Abstract
Mesenchymal stem cells (MSCs) show high expression of PD-L1. Exosomes can transmit signals between tumor cells and stromal cells; however, the effect of tumor-derived exosomes on stromal cell signaling pathways is unclear. The expression of PD-L1 in bone marrow mesenchymal stem cells (BMMSCs) was detected by western blot and RT-PCR. The effects of gastric cancer cell line-derived exosomes on BMMSC migration and the effects of conditioned medium from educated BMMSCs on tumor cell migration were analyzed. Our results demonstrate that exosomes derived from gastric cancer cell lines activate the AKT/c-Myc pathway of BMMSCs and upregulate PD-L1 expression on BMMSCs. Moreover, the pro-migration effect of exosomes from gastric cancer cell lines on BMMSCs is dependent on PD-L1. The upregulation of PD-L1 on BMMSCs promoted the migration of gastric cancer cells. In conclusion, tumor-derived exosomes could upregulate the expression of PD-L1 on BMMSCs to promote tumor migration. Our results provide a new therapeutic avenue in gastric cancer.
Introduction
Gastric cancer (GC) is the fifth most common cancer and the third leading cause of cancer death in the world (So et al. Citation2021). The expression of PD-L1 in gastric cancer has biological significance and is associated with poor prognosis and drug resistance (Chen et al. Citation2021; Wu et al. Citation2021). In addition, PD-L1 also plays a carcinogenic role since it causes proliferation and metastasis of gastric cancer cells (Mu et al. Citation2021). Recent studies indicate that miR-1290 in extracellular vesicle (EV) secreted by GC cells is involved in immune escape through the GRHL2 /ZEB1/PD-L1 axis (Liang et al. Citation2020). Macrophages induce tumor cells to express PD-L1, which helps GC tumor cells escape killing by cytotoxic T cells and promotes the proliferation of GC tumor cells (Ju et al. Citation2020). Our previous work revealed that mesenchymal stem cells (MSCs) upregulate PD-L1 expression in gastric cancer cells and promote the growth of GC (Chen et al. Citation2018; Sun et al. Citation2018; Sun et al. Citation2020).
The tumor microenvironment (TME) plays an important role in the development of gastric cancer (Zhang et al. Citation2020). PD-L1 is expressed on a variety of cells in the TME. One previous study has shown that tumor-associated macrophages express PD-L1, and that intraepithelial memory T-cell infiltration is inversely correlated with PD-L1 expression (Pan et al. Citation2021). Another study has shown that PD-L1 expression is upregulated in human tumor-associated macrophages after chemotherapy (Shirinbak et al. Citation2020). The increased expression of PD-L1 in dendritic cells significantly inhibits the proliferation and activation of lymphocytes (Li et al. Citation2021). It has been reported that the expression of PD-L1 in tumor-infiltrating myeloid cells contributes to the immunosuppressive characteristics of the tumor environment (Antonangeli et al. Citation2020). Surprisingly, PD-L1 was found to be more highly expressed in stromal cells than in tumor cells (Ghoshdastider et al. Citation2021). IL-17 can induce bone marrow MSCs (BMMSCs) to express PD-L1 through inducible nitric oxide synthase, thus shaping the immunosuppressive TME and promoting the development of tumors (Wang et al. Citation2020). MSCs have different regulatory effects on different phenotypes of T cells through PD-1/PD-L1 interaction between MSCs and T cells (Liu et al. Citation2020). For example, in the presence of IFN-γ and TNF-α, the expression of PD-L1 is upregulated on the surface of MSCs infiltrated by prostate cancer, inhibiting the proliferation of T cells (Krueger et al. Citation2018).
Tumor cells can produce exosomes that affect differentiation and metastasis of cancer-associated fibroblasts (Jena and Mandal Citation2020). Tumor-derived exosomes can reprogram MSCs and transform their functional profile from normally trophic to pro-tumorigenic (Whiteside Citation2018). It is amazing that tumor cell-derived exosomes confer BMMSCs with the ability to enhance tumor growth (Lin et al. Citation2016). The study by Haga et al. (Citation2015) examined the role of EV-mediated intercellular signaling between BMMSCs and tumor cells in human cholangiocarcinoma, and found that tumor-derived EVs can promote the production of tumor stroma through fibroblast differentiation of MSCs. However, the regulatory mechanism and role of PD-L1 on the surface of BMMSCs remain unclear.
In this study, tumor-derived exosomes were applied to BMMSCs to explore their function in stromal cells and tumor cells. Our results show that tumor-derived exosomes upregulate PD-L1 expression on BMMSCs and promote the migration of bone marrow stromal stem cells and tumor cells. In conclusion, tumor-derived exosomes promote gastric cancer cell migration through the upregulation of PD-LI expression on BMMSCs. This provides a novel strategy for the treatment of gastric cancer.
Materials and methods
Cell culture
Human BMMSCs were obtained from healthy donors and GC tissues were obtained from GC patients treated at the Affiliated People’s Hospital of Jiangsu University (Zhenjiang, Jiangsu, China). This research protocol was approved by the Ethics Committee of Jiangsu University. In addition, all donors and patients gave informed consent. The bone marrow was gently mixed with an equal-volume of PBS and slowly superimposed over the Ficoll separation solution, then, centrifuged at 800g rising and falling slowly for 20 min. The middle white film layer was carefully absorbed and then washed in equal-volume of PBS, and cultured in MEM-ALPHA (Biological Industries, Israel) containing 10% fetal bovine serum (FBS, Biological Industries) at 37°C with 5% CO2. The adherent cells obtained 4 days later were BMMSCs and were passaged up to six times. Gastric cancer MSCs (GCMSCs) were isolated from human GC tissues, as previously described (Yang et al. Citation2018).
The human gastric cancer cell lines HGC-27, SGC-7901, and MGC-803 were purchased from the Chinese Academy of Sciences Type Culture Collection Committee Cell Bank (Shanghai, China) and were maintained in RPMI-1640 (Biological Industries) with 10% FBS at 37°C in 5% CO2.
Isolation and characterization of gastric cancer cell-derived exosomes
When the GC cells were fused to 90%, the RPMI-1640 medium containing 10% exosome-free FBS (FBS was centrifuged at 100,000g for 10 min) was replaced, and we collected supernatant that had been cultured for 48 h. The cell culture supernatant was collected by centrifugation at 300g for 10 min to remove the cells, 2000g for 20 min to remove larger cell fragments, and 10,000g for 30 min to remove small cell fragments so as to prevent blockage of filter pores. Next, the supernatant was concentrated with a 100 kDa molecular weight cut-off hollow fiber membrane (Millipore, MA, USA) at 100,000g to pellet the exosomes. The final concentrate was mixed with ExoQuick-TC exosome isolation reagent (System Biosciences, CA, USA) in an EP tube overnight at 4°C. On the second day, the mixed concentrate was centrifuged for the last time at 1500g for 30 min. In the end, the final pellet containing exosomes was re-suspended in PBS and stored at −80°C. The total exosomal protein concentrations were determined by using the BCA Protein Assay kit (CWBIO, Beijing, China).
For characterization of tumor-derived exosomes, nanoparticle tracking analysis was used to analyze the particle size of exosomes. Western blot was performed to detect surface markers of exosomes, such as CD9, CD81, and CD63. The morphology of exosomes was observed using transmission electron microscopy.
Western blot analysis
Cellular or exosomal proteins were extracted with RIPA lysis buffer (Invitrogen, Carlsbad, CA, USA). After the protein concentration was determined, 40 µg protein was isolated by 10% sodium dodecyl sulfate polyacrylamide gel electrophoresis and then the protein was transferred to poly-vinylidene fluoride membranes (Millipore). Membranes were then blocked with 5% non-fat milk and incubated with CD63 (1:300; Cwbio), CD9 (1:600, Cwbio), CD81 (1:600, Cwbio), c-Myc (1:1000, CST), PD-L1 (1:1000, CST), GAPDH (1:2000, Cwbio), Actin (1:1000, CST), p-AKT (1:1000, CST), AKT (1:1000, CST), p-ERK (1:1000, CST), and ERK (1:1000, CST) antibodies overnight at 4°C and then incubated with goat anti-mouse (International, 1:2000; CW0102, CWBIO, Beijing, China) and goat anti-rabbit (dilution, 1:3000; CW0103, CWBIO) secondary antibodies for 1 h at 37°C. Finally, the blots were visualized using the enhanced chemiluminescent detection system (Amersham, Amersham, UK) and analyzed using Image-Pro Plus version 5.1 (Media Cybernetics Inc., Rockville, MD, USA). The protein was quantified by Image J.
Flow cytometry
Cells were digested to a single cell suspension for staining and washed with PBS. Cells were then resuspended with 200 µL PBS and incubated with CD29-PE, CD105-PE, CD90-PE, CD19-FITC,CD34-FITC, or CD45-FITC (eBioscience, USA) for 30 min on ice, then mixed gently every 10 min. Finally, the suspension was washed with PBS and detected by flow cytometry. Data were acquired by a flow cytometer (FACS Calibur; BD Biosciences)
Adipogenic and osteogenic differentiation
Cells were plated in 35 mm × 10 mm plates and cultured in adipogenic or osteogenic differentiation medium (Biological Industries). Ten days later, the cells were stained using the corresponding adipo-staining kit or osteo-staining kit (Biological Industries) to observe the potential for adipogenesis and osteogenesis.
RNA extraction and RT-qPCR assay
Total RNA was isolated by using TRIzol reagent (Invitrogen). cDNA was synthesized using a qRT-PCR kit (CWBIO) and qRT-PCR was performed using the UltraSYBR Mixture (CWBIO). The sequences for PD-L1 primers were as follows: forward, 5′-TCACTTGGTAATTCTGGGAGC-3′, PD-L1 reverse, 5′-CTTTGAGTTTGTATCTTGGATGCC-3′.
Preparation of MSC-conditioned medium
MSCs were cultured in six-well (8 × 104/well) plates and treated with or without exosomes when the culture reached approximately 70% confluency, after which the culture medium was harvested at 96 h. The conditioned medium (CM) was centrifuged at 800g for 5 min, and then filtered through a 0.22 µm membrane (Millipore, Germany) and stored at −80°C.
PD-L1 knockdown. PD-L1 small interfering RNA (siRNA, 50 nM) (sense: 5′-GGCACAUCCUCCAAAUGAATT-3′, antisense 5′-UUCAUUUGGAGGAUGUGCCTT-3′) and negative control (NC, 50 nM) (sense:5′-UUCUCCGAACGUGUCACGUTT-3′), antisense (5′-ACGUGACACGUUCGGAGAATT-3′) were purchased from Suzhou Gene Pharma. siRNA were transfected into BMMSCs with Lipofectamine® 2000 reagent (Invitrogen; Thermo Fisher Scientific Inc.) according to the manufacturer’s instructions.
Migration assay
For HGC-27 migration assays, 6 × 104 cells were diluted in 200 µL of serum-free RPMI-1640 and seeded into the upper compartment of Transwell assay filters (24-well plates with a pore size of 8.0 µm; Corning). Then, 300 µL of RPMI-1640 supplemented with 10% FBS mixed with 300 µL was added to the bottom, and cells were incubated for 10 h at 37°C with 5% CO2. For HGC-27 migration assays, 6 × 104 cells were diluted in 200 µL of serum-free MEM-ALPHA and seeded into the upper compartment, after which 600 µL of MEM-ALPHA supplemented with 10% FBS was added to the bottom, and cells incubated for 12 h at 37°C with 5% CO2. Cotton swabs were used to remove the cells that did not migrate. The migrated cells were fixed with 4% paraformaldehyde for 30 min, stained with crystal violet for 3 min, and imaged.
Statistical analysis
All data analyses were performed using GraphPad Prism 6 (Graph Software, La Jolla, CA, USA). Significance was tested by the Student’s t test or one-way ANOVA test. Results was considered to be statistically significant when P value < 0.05.
Results
Exosomes derived from GC cells were extracted and identified, and MSCs were isolated
First the exosomes isolated from HGC-27 tumor cells were characterized. The size of the separated exosomes was uniformly distributed at about 130 nm by nanoscale particle tracking analysis (Figure (A)). Western blot showed that CD9, CD63, and CD81 were expressed on SGC-7901 and HGC-27-derived exosomes (Figure (B)). The morphology of exosomes observed by transmission electron microscopy conformed to typical cup-shaped spherical vesicles (Figure (C)). BMMSCs appeared fusiform and grew from clumps of cells (Figure (D)). GCMSCs were tissue-centered, grew radially, and were long spindle-shaped (Figure (E)). Differentiation assays showed that BMMSCs and GCMSCs differentiated into adipocytes and bone cells successfully (Figure (F,G)). Immunophenotypic analysis of BMMSCs and GCMSCs showed positive expression of CD105, CD90, and CD29, and negative expression of CD45, CD34, and CD19 (Figure (H)).
Figure 1. Identification of exosomes in gastric cancer (GC) cells and primary culture of cells. (A) Diameter ranges of GC-derived exosomes. (B) Protein expression of CD9, CD63, and CD81 in GC-derived exosomes was detected by western blot. (C) Transmission electron photomicrograph of GC-derived exosomes. (scale bar: 100 nm). (D) Primary culture of bone marrow mesenchymal stem cells (BMMSCs) (scale bar: 100 µm). (E) Primary culture of gastric cancer mesenchymal stem cells (GCMSCs) (scale bar: 100 µm) (F) Adipogenic and osteogenic differentiation of BMMSCs (scale bar: 100 µm). (G) Adipogenic and osteogenic differentiation of GCMSCs (scale bar: 100 µm). (H) Flow cytometry analysis of the surface markers on BMMSCs and GCMSCs (EX: exosome).
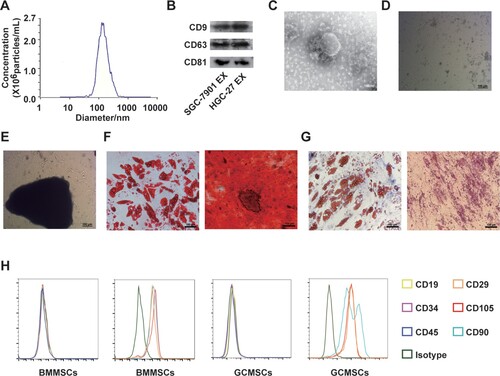
Tumor exosomes increased PD-L1 expression on of BMMSCs via upregulation of AKT/c-Myc
HGC-27-derived exosomes upregulated PD-L1 on BMMSCs (Figure (A)). The statistical analysis is shown in Figure (B). Western blot was used to detect the expression of different GC cell lines and the exosomes they secreted. The results showed that GC cell-derived exosomes carried PD-L1 weakly, even though the cells overexpressed PD-L1 (Figure (C)). Therefore, we hypothesized that exosomes upregulated PD-L1 expression on BMMSCs by activating internal signaling pathways. We showed that p-AKT, c-Myc, and PD-L1 were upregulated, but there was no difference in p-ERK expression (Figure (D)). The statistical analysis is shown in Figure (E). Western blot analysis verified that p-AKT, c-Myc, and PD-L1 were upregulated on BMMSCs after treatment with exosomes using different GC cell line sources (Figure (F)). The statistical analysis is shown in Figure (G). To further verify that exosomes upregulate PD-L1 expression on BMMSCs by the AKT/c-Myc pathway, we used the c-Myc inhibitor JQ1, AKT inhibitor MK2206, and PD-L1 antibody to effectively inhibit AKT/c-Myc signaling on BMMSCs, as well as exosome-induced upregulation of PD-L1 on BMMSCs (Figure (H)). The statistical analysis is shown in Figure (I). The results of quantitative PCR (Figure (J)) were consistent with those of western blotting. Both the protein and mRNA levels showed that gastric tumor-derived exosomes upregulated PD-L1 expression on BMMSCs through the AKT/c-Myc pathway.
Figure 2. Exosomes derived from gastric cancer (GC) cells promote PD-L1 expression on BMMSCs via regulation of the pAKT/c-Myc pathway. (A) Western blot was used to detect the expression of PD-L1 on BMMSCs after treatment with or without exosomes and GCMSCs. (B) Quantification of PD-L1 protein changes. (C) Western blot was used to detect the expression of PD-L1 and CD81 in cell lysates or exosomes. (D) Western blot was used to detect the expression of p-AKT, AKT, p-ERK, ERK c-Myc, and PD-L1 on BMMSCs. (E) Quantification of p-AKT, AKT, p-ERK, ERK c-Myc, and PD-L1 protein changes. (F) Western blot was used to detect the expression p-AKT, AKT, c-Myc, and PD-L1 on BMMSCs after treatment with HGC-27 EX or SGC-7901 EX and GCMSC. (G) Quantification of p-AKT, AKT, c-Myc, and PD-L1 protein changes. (H) Western blot was used to detect the expression p-AKT, AKT, c-Myc, PD-L1on BMMSCs after treatment with HGC-27 EX, HGC-27 EX + JQI, HGC-27 EX + MK2206, HGC-27 EX + PD-L1Ab, and GCMSCs for 96 h in vitro. (I) Quantification of p-AKT, AKT, c-Myc, and PD-L1 protein changes. (J) The expression of PD-L1 on BMMSCs after treatment with or without HGC-27 EX, HGC-27 EX + JQI, HGC-27 EX + MK2206, HGC-27 EX+ antiPD-L1 for 96 h in vitro was detected by RT-PCR. The data are expressed as means ± SD. *P < 0.05, **P < 0.01, ****P < 0.001 by one-way ANOVA (EX: exosome).
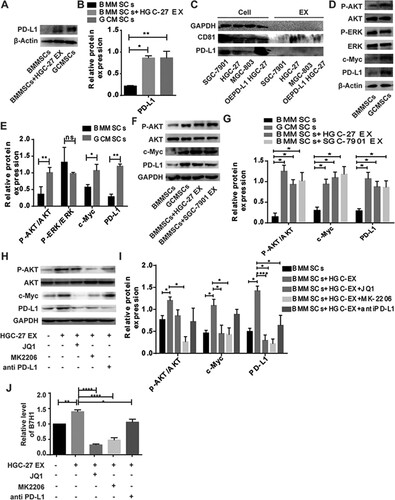
Migration ability of BMMSCs after tumor cell-derived exosome exposure
To examine the effect of gastric tumor cell-derived exosomes on BMMSC behavior, we next studied whether gastric tumor cell-derived exosomes could modulate the migratory ability of BMMSCs; for this, transwell cell migration assays were used. Incubation of BMMSCs with HGC-27-derived exosomes resulted in increased cell migration after 12 h compared to control MSCs that were not exposed to HGC-27-derived exosomes (Figure (A,B)). To evaluate the role of PD-L1, the expression of PD-L1 on BMMSCs was modulated with siRNA, and we selected the second interference fragment which was valid (Figure (C)). The statistical analysis is shown in Figure (D). Moreover, our results showed that after PD-L1 knockdown of BMMSCs, the pro-migration effect of HGC-27-derived exosomes on BMMSCs was inhibited (Figure (E,F)).
Figure 3. HGC-27-derived exosomes increase migration of BMMSCs. (A, B) The migratory ability of BMMSCs treated with or without exosomes were analyzed by transwell assay (scale bar: 500 µm). The data are expressed as means ± SD. ****P < 0.0001 by two-tailed Student’s t test. (C) PD-L1 protein levels on BMMSCs were analyzed by western blot after transfection with siRNA negative control (NC), siRNA1, siRNA2, or siRNA3. β-actin served as a loading control. (D) Quantification of PD-L1 protein changes. (E, F) The migratory ability of BMMSCs was analyzed by transwell assays. BMMSCs and siPD-L1BMMSCs treated with or without HGC-27 EX (scale bar: 500 µm). The data are expressed as means ± SD. ****P < 0.0001 by one-way ANOVA (EX: exosome; NC: negative control).
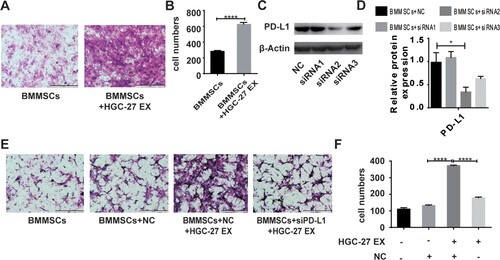
HGC-27 migration in response to BMMSCs exposed to tumor cell-derived exosomes
HGC-27 migration was evaluated using transwell migratory assays in response to incubation with CM from BMMSCs and BMMSCs exposed to exosome or cell-free media (Figure (A,B)). Cell migration was assessed after 12 h. Compared with control media, HGC-27 migration was increased by BMMSC-CM, especially BMMSC-CM obtained in the presence of tumor cell-derived exosomes. However, a weakened pro-migration effect of BMMSC-CM obtained in the presence of tumor cell-derived exosomes was found after interference of PD-L1 expression on BMMSCs (Figure (C,D)).
Figure 4. Conditioned medium from BMMSCs exposed to tumor cell-derived exosomes enhances tumor cell migration and depends on PD-L1. (A, B) The migratory ability of HGC-27 was analyzed by transwell assay. HGC-27 was treated with CM from MSCs and MSCs exposed to HGC-27 EX (scale bar: 200 µm). The data are expressed as means ± SD. ****P < 0.0001 by one-way ANOVA. (C, D) The migratory ability of HGC-27 was analyzed by transwell assay. HGC-27 was treated with CM from MSCs, MSCs exposed to HGC-27 EX, and siPD-L1 MSCs exposed to HGC-27 EX (scale bar: 200 µm). (E) Schematic diagram network of tumor-derived exosome-mediated PD-L1 upregulation of BMMSCs. The data are expressed as means ± SD. ****P < 0.0001 by one-way ANOVA (EX: exosome; CM: conditioned medium; NC: negative control).
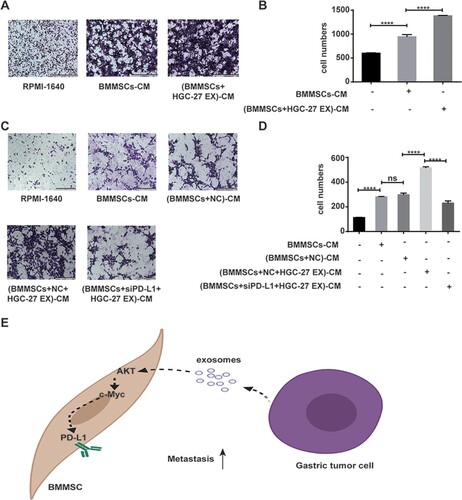
Discussion
MSCs are altered in the tumor microenvironment and promote tumor growth. Many studies have shown that MSCs can promote tumor migration (Waghray et al. Citation2016; Hochheuser et al. Citation2020; Lin et al. Citation2020). One study found that prostate cancer-derived exosomes dominate the differentiation process of MSCs, producing myofibroblasts with functional properties consistent with disease promotion (Haga et al. Citation2015). Another study showed that exosomes derived from tumor cells could affect the biological characteristics of MSCs (Shen et al. Citation2019). However, the mechanism of action of tumor exosomes on MSCs remains unclear. We speculated that exosomes affect cell function by altering the signaling pathways of MSCs.
PD-L1 can be expressed on the cell surface of tumor cells, immune cells, and other tumor microenvironment cells (Daassi et al. Citation2020). The increase in PD-L1 on the cell surface can inhibit anti-tumor immunity and promote tumor migration. Studies have shown that exosomes can carry PD-L1 to other cells and promote immune escape by tumors (Yang et al. Citation2018; Zhou et al. Citation2020). However, our study found that exosomes from gastric cancer cell lines carried little or no PD-L1. We found that exosome-treated BMMSCs can upregulate PD-L1, similar to GCMSCs. We hypothesized that exosomes may upregulate PD-L1 through the MSC signaling pathways. Recent studies have shown that activation of AKT, ERK, and c-Myc signals is associated with the regulation of PD-L1 (Liu et al. Citation2017; Li et al. Citation2018; Sun et al. Citation2018). Next, western blot was used to detect differences in the signaling pathways between BMMSCs and GCMSCs. The results showed that compared with BMMSCs, p-Akt, c-Myc, and PD-L1 were increased in GCMSCs, while there was no difference in p-ERK. In order to further explore the effect of exosomes from GC cells on the signaling pathway of BMMSCs, BMMSCs were treated with exosomes from different GC cell lines. It was found that exosomes from GC cells could upregulate p-Akt, c-Myc, and PD-L1. Therefore, we proposed that the exosomes derived from GC cells upregulate PD-L1 BMMSC by activating the AKT/c-Myc pathway.
A recent study has shown that exosomes derived from tumor cells can promote the proliferation of MSCs (Haga et al. Citation2015). Exosomes promote MSC migration in a time- and concentration-dependent manner (Sharma et al. Citation2018). However, the mechanism by which exosomes promote MSC migration is still unclear. Another study showed that the expression of PD-L1 on MSCs is related to migration ability (Wang et al. Citation2013). Moreover, MSCs can promote the migration of tumor cells (Lu et al. Citation2020). Therefore, we speculated that exosomes from gastric cancer cell lines could promote the migration of BMMSCs by upregulating the expression of PD-L1, thus enhancing the pro-tumor migration of BMMSCs. Our studies have shown that after interfering with the expression of PD-L1 on BMMSCs, the pro-migration effect of exosomes from tumor cell lines on BMMSCs was weakened. Since PD-L1 is a secretory molecule, it can also be expressed in the supernatant in addition to cell vesicles (Davies et al. Citation2017; Xu et al. Citation2022). In order to consider all the functions of PD-L1, conditioned medium of BMMSCs from different treatment groups were used; the results showed that BMMSC-CM can promote migration of HGC-27, and that this phenomenon was PD-L1-dependent. The expression of TNF-α is high in the exosomes secreted by cancer cells (Baglio et al. Citation2017) and its expression may also be related to the regulation of PD-L1. However the specific mechanism related to a TNF-α/PD-L1 axis is still unclear. Our results show that exosomes derived from gastric cancer cell lines can regulate the expression of PD-L1 on BMMSCs, thereby remodeling MSCs and increasing their tumor-promoting effect.
In conclusion, our study reveals the mechanism of exosome-mediated tumor-stromal cell interaction and contribution of this cell interaction to the growth of gastric cancer. This study shows that tumor cell-derived exosomes upregulate PD-L1 expression on BMMSCs by the AKT-c-Myc signal axis, and promote cell migration (Figure (E)). The BMMSCs educated by exosomes and their effects on GC cells provide a new treatment avenue for gastric cancer.
Data availability statement
All necessary experimental raw data were uploaded to Zenodo Database via link below https://doi.org/10.5281/zenodo.5808905.
Disclosure statement
No potential conflict of interest was reported by the author(s).
Additional information
Funding
References
- Antonangeli F, Natalini A, Garassino MC, Sica A, Santoni A, Di Rosa F. 2020. Regulation of PD-L1 expression by NF-kappaB in cancer. Front Immunol. 11:584626.
- Baglio SR, Lagerweij T, Perez-Lanzon M, Ho XD, Leveille N, Melo SA, Cleton-Jansen AM, Jordanova ES, Roncuzzi L, Greco M, et al. 2017. Blocking tumor-educated MSC paracrine activity halts osteosarcoma progression. Clin Cancer Res. 23:3721–3733.
- Chen B, Yu J, Wang Q, Zhao Y, Sun L, Xu C, Zhao X, Shen B, Wang M, Xu W, et al. 2018. Human bone marrow mesenchymal stem cells promote gastric cancer growth via regulating c-Myc. Stem Cells Int. 18:9501747.
- Chen L, Wang L, Li X, Zhang G, Li Z, Wang Y. 2021. Clinic-pathological characteristics and prognostic value of PD-L1 and HER2 in gastric cancer. DNA Cell Biol. 40:405–413.
- Daassi D, Mahoney K, Freeman GJ. 2020. The importance of exosomal PDL1 in tumour immune evasion. Nat Rev Immunol. 20:209–215.
- Davies LC, Heldring N, Kadri N, Le Blanc K. 2017. Mesenchymal stromal cell secretion of programmed death-1 ligands regulates T cell mediated immunosuppression. Stem Cells. 35:766–776.
- Ghoshdastider U, Rohatgi N, Mojtabavi Naeini M, Baruah P, Revkov E, Guo Y, Rizzetto S, Wong A, Solai S, Nguyen T, et al. 2021. Pan-cancer analysis of ligand-receptor crosstalk in the tumor microenvironment. Cancer Res. 81:1802–1812.
- Haga H, Yan IK, Takahashi K, Wood J, Zubair A, Patel T. 2015. Tumour cell-derived extracellular vesicles interact with mesenchymal stem cells to modulate the microenvironment and enhance cholangiocarcinoma growth. J Extracell Vesicles. 4:24900.
- Hochheuser C, van Zogchel LMJ, Kleijer M, Kuijk C, Tol S, van der Schoot CE, Voermans C, Tytgat GAM, Timmerman I. 2020. The metastatic bone marrow niche in neuroblastoma: altered phenotype and function of mesenchymal stromal cells. Cancers. 12:3231.
- Jena BC, Mandal M. 2020. The emerging roles of exosomes in anti-cancer drug resistance and tumor progression: An insight towards tumor-microenvironment interaction. Biochim Biophys Acta Rev Cancer. 1875:188488.
- Ju X, Zhang H, Zhou Z, Chen M, Wang Q. 2020. Tumor-associated macrophages induce PD-L1 expression in gastric cancer cells through IL-6 and TNF-α signaling. Exp Cell Res. 396:112315.
- Krueger TE, Thorek DLJ, Meeker AK, Isaacs JT, Brennen WN. 2018. Tumor-infiltrating mesenchymal stem cells: drivers of the immunosuppressive tumor microenvironment in prostate cancer? Prostate. 79:320–330.
- Li F, Zhu T, Yue Y, Zhu X, Wang J, Liang L. 2018. Preliminary mechanisms of regulating PDL1 expression in nonsmall cell lung cancer during the EMT process. Oncol Rep. 40:775–782.
- Li T, Ma R, Zhu J. 2021. Up-regulation of donor dendritic cell PD-L1 expression reduced recipient lymphocyte activation and proliferation in vitro. Transpl Proc. 53:716–723.
- Liang Y, Liu Y, Zhang Q, Zhang H, Du J. 2020. Tumor-derived extracellular vesicles containing microRNA-1290 promote immune escape of cancer cells through the Grhl2/ZEB1/PD-L1 axis in gastric cancer. Transl Res. 13:S1931–S5244.
- Lin LY, Du LM, Cao K, Huang Y, Yu PF, Zhang LY, Li FY, Wang Y, Shi YF. 2016. Tumour cell-derived exosomes endow mesenchymal stromal cells with tumour-promotion capabilities. Oncogene. 35:6038–6042.
- Lin W, Chang Y, Hong M, Hsu T, Lee K, Lin C, Lee J. 2020. STAT3 phosphorylation at Ser727 and Tyr705 differentially regulates the EMT-MET switch and cancer metastasis. Oncogene. 40:791–805.
- Liu S, Liu F, Zhou Y, Jin B, Sun Q, Guo S. 2020. Immunosuppressive property of MSCs mediated by cell surface receptors. Front Immunol. 11:1076.
- Liu Y, Marin A, Ejlerskov P, Rasmussen LM, Prinz M, Issazadeh-Navikas S. 2017. Neuronal IFN-beta-induced PI3K/Akt-FoxA1 signalling is essential for generation of FoxA1(+)Treg cells. Nat Commun. 8:14709.
- Lu M, Xie K, Lu X, Lu L, Shi Y, Tang YJ. 2020. Notoginsenoside R1 counteracts mesenchymal stem cell-evoked oncogenesis and doxorubicin resistance in osteosarcoma cells by blocking IL-6 secretion-induced JAK2/STAT3 signaling. Invest New Drugs. 39:416–425.
- Mu L, Wang Y, Su H, Lin Y, Sui W, Yu X, Lv Z. 2021. HIF1A-AS2 promotes the proliferation and metastasis of gastric cancer cells through miR-429/PD-L1 axis. Dig Dis Sci. 66:4314–4325.
- Pan C, Wang Y, Liu Q, Hu Y, Fu J, Xie X, Zhang S, Xi M, Wen J. 2021. Phenotypic profiling and prognostic significance of immune infiltrates in esophageal squamous cell carcinoma. Oncoimmunology. 10:1883890.
- Sharma S, Alharbi M, Kobayashi M, Lai A, Guanzon D, Zuniga F, Ormazabal V, Palma C, Scholz-Romero K, Rice GE, et al. 2018. Proteomic analysis of exosomes reveals an association between cell invasiveness and exosomal bioactivity on endothelial and mesenchymal cell migration in vitro. Clin Sci. 132:2029–2044.
- Shen Y, Xue C, Li X, Ba L, Gu J, Sun Z, Han Q, Zhao RC. 2019. Effects of gastric cancer cell-derived exosomes on the immune regulation of mesenchymal stem cells by the NF-kB signaling pathway. Stem Cells Dev. 28:464–476.
- Shirinbak S, Chan RY, Shahani S, Muthugounder S, Kennedy R, Hung LT, Fernandez GE, Hadjidaniel MD, Moghimi B, Sheard MA, et al. 2020. Combined immune checkpoint blockade increases CD8+CD28+PD-1+effector T cells and provides a therapeutic strategy for patients with neuroblastoma. Oncoimmunology. 10:1838140.
- So JBY, Kapoor R, Zhu F, Koh C, Zhou L, Zou R, Tang YC, Goo PCK, Rha SY, Chung HC, et al. 2021. Development and validation of a serum microRNA biomarker panel for detecting gastric cancer in a high-risk population. Gut. 70:829–837.
- Sun L, Huang C, Zhu M, Guo S, Gao Q, Wang Q, Chen B, Li R, Zhao Y, Wang M, et al. 2020. Gastric cancer mesenchymal stem cells regulate PD-L1-CTCF enhancing cancer stem cell-like properties and tumorigenesis. Theranostics. 10:11950–11962.
- Sun L, Wang Q, Chen B, Zhao Y, Shen B, Wang H, Xu J, Zhu M, Zhao X, Xu C, et al. 2018. Gastric cancer mesenchymal stem cells derived IL-8 induces PD-L1 expression in gastric cancer cells via STAT3/mTOR-c-Myc signal axis. Cell Death Dis. 9:928.
- Waghray M, Yalamanchili M, Dziubinski M, Zeinali M, Erkkinen M, Yang H, Schradle KA, Urs S, Di Magliano M P, Welling TH, et al. 2016. GM-CSF mediates mesenchymal-epithelial cross-talk in pancreatic cancer. Cancer Discov. 6:886–899.
- Wang G, Zhang S, Wang F, Li G, Zhang L, Luan X. 2013. Expression and biological function of programmed death ligands in human placenta mesenchymal stem cells. Cell Biol Int. 37:137–148.
- Wang S, Wang G, Zhang L, Li F, Liu K, Wang Y, Shi Y, Cao K. 2020. Interleukin-17 promotes nitric oxide-dependent expression of PD-L1 in mesenchymal stem cells. Cell Biosci. 10:73.
- Whiteside TL. 2018. Exosome and mesenchymal stem cell cross-talk in the tumor microenvironment. Semin Immunol. 35:69–79.
- Wu L, Cai S, Deng Y, Zhang Z, Zhou X, Su Y, Xu D. 2021. PD-1/PD-L1 enhanced cisplatin resistance in gastric cancer through PI3 K/AKT mediated P-gp expression. Int Immunopharmacol. 94:107443.
- Xu F, Fei Z, Dai H, Xu J, Fan Q, Shen S, Zhang Y, Ma Q, Chu J, Peng F, et al. 2022. Mesenchymal stem cell-derived extracellular vesicles with high PD-L1 expression for autoimmune diseases treatment. Adv Mater. 34:e2106265.
- Yang Y, Li CW, Chan LC, Wei Y, Hsu JM, Xia W, Cha JH, Hou J, Hsu JL, Sun L, et al. 2018. Exosomal PD-L1 harbors active defense function to suppress T cell killing of breast cancer cells and promote tumor growth. Cell Res. 28:862–864.
- Zhang B, Wu Q, Li B, Wang D, Wang L, Zhou YL. 2020. m(6)A regulator-mediated methylation modification patterns and tumor microenvironment infiltration characterization in gastric cancer. Mol Cancer. 19:53.
- Zhou K, Guo S, Li F, Sun Q, Liang G. 2020. Exosomal PD-L1: new insights into tumor immune escape mechanisms and therapeutic strategies. Front Cell Dev Biol. 8:569219.