Abstract
B7 family members and their receptors have been confirmed to participate in T cell response signaling through the induction of immunosuppressive effects. The V-domain Ig suppressor of T-cell activation (VISTA) is expressed in myeloid cells as well as T lymphocytes and plays a crucial role in immune responses. In this study, the phage display technique was employed to isolate specific antibodies targeting VISTA in chickens. In in vitro experiments, VISTA could trigger the inhibition of T-cell activation through interaction during binding. However, isolated single-chain variable fragments (scFvs) effectively inhibited immune cells from producing exhaustion responses, freeing the T cells in peripheral blood mononuclear cells from inhibition and allowing them to remain activated. In addition, the isolated scFvs can block the interaction between VISTA and the VSIG3 ligand. Further analysis on one scFv VS10 revealed that a significant insertion mutation occurred at the CDR3 of the VH domain compared to the chicken germline sequence. Finally, the complex structures of scFv VS10 and VISTA were simulated using molecular docking, and the interaction produced was analyzed. The experimental results support the applicability of VISTA as a target for immune checkpoints and contribute to explaining geometric structures and properties in chicken antibodies.
Introduction
domain–containing immunoglobulin suppressor of T-cell activation (VISTA), a type I immunoglobulin membrane protein, is also called PD-1H, DD1α, or GI24. A member of the B7 family, VISTA has an extracellular domain homologous to PD-L1 (Flajnik et al. Citation2012; Wang et al. Citation2011). Moreover, VISTA has the most conserved amino acid sequence among the B7 family members and exhibits a similarity of 76% between mice and humans (Wang et al. Citation2011). Human VISTA is primarily expressed in hematopoietic cells and myeloid cells; its expression levels in CD4+, CD8+, and regulatory T cells are lower. VISTA is barely expressed in B cells or NK cells, yet significant VISTA expression has been found in tissue-infiltrating lymphocytes (Wang et al. Citation2011; Lines et al. Citation2014). During immune response regulation, VISTA may act as both a ligand and a receptor. Research has revealed that using recombinant VISTA-Ig fusion protein could significantly inhibit T-cell activation and proliferation responses induced through anti-CD3 stimulation as well as reduce the secretion of cytokines interleukin-2 (IL-2) and interferon-γ (IFN-γ); these findings indicate that VISTA could be a negative checkpoint regulator of T-cell activation or function as a co-inhibitory ligand (Le Mercier et al. Citation2014). In a graft-versus-host disease mouse model, VISTA of T cell surfaces was bound through anti-VISTA antibodies could directly inhibit the T cells, reduce the expansion of donor T cells in the spleen and liver, and further increase the survival rate of mice (Flies et al. Citation2015). VISTA expression in cancer cells has rarely been studied. Mulati et al. found that VISTA was highly expressed in human ovarian cancer and endometrial cancer cells. Elevated VISTA expression in endometrial cancer is associated with the methylation status of the VISTA promoter (Mulati et al. Citation2019). VISTA is also expressed in human non-small-cell lung cancer and correlated with increased tumor-infiltrating lymphocytes (TIL) as well as the expression and outcome of PD-1 protein markers (Villarroel-Espindola et al. Citation2018). Treatment using anti-VISTA antibodies significantly reduced the growth of various solid tumors (such as melanoma and bladder carcinoma), probably because anti-VISTA treatment enhanced the proliferation of TILs and promoted T-cell activity and effect (Le Mercier et al. Citation2014). However, in terms of immune regulation, the mechanism of the partners that can be bound to VISTA remain to be determined. Recently, VSIG-3/IGSF11 was revealed to be a potential partner for VISTA and capable of inhibiting the function of human T cells through the VSIG-3/VISTA binding pathway (Wang et al. Citation2019). Thus, in cancer immunotherapy, blocking antibodies could block the action of VISTA on cancer cell surfaces bound to T cells or directly target VISTA expressed on T cells to prevent it from providing negative signals in immune regulation.
Antibodies are protein molecules produced in response to antigens. Given the ability of antibodies to bind to specific targets, they are extensively applied in diagnosis, therapy, and research. Compared with the traditional method of preparing monoclonal antibodies using hybridoma, which was cumbersome, difficult, and time-consuming (Groves and Morris Citation2000), the phage display technique is efficient, convenient, and rapid. Through this efficient in vitro screening method, a high-throughput screening method called panning allows for specific antibodies from a complicated library of phage antibodies to be enriched (Barbas et al. Citation1991). Currently, this technique is used clinically and in research for developing numerous antibodies as effective targeted therapy (Rahbarnia et al. Citation2017; Shim Citation2016). To induce antibody responses, small rodents such as mice are often used as animal models for immunization. However, many mammalian proteins have highly conserved amino acid sequences and easily build tolerance during immunization; thus, inducing high-valence immune responses is difficult, which may hinder antibody research. A potential solution is to use chickens as animal models for immunization. Given the phylogenetic distance between chickens and mammals and considering the highly conserved nature of mammalian proteins, highly specific immune responses can be effectively induced in chickens (Gassmann et al. Citation1990). Compared with general mouse-derived antibodies, chicken-derived antibodies generally have longer complementarity-determining regions (CDRs) and higher variability; these are advantageous for inducing effective somatic mutations, which produce a neutralizing effect in the face of antigens (Tsurushita et al. Citation2004; Tsai et al. Citation2018). Thus, chicken-derived antibodies—either the specific poly-IgY antibody produced by chickens or isolated monoclonal single-chain variable fragments (scFvs)—can be effectively applied clinically and in research (Cai et al. Citation2012; Rahman et al. Citation2013).
This study focused on VISTA, and highly specific chicken-derived scFv antibodies were prepared. In addition, this study verified the blockade function of the antibodies; the activation response of immune cells could be protected through binding to VISTA. This finding supported the feasibility of developing VISTA inhibitors for application in immunotherapy. In addition, through molecular modeling, the most rational antibody conformation was constructed and simulated by analyzing the structure of CDRs. Possible epitopes on VISTA molecules were analyzed; moreover, molecular docking was used to predict possible binding directions regarding antibodies and VISTA, and the interaction force likely to be produced between antibody CDR sequences and VISTA was explored. The findings of this study can serve as a reference for developing antibody related to the immune-checkpoint protein VISTA or for other clinical applications.
Materials and methods
Chicken scFv library construction
Female white leghorn (Gallus domesticus) chickens were purchased from the National Laboratory Animal Center, Taiwan, and maintained in the animal facility of the Taipei Medical University. The chickens were maintained carefully, and all animal experiments followed ethical standards. The protocols were reviewed and approved by the Animal Use and Management Committee of Taipei Medical University (IACUC number: LAC-2015-0312).
To generate the antibody gene library, 50 µg of recombinant VISTA extracellular fragment protein (Cat: 13482-H08H; Sino Biological, Inc.) was mixed with an adjuvant (Sigma-Aldrich, Inc.) to immunize female white leghorn chickens through intramuscular injection. The chickens were immunized four times; we used Freund’s Complete Adjuvant the first time and Freund’s Incomplete Adjuvant the remaining three times. Three additional immunizations were performed at intervals of 7 days. The spleens of the chickens were harvested 7 days after the final immunization to construct an scFv antibody library. The library construction method followed published protocols with minor modifications (Andris-Widhopf et al. Citation2000).
Biopanning
Before the experiment, the recombinant VISTA protein was coated in the well of a microtiter plate at 4°C overnight. After the VISTA protein was removed, the well was blocked with 3% bovine serum albumin at room temperature for 1 h. Next, the recombinant phage particles (1011) from the constructed scFv library were added to the well and incubated at room temperature for 2 h. Unbound phages were subsequently removed, and the well was washed through pipetting with PBST (phosphate-buffered saline [PBS] with 0.05% Tween 20) 10 times. Bound phages were eluted with 0.1 M HCl–glycine (pH 2.2)/0.1% bovine serum albumin elution buffer and neutralized with 2 M Tris-base buffer. Subsequently, the eluted phages were used for Escherichia coli strain ER2738 infection for recombinant phage amplification. Amplified phages were precipitated and recovered according to the described method and used in the next round of panning (Barbas et al. Citation1991). The panning procedure was repeated four times. Subsequently, total library DNA was purified and transformed into Escherichia coli strain TOP 10F’ (Invitrogen, a nonsuppressor strain) for scFv expression. The expressed scFvs were further purified with Ni2+-charged sepharose according to the manufacturer’s instructions (GE Healthcare Life Science, Inc.).
Sequence analysis
A designed primer ompseq (5’-AAGACAGCTATCGCGATTGCAGTG-3’) complementary to the outer membrane protein A (ompA) signal sequence in front of the light-chain variable region was used for scFv clone sequencing. The International ImMunoGeneTics information system/V-QUEry and STandardization (http://imgt.org) were used to compile and analyze the sequence data in accordance with the germline genes.
Enzyme-linked immunosorbent assay
The microtiter plate was coated with recombinant VISTA protein (0.5 µg/well) at 4°C overnight. After blocking with 3% BSA, the scFv or phage was added to the wells and incubated for 1 h at room temperature. The wells were washed with PBST, and the bound scFv or phage was then detected and developed using horseradish peroxidase (HRP)–conjugated goat antichicken light-chain antibodies (Bethyl Laboratories, Inc.) or HRP-conjugated anti-M13 antibodies (GE Healthcare Life Science, Inc.). Then, a 3,5,5-tetramethubezidine dihydrochloride (TMB) substrate was added for binding signal development. The reaction was stopped through the addition of 1 N HCl, and absorbance was measured at 450 nm.
The competitive binding assay was performed to determine the binding specificity of isolated scFvs to VISTA. Microtitre plates were coated with recombinant VISTA (0.2 µg/well) and blocked with 3% BSA. After one-hour incubation at room temperature, the plates were washed with PBST twice. Each scFv was incubated with various concentrations of soluble VISTA protein diluted in PBST (ranging from 0.1–100 µg/mL) at room temperature for 1 h. The mixtures were then added to the wells to react with coated VISTA protein. After being incubated at room temperature for 1 h, the plates were washed with PBST three times. Then, HRP- conjugated goat antichicken light chain antibodies were incubated at room temperature for 1 h to detect bound scFv protein. After being washed with PBST three times, TMB substrate was added to each well for development. The reaction was stopped with 1 N HCl, and signal intensity was measured at 450 nm.
T-cell activation assay
In the T-cell activation assay, we used plate-bound recombinant VISTA protein to inhibit of T-cell activation and proliferation. Before the experiment, 10 µg/mL of the VISTA protein was coated in 100 µL per well onto 96-well tissue culture plates at 4 °C overnight. The next day, the VISTA protein solution was removed, and 0.25 µg/mL of the anti-CD3 monoclonal antibodies (clone OKT3, eBioscience, Inc.) were coated in 100 µL per well for 2 h at room temperature. Once the anti-CD3 antibodies were removed, 1 × 105 peripheral blood mononuclear cells (PBMCs) from healthy people (Stemcell Technologies, Inc.) labeled with 0.5 µM carboxyfluorescein succinimidyl ester (CFSE) (eBioscience, Inc.) were distributed into each protein-coated well. Subsequently, the test scFvs were added to the final concentration of 10 or 100 µg/mL and incubated for a 5-day cell culture. Finally, T-cell activation was analyzed on the basis of the CFSE signals of the cells using flow cytometry.
Flow cytometry analysis
Human leukemia cell lines including K562, THP1, HL60, KG1, CCRFCEM, and Kasumi-1 were analyzed using commercial sheep anti-VISTA antibodies (Cat. AF7126; R&D Systems, Inc.). The binding reactivity was subsequently detected using commercial rabbit antisheep antibodies (Jackson ImmunoResearch Laboratories, Inc.) to observe the VISTA membrane protein expression. In another assay, the THP1 cells with endogenous VISTA protein expression were analyzed through flow cytometry to confirm the binding reactivity of scFvs. Freshly prepared cancer cells were harvested and washed twice with PBS before the scFv was added for the reaction. The bound scFv was visualized using goat antichicken light-chain antibodies (Bethyl Laboratories, Inc.) and then subsequently using donkey antigoat antibodies conjugated with fluorescein isothiocyanate (Jackson ImmunoResearch Laboratories, Inc.). A FACScan flow cytometer was used for analysis (BD Biosciences Systems & Reagents, Inc.).
Competitive inhibition assay
A competitive inhibition assay was used to determine the ability of scFv VS10 to block VISTA−VSIG3 interaction. In brief, the wells of microtiter plates were coated with human VISTA recombinant protein (0.5 µg/well). After blocking with 5% skim milk for 1 h at room temperature, the plates were washed twice with PBST. Human Fc-fused VSIG3 was first incubated with various concentrations of scFv VS10 (from 0.1–100 µg/mL) and then introduced into the wells to react with the coated VISTA protein. After the plates were incubated at room temperature for 1 h, the plates were washed six times with PBST. Goat anti-human Fc antibodies (Jackson ImmunoResearch Laboratories, Inc.) were added to detect bound VSIG3 molecules. Then, HRP-conjugated donkey anti-goat antibodies (Jackson ImmunoResearch Laboratories, Inc.) were used for the reaction. After washing, the TMB substrate was added to each well for development. The reaction was stopped with 1 N HCl, and signal intensity was measured at 450 nm.
Molecule modeling and docking
To investigate how chicken scFvs interact with VISTA, a structural model of scFv VS10 was developed in accordance with the protocols of the Antibody Modeling Cascade modules in Discovery Studio v. 2021 (BIOVIA, Inc). The light chain and heavy chain of the scFv VS10 model were generated using the crystallographic structure of anticardiac Troponin I (PDB ID: 4P48) and the X-ray structure of anti-p-tau (PDB ID: 4GLR) as scFv structural templates, respectively. We developed our complex structural model of scFv–VISTA interaction in the antibody–antigen docking program ZDOCK (Akita and Nakai Citation1993) using default parameters, and we evaluated shape complementarity and desolvation energy to obtain an scFv–VISTA complex. We selected the PDB ID of 6OIL as the antigen of the three-dimensional X-ray crystallographic structure of VISTA. To obtain a reliable complex structure, we used an RDOCK program to refine the docked protein poses of cluster 1 from ZDOCK by performing energy minimization in CHARMm. Finally, the rational antibody–antigen complex model satisfied the CDRs of the scFv VS10 antibody interacting with the VISTA binding site at the antigen–antibody interface.
Results
Chicken scFv antibody library construction and biopanning
Regarding the experimental design, chickens were selected as the animal model for immunization with the expectation of inducing a neutralizing humoral antibody response against immunogen through species difference. Once recombinant VISTA proteins had been used to immunize chickens and induce high-valence antibody responses, the chickens were sacrificed to construct a highly complicated scFv antibody library (3.2 × 108) expressed through bacteriophages. Recombinant VISTA proteins were subjected to panning using the constructed phage-displaying scFv library. As depicted in Figure A using the library phage for panning, the eluted (bound) phage titer significantly increased as the number of rounds of panning increased compared to in the wild-type M13 phage experiment. After the fourth round of panning, the eluted phage titer had increased 138-fold compared with that after the first round; this meant that specific anti-VISTA clones were enriched in the library. In addition, regarding the reamplified phage library after panning, the VISTA proteins were subjected to phage ELISA; compared with the initial phage library, which exhibited no binding reactivity, the results indicated that the library phage following the third round of panning began to exhibit binding reactivity toward the VISTA proteins, and following the fourth round, the amplified library phage exhibited significant binding signals (Figure B).
Figure 1. Biopanning and single clone analysis. (A) Regarding the anti-VISTA phage library, the number of eluted phages was calculated after each panning round; this number represents the quantity of specific phages bound to VISTA after each panning round. M13 represents the wild-type phage used as the negative control. (B) The binding reactivity of the re-amplified phage library regarding VISTA after each panning round was tested using phage ELISA. (C) After panning, the seven isolated representative scFvs underwent serial dilutions, and their binding reactivity toward VISTA was tested. (D) Competitive binding assay of scFv against the VISTA protein. The amount of bound scFv in the presence of free VISTA inhibitor was measured and expressed as a percentage of the binding of scFv in the absence of an inhibitor. B and B0 denoted the amount of bound scFv in the presence and absence of the inhibitor, respectively.
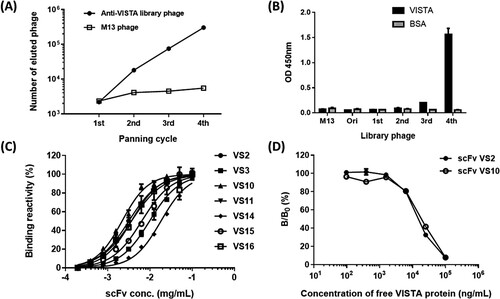
Single scFv clone analysis
After the panning process, 30 clones were randomly selected for a single scFv analysis. The sequencing results revealed repeated similar sequences caused by specific clones being enriched during panning. The 30 clones represented seven sequences. Once the seven representative scFv clones had been expressed, the VISTA protein was subjected to the binding reactivity of scFv with serially diluted concentrations (Figure C). The results showed that the EC50 of scFv clone VS10 was approximately 73 nM, and this scFv clone had the best binding reactivity of all the clones; the other three scFv clones, namely VS2, VS11, and VS16, formed the group with the second-best binding reactivity; their EC50 was approximately 100–120 nM. Finally, the two scFv clones with the optimal binding reactivity and the highest repeatability in their sequences, namely scFv VS2 (8/30) and VS10 (10/30), were selected for subsequent analysis. In the Figure D, we used a competitive ELISA to demonstrate the specificity of scFv VS2 and scFv VS10 in binding VISTA protein. The results indicate that VISTA protein in a free form can compete the reaction of the scFv targeting to the VISTA coated on the wells and producing a dose-dependent response. The results thus confirm the specificity of scFv that binds VISTA protein.
Blockade effect of scFvs on VISTA-induced T-cell exhaustion in PBMCS
A further experiment was conducted to understand whether two isolated anti-VISTA scFvs, namely VS2 and VS10, were capable of blocking a T-cell immunosuppressive response induced by VISTA. We determined that providing VISTA at various concentrations can effectively inhibit the anti-CD3 Ab-induced T-cell activation reaction and produce a dose-dependent effect (Supplemental Figure 1A). We also demonstrated that cocoating VISTA and anti-CD3 Ab did not interfere with the anti-CD3 Ab-induced T-cell activation reaction. The negative control proteins (NCPs) used for cocoating under the same conditions did not produce the same inhibition reaction as that produced by VISTA. Compared to the group that only anti-CD3 Ab was used, the NCPs with cocoating did not interfere with the stimulation by anti-CD3 Ab (supplemental Figure 1B). As depicted in Figure A, the PBMCs of healthy people significantly stimulated T-cell activation and aggregation responses through the action of anti-CD3 antibodies, and the production of such a response was inhibited by the addition of recombinant VISTA proteins. The experiment showed that T-cell aggregation activation was preserved in the test group after scFv VS2 or scFv VS10 treatment, meaning that scFv VS2 or scFv VS10 bound to VISTA could effectively neutralize the immunosuppressive response induced by VISTA, thus preventing the reduction of T-cell activation. In another cell experiment (Figure B and Supplemental Figure 2), T-cell activation was effectively induced after adding anti-CD3 antibodies into CSFE-stained PBMCs, causing cell division and proliferation; such a response could be inhibited through the addition of VISTA. After scFv VS2 or scFv VS10 was added to target VISTA, the blockade effect was induced, which prevented the inhibition of T-cell activation. Calculation of the effects of scFv VS2 and scFv VS10 revealed that 21% and 23%, respectively, of the activity could be preserved after the initial T cells were activated (Figure C).
Figure 2. Anti-VISTA scFvs can block T-cell exhaustion caused by VISTA. (A) Recombinant VISTA proteins and specified scFvs of 100 µg/mL were used to treat PBMCs from healthy people and developed for 5 days, followed by microscopic observation. Anti-CD3 antibody was used to induce T-cell activation; after activation, the T cells resulted in proliferation and aggregation responses. (B) Recombinant VISTA proteins and specified scFvs of 100 µg/mL were used to treat CSFE-stained PBMCs and developed for 5 days, and they were then subjected to a flow cytometry assay. Activated T cells resulted in division and proliferation responses, causing the movement and shift of fluorescent signals. (C) Normalized quantitative values to anti-CD3 antibody only group (%) are used to represent outcomes of the activation responses of CSFE-stained PBMCs after scFv treatment in (B).
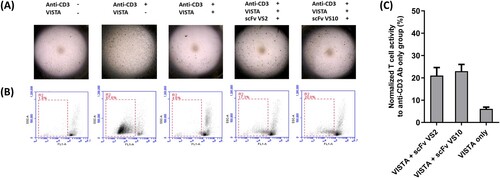
Ability of scFvs to bind to endogenous VISTA on THP1 cells
The expression of endogenous VISTA on various human leukemia cell lines was tested; the six human leukemia cell lines K562, THP1, HL60, KG1, CCRFCEM, and Kasumi-1 were subjected to a flow cytometry analysis using commercial anti-VISTA antibodies. The results revealed that VISTA was barely expressed in HL60 cells and highly expressed in THP1 cells (Figure A). In a further experiment, freshly prepared THP1 cells were used to test the binding abilities of scFv VS2 and scFv VS10 regarding endogenous VISTA. The experimental results are presented in Figure B; scFv VS2 and scFv VS10 exhibited a binding response toward THP1 cells, meaning that these scFvs could indeed effectively bind to endogenous VISTA on cancer cell membranes.
Figure 3. Binding reactivity of scFvs toward endogenous VISTA on human leukemia cells. (A) Test of the expression of VISTA on various human leukemia cell lines using commercial anti-VISTA antibodies. The six human leukemia cell lines were K562, THP1, HL60, KG1, CCRFCEM, and Kasumi-1. (B) The binding reactivity of scFvs VS2 and VS10 of different concentrations (1 µg/mL and 10 µg/mL) toward endogenous VISTA on THP1 leukemia cell surface. CTR represents the nonstaining control; PC indicates that commercial anti-VISTA antibodies were used as a positive control in the test; NC signifies an irrelevant scFv without binding signals.
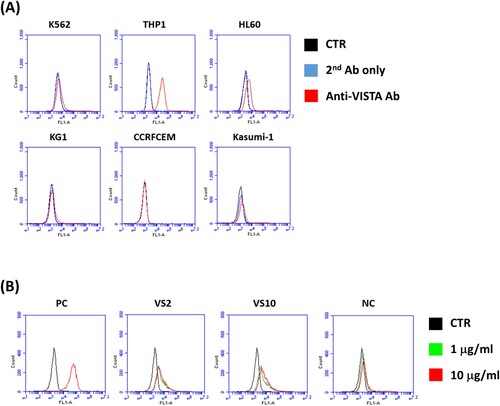
Completion of VISTA binding to ligand VSIG3 by scFvs
To date, the inhibition mechanism of VISTA toward the activity of T cells has not been determined. However, studies have indicated that VSIG3 is a possible binding ligand for VISTA and that VSIG3 itself inhibited T-cell activity. Thus, we tested whether the interaction between VISTA and VSIG3 could be blocked by binding anti-VISTA scFvs to VISTA. As illustrated in Figure , in the experiment involving competitive ELISA, both scFv VS2 and scFv VS10 could significantly block interaction between VISTA and VSIG3 and exhibited dose-dependent responses; regarding the extent of interaction affected, when the scFv density was 50 µg/mL, scFv VS2 and scFv VS10 inhibited 48% and 56% of the interaction, respectively.
Figure 4. ScFvs inhibited VISTA–VSIG3 interaction. The competitive effects of scFvs VS2 and VS10 were confirmed separately through interference in the protein−protein interaction. The amount of VISTA bound to the VSIG3 in the presence of a free scFv VS10 competitor at different concentrations. The result is presented as a percentage of the binding of VSIG3 in the absence of a competitor.
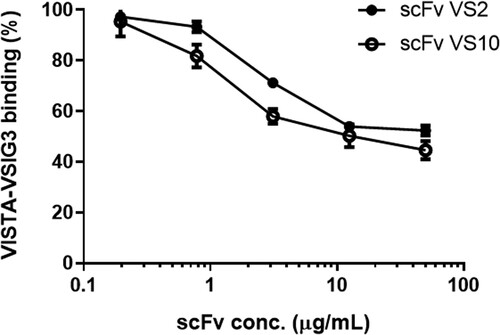
Gene sequence analysis of scFv VS10
The amino acid sequence of scFv VS10 and the germline gene sequence of chicken Ig were compared to understand the genetic variability of scFv VS10. As illustrated in Figure , compared with the germline gene sequence, the total mutation rates in the VL and VH domains in the scFv VS10 gene were 18.3% and 29.1%, respectively; mutations located in CDRs were much than in the framework regions (FRs). Regarding VL, no change was observed in the length of the germline gene. However, regarding VH, a significant insertion mutation was found at the location of heavy-chain CDR3 (CDR-H3), which allowed CDR-H3 to be lengthened to 19 aa, thus signifying the crucial role that CDR-H3 would play in the interaction when scFv VS10 binding to VISTA.
Figure 5. Sequence analysis of scFv VS10 genes. VH and VL nucleotide sequences were determined and translated into amino acid sequences to be aligned with the chicken germline gene. The background of the residues is colored according to sequence similarity. Dark colors indicate conserved residues in all sequences. The color scheme is from dark to light, corresponding to identity, highly conserved residues, and low-conserved residues, respectively. The residue backgrounds colored in white are not similar. Sequence gaps were introduced to maximize the alignment; these are indicated by dots. FR and CDR boundaries are indicated above the germline sequences.
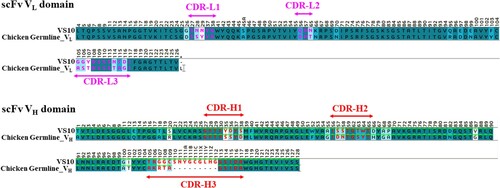
Homology modeling of scFv vs10 and molecular docking of scFv VS10 to VISTA
The structure of CDR loops of the chicken antibody scFv VS10 was constructed using homology modeling. On the basis of three published X-ray crystallographic chicken antibody structures, 4P48 (PDB code) is a chicken anticardiac Troponin I scFv that is most similar to the scFv VS10 framework. As depicted in Figure , the lengths of the CDR-L1 and CDR-L2 loops of scFv 4P48 are greater than the lengths of such loops of scFv VS10. The extra amino acids in the CDR-L1 loop are one glycine, one arginine, and two tyrosines, whereas the CDR-L3 loop contains one aspartic amino acid. The lengths of the other CDR loops are the same and the CDR-H1 sequence is identical to scFv VS10. Comparing the constructed structure of scFv VS10 with scFv 4P48 revealed a shorter CDR-L1, the variability of which was similar to that of CDR-H3, exhibiting high tolerance to display modeling antibody.
Figure 6. Molecular modeling of scFv VS10–VISTA interaction and secondary structure for the scFv VS10 antibody–VISTA antigen model. The VISTA antigen and scFv VS10 are colored in light cyan and gray, respectively. The schematic presents all interactions between scFv VS10 and the VISTA epitope. The interface residues of scFv VS10 and VISTA were concentrated on the CDR-L1 (red), CDR-L2 (orange), CDR-L3 (yellow), CDR-H1(green), CDR-H2 (blue), and CDR-H3 (magenta) loops. The interactions between scFv VS10 and VISTA are illustrated using green, yellow, orange, and brown dotted lines to indicate hydrogen bonds, hydrophobic interactions, ionic interactions, and pi–pi interactions, respectively.
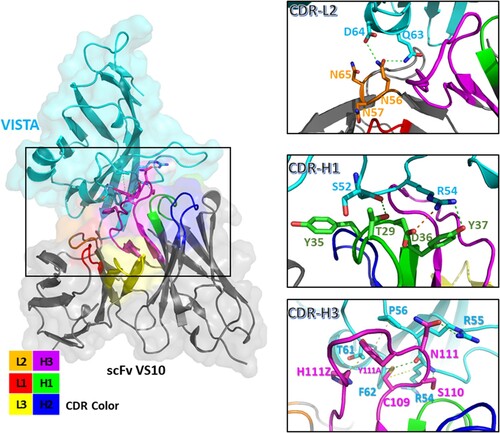
Moreover, antibody–antigen docking revealed that the CDRs of scFv VS10, namely CDR-L2, CDR-H1, and CDR-H3, were involved in the interaction of the epitope on VISTA with scFv VS10 (Figure ). The Asn56 side chain on the CDR-L2 was involved in the Gln63 and Asp64 side chains of the VISTA epitope through two hydrogen bonds. The side chains of Tyr37 and Asp36 of CDR-H1 interacted with Arg54 through a hydrogen bond and an ionic interaction, respectively. The side chain of Thr29 interacted with the main chain of Ser52 through a hydrogen bond. In addition, the side chain of Cys109 on the CDR-H3 loop interacted with the side and main chains of Arg54 and the side chain of Phe62 through a hydrophobic interaction, a hydrogen bond, and a pi-sulfur interaction, respectively. Furthermore, the side chains of Ser110 and Asn111 on the CDR-H3 loop interacted with the main chain of Arg54 and the side chain of Arg55 through two hydrogen bonds, respectively. Moreover, the Tyr111A and His111Z side chains on the CDR-H3 loop interacted with the side chains of Pro56 and Thr61 through pi–pi interaction and hydrophobic interactions, respectively.
Discussion
Studies have revealed high expression of VISTA in myeloid cells. Thus, antibody recognition response was observed in myeloid-derived THP1 leukemia cancer cells. Studies have suggested inferior expression of VISTA in tumor cells; compared with cancer cell expression in the tumor microenvironment (TME), high expression of VISTA was found on high density-infiltrating immune cells. However, given the immunosuppressive regulation exhibited by VISTA, VISTA indeed has potential for use as a therapeutic target in immunotherapy (Blando et al. Citation2019; Xie et al. Citation2018). Moreover, studies have confirmed a correlation between VISTA expression and the survival rates of cancer patients (Kuklinski et al. Citation2018). Research has also demonstrated that the activation and differentiation of tumor-infiltrating T cells could be promoted effectively in mice directly subjected to therapy with VISTA antibodies (Le Mercier et al. Citation2014; Lines et al. Citation2014). Thus, the effectiveness of using targeted immunotherapy aimed at VISTA has been confirmed, and it was not affected by the expression levels of VISTA on tumors. The results of studies on mice tumors have indicated an opportunity for treatment and facilitated the launching of phase I clinical trials on anti-VISTA monotherapy (NCT02671955). Regarding immunotherapy against immune checkpoint targets, the combined use of multiple biologics is attractive for improving the effect of immunotherapy. Thus, in addition to therapy using single-target inhibitors, several treatment strategies that bind several immune checkpoint inhibitors or combine checkpoint inhibitors with chemotherapy or cancer vaccines were applied in clinical experiments (Kon and Benhar Citation2019). In the immunomodulatory pathways that PD-1 is involved in, VISTA likely represents an auxiliary target that can be used to enhance the effect of immunotherapy; this further indicates its clinical potential for cancer treatment. Thus, VISTA inhibitors have potential use in combination therapy, and synergistic therapeutic effects can be produced in combination with biologics directly aimed at targets on tumors.
Given species differences, more effective somatic mutations can often be induced using immunogens from mammalian proteins with chickens as animal models for immunization. Notably, different from the chicken germline, the somatic mutations in scFv clones were primarily the insertion mutations of amino acid produced on CDR-H3 (Figure ). The function of antibody CDRs is to generate interactions with an antigen epitope, which further affects the antibody affinity and the effect of neutralization; the CDR-H3 length of scFv VS10 is long, enabling CDR loops to enter more profound domains in an antigen epitope. Compared with the variability on VH (FRs + CDRs; 35/126, 28%), that on VL was inferior (19/103, 18%) and its sequence length was the same as that of the germline, meaning that the VL domain probably functions as a structure stabilizer for scFv composition. In addition, analysis using molecular docking revealed that the binding of scFv VS10 to VISTA was mostly undertaken by the VH domain, and such a configuration of antibody–antigen binding was probably caused by the outcomes of random assembly in the VH and VL domains when scFv antibody genes are constructed using the phage display system. Similar configurations of antibody binding exist in numerous clinical antibody drugs (Muller et al. Citation1998), whereas the stabilizing VL domain has potent use in antibody optimization.
An insertion mutation of amino acid on CDR-H3 was found in antibodies induced using chickens as the model for immunization, making CDR-H3 19 aa long; through participating in antigen binding, the longer CDR-H3 loop became more similar to the antigens’ structure and generated a greater binding force. Analysis and observation through molecular docking revealed that the CDR-H3 of scFv VS10 formed an oval-like configuration to bind to VISTA. Notably, a similar CDR-H3 configuration was found in another launched chicken-derived antibody (PDB id: 4GLR), which also underwent somatic mutation, and interaction was achieved through a longer CDR-H3 loop that recognized the epitope configuration (Shih et al. Citation2012). Such findings illustrate that the neutralization reaction of antigens can be effectively induced when the species difference is favorable for immunization. This is followed by humanization through antibody engineering before further application in clinical drug development. To date, positive outcomes have been obtained regarding clinical cancer treatment using immunotherapy. Inhibitors aimed at the immune-checkpoint VISTA proteins are likely to be combined with existing treatment strategies to enhance the effect of therapy. Thus, anti-VISTA antibodies can serve as an auxiliary strategy in the immune escape process of tumor development to overcome immune cell exhaustion, thus providing more opportunities for the future development of anticancer drugs.
Data availability statement
The data that support the findings of this study are available in figshare at http://doi.org/10.6084/m9.figshare.16817530.
Disclosure statement
No potential conflict of interest was reported by the author(s).
Correction Statement
This article has been corrected with minor changes. These changes do not impact the academic content of the article.
Additional information
Funding
References
- Akita EM, Nakai S. 1993. Production and purification of Fab’ fragments from chicken egg yolk immunoglobulin Y (IgY). J Immunol Methods. 162:155–164. doi:10.1016/0022-1759(93)90380-p.
- Andris-Widhopf J, Rader C, Steinberger P, Fuller R, Barbas Iii CF. 2000. Methods for the generation of chicken monoclonal antibody fragments by phage display. J Immunol Methods. 242:159–181. doi:10.1016/s0022-1759(00)00221-0.
- Barbas CF, Kang AS, Lerner RA, Benkovic SJ. 1991. Assembly of combinatorial antibody libraries on phage surfaces: the gene III site. Proc Natl Acad Sci U S A. 88:7978–7982. doi:10.1073/pnas.88.18.7978.
- Blando J, Sharma A, Higa MG, Zhao H, Vence L, Yadav SS, Kim J, Sepulveda AM, Sharp M, Maitra A, Wargo J. 2019. Comparison of immune infiltrates in melanoma and pancreatic cancer highlights VISTA as a potential target in pancreatic cancer. Proc Natl Acad Sci U S A. 116:1692–1697. doi:10.1073/pnas.1811067116.
- Cai YC, Guo J, Chen SH, Tian LG, Steinmann P, Chen MX, Li H, Ai L, Chen JX. 2012. Chicken egg yolk antibodies (IgY) for detecting circulating antigens of Schistosoma japonicum. Parasitol Int. 61:385–390. doi:10.1016/j.parint.2012.01.008.
- Flajnik MF, Tlapakova T, Criscitiello MF, Krylov V, Ohta Y. 2012. Evolution of the B7 family: co-evolution of B7H6 and NKp30, identification of a new B7 family member, B7H7, and of B7's historical relationship with the MHC. Immunogenetics. 64:571–590. doi:10.1007/s00251-012-0616-2.
- Flies DB, Higuchi T, Chen L. 2015. Mechanistic Assessment of PD-1H Coinhibitory Receptor-Induced T Cell Tolerance to Allogeneic Antigens. J Immunol. 194:5294–5304. doi:10.4049/jimmunol.1402648.
- Gassmann M, Thömmes P, Weiser T, Hübscher U. 1990. Efficient production of chicken egg yolk antibodies against a conserved mammalian protein. FASEB J. 4:2528–2532. doi:10.1096/fasebj.4.8.1970792.
- Groves DJ, Morris BA. 2000. Veterinary sources of nonrodent monoclonal antibodies: interspecific and intraspecific hybridomas. Hybridoma. 19:201–214. doi:10.1089/02724570050109602.
- Kon E, Benhar I. 2019. Immune checkpoint inhibitor combinations: Current efforts and important aspects for success. Drug Resist Updat. 45:13–29. doi:10.1016/j.drup.2019.07.004.
- Kuklinski LF, Yan S, Li Z, Fisher JL, Cheng C, Noelle RJ, Angeles CV, Turk MJ, Ernstoff MS. 2018. VISTA expression on tumor-infiltrating inflammatory cells in primary cutaneous melanoma correlates with poor disease-specific survival. Cancer Immunol Immunother. 67:1113–1121. doi:10.1007/s00262-018-2169-1.
- Le Mercier I, Chen W, Lines JL, Day M, Li J, Sergent P, Noelle RJ, Wang L. 2014. VISTA Regulates the Development of Protective Antitumor Immunity. Cancer Res. 74:1933–1944. doi:10.1158/0008-5472.CAN-13-1506.
- Lines JL, Pantazi E, Mak J, Sempere LF, Wang L, O'Connell S, Ceeraz S, Suriawinata AA, Yan S, Ernstoff MS, Noelle R. 2014a. VISTA is an immune checkpoint molecule for human T cells. Cancer Res. 74:1924–1932. doi:10.1158/0008-5472.CAN-13-1504.
- Lines JL, Sempere LF, Broughton T, Wang L, Noelle R. 2014b. VISTA is a novel broad-spectrum negative checkpoint regulator for cancer immunotherapy. Cancer Immunol Res. 2:510–517. doi:10.1158/2326-6066.CIR-14-0072.
- Mulati K, Hamanishi J, Matsumura N, Chamoto K, Mise N, Abiko K, Baba T, Yamaguchi K, Horikawa N, Murakami R, Taki M. 2019. VISTA expressed in tumour cells regulates T cell function. Br J Cancer. 120:115–127. doi:10.1038/s41416-018-0313-5.
- Muller YA, Chen Y, Christinger HW, Li B, Cunningham BC, Lowman HB, de Vos AM. 1998. VEGF and the Fab fragment of a humanized neutralizing antibody: crystal structure of the complex at 2.4 A resolution and mutational analysis of the interface. Structure. 6:1153–1167. doi:10.1016/s0969-2126(98)00116-6.
- Rahbarnia L, Farajnia S, Babaei H, Majidi J, Veisi K, Ahmadzadeh V, Akbari B. 2017. Evolution of phage display technology: from discovery to application. J Drug Target. 25:216–224. doi:10.1080/1061186X.2016.1258570.
- Rahman S, Van Nguyen S, Icatlo Jr FC, Umeda K, Kodama Y. 2013. Oral passive IgY-based immunotherapeutics: a novel solution for prevention and treatment of alimentary tract diseases. Hum Vaccin Immunother. 9:1039–1048. doi:10.4161/hv.23383.
- Shih HH, Tu C, Cao W, Klein A, Ramsey R, Fennell BJ, Lambert M, Shúilleabháin DN, Autin B, Kouranova E, Laxmanan S. 2012. An ultra-specific avian antibody to phosphorylated tau protein reveals a unique mechanism for phosphoepitope recognition. J Biol Chem. 287:44425–44434. doi:10.1074/jbc.M112.415935.
- Shim H. 2016. Therapeutic Antibodies by Phage Display. Curr Pharm Des. 22:6538–6559. doi:10.2174/1381612822666160923113714.
- Tsai KC, Chiang CW, Lo YN, Chang FL, Lin TY, Chang CY, Chen WC, Lee YC. 2018. Generation and characterization of avian-derived anti-human CD19 single chain fragment antibodies. Anim Biotechnol. 1–9. doi:10.1080/10495398.2018.1486323.
- Tsurushita N, Park M, Pakabunto K, Ong K, Avdalovic A, Fu H, Jia A, Vásquez M, Kumar S. 2004. Humanization of a chicken anti-IL-12 monoclonal antibody. J Immunol Methods. 295:9–19. doi:10.1016/j.jim.2004.08.018.
- Villarroel-Espindola F, Yu X, Datar I, Mani N, Sanmamed M, Velcheti V, Syrigos K, Toki M, Zhao H, Chen L, Herbst RS. 2018. Spatially Resolved and Quantitative Analysis of VISTA/PD-1H as a Novel Immunotherapy Target in Human Non-Small Cell Lung Cancer. Clin Cancer Res. 24:1562–1573. doi:10.1158/1078-0432.CCR-17-2542.
- Wang J, Wu G, Manick B, Hernandez V, Renelt M, Erickson C, Guan J, Singh R, Rollins S, Solorz A, Bi M. 2019. VSIG-3 as a ligand of VISTA inhibits human T-cell function. Immunology. 156:74–85. doi:10.1111/imm.13001.
- Wang L, Rubinstein R, Lines JL, Wasiuk A, Ahonen C, Guo Y, Lu LF, Gondek D, Wang Y, Fava RA, Fiser A. 2011. VISTA, a novel mouse Ig superfamily ligand that negatively regulates T cell responses. J Exp Med. 208:577–592. doi:10.1084/jem.20100619.
- Xie X, Xuan C, Li T, Wang L, Teng L, Liu J. 2018. High-Density Infiltration of V-domain Immunoglobulin Suppressor of T-cell Activation Up-regulated Immune Cells in Human Pancreatic Cancer. Pancreas. 47:725–731. doi:10.1097/MPA.0000000000001059.