. There was again linear relationship between the crop evapotranspiration and the yield of lettuce in all substrates (Figure ).
Figure 3. The relationship between lettuce yield and cumulative evapotranspiration under different irrigation and leonardite levels.
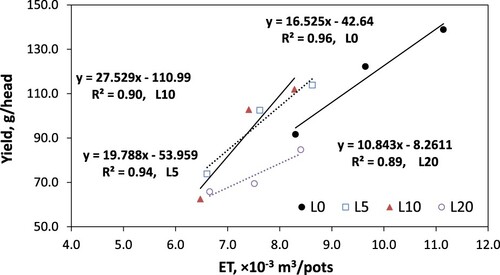
Yield increased as the water application increased up to 138.89 g/head for lettuce with associated seasonal evapotranspiration of 11.14 × 10−3 m3/pots in L0I100 treatment (Table ). Similar increases were observed in all full-irrigated plots (control) where the increase of yield was up to 113.89, 111.94 g/head with associated seasonal evapotranspiration of 8.63 × 10−3 m3/pots and 8.28 × 10−3 m3/pots from L5I100, L10I100, respectively. The decrease in seasonal evapotranspiration by increasing water deficit was similar in some other studies. Lettuce evapotranspiration was the highest in I1 (100% of evaporated water from a Class A pan) treatment (214.1 mm). Therefore, I1 treatment provided the maximum growth and marketable yield (2.17 kg/m2) (Sahin et al. Citation2016).
Water use efficiency (WUE) and irrigation water use efficiency (IWUE)
Water use efficiency (WUE), indicating the amount of production per unit water, was affected by irrigation treatments (p < 0.05). WUE values ranged from 27.72 kg/m3 of water in L20I80 to 41.63 kg/m3 of water in L10I80 treatments. The highest IWUE (46.55 kg/m3) was observed in L10I80 treatment (Table ). In general, I80 and I100 (control) treatments yielded more successful outcomes. The lowest values were observed in treatments with high water stress levels. Although leonardite increased water holding capacity, it decreased yield levels.
Lettuce contains 85-90% water in its structure and needs a significant amount of water during plant development. Soils have quite a high available water levels at field capacity. Alenazi et al. (Citation2016) showed that the use of humic acid and correct irrigation regime had an important role in increasing water use efficiency (WUE).
In this study, the yield values decreased in all levels of leonardite as water stress increased. With the high WUE in I80 irrigation treatment (20% deficit), the yield reduction was at least 8.19% in L10I80 (Table ). Thus, the highest WUE was calculated as 41.63% in this treatment. The minimum relative yield for 40% deficit (I60) was obtained from L20 treatments. Acar et al. (Citation2008) reported 14% and 39% yield reductions with I80 and I60 treatments, respectively. Kırnak et al. (Citation2002) reported about 50% yield reduction with 75% water deficit treatment.
Silva et al. (Citation2018) reported the highest WUE of lettuce (285.9 kg/ha/mm) for autumn growing season and the lowest WUE values for winter and summer growing seasons. The experiments point out that accurate management of irrigation water using a drip irrigation system associated with soil mulching increased yield and improved WUE of lettuce crops in the Central Dry Zone, Myanmar (Michelon et al. Citation2020). Moreover, Maraseni et al. (Citation2012) detected a WUE of 19 kg/m3 in lettuce grown under drip irrigation system in eastern Australia. Molina-Montenegro et al. (Citation2011) indicated that slight decreases in water availability could enable lettuce plants to exhibit a high WUE, maintaining high levels of physiological performance and productivity. The greatest water use efficiencies were observed in 75% irrigation treatments (p < 0.0001), followed respectively by 100% irrigation and 50% irrigation treatments.
Although increasing water use efficiency is a strategy to contribute the sustainable use of limited water resources, the results of this study showed that water use efficiency in lettuce decreased with deficit irrigations, especially with 40% deficit (I60). Therefore, present findings were not similar with the results of different researchers reporting increasing WUE and IWUE values with decreasing irrigation water quantities (Chala and Yohannes Citation2015; Gianino et al. Citation2015). However, similar to present findings, some researchers also obtained higher WUE and IWUE values in full irrigation treatments (Capra et al. Citation2008; Yazgan et al. Citation2008).
Effect of leonardite levels and irrigation levels on yield parameters
Yield response of lettuce to four different leonardite levels and three different irrigation levels were determined in this study. Analysis of variance was performed to determine the effects of leonardite and irrigation levels on lettuce yield. Effects of leonardite and irrigation levels on different variables were determined by applying Tukey’s test (p < 0.05) (Table ). Yield was affected by leonardite and irrigation levels. Figure (A) shows variations in head weight at the end of the experiment. Decreasing head weights (yield) were observed with increasing leonardite and water-deficit levels. Yields reached maximum levels in full irrigation (control) treatments. Deficit irrigations caused a decrease in yield at all leonardit levels. The highest yield was obtained from I100 (control) and the lowest from I60 treatments. However, the highest yield was obtained from the plants of L0I100 (138.89 ± 5.89 g/head) and the lowest yield from the plants of L10I60 treatments (62.50 ± 3.48 g/head).
Figure 4. Effect of different irrigation and leonardite levels on yield and growth parameters of lettuce (Tukey’s test, p < 0.05). Vertical bars denote the standard error of the mean (SEM).
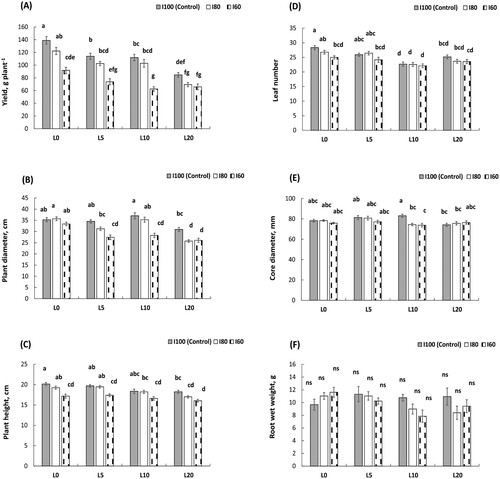
Acar et al. (Citation2008) reported that the highest head weight and marketable head weight (355.17 and 334.78 g) were obtained I100 (fully-irrigated) treatments. Çivit (Citation2010) stated that the highest head (plant) weight (396.7 g/plant) was obtained from leonardite-containing (25%) pots, followed by 15% leonardite-containing pots (389.2 g/plant) and 5% leonardite-containing pots (379.1 g/plant). Bozkurt et al. (Citation2009) reported the highest yield (595.7 g/plant) for Kcp100 (full irrigation level) treatments. In another study, the maximum growth and marketable yield were obtained from I100 (full-irrigated) treatment (Sahin et al. Citation2016). Rolbiecki and Rolbiecki (Citation2007) reported that irrigation significantly increased the marketable yield of lettuce. Present findings do not comply with the above-given results since leonardite was not mixed with the other fertilizers and materials in the present study. Because this depends on the origin of the material, extraction method, and concentration and composition of the humic extract. Besides these factors, the development stage and growing environment (under stress conditions) influence the effect of humic substances.
Effect of leonardite levels and irrigation levels on growth parameters
Treatment means were separated by applying Tukey’s test (p < 0.05). Interaction effects of leonardite and irrigation levels on plant diameter were significantly different (p < 0.05) (Table ). The highest plant diameter (37.05 ± 1.35 cm) was obtained from L10I100 treatment, followed by respectively by L0 (35.27 ± 0.90 cm), L5 (34.54 ± 0.76 cm) and L20 (30.95 ± 0.85 cm) treatments and the lowest values were obtained from L20I80 (25.76 ± 0.58 cm) and L20I60 (26.09 ± 0.95 cm) treatments (Figure (B)). Effects of interactions on plant heights were not found to be significant (p > 0.05) (Table ). Considering the leonardite treatments independently, it was observed that L0 and L5 treatments were included in the same group for average plant heights. Irrigation water levels had greater effects on plant sizes than the leonardite levels. The greatest plant height (20.14 ± 0.35 cm) was obtained from L0I100 treatment (Figure (C)). Other treatments had differences based on irrigation levels. As the water deficit and leonardite levels increased, plant heights decreased. The reductions in plant height under 20% water stress were more apparent in L0 and L20 (4.41% and 6.80%) treatment, while such reductions were quite close to each other in L5 and L10 treatments (1%). The lowest value was found in L20I60 treatment. Leonardite and irrigation levels had saparetely significant (p < 0.05) effects on number of leaves, while interactions (leonardite × irrigation) did not (p > 0.05) (Table .). In addition, I100 and I80 irrigation treatments were placed into the same group and number of leaves was higher in these treatments. Remarkably, there was no significant difference in number of leaves of L10 treatments. The lowest number of leaves varied between 22.11 ± 0.65 and 22.56 ± 0.71. The highest number of leaves was recorded in L0I100 (28.33 ± 0.70) (Figure (D)). The highest core diameter (83.06 ± 1.65 mm) was observed in L10I100 treatment and the lowest (73.78 ± 1.81 mm) in L10I60 treatment (Figure (E)). As water stress increased in the other leonardite levels, there was no significant difference and they were included in the same group. Root fresh weights varied between 7.84 ± 0.96 and 11.61 ± 0.78 g. While root fresh weights decreased in L5, L10 and L20 treatments under deficit irrigation, but increased in L0 treatment (Figure (F)).
Kuslu et al. (Citation2008) reported that the highest plant length, plant width, stem diameter and number of leaves were obtained from T-100 (full irrigation) treatments and the lowest from T-20 (80% water deficit) treatments. Present findings comply with those earlier ones. Acar et al. (Citation2008) reported that different irrigation levels did not significantly affect mean number of leaves, head height and head diameter. Although irrigation levels had significant effects on plant and root fresh weights, they did not affect the plant dry weight. The highest growth parameters were observed in the highest-irrigated (I1) treatment. Çivit (Citation2010) stated that the highest plant diameter, plant length, number of leaves, core diameter and root fresh weight were obtained from Leonardit-25 (the highest application) treatments. The highest yield, plant and core diameters, head tightness and number of marketable leaves were obtained from Kcp125, while the lowest values were observed in Kcp0 (control) treatments. In Kcp125 and Kcp100 treatments, core diameter was 120.1 and 118.3 mm, respectively, and root fresh weight was 11.7 and 12.3 g, respectively (Bozkurt et al. Citation2009).
Effects of leonardite and water treatments on nitrogen and phosphorus content of lettuce
In general, decreasing phosphorus and nitrogen contents were observed with decreasing irrigation water levels. While, the treatments significantly (p < 0.05) affected Phosphorus uptake, not in nitrate uptake of lettuce. Considering the effects of leonardite levels, the highest phosphorus contents were observed in L10I80 (5060 ± 31 mg/kg) and L0I80 (5028 ± 28 mg/kg) treatments (Table ). It can be concluded that intake of plant nutrients was high when the water use efficiency was the highest. The highest nitrogen content (4.43 ± 0.32%) was observed in L10I100 treatment and the lowest (3.82 ± 0.20%) in L20I60 treatment. This trend was similar for phosphorus content of L20I60 treatment (3309 ± 53 mg/kg). Research findings have revealed that the uptake of plant nutrients such as nitrogen and phosphorus into the leaves will be more successful at full irrigation (I100) and I80 irrigation levels with a combination of L10 leonardite application, whereas the lowest effect was seen in deficit irrigation treatments with the highest leonardite application.
Table 7. Phosphorus and nitrogen uptake values (mean ± SD) of leaves.
Water and nutrients exist together in close association because plant-available nutrient ions are dissolved in soil solution and nutrient uptake of plants largely depends on water flow through the soil-root-shoot pathway. Leaf transpiration generates the tension necessary for the roots to absorb the essential solution, but in relatively dry soils, uptake of water and nutrients becomes progressively more difficult for plants. Low water potentials of the root environment decrease ion uptake and transport to shoots. In soil, smaller uptake may be resulted both from impairment of absorption processes in the root and from decreased mobility of ions and water in the soil (Marschner Citation1995; Mengel et al. Citation2001). In this study, L10 level was observed. Various studies on leonardite showed positive effects on the intake of plant nutrients. In addition, the results may obviously vary in conditions such as different plant types, amount of organic matter, irrigation regimes, agricultural activities, climate, precipitation, root morphology, soil properties and fertilization. Increasing mineral contents under deficit irrigation conditions in this study could be explained by increasing soil solute concentrations with water deficiency (Bhardwaj Citation2012). However, Sahin et al. (Citation2016) and Kuslu et al. (Citation2008) determined higher macro and micro element contents for lettuce in full-irrigation conditions. According to Akıncı and Lösel (Citation2012), although many studies conducted on different plants stated that water stress mostly caused a reduction in mineral uptake, some of them also reported increased mineral uptakes with increasing water stress.
Conclusion
Considering all applications, as the leonardite levels and water shortage increased, the amount of water consumption decreased. The lowest water consumption was calculated for L20I60 treatment. Present findings revealed that water consumption decreased with increasing leonardite levels. WUE was higher in pots with full irrigation (I100) and 20% water deficit (I80) treatments of L0, L5 and L10 leonardite levels, but there was a decrease in water use efficiency of L20 level (the highest leonardite level). Research findings showed that foliar uptake of plant nutrients such as nitrogen and phosphorus was more successful at full irrigation (I100) and I80 irrigation levels with L10 leonardite application. Increasing plant nutrient uptakes were seen at high water use efficiencies. It was concluded based on present findings that the most successful application was L0I100 for yield and L10I80 for WUE and plant nutrient uptake. Increasing water holding capacities and decreasing water consumption were observed with increasing leonardite levels. Thus, with leonardite treatments, significant water savings were achieved and contributions were made to more efficient use of water. This study reveals the necessity of providing/making and determining the application conditions very well. The use of leonardite as a soil conditioner is more appropriate. In addition, it is necessary to determine the effect of leonardite on soil and plant growth over many years with appropriate alternation systems. It is thought that not sufficient to use organic fertilizer application alone to the soil during the growing season for especially different plants, and necessary to work in chemical fertilizer and chemical + organic fertilizer combination with applications. Moreover, the adaptation options include considerable cost and require economic evaluations.
Acknowledgement
The author is grateful to Prof. Dr. Zeki Gokalp (a certified English translator and an expert in Biosystems Engineering) for his critical reading and through syntactic corrections of the manuscript.
Data availability statement
The data that support the findings of this study are openly available in figshare.com at https://doi.org/10.6084/m9.figshare.17693783.
Disclosure statement
No potential conflict of interest was reported by the author(s).
Additional information
Funding
References
- Abd El-Wahed MH, Baker GA, Ali MM, Abd El-Fattah FA. 2017. Effect of drip deficit irrigation and soil mulching on growth of common bean plant, water use efficiency and soil salinity. Sci Hortic. 225:235–242.
- Acar B, Paksoy M, Türkmen Ö, Seymen M. 2008. Irrigation and nitrogen level affect lettuce yield in greenhouse condition. Afr J Biotechnol. 7(24):4450–4453.
- Afzal M, Battilani A, Solimando D, Ragab R. 2016. Improving water resources management using different irrigation strategies and water qualities: field and modelling study. Agric Water Manag. 176:40–54.
- Akinremi OO, Janzen RL, Lemke RL, Larney FJ. 2000. Response of canola, wheat and green beans to leonardite additions. Can J Soil Sci. 80:437–443.
- Akıncı Ş, Lösel DM. 2012. Plant water-stress response mechanisms. In I.M. Rahman, H. Hasegawa, editors. Water stress. Rijeka, Croatia: InTech Europe; p. 15–42.
- Alenazi M, Wahb-Allah MA, Abdel-Razzak HS, Ibrahim AA, Alsadon A. 2016. Water regimes and humic acid application influences potato growth, yield, tuber quality and water use efficiency. Am. J. Potato Res. 93(5):463–473.
- Ali MGM, Ibrahim MM, El Baroudy A, Fullen M, Omar EH, Ding Z, Kheir AMS. 2020. Climate change impact and adaptation on wheat yield, water use and water use efficiency at North Nile Delta. Front Earth Sci. 14(3):522–536.
- Bae J, Dall’erba S. 2018. Crop production, export of virtual water and water-saving strategies in Arizona. Ecol Econ. 146:148–156.
- Bhardwaj ML. 2012. Effect of climate change on vegetable production in India. In M.L. Bhardwaj, H.D. Sharma, M. Kumar, et al., editors. Vegetable production under changing climate scenario. Solan: Dr. Y. S. Parmar University of Horticulture and Forestry; p. 1–12.
- Bozkurt S, Mansuroğlu GS, Kara M, Önder S. 2009. Responses of lettuce to irrigation levels and nitrogen forms. Afr J Agric Res. 4(11):1171–1177.
- Brempong MB, Norton U, Norton JB. 2019. Compost and soil moisture effects on seasonal carbon and nitrogen dynamics, greenhouse gas fluxes and global warming potential of semi-arid soils. Int J Recycl Org Waste Agric. 8(1):367–376.
- Calvo P, Nelson L, Kloepper JW. 2014. Agricultural uses of plant biostimulants. Plant Soil. 383:3–41.
- Capra A, Consoli S, Russo A, Scicolone B. 2008. Integrated agroeconomic approach to deficit ırrigation on lettuce crops in Sicily (Italy). J Irrig Drain Eng. 134(4):437–445.
- Chala M, Yohannes KW. 2015. Effect of irrigation application levels on yield and water productivity of drip irrigated lettuce (Lactuca sativa L.), Gedio Zone. Southern Ethiopia. Int J Appl Sci. 4(4):229–234.
- Chen Y, Aviad T. 1990. Effects of humic substances on plant growth. In: MacCarthy P, editor. Humic substances in soil and crop science: selected readings. Madison: American Society of Agronomy and Soil Science Society of America; p. 161–186.
- Çivit B. 2010. Bazı doğal maddelerin (gidya, zeolit ve leonardit) marulda (lactuca sativa l.var longifolia) verim ve büyüme üzerine etkisi. Kahramanmaraş Sütçü İmam Üniversitesi Fen Bilimleri Enstitüsü Bahçe Bitkileri Anabilim Dalı Yüksek Lisans Tezi. Kahramanmaraş.
- Demir M, Noyan ÖF, Oğuz İ. 2012. Leonardit kullanımı ile birlikte azaltılmış azotlu gübre uygulamalarının bitki verim ve toprak özellikleri üzerine etkileri. Orta Karadeniz Geçit Kuşağı Tarımsal Araştırma İstasyonu Müdürlüğü. Gaziosmanpaşa Üniversitesi, Ziraat Fakültesi, Toprak Bölümü- SAÜ Fen Edebiyat Dergisi (2012-1). Tokat.
- Ding Z, Ali EF, Elmahdy AM, Ragab KE, Seleiman MF, Kheir AMS. 2021. Modeling the combined impacts of deficit irrigation, rising temperature and compost application on wheat yield and water productivity. Agric Water Manag. 224:1–12.
- Ding Z, Kheir AMS, Ali MGM, Ali OAM, Abdelaal AIN, Lin X, Zhou Z, Wang B, Liu B, He Z. 2020. The integrated effect of salinity, organic amendments, phosphorus fertilizers, and deficit irrigation on soil properties, phosphorus fractionation and wheat productivity. Sci Rep. 10:2736.
- Erice G, Louahlia S, Irigoyen JJ, Sanchez-Diaz M, Alami IT, Avice JC. 2011. Water use efficiency, transpiration and net CO2 exchange of four alfalfa genotypes submitted to progressive drought and subsequent recovery. Environ Exp Bot. 72(2):123–130.
- Erkoç İ. 2009. Sera domates yetiştiriciliğinde kükürt ve leonardit uygulamalarının fosfor yarayışlılığına etkileri. Adana: Çukurova Üniversitesi Fen Bilimleri Enstitüsü Yüksek Lisans Tezi.
- FAOSTAT. 2020. The total production of lettuce and chicory by Turkey. Retrieved on Febuary 06, 2020 from: http://www.fao.org/faostat/en/#data/QC/visualize.
- Gallardo M, Jackson LE, Schulbach K, Snyder RL, Thompson RB, Wyland LJ. 1996. Production and water use in lettuces under variable water supply. Irrig Sci. 16:125–137.
- Garcia MV, Estrella FS, Lopes M, Moreno J. 2008. Influence of compost amendment on soil biological properties and plants. Dyn Soil Dyn Plant. 1:1–9.
- Gianino D, Malladi A, van Iersel MW. 2015. Water use efficiency of four types of lettuce (Lactuca sativa) in response to different drought severities. ASHS 2015 Annual Conference; New Orleans, 4–7 August 2015.
- Hartz T, Bottoms T. 2010. Humic substances generally ineffective in improving vegetable crop nutrient uptake or productivity. Hortic Sci. 45:906–910.
- Hassanpanah D, Khodadadi M. 2009. Evaluation of potassium humate effects on germination, yield and yield components of HPS-II/67 hybrid true potato seeds under in vitro and in vivo conditions. Am J Plant Physiol. 4:52–57.
- Kaçar B. 2014. Bitki, toprak ve gübre analizleri 2, kolay uygulanabilir bitki analizleri. Nobel Akademik Yayıncılık, Yayın No:910, Ankara, ISBN:978-605-133-812-5.
- Karam F, Mounzer O, Sarkis F, Lahoud R. 2002. Yield and nitrogen recovery of lettuce under different irrigation regimes. J Appl Hortic. 4(2):70–76.
- Ket P, Garré S, Oeurng C, Hok L, Degré A. 2018. Simulation of crop growth and water-saving irrigation scenarios for lettuce: a monsoon-climate case study in Kampong Chhnang, Cambodia. Water (Basel). 10(5):666.
- Kirda C. 2002. Deficit irrigation scheduling based on plant growth stages showing water stress tolerance. In Deficit irrigation practices, water reports. Vol. 22. Rome: Food and Agriculture Organization of the United Nations; p. 3–10.
- Kırnak H, Demir S, Tas İ, Çakmaklı M. 2002. Response of different irrigation water applications on yield and growth of lettuce grown in greenhouse. J Agric Fac Harran Univ. 6:47–54.
- Klute A. 1986. Water retention: laboratory methods. In A. Klute, editor. Methods of soil analysis, Part 1, Physical and mineralogical methods. Madison, WI: Agronomy. Monograph. American Society of Agronomy and Soil Science Society of America; p. 635–662.
- Kögler F, Söffker D. 2017. Water (stress) models and deficit irrigation: system-theoretical description and causality mapping. Ecol Modell. 361:135–156.
- Kolay B, Gürsoy L S, Avşar Ö, Bayram N, Öztürkmen AR, Aydemir S, Aktaş H. 2016. Buğday bitkisine uygulanan farklı miktarlarda leonarditin bazı toprak özelliklerine etkisi. Toprak Su Dergisi. 5(2):32–36.
- Křístková E, Doležalová I, Lebeda A, Vinter V, Novotná A. 2008. Description of morphological characters of lettuce (Lactuca sativa L.) genetic resources. Hortic Sci. 35:113–129.
- Kurunc A, Unlukara A, Cemek B. 2011. Salinity and drought affect yield response of bell pepper similarly. Acta Agric Scand, Sect B. 61:514–522.
- Kuslu Y, Dursun A, Sahin U, Kiziloglu FM, Turan M. 2008. Short communication. Effect of deficit irrigation on curly lettuce grown under semiarid conditions. Span J Agric Res 6:714–719.
- Lopez JR, Winter JM, Elliott J, Ruane AC, Porter C, Hoogenboom G. 2017. Integrating growth stage deficit irrigation into a process based crop model. J Agric Meteorol. 243:84–92.
- Maraseni TN, Mushtaq S, Reardon-Smith K. 2012. Integrated analysis for a carbon-and water-constrained future: an assessment of drip irrigation in a lettuce production system in eastern Australia. J Environ Manag 111:220–226.
- Marschner H. 1995. Mineral nutrition of higher plants, 2nd ed. London: Academic Press.
- Mengel K, Kirkby EA, Kosegarten H, Appel T. 2001. Principles of plantnutrition. Dordrecht: Kluwer Academic.
- Michelon N, Pennisi G, Myint NO, Orsini F, Gianquinto G. 2020. Strategies for improved water use efficiency (WUE) of field-grown lettuce (Lactuca sativa L.) under a semi-arid climate. Agronomy. 10(5):668.
- Moghadam HT, Khamene MK, Zahedi H. 2014. Effect of humic acid foliar application on growth and quantity of corn in irrigation withholding at different growth stages. Maydica. 59:124–128.
- Molina-Montenegro MA, Zurita-Silva A, Oses R. 2011. Effect of water availability on physiological performance and lettuce crop yield (Lactuca sativa). Cien Inv Agr. 38:65–74.
- Moreira MA, Dos Santos CAP, Lucas AAT, Bianchini FG, De Souza IM, Viégas PRA. 2014. Lettuce production according to different sources of organic matter and soil cover. Agric Sci. 5:99–105.
- Oğuz İ, Karaş E, Erşahin S, Susam T. 2016. Land Degradation in the Çelikli Basin, Turkey. In Abiud Kaswamila, editor. Land degradation and desertification – a global crisis. London: IntechOpen Book Series; p. 93–114. doi:10.5772/64624.
- Patanè C, Tringali S, Sortino O. 2011. Effects of deficit irrigation on biomass, yield, water productivity and fruit quality of processing tomato under semi-arid Mediterranean climate conditions. Sci Hortic. 129:590–596.
- Rolbiecki R, Rolbiecki S. 2007. Effects of micro-irrigation systems on lettuce and radish production. Acta Hortic. 729:331–335.
- Sahin U, Kuslu Y, Kiziloglu FM, Cakmakci T. 2016. Growth, yield, water use and crop quality responses of lettuce to different irrigation quantities in a semi-arid region of high altitude. J Appl Hortic. 18(3):195–202.
- Silva VPR, Tavares AL, Sousa IF, Silva TGF, Holanda RM, Souza EP, Silva BB, Braga CC, Almeida RSR. 2018. Evapotranspiration, water use efficiency and crop coefficient of three lettuce varieties grown in a tropical region. Rev Fac Cienc Agra. 41(3):798–805.
- Stevenson FJ. 1994. Humus chemistry: genesis, composition, and reactions. New York: John Wiley.
- Torun B. 2009. Tarla koşullarında gyttja uygulamasının tahılların Dane verimine ve toprağın fiziksel ve kimyasal özelliklerine etkisi. Harran Üniversitesi, Z.F.Dergisi. 13(3):60–72.
- Ünlükara A, Kurunç A, Kesmez GD, Yurtseven E, Suarez DL. 2010. Effects of salinity on eggplant (Solanum melongena L.) growth and evapotranspiration. Irrig Drain. 59:203–214.
- Yang C, Luo Y, Sun L, Wu N. 2015. Effect of deficit irrigation on the growth, water use characteristics and yield of cotton in arid northwest China. Pedosphere. 25:910–924.
- Yazgan S, Ayas S, Demirtas C, Büyükcangaz H, Candogan BN. 2008. Deficit irrigation effects on lettuce (Lactuca sativa var. Olenka) yield in unheated greenhouse condition. J Food Agric Environ. 6:357–361.
- Yılmaz E, Alagöz Z, Öktüren F. 2008. Farklı organik materyal uygulamalarının toprak agregatları üzerine etkisi. Akdeniz Univ Ziraat Fak Derg. 21(2):213–222.
- Zandonadi DB, Santos MP, Dobbss LB, Olivares FL, Canellas LP, Binzel ML. 2010. Nitric oxide mediates humic acids-induced root development and plasma membrane H+-ATPase activation. Planta. 231:1025–1030.