Abstract
Brachystegia boehmii leaf extracts are ethnopharmacological known to treat microbial infections in burn wounds. Coupling the action of the traditional plant medicine and nanotechnology gives rise to innovative strategies for healing burn wounds. This study aimed to determine the effect of B. boehmii extracts and their green synthesised nanoparticles on bacteria which infect burn wounds. The effects of water, chloroform and methanolic leaf extracts were compared with the effects of the phytosynthesised silver nanoparticles on burn wound infectious bacteria. Dried leaves were extracted using the maceration technique, followed by filtration and concentration of the filtrate using a rotavapor. Drying was achieved by using a centrivap and appropriate masses were dissolved in dimethyl sulfoxide to achieve 100 mg/ml concentrations. Silver nanoparticles were synthesised using the methanol extract of the plant leaves and characterised using a UV–VIS spectrophotometer. The disc diffusion method was used to assess the bacterial susceptibility levels with Ciprofloxacin as the positive control and DMSO and 1 mM silver nitrate as the negative controls. The green synthesised silver nanoparticles produced a yellowish colour with a peak at 420 nm wavelength. They exhibited antibacterial activity against all five bacteria; Pseudomonas aeruginosa, Klebsiella pneumoniae, Escherichia coli, Staphylococcus aureus and Staphylococcus epidermidis comparable to those of the B. boehmii leaf solvent extracts. A zone of inhibition of 20 ± 1.00 mm was obtained on the action of B. boehmii leaf extract against P. aeruginosa. Plant synthesised nanoparticles exhibited broad spectrum antibacterial activity and hence are potential future burn wound antibacterial therapeutics.
1. Introduction
Bacterial infections that take place after burns are a major concern as they can lead to serious complications such as pneumonia, sepsis and even death (Wang et al. Citation2018). Second and third degree burns are a major cause for concern as the wounds are prone to infection. Such burn wound infections may lead to complications which result in 45–65% of burn wound-related deaths (Lachiewicz et al. Citation2017). In addition, considering the illustration by Jackson's theory, the zones of coagulation which undergo coagulative necrosis and the zone of stasis provide a conducive environment for bacterial growth (Rose and Chan Citation2016). The bacterial growth in this region in turn hinders prompt wound healing. Burn treatment, therefore, requires a drug that accelerates the rate of wound healing and confers some antimicrobial activity (Rousselle, Braye, and Dayan Citation2018).
Multidrug resistant (MDR) bacteria prove to be a menace in burn wounds of hospitalised patients, especially for patients with full thickness burns. These MDR include Gram negative P. aeruginosa, K. pneumoniae, E. coli and Gram positive S. aureus (Wang et al. Citation2018). Burn wound infections are becoming difficult to treat due to the increase in strain’s antimicrobial resistance (Mussin et al. Citation2021). Pathogens are evolving and becoming more resistant. Therefore, there is a need for effective antimicrobials in order to lower the morbidity and mortality of burn patients.
Currently, the standard for burn wound treatment in Zimbabwe is silver sulfadiazine (SSD) cream (Ministry of Health and Child Welfare Citation2015; Dai et al. Citation2018) which has, however, been reported to have poor effectiveness (Yaman et al. Citation2010; Karunanidhi et al. Citation2017). SSD is a dihydropteroate synthase inhibitor that acts as a bacteriostatic agent in pathogens (Achari et al. Citation1997). Its mechanism of action is based on the competitive inhibition of the enzymes’ substrate, known as para-aminobenzoic acid (pABA), by the sulphonamide. This action inhibits folate production, resulting in microbial death. In spite of this action, there are reports of SSD cream being cytotoxic to the skin cells, causing neutropenia and erythema (Karunanidhi et al. Citation2017). Plants harbour many curative properties derived from their phytochemical contents, hence some can be used as alternative antimicrobial agents.
The long-established use of traditional plant medicines is based on the fact that it is an easily accessible and affordable source of treatment in the primary healthcare system (Maroyi Citation2013; Budovsky et al. Citation2015; Mussin and Giusiano Citation2020). Of particular interest are two plants, Ximenia caffra and Brachystegia boehmii which are used for wound healing by the people in Mashonaland West Province of Zimbabwe with reports of rapid burn wound healing effects on second and third degree burns. Focused group discussion data indicate that a concoction of the two plant leaves removes what the local people of Mashonaland West Province call ‘Green’ which is in actual fact a greenish-blue slime that occurs on long-term burn wounds generated from the compounds pyocyanin and pyoverdin of P. aeruginosa bacteria (Palamthodi et al. Citation2011).
X. caffra has been comprehensively explored and reported to have antimicrobial activity on a variety of microbes including Bacillus subtilis, E. coli, K. pneumoniae S. epidermidis, P. aeruginosa and S. aureus (Maroyi Citation2016). Other findings on a Ximenia species, X. americana, suggest that the species extracts harbour antimicrobial, antigonococal and antifungal activity (Maikai et al. Citation2009; Queiroz Monte et al. Citation2012). There is, however, a dearth of information on the pharmacological effects of B. boehmii as it has not been exhaustively researched. The plant is only reported to have anti-inflammatory activity and ethanolic extract antimicrobial effects against S. aureus, P. aeruginosa, E. coli, Bacillus cereus and B. subtilis (Chitemerere and Mukanganyama Citation2011; Chirisa and Mukanganyama Citation2016; Isabel et al. Citation2018). To counteract the effect of the persistent burn wound infections, the use of B. boehmii leaf extracts and their modifications may be the solution to cheap and reliable therapeutics.
The importance of nanotechnology in the field of biomedical studies has opened avenues to disease treatment (Mo et al. Citation2015; Ahn et al. Citation2019). This is due to nanotechnologys’ enhanced antimicrobial properties and rapid absorption properties that are endorsed by their unique structures, size and morphology. Biofabrication of nanoparticles (NPs) is a recent technique which include the use of microorganisms, enzymes and plant extracts (Azizi et al. Citation2014; Permana et al. Citation2021). These organisms act as bioreductants, hence they are regarded as eco-friendly alternatives to chemical and physical methods of NPs synthesis. In particular, phyto-fabrication of NPs brings about a link between the herbal medicines and the emerging era of nanotechnology. This is advantageous over other biological processes as it eliminates the elaborate process of maintaining cell cultures or using large quantities of biomass for treatment (Singh et al. Citation2016). A number of plant extracts have since been used for the production of nanoparticles (Ahmed et al. Citation2016; Ahmed and Mustafa Citation2020).
Currently, a considerable amount of biomedical and therapeutics research and development make use of nanotechnology in wound healing (Ahmed et al. Citation2016; Patra et al. Citation2018). Such use of nanoparticles in wound healing is thought to enhance healing through promoting the antimicrobial and anti-inflammatory activities of herbal extracts (Pannerselvam et al. Citation2017; Rajendran et al. Citation2018; Permana et al. Citation2021). Nanotechnology promotes sustainable use of medicines, improves health outcomes and helps minimise side effects (Negut et al. Citation2018; Patra et al. Citation2018). As such, in vivo wound antimicrobial activities of B. boehmii crude extracts and their synthesised silver nanoparticles were evaluated. In this case, it is expected that instead of using higher concentrations of leaf extract, modified B. boehmii silver nanoparticles will work effectively at a less concentration.
The study at hand seeks to investigate and compare the antimicrobial activity of different extracts of B. boehmii leaf extracts (water, chloroform and methanol) and those of the plants’ synthesised silver nanoparticles against burn wound infectious bacteria.
2. Methods
2.1. Plant extraction
Plant materials were collected from the Chinhoyi University of Technology campus (17°21'05.5"S 30°12'23.6"E). Relevant permissions to access the local lands for plant harvesting were granted by the District Administrator for Mashonaland West Province. Further approval was granted by the Provincial Governor and resident Minister of Mashonaland West Province.
Positive identification of the collected plant material was done at the Zimbabwe National Herbarium by Mr Christopher Chapano. The voucher specimens were deposited at the Chinhoyi University Biology herbarium collection (voucher number 2017001).
Care was taken to remove debris, wash and dry the leaves at 40°C for 36 h in an oven. The leaves were ground to a powder using a pestle and mortar and the particles were sieved through a 600 µm pore sized mesh sieve (USA standard testing sieve A-S-TM E11).
Extraction was done using three solvents; Chloroform, Methanol and Water via the maceration technique. The plant powder was soaked in solvent for 3 days at a ratio of 1:10 plant powder to solvent (Thakur et al. Citation2011). After 3 days, the extract was filtered and pressed to remove all solid material using a vacuum filter, Buchner funnel, cotton wool and a Whatman filter paper (Grade 1). Filtrate concentration was achieved by using rotavapor (Buchi R-200 Rotavapor System) till semisolid. This was followed by drying of the extract using a centriVap vacuum concentrator (Labconco 7812010 CentriVap® Mobile System) at a temperature of 40°C (Begashaw et al. Citation2017).
2.2. Green synthesis of silver nanoparticles using Brachystegia boehmii
A volume of 2 ml of B. boehmii methanol extract was added dropwise to 100 ml of boiling 1 mM AgNO3 whilst applying constant stirring. The reaction was terminated by placing reactants in ice immediately after observing a yellow colour. The solution was centrifuged at 13,000 rpm for 15 min using the eppendorf centrifuge (Eppendorf 5804R, Brinkmann Instruments, Westbury, NY), and the pellet was washed twice to remove debris.
The silver nanoparticles used as the control were synthesised from stabilising and reducing silver nitrate with sodium citrate. This was done following the protocol by Salomoni (Salomoni Citation2017).
2.3. Nanoparticle characterisation
Nanoparticle characterisation was done using a Cecil (CE 1021) UV/VisSpectrophotometer (Cecil Instruments, England). Optical Glass cuvettes were used for the characterisation process. The positive control for nanoparticle synthesis was chemically synthesised by reducing AgNO3 with sodium citrate.
2.4. Preparation of bacterial strains
Glycerol stocks of the following organisms; K. pneumoniae, P. aeruginosa ATCC 27853, S. aureus ATCC 25923, S. epidermidis and E. coli were obtained from Chinhoyi Provincial Hospital laboratory. The cultures were revived by transferring a loopful of cells from the cell vial to Mueller Hinton broth (MHB) (Sigma Aldrich) incubating the broth for 24 h at 37°C. Dilution of the MHB was done to achieve the McFarland 0.5 standard (1.5× 108 cfu/ml) using normal saline water solution before use in the drug sensitivity tests. All activities were done under aseptic conditions with sterilised apparatus.
2.4.1. Drug sensitivity tests
2.4.1.1. Disc diffusion
100 µl of 100 mg/ml of each plant extract was inoculated onto autoclaved 6 mm Whatman discs and left under UV light for 10 min to allow disc diffusion in a sterile environment. Seven different treatments (extracts) were used on the five organisms. Each mode of treatment was tested thrice. Plant derived silver nanoparticles were used at a concentration of approximately 0.8 mg/ml with 1 mM AgNO3 as the negative control.
Disc diffusion assay was carried out using the Kirby Bauer technique (Hudzicki Citation2009). About 12 ml molten Mueller Hinton Agar (MHA) was uniformly poured into clearly labelled sterile plates. The plates were left to set and solidify for 15 min. A sterile swab was immersed into the prepared inoculum and swabbed onto the plates using the lawn technique.
The loaded discs were placed on the surface of the inoculated agar plates and incubated for 12 h at 37°C. Ciprofloxacin (5 µg) was used as the positive control and DMSO used as the negative control. Zones of inhibition were noted after the incubation period, with zones greater than 9 mm recorded as antimicrobial activity.
2.5. Data analysis
Data were expressed as mean values ± standard deviation (S.D). Repeated measures ANOVA was used to test for the level of significance for both the treatment trials and the organisms. This was done in order to compare the mean differences between and within groups. Values p < 0.05 were taken to imply statistical significance.
3. Results and discussion
3.1. Characterisation of NPs
In this study, phyto-fabrication of silver nanoparticles was successfully demonstrated using the B. boehmii extract as the reducing agent, producing a yellowish-brown appearance (). The visual appearance of the nanoparticles produced is a characteristic of silver nanoparticles, and it is in line with other published works (Ethiraj et al. Citation2016; Behravan et al. Citation2019; Ganesan et al. Citation2019).
Figure 1. UV–VIS spectra of silver nanoparticles: green synthesised B. boehmii and sodium citrate synthesised (control).
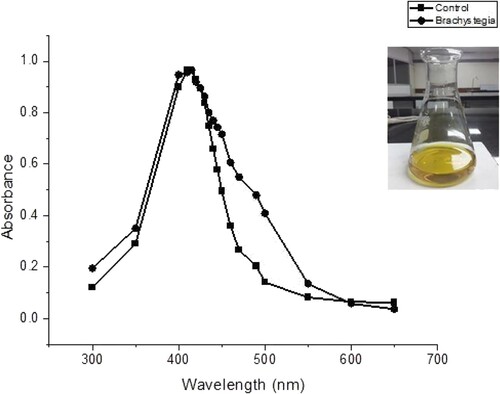
shows the peaks observed between 400 and 450 nm, confirming the formation of nanoparticles in accordance with previous studies (Mucheneri et al. Citation2017; Moodley et al. Citation2018). The UV absorption spectrum of B. boehmii NPs (green) showed a surface plasmonic resonance peak of 420 nm which is in line with the characteristic of silver nanoparticles (Ethiraj et al. Citation2016). The absorption band peaking at 415 nm is the control (red), nanoparticles synthesised from sodium citrate.
Recent years have seen the production of biogenic-metal nanoparticles for the enhanced treatment of diseases and wounds (Patra et al. Citation2018). Green synthesis of nanoparticles depends on the plant species and different phytochemical components such as proteins, amino acids, polysaccharides, polyphenols, terpenoids and organic acids which are known to reduce silver ions forming silver nanoparticles (Azizi et al. Citation2014; Ahmed et al. Citation2016). B. boehmii may, therefore, have the aforementioned phytochemicals in its leaves, hence proving its bio-reduction capabilities. The phytochemicals may have acted by reducing and capping the silver ions forming stable ions that remained in colloidal state for up to three months. Durability of the silver nanoparticles formed in this study is, however, lower than the six-month period of A. australe synthesised nanoparticles (Mussin et al. Citation2021). The difference in durability is possibly subject to the capping and stabilising agents within the phytochemical profile of the plants.
3.2. Drug sensitivity tests
The effect of the seven different modes of treatment on the five different infectious bacteria is presented in . Ciprofloxacin (A) showed effectiveness on the bacterial infections, with all the organisms being susceptible to it. The negative controls, DMSO and AgNO3, were unreactive with the bacteria, suggesting that they were not effective against all the bacteria tested. The zones of inhibition considered to be antimicrobial activity were greater than 9 mm hence anything less than 9 mm was non-activity. While both DMSO and AgNO3 are known to have some form of antimicrobial activity at high percentages, the concentrations used in this study were far too low to confer antimicrobial activity. The silver ions in AgNO3 are also known to be easily oxidised hence the reason why the use of 5% silver nitrate was ruled off as an antimicrobial agent in the 1960s (Leaper et al. Citation2012).
Table 1. Antibacterial activity of extracts of B. boehmii leaves by agar-diffusion method as expressed by the mean ± standard deviation. Ciprofloxacin (A), B. boehmii water extract (B), Methanol extract (BM), Chloroform extract (BC) and Nanoparticles (BN).
Plant bioactive compounds have been used in the treatment and management of burn wounds (Thakur et al. Citation2011; Shah and Amini-Nik Citation2017; Vaghardoost et al. Citation2018). Such plants produce metabolites with several curative properties that benefit the burn wound healing process in a variety of ways. One such benefit of the plant metabolites is the antimicrobial activity. Plant phytochemicals such as tannins, alkaloids, flavonoids, phenolic compounds and several other aromatic compounds are potential defence mechanisms against many microorganisms (Rex et al. Citation2018, Wasef et al. Citation2019). In this study, the antimicrobial activities of B. boehmii leaf extracts are successfully demonstrated in burn wounds infectious bacteria.
Water extracts of the tree leaves provided low antimicrobial effect, with an average maximum of 10 mm zone of inhibition on the five bacteria. K. pneumoniae and S. aureus proved to be resistant to the water extract. Chloroform and methanol extracts exhibited better action, with 4 bacterial species indicating signs of being susceptible. These observations may be due to the fact that the latter solvent extracts contain concentrated amounts of phytochemicals that promote bacterial elimination. Chloroform extracts contain high quantities of flavonoids and terpenoids while methanol extracts contain a much-concentrated amount of anthocyanins, flavones, lactones, polyphenols, phenones, quassinoids, saponins, tannins, terpenoids, totarol and xanthoxyllines (Rex et al. Citation2018; Selvakumar et al. Citation2018). Depending on the phytochemical composition of B. boehmii, which is yet to be analysed, the extracts may be rich in metabolites promoting antimicrobial activity.
The plant extract showed greater potency to the Gram-positive bacteria than to the Gram-negative bacteria. E. coli, K. pneumoniae and P. aeruginosa exhibited signs of resistance to the plant water extract, with a lower susceptible level presented by the methanol and chloroform extracts. On the other hand, the Gram-positive bacteria S. aureus and S. epidermis showed a higher susceptibility to the plant extract, especially the chloroform extract. This is in line with findings that showed that Gram-negative bacteria generally have higher intrinsic antibacterial resistance (Fankam et al. Citation2015; Begashaw et al. Citation2017). Gram-negative bacteria have an envelope consisting of three layers, and the outer membrane is the main reason for most antibiotic resistance. P. aeruginosa is classified by the WHO to be among the critical priority pathogens in terms of resistance (Breijyeh et al., Citation2020). The bacteria, however, presented susceptibility towards the B. boehmii methanol extract with an average zone of inhibition of 20 mm. Comparing the latter results to that of the positive control (19.67 mm), it is evident that phytochemicals from the B. boehmii leaf extract are potential future lead antibiotics against P. aeruginosa.
3.3. Statistical analysis
The results obtained show that the antimicrobial effects of B. bohemia water, methanol and chloroform extracts together with that of the B. bohemia nanoparticle derivatives on burn wound infectious bacteria was statistically significant with a p < 0.05. This analysis suggests that each treatment is different from the positive control, ciprofloxacin. Individual differences in response action toward the treatment are exhibited by the different organisms. The results indicate that the organisms’ action have individual differences as shown in .
Figure 2. Antimicrobial effect of B. boehmii extracts on burn wound bacteria; E. coli (EC), K. pneumoniae (KLE), P. aeruginosa (PA), S. aureus (SA) and S. epidermidis (SE).
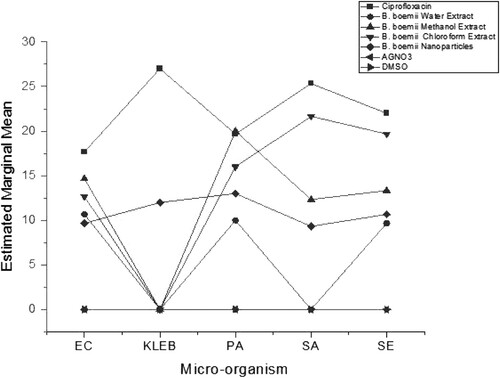
shows the effect of the extracts against those of the control and the silver nanoparticles. The results shown support the hypothesis that the use of plant-based nanoparticles is effective broad-spectrum antibacterial. The silver NPs proved to have antibacterial activity against all the five burn wound infectious bacteria. The action of these nanoparticles is in line with studies that suggest similar action (Ajitha et al. Citation2015; Khatami et al. Citation2015; Espenti et al. Citation2016; Mucheneri et al. Citation2017; Paladini and Pollini Citation2019). Silver destabilises the bacterial plasma membrane, reacting with the sulphur and phosphorus elements, thereby disrupting the bacteria’s metabolic pathways (Rao et al. Citation2016). While silver is known to exhibit antimicrobial activity, the formation of plant-based silver nanoparticles stands to be of benefit as the nanoparticles have a larger surface area as compared to the use of the plant leaf extract. This goes on to prove the efficacy of the use of nanoparticles in comparison to the plant extract alone.
As observed in this study, the silver nanoparticles exhibited comparable antibacterial activity with reference to the plant extract. Silver nanoparticles are said to have a slow and continual release of silver ions, which assist in killing microbes on the burn wound bed (Zarrintaj et al. Citation2017; Yin et al. Citation2020). The silver ions have electrostatic attraction and a high affinity to sulphur proteins, hence they adhere to the cell wall and cytoplasmic membrane leading to disrupted cell permeability and cell death (Yin et al. Citation2020).
The NPs zones of inhibition are slightly less than the plants extract zones in P. aeruginosa, S. aureus, S. epidermidis and E.coli. This may be subject to the concentration of the nanoparticles being several times less than that of the plant extracts. Despite the use of the nanoparticles at a very low concentration of 0.8 ± 0.1 mg/ml, they effectively worked against all the bacteria as compared to the plant extract action at a higher concentration of 100 mg/ml. This is due to the NPs rapid absorption properties that are endorsed by their unique structures, size and morphology (Rai et al. Citation2009; Abdellah et al. Citation2018). Based on a similar study where the effect of concentration on the zone of inhibition was assessed, it is expected that an increase in the nanoparticle concentration may give rise to enhanced antimicrobial activity (Ganesan et al. Citation2019). This has potential in producing an effective antibacterial agent for the treatment of burn wound infections.
4. Conclusion
B. boehmii aqueous, chloroform and methanol generally proved to have antibacterial effects against burn wound infectious bacteria except for K. pneumonia. A zone of inhibition of 20 ± 1.00 mm was obtained in the action of B. boehmii leaf extract against P. aeruginosa. The green synthesised nanoparticles exhibited broad spectrum antibacterial activity, as all the bacteria were susceptible to it at a lesser concentration. The nanoparticles may exert a greater antibacterial activity at a higher dosage, thereby being a potential reliable therapeutic against burn wound infectious bacteria. We, therefore, recommend that further tests be done on characterisation of the nanoparticle for its shape and structure. Toxicity tests are also recommended before formulating into a therapeutic product. Profiling the plant phytochemicals will also assist in determining the main constituents of the extract extracts which are responsible for the antimicrobial activity and the bioreductant potential.
Acknowledgements
We would like to acknowledge Chinhoyi Provincial Hospital for providing the laboratory space. We would also like to acknowledge Pretty Matashu for providing us with ethnobotanical data that was used in this work. Joice Ndlovu and Ryman Shoko conceived and designed the study. Joice Ndlovu identified and collected plant material. Sipho Sibanda performed the laboratory analysis. Sipho Sibanda, Peter Chimwanda, Kudzayi Chishaya and Stephen Nyoni carried out data interpretation. Sipho Sibanda wrote the first version of the manuscript and all authors revised the previous versions of the manuscript critically for intellectual content. All authors have read and agreed to have the final version of the manuscript published.
Disclosure statement
No potential conflict of interest was reported by the author(s).
Data availability statement
The datasets generated during and analysed during the current study are available in the Figshare repository, https://doi.org/10.6084/m9.figshare.19802719.
Additional information
Funding
References
- Abdellah AM, Sliem MA, Bakr M, Amin RM. 2018. Green synthesis and biological activity of silver-curcumin nanoconjugates. Future Med Chem. 10(22):2577–2588.
- Achari A, Somers DO, Champness JN, Bryant PK, Rosemond J, Stammers DK. 1997. Crystal structure of the anti-bacterial sulfonamide drug target dihydropteroate synthase. Nat Struct Biol. 4(6):490–497.
- Ahmed RH, Mustafa DE. 2020. Green synthesis of silver nanoparticles mediated by traditionally used medicinal plants in Sudan. Int Nano Lett. 10(1):1–14. doi:10.1007/s40089-019-00291-9.
- Ahmed S, Ahmad M, Swami BL, Ikram S, Agarwal H, et al. 2016. A review on plants extract mediated synthesis of silver nanoparticles for antimicrobial applications: a green expertise. Resour Technol. 9(1):1–7. doi:10.1016/j.jare.2015.02.007.
- Ahn EY, Jin H, Park Y. 2019. Green synthesis and biological activities of silver nanoparticles prepared by Carpesium cernuum extract. Arch Pharm Res. 42(10):926–934. doi:10.1007/s12272-019-01152-x.
- Ajitha B, Ashok Kumar Reddy Y, Sreedhara Reddy P. 2015. Green synthesis and characterization of silver nanoparticles using Lantana camara leaf extract. Mater Sci Eng C. 49:373–381.
- Azizi S, Ahmad MB, Namvar F, Mohamad R. 2014. Green biosynthesis and characterization of zinc oxide nanoparticles using brown marine macroalga Sargassum muticum aqueous extract. Mater Lett. 116:275–277.
- Begashaw B, Mishra B, Tsegaw A, Shewamene Z. 2017. Methanol leaves extract Hibiscus micranthus Linn exhibited antibacterial and wound healing activities. BMC Complement Altern Med. 17(1):337; [accessed 2020 Aug 11]. http://bmccomplementalternmed.biomedcentral.com/articles/10.1186s12906-017-1841-x.
- Behravan M, Hossein Panahi A, Naghizadeh A, Ziaee M, Mahdavi R, Mirzapour A. 2019. Facile green synthesis of silver nanoparticles using Berberis vulgaris leaf and root aqueous extract and its antibacterial activity. Int J Biol Macromol. 124:148–154.
- Breijyeh Z, Jubeh B, Karaman R. 2020. Resistance of gram-negative bacteria to current antibacterial agents and approaches to resolve it. Molecules. 25(6):1340. doi:10.3390/molecules25061340.
- Budovsky A, Yarmolinsky L, Ben-Shabat S. 2015. Effect of medicinal plants on wound healing. Wound Repair Regen. 23:171–183.
- Chirisa E, Mukanganyama S. 2016. Evaluation of in vitro anti-inflammatory and antioxidant activity of selected Zimbabwean plant extracts. J Herbs Spices Med Plants. 22(2):157–172. doi:10.1080/10496475.2015.1134745.
- Chitemerere T, Mukanganyama S. 2011. In vitro antibacterial activity of selected medicinal plants from Zimbabwe. African J Plant Sci Biotechnol. 5(1):1–7.
- Dai NT, Chang HI, Wang YW, Fu KY, Huang TC, Huang NC, et al. 2018. Restoration of skin pigmentation after deep partial or full-thickness burn injury. Adv Drug Deliv Rev. 123(325):155–164. doi:10.1016/j.addr.2017.10.010.
- Espenti CS, Rao KSVK, Rao KM. 2016. Bio-synthesis and characterization of silver nanoparticles using Terminalia chebula leaf extract and evaluation of its antimicrobial potential. Mater Lett. 174:129–133. doi:10.1016/j.matlet.2016.03.106.
- Ethiraj AS, Jayanthi S, Ramalingam C, Banerjee C. 2016. Control of size and antimicrobial activity of green synthesized silver nanoparticles. Mater Lett. 185:526–529.
- Fankam AG, Kuiate JR, Kuete V. 2015. Antibacterial and antibiotic resistance modifying activity of the extracts from Allanblackia gabonensis, Combretum molle and Gladiolus quartinianus against gram-negative bacteria including multi-drug resistant phenotypes. BMC Complement Altern Med. 15(1). doi:10.1186/s12906-015-0726-0.
- Ganesan S, Mehalingam P, Selvam GS. 2019. Green synthesis of silver nanoparticles from de-oiled rhizomes of Curcuma longa L. and its biomedical potential. Cham: Springer; p. 94–106; [accessed 2020 Sep 10]. https://link.springer.com/chapter/10.1007978-3-030-25135-2_10.
- Hudzicki J. 2009. Kirby-Bauer disk diffusion susceptibility test protocol author information. Am Soc Microbiol. 15:55–63. https://www.asm.org/Protocols/Kirby-Bauer-Disk-Diffusion-Susceptibility-Test-Pro.
- Isabel M, Jossias AD, Maria JS, Natasha R. 2018. Woody species from the Mozambican Miombo woodlands: a review on their ethnomedicinal uses and pharmacological potential. J Med Plants Res. 12(2):15–31.
- Karunanidhi A, Ghaznavi-Rad E, Nathan JJ, Abba Y, Van Belkum A, Neela V. 2017. Allium stipitatum extract exhibits in vivo antibacterial activity against methicillin-resistant staphylococcus aureus and accelerates burn wound healing in a full-thickness murine burn model. Evidence-based complement. Altern Med. 2017:1914732.
- Khatami M, Pourseyedi S, Khatami M, Hamidi H, Zaeifi M, Soltani L. 2015. Synthesis of silver nanoparticles using seed exudates of Sinapis arvensis as a novel bioresource, and evaluation of their antifungal activity. Bioresour Bioprocess. 2(1):19. http://www.bioresourcesbioprocessing.com/content/2/1/19.
- Lachiewicz AM, Hauck CG, Weber DJ, Cairns BA, Van Duin D. 2017. Bacterial infections after burn injuries: impact of multidrug resistance. Clin Infect Dis. 65(12):2130–2136.
- Leaper D, Ayello EA, Carville K, Fletcher J, Keast DH. 2012. International consensus appropriate use of silver. MacGregor L, editor. Wounds Int. London: Kathy Day.
- Maikai V, Kobo P, Maikai B. 2009. Antimicrobial properties of stem bark extracts of Ximenia americana. J Agric Sci. 1(2):30.
- Maroyi A. 2013. Traditional use of medicinal plants in south-central Zimbabwe: review and perspectives. J Ethnobiol Ethnomed. 9(1):1–8.
- Maroyi A. 2016. Ximenia caffra Sond. (Ximeniaceae) in sub-Saharan Africa: a synthesis and review of its medicinal potential. J Ethnopharmacol. 184:81–100. doi:10.1016/j.jep.2016.02.052.
- Ministry of Health and Child Welfare. 2015. EDLIZ 2015 7th essential medicines list and standard treatment guidelines for Zimbabwe. 7th ed. Harare. www.mohcc.gov.zw.
- Mo YY, Tang YK, Wang SY, Lin JM, Zhang HB, Luo DY. 2015. Green synthesis of silver nanoparticles using eucalyptus leaf extract. Mater Lett. 144:165–167.
- Moodley JS, Babu S, Krishna N, Pillay K, Govender P, Indexed S, Aujla GS, Pandey S, Trivedi Y, Ghosh D, et al. 2018. The good and the bad collagens of fibrosis – their role in signaling and organ function. Adv Drug Deliv Rev. 123(1–3):33–64. doi:10.1016/j.addr.2017.11.011.
- Mucheneri N, Muchenje T, Nyoni S, Shumba M, Mupa M, Gwatidzo L, Rahman A. 2017. Green synthesis of silver nanoparticles using Euphorbia confinalis stem extract, characterization and evaluation of antimicrobial activity. J Nanomater Mol Nanotechnol Res. 6(3). doi:10.4172/2324-8777.1000224.
- Mussin J, Giusiano G. 2020. Agricultural, forestry and bioindustry biotechnology and biodiscovery. Cham: Springer.
- Mussin J, Robles-Botero V, Casañas-Pimentel R, Rojas F, Angiolella L, San Martín-Martínez E, et al. 2021. Antimicrobial and cytotoxic activity of green synthesis silver nanoparticles targeting skin and soft tissue infectious agents. Sci Rep. 11(1):1–12. doi:10.1038/s41598-021-94012-y.
- Negut I, Grumezescu V, Grumezescu AM. 2018. Treatment strategies for infected wounds. Molecules. 2(3):1–23.
- Paladini F, Pollini M. 2019. Antimicrobial silver nanoparticles for wound healing application: progress and future trends. Materials. 12(16):2540.
- Palamthodi SM, Gaikwad VJ, Ghasghase N V, Patil SS. 2011. Antibacterial targets in Pseudomonas aeruginosa. Int J Pharm Appl 2(3):159–164. http://www.bipublication.com.
- Pannerselvam B, Dharmalingam Jothinathan MK, Rajenderan M, Perumal P, Pudupalayam Thangavelu K, Kim HJ, et al. 2017. An in vitro study on the burn wound healing activity of cotton fabrics incorporated with phytosynthesized silver nanoparticles in male Wistar albino rats. Eur J Pharm Sci 100:187–196. doi:10.1016/j.ejps.2017.01.015.
- Patra JK, Das G, Fraceto LF, Campos EVR, Rodriguez-Torres MDP, Acosta-Torres LS, et al. 2018. Nano based drug delivery systems: recent developments and future prospects 10 technology 1007 nanotechnology 03 chemical sciences 0306 physical chemistry (incl. structural) 03 chemical sciences 0303 macromolecular and materials chemistry 11 medical and He. J Nanobiotechnology. 16(1):1–33. doi:10.1186/s12951-018-0392-8.
- Permana AD, Anjani QK, Sartini UE, Volpe-Zanutto F, Paredes AJ, et al. 2021. Selective delivery of silver nanoparticles for improved treatment of biofilm skin infection using bacteria-responsive microparticles loaded into dissolving microneedles. Mater Sci Eng C. 120:111786. doi:10.1016/j.msec.2020.111786.
- Queiroz Monte FJ, de Lemos TLG, de Arajo MRS, de Sousa Gomes E. 2012. Ximenia americana: chemistry, pharmacology and biological properties, a review. In: Rao V, editor. Phytochemicals – a global perspective of their role in nutrition and health. InTech. http://www.intechopen.com/books/phytochemicals-a-global- perspective-of-their-role-in-nutrition-and-health/ximenia-americana-chemistry-pharmacology-and-biological- properties-a-review.
- Rai M, Yadav A, Gade A. 2009. Silver nanoparticles as a new generation of antimicrobials. Biotechnol Adv. 27(1):76–83. doi:10.1016/j.biotechadv.2008.09.002.
- Rajendran NK, Kumar SSD, Houreld NN, Abrahamse H. 2018. A review on nanoparticle based treatment for wound healing. J Drug Deliv Sci Technol. 44:421–430.
- Rao NH, Lakshmidevi N, Pammi SVN, Kollu P, Ganapaty S, Lakshmi P. 2016. Green synthesis of silver nanoparticles using methanolic root extracts of Diospyros paniculata and their antimicrobial activities. Mater Sci Eng C. 62:553–557. http://www.ncbi.nlm.nih.gov/pubmed/26952458%5Cnhttp://ac.els-cdn.com/S0928493116300716/1-s2.0-S0928493116300716-main.pdf?_tid=94d79c70-f0c7-11e5-bc74-00000aacb362&acdnat=1458717749_4c3ea9b5783ecd666f675bb3e8007f4e.
- Rex JRS, Muthukumar NM, Selvakumar PM. 2018. Phytochemicals as a potential source for anti-microbial, anti-oxidant and wound healing – a review. MOJ Bioorganic Org Chem. 2(2):61–70.
- Rose LF, Chan RK. 2016. The burn wound microenvironment. Adv wound care. 5(3):106–118. doi:10.1089/wound.2014.0536.
- Rousselle P, Braye F, Dayan G. 2018. Re-epithelialization of adult skin wounds: cellular mechanisms and therapeutic strategies. Adv Drug Deliv Rev. 46:344–365. doi:10.1016/j.addr.2018.06.019.
- Salomoni R. 2017. Antibacterial effect of silver nanoparticles in Pseudomonas aeruginosa. Nanotechnol Sci Appl. 10:115–121.
- Selvakumar PM, Rajkumar RJ, Nadar M. 2018. Plant-derived compounds for wound healing – a review. Org Med Chem Int J. 5(1):1–5.
- Shah A, Amini-Nik S. 2017. The role of phytochemicals in the inflammatory phase of wound healing. Int J Mol Sci. 18(5):1068. doi:10.3390/ijms18051068.
- Singh P, Kim YJ, Zhang D, Yang DC. 2016. Biological synthesis of nanoparticles from plants and microorganisms. Trends Biotechnol. 34:588–599. doi:10.1016/j.tibtech.2016.02.006.
- Thakur R, Jain N, Pathak R, Sandhu SS. 2011. Practices in wound healing studies of plants. Evidence-based complement. Altern Med. 2011:438056.
- Vaghardoost R, Mousavi Majd SG, Tebyanian H, Babavalian H, Malaei L, Niazi M, Javdani A. 2018. The healing effect of sesame oil, camphor and honey on second degree burn wounds in rat. World J Plast Surg 7(1):67–71.
- Wang Y, Beekman J, Hew J, Jackson S, Issler-Fisher AC, Parungao R, et al. 2018. Burn injury: challenges and advances in burn wound healing, infection, pain and scarring. Adv Drug Deliv Rev. 123:3–17. doi:10.1016/j.addr.2017.09.018.
- Wasef LG, Shaheen HM, El-sayed YS, Shalaby TIA, Samak DH. 2019. Effects of silver nanoparticles on burn wound healing in a mouse model. Biol Trace Elem Res. 193(2):456–465. doi:10.1007/s12011-019-01729-z.
- Yaman I, Durmus AS, Ceribasi S, Yaman M. 2010. Effects of Nigella sativa and silver sulfadiazine on burn wound healing in rats. Vet Med. 55(12):619–624.
- Yin IX, Zhang J, Zhao IS, Mei ML, Li Q, Chu CH. 2020. The antibacterial mechanism of silver nanoparticles and its application in dentistry. Int J Nanomedicine. 15:2555–2562.
- Zarrintaj P, Moghaddam AS, Manouchehri S, Atoufi Z, Amiri A, Amirkhani MA, Nilforoushzadeh MA, Saeb MR, Hamblin MR, Mozafari M. 2017. Can regenerative medicine and nanotechnology combine to heal wounds? the search for the ideal wound dressing. Nanomedicine 12(19):2403–2422. doi:10.2217/nnm-2017-0173.