Abstract
Camellia chekiangoleosa Hu. is a unique woody oil tree with significant economic and ornamental value in China, but the fruit is easily sunburned in low-altitude areas. However, few studies on their sunburn mechanism, especially the types and content of endogenous hormones with stress resistance, have not been reported, making it challenging to take reasonable preventive measures. Here, we analyzed the endogenous hormones before sunburn and found that salicylic acid (SA) and gibberellins (GAs) were very significantly correlated between pericarp and seed kernel. However, there weren't closely related to abscisic acid (ABA), auxin, jasmonic acids (JAs), and cytokinin. The content of indole-3-carboxylic acid (ICA) and 3-indolebutyric acid (IBA) in the pericarp was higher than in seed kernel, and IBA was only found in the pericarp. GAs in seed kernel were higher than that in pericarp, and GA3 and GA15 may regulate pericarp development. Contents of trans-Zeatin (tZ), indole-3-acetic acid (IAA), and GAs in seed kernel and pericarp gradually decreased to very low, while ABA and indole-3-carboxaldehyde (ICAld) gradually increased. SA was positively correlated with ABA in pericarp and GAs and SA in seed kernel and pericarp. JAs in fruit generally showed a downward trend, and methyl jasmonate (ME-JA) appeared in pericarp.
Introduction
Camellia chekiangoleosa Hu., a high-quality tree with ornamental, oil, and medicinal uses unique to southern China, blooms mainly in March and is primarily distributed in middle and high-altitude mountainous areas (Liu et al. Citation2007; Liu et al. Citation2007). The oil of seeds is rich in unsaturated fatty acids and nutrients and has significant economic value (Wang et al. Citation2022; Wang et al. Citation2011). So it was promoted that the systemic introduction of the species carried out from high-altitude to low-altitude areas for cultivation. During the introduction process, sunburn of the pericarp often occurs on the sunny side of the fruit under the influence of high temperature and intense light in July, which causes the pericarp to crack with dries up and drops of the fruit. The yield decreases or even becomes extinct, which limits the application of C. chekiangoleosa (He et al. Citation2020). Therefore, an in-depth understanding of the pericarp development and characteristics of C. chekiangoleosa is required to solve the problem of fruit sunburn problem. Most studies on C. chekiangoleosa have focused on seed maturation and oil composition changes, germplasm resource investigation, and ornamental properties (Zhou et al. Citation2019; Liu et al. Citation2015; Liu et al. Citation2015; Huang et al. Citation2022; Yin et al. Citation2021; Xie and Wang Citation2018; Wei et al. Citation2022). However, it was reported that the thickness of pericarp in C. chekiangoleosa after pollination increased quickly from 45 d to 60 d. The pericarp stops growing at 106 d. Lignin accumulates only near the inner seed pericarp, and pericarp lignification occurs inwardly and outwardly. The seeds complete morphological establishment at 75 d, the seed kernel completes development, and the pericarp begins to lignify from 75 d to 106 d. The lignification of the pericarp at 121 d (Yan et al. Citation2020) indicates that the fruit of C. chekiangoleosa development goes through three stages of rapid-slow-stopping growth. Then the pericarp and seeds are about to meet with high summer heat and intense light, so the fruit traits at 60–120 d of the slow growth of young fruits will affect the scorching effect.
Although plant responses to abiotic stresses depend on various factors, plant hormones are the most critical endogenous substances that regulate physiological and molecular responses. These are essential for the survival of plants, which need to regulate their growth and development to survive in a changing environment (Tang et al. Citation2005; Wang et al. Citation2021; Šimura et al. Citation2018). These reported that fruit sunburn is a physiological disorder of fruit induced by excessive radiative heating or exposure to excessive sunlight, caused by humidity, wind speed, fruit adaptation, and cultivation (Floková et al. Citation2014). The types of sunburn include necrosis, browning, and photooxidative (Cui et al. Citation2015; Eriksson et al. Citation2006), which are common in apple, lychee, and pomegranate fruits and cause severe losses (Tang et al. Citation2005; Olsen et al. Citation1997; He and Yamamuro Citation2022). In response to light-stressed environments, fruits employ a variety of physiological and biochemical mechanisms as a complex defense system to reduce injury (Gan et al. Citation2022; Li et al. Citation2020). These environmental stimuli often regulate plant growth and development through phytohormones’ actions (Tang et al. Citation2005). For example, IAA was not directly related to the burning occurrence, but the content at the burn site decreased dramatically by 60%. Moreover, the concentrations of ABA, JA, SA, and ethylene increased significantly (P ≤ 0.05) in burn tissue, but their concentrations (except ethylene) were not affected by sunlight (Torres et al. Citation2017). These findings suggest that hormones regulate fruit sunburn during the development of sunburn symptoms. Fruit responses to bright light and temperature involve complex pathways and interactions. The generation of their physiological disorders is a complex and unstudied process that requires the study of physiological processes before and after the onset of injury by high temperature and bright light. In this study, we explore the dynamic changes in the endogenous hormone content in seed kernels and pericarp of C. chekiangoleosa fruits and clarify the correlation intensity in various endogenous hormones between grain and pericarp. These can help develop more robust strategies to mitigate sunburn to meet the challenges related to sunburn in production.
Materials and methods
Plant materials
The C. chekiangoleosa without disease and healthy growth was taken from Changshan County, Zhejiang Province. We selected the unopened flower buds during March 20-23. Then we peeled off the petals and removed the stamens. Then it was pollinated to stigma with a brush and covered the pollinated flowers with sulfuric acid paper bags. We collected seed kernels (CS) and pericarp (CP) in fruits of C. chekiangoleosa after pollination at 60d (CS1, CP1), 90d (CS2, CP2), and 120d (CS3, CP3). The samples were immediately frozen in liquid nitrogen for 2 h and stored at −80°C for subsequent hormone determination (Figure ). Each test consisted of three biological replicates. Collecting all the samples complies with institutional, national, or international guidelines and legislation. The local forestry management department authorized the collection of all samples for this research. The varieties owner has approved us to use in current research.
Determination of endogenous hormones
Twenty-five hormones, including auxin, gibberellins (GAs), cytokinin (CTK), abscisic acid (ABA), jasmonic acids (JAs), and salicylic acid (SA), were determined using Ultra-high Performance Liquid Chromatography / Mass Spectrometry (UPLC -MS) (Šimura et al. Citation2018; Floková et al. Citation2014; Cui et al. Citation2015). (1) The ultra-low temperature preserved biological material samples were removed and ground (30 Hz, 1 min) to powder form using a grinder (MM 400, Retsch): (2) 50 mg of the ground samples were weighed, added to an appropriate amount of internal standard, and extracted with methanol: water: formic acid = 15:4:1 (v:v:v): (3) The extracts were concentrated and then used 100 µl of 80% methanol–water solution The extracts were re-solubilized with 0.22 µm PTFE filter membrane and placed in a feeding vial for UPLC-MS/MS analysis. Phytohormone contents were determined by MetWare (http://www. metware.cn/) based on the ABSciex QTRAP 6500 LC-MS/MS platform. Each experiment was repeated three times.
Liquid phase conditions: 1) column: Waters ACQUITY UPLC HSS T3 C18 1.8 µm, 2.1 mm*100 mm; 2) mobile phase: ultrapure water (with 0.05% formic acid) for the aqueous phase and acetonitrile (with 0.05% formic acid) for the organic phase; 3) elution gradient: 0 min water/acetonitrile (95:5 V/V), 1 min water/acetonitrile (95:5 V/V), 8.0 min 5:95 V/V, 9.0 min 5:95 V/V, 9.1 min 95:5 V/V. (3) elution gradient: 0 min water/acetonitrile (95:5 V/V), 1 min water/acetonitrile (95:5 V/V), 8.0 min 5:95 V/V, 9.0 min 5:95 V/V, 9.1 min 95:5 V/V, 12.0 min 95:5 V/V; 4) flow rate 0.35 mL/min: column temperature 40 °C: injection volume 2 µL. The mass spectrometry conditions include Electrospray ionization (ESI) at 500 °C, mass spectrometry voltage 4500 V, curtain gas (CUR) at 35 psi, and the collision-activated dissociation (CAD) parameter as medium. In the Q-Trap 6500+, the optimized declustering voltage (CUR) analyzed each ion pair (Table S1).
Data analysis
WPS11.0 was used to record the data. DPS 18.0 (Ruifeng Info Technology Ltd., Hangzhou, China) was used to conduct a one-way Analysis of Variance (ANOVA) of the data of the groups, followed by Duncan’s multiple range test for comparison of specific mean values. The correlation of various hormones was calculated by Pearson’s correlation coefficient.
Results
The difference in varieties and content of auxin in pericarp and seed kernel
The content of IAA in the seed kernels declined during fruit development, with IAA decreasing from 86.10–11.68 ng/g. There are significant differences among stages (Figure ). The content in the pericarp was at a lower level, with IAA in the range of 0.47–0.85 ng/g. Methyl indole-3-acetate (ME-IAA) declined significantly after attaining a maximum value of 3.20 ng/g at CS2 and was elevated at CP3 with only 0.06 ng/g. ICA reached a high value of 1.66 ng/g at CS1. ICAld steadily increased from 0.41–1.65 ng/g with the completion of seed kernel development and ranged from 0.28–0.38 ng/g in the pericarp. IBA was only found in the pericarp, showing a declining trend from 0.67–0.34 ng/g.
Figure 2. Change of content in auxin between seed kernel and pericarp. A. IAA; B. ME-IAA; C. IBA; D. ICA; E. ICAld. Data represent the average of three replicates (n = 3) ± standard erro r. Different letters indicate significant differences by Duncan’s Multiple Range Test at p ≤ 0.05.
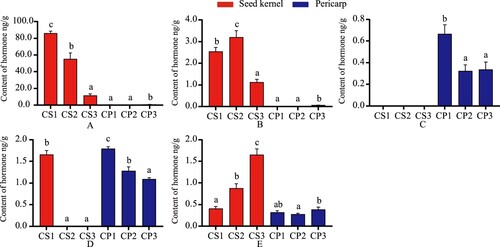
To sum up, IAA, MEIAA, and ICAld have more significance in the seed kernels than in pericarp that the contents of seed kernels are 4–100 times more than in pericarp. ICA and IBA elevated in the pericarp than seed kernels. IBA was a hormone specific to the pericarp, ICA content in the pericarp was higher than in the seed kernels, and ICA was only present in the pericarp later stage.
More content of CTK in seed kernel than pericarp
The changes in tZ and n6-isopentenyladenine (IP) content were detected (Figure ). They were found in both seed kernel and pericarp, with seeds decreasing in content over time from 3.67–0.08 ng/g and IP ranging from 0.11–0.03 ng/g. The content of tZ and IP in pericarp ranged from 0.04–0.11 ng/g and iP in the range of 0.005–0.013 ng/g, with low values occurring in CP2. As fruit morphology gradually stabilizes, the need for cells can be seen. The decrease in the number requirement may lead to low cytokinin content in the pericarp, while the seed morphological establishment period, which makes the cells divide vigorously, requires a higher concentration of cytokinin. However, the content gradually decreases as the seed cavity was gradually filled.
More varieties of GAs in seed kernel than pericarp
The content variation of gibberellin, one of the main hormones for fruit development, is shown in Figure . GA15 was present in all periods within seed kernel and pericarp, with contents ranging from 0.349–1.35 ng/g in seeds and 0.029–0.985 ng/g in pericarp, respectively. GA3 was found in CS1 with 14.167 ng/g, while it was present in all periods of pericarp with contents ranging from 3.793–20.6 ng/g. GA4, GA9, GA24, and GA53 were only found in CS1 and CS2, with content variations ranging from GA4 (2.58-147.333 ng/g), GA9 (3.737-3.81 ng/g), GA24 (5.61-8.563 ng/g) and GA53 (3.84-4.867 ng/g), where GA4 and GA53 decreased with time, and GA9 and GA24 increased in early stage and then decreased in later stage. GA7 was only present in CS1 and was not detected in later stages; GA1, GA19, and GA20 were all found in CS1 and CS2, but it was present in CP1, CP3, and CP2 in pericarp, respectively. In summary, it can be seen that the 10 gibberellins have different roles in each stage of seed kernel and pericarp. Most hormones only appear in the early stage and gradually disappear as the fruit stops growing. GA15 may be the primary hormone involved in the developmental stage of seed kernel and pericarp; GA3 regulates the primary hormone of pericarp growth, while GA1, GA19, and GA20 may be involved in the growth and development of specific parts, which needs to be further investigated.
Figure 4. Change of content in GAs between seeds kernel and pericarp. A. GA1; B. GA3; C. GA4; D. GA7; E. GA9; F. GA15; G. GA19; H. GA20; I. GA24; J. GA53. Data represent the average of three replicates (n = 3) ± standard erro r. Different letters indicate significant di fferences by Duncan’s Multiple Range Test at p ≤ 0.05.
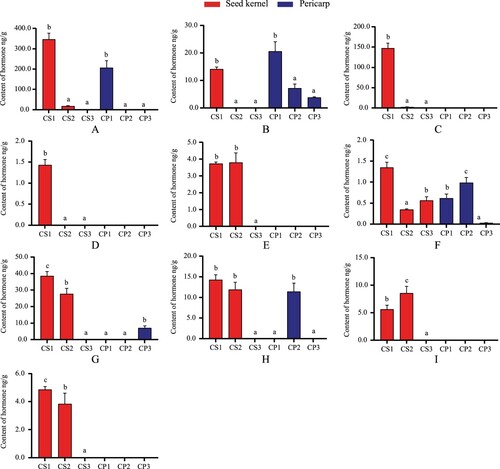
Reverse changes of content in ABA within pericarp and seed kernel
In this study, ABA showed two different patterns of changes in seed kernel and pericarp overall (Figure ). With the completion of seed development, ABA increased incrementally from 4.657 ng/g in CS1 at the beginning to 122 ng/g in CS3, and its content increased approximately 26-fold. ABA in pericarp decreased from 72 in CP1 to 14.9 ng/g in CP2, then increased to 30.833 ng/g in CP3. The above results indicated that ABA maintained a high content in seed kernel and pericarp levels, inhibiting seed development and affecting pericarp development.
The difference in varieties and content of JAs in pericarp and seed kernel
As endogenous substances promote uniform plant growth and development, jasmonic acid inhibits seed germination and regulates fruit ripening and pigment accumulation. This study found high levels of JA and jasmonoyl-l-isoleucine (JA-ILE) in seed kernels and pericarp (Figure ). The JA content in seed kernel and pericarp had the opposite trend. The range of JA in seed kernel was 1.233–88.967 ng/g, and 16.7–15.4 ng/g in pericarp during the same period. The content of JA-ILE in seed kernel and pericarp decreased with fruit growth. The JA-ILE content in seed kernel decreased from 33.3–1.63 ng/g, and from 17.633–3.54 ng/g in the pericarp. ME-JA) was found in CP2, and Dihydrojasmonic acid (H2JA) appeared in CS1and CS2 (Figure ). ME-JA formed by jasmonate is 0.437 ng/g in the peel, which may be related to the high content of JA at this stage. The content of H2JA in the seed kernel was low. After it increased from 0.031–0.106 ng/g, the hormone was not found when the fruit stopped growing.
The similar dynamic changes in SA in seed kernel and pericarp
SA is involved in various metabolic processes and has several roles in regulating fruit ripening, membrane permeability, and ion uptake, improving disease resistance, and signal transduction. With the gradual slowdown of fruit development, the content of SA in seed kernel and pericarp decreased from 134 and 75.267 ng/g at the beginning to 37.533 and 40.2 ng/g, respectively. There were significant differences between seeds kernel and pericarp CP1 in the latter two stages (Figure ). As seen above, although fruit growth and development gradually stopped, SA still had high content in seed kernel and pericarp.
The ratio on (auxin + cytokinin + gibberellin)/ABA
Some studies have found that the signaling networks among different hormones cross each other and regulate the growth and development process of plants through cooperation or antagonism (Cheng et al. Citation2013). Moreover, the ratio after successful pollination is more than 3 times that of failed pollination. With the completion of development, the ratio decreases rapidly. The larger the ratio is, the better the development of seed kernel (Li et al. Citation2020; He et al. Citation2012). The ratio of (auxin + cytokinin + gibberellin)/ABA in pericarp and seed kernel was quickly decreased (Figure ). After pollination, it was relatively high 60 days in the seed kernel to pericarp ratio. However, it showed a rapid downward trend, and the ratio in pericarp was significantly lower than that in seed kernel. The ratio in CS1, CS2, and CS3 decreased to 46.15, 4.695, and 0.126, respectively. The ratio in CP1, CP2, and CP3 decreased from 3.324, 1.485, and 0.449, respectively.
Correlation in various hormones between seed kernel and pericarp
The correlation of the contents of auxin, tZ, GAs, JAs, and SA in seeds kernel and pericarp was performed (Table ). SA and GAs contents were very significantly correlated in seeds kernel and pericarp with correlation coefficients of 0.973 and 0.970, respectively. The correlation coefficients of auxin, tZ, ABA, and JAs were 0.534, 0.530, −0.392, and −0.335, respectively. It could be seen that SA and GAs interacted closely between seed kernel and pericarp. Auxin and tZ, although not reaching significant correlation levels, varied synergistically between seed kernel and pericarp, while the negative correlation between ABA and JAs indicated this group of hormones in seed kernel and pericarp was in contrast to each other.
Table 1. The correlation of various hormone between seed kernel and pericarp in C. chekiangoleosa
There were significant differences in the correlation of various hormones between seed kernel and pericarp (Table S2). As a specific hormone in the pericarp, IBA was significantly correlated with ICA, JAILE, and SA. It is noteworthy that as ABA and SA hormones improve the ability of sun injury resistance, ABA has a significant or significant negative correlation with gibberellin, IAA, ME-IAA, and SA in seed kernel, but a very significant or significant positive correlation in the pericarp. SA has a very significant positive correlation with ICA and JA-ILE and a very significant negative correlation with IP.
Discussion
This study found multiple types of hormones in the pericarp and seeds. There were apparent differences in the contents and types, reflecting the complexity of the growth and development of seed kernel and pericarp of C. chekiangoleosa. The contents of growth hormones IAA and MEIAA in seeds were much higher than those in pericarp and gradually decreased to shallow values. ICAld gradually increased in seed kernel and pericarp, and the contents of ICA and IBA in pericarp were higher than those in seeds. IBA was only found in the pericarp. There were more erythromycin-like hormones in seeds than in pericarp, among which GA4, GA7, GA9, GA24, and GA53 were hormones specific to intra-specific development at this stage, GA3 and GA15 might regulate pericarp development. Changes in total GAs content within seed kernel and pericarp are closely linked, but differences in concentration and hormone type may regulate their respective developmental goals. It has been reported that GA53 in rice generates GA1 through a multi-step oxidation reaction, while GA4 in Arabidopsis directly generates GA1 in the presence of cytochrome P450 genes (CYP72A9). The biological activity of GA1 is about 1000-fold lower than GA4 (Eriksson et al. Citation2006; He et al. Citation2019). The content of GA1 and GA4 varied similarly in this study. At the same time, there were differences in GA53, suggesting that there may be similar variations between GA1 and GA4 as in Arabidopsis, while GA53 may have other roles. GA4 may play an essential role in seed kernel development. It has been reported that GA19, GA20, and GA53 have higher levels under long daylight conditions (Olsen et al. Citation1997), suggesting that hormonal changes are individual transformations regulated by the external environment.
Auxin and gibberellin are essential hormones that promote fruit development. This indicates that these hormones and their interactions may play a key role in regulating fruit development (He and Yamamuro Citation2022). This study found that the IAA and ICAld in the seed kernel synergized with gibberellin except for GA15. In contrast, GA15 only had a synergistic effect with ICA. However, there was a highly significant negative correlation between GA15 and GA20 and IAA in the pericarp, indicating that gibberellins and IAA have different effects. Some plant hormones and plant hormone conjugates, especially GAs and IAA conjugates, may degrade under acidic conditions, resulting in negative regulation (Šimura et al. Citation2018).
As the regulator of cell proliferation, CTK plays an essential role in regulating the growth and development of many fruits interacting with auxin and GAs to regulate pericarp thickness and fruit size (Gan et al. Citation2022). It has been reported that the high ratio of (auxin + gibberellin + cytokinin)/ABA in chestnut and wheatberry fruits may favor seed embryo development and inhibit seed kernel growth (Li et al. Citation2020; He et al. Citation2012). In this study, the ratio of (auxin + GAs + CTK)/ABA maintained a high level at about 60 d after pollination (CS1) and rapidly decreased to a low value. Yan et al. found that fruit growth slowed down at 60 d of pollination, stopped at 120 d, and the seeds were gradually filled with seed kernels during the same period (Huang et al. Citation2022), which is consistent with the results of the present study. The results of the present study also showed that most of the various hormones promoting cell cytokinesis and elongation decreased or even became zero when the fruit stopped growing entirely. ABA and ICAld gradually increased, inhibiting seed germination and inducing chemical defense, respectively (Iven et al. Citation2012; Bednarek et al. Citation2005). As a stress-related hormone, SA could regulate photosynthetic and antioxidant protection, heat tolerance, growth and development, and disease resistance delaying fruit development (Kumar et al. Citation2013; Javanmardi and Akbari Citation2016). This study's correlation coefficient between seed and pericarp contents reached r0.01 > 0.9, and SA was significantly negatively correlated with ABA in seeds and highly positively correlated with ABA in pericarp, suggesting that SA may be involved in regulating seed kernel and pericarp growth and development. As JAs improve stress tolerance, the overall content of the four hormones gradually decreases. However, higher JA and JAILE were observed in seed kernel and pericarp, while H2JA and MEJA were found only in seed kernel and pericarp, respectively. Since JA and MeJA can promote the germination of dormant seeds (Wei et al. Citation2020; Su et al. Citation2020) and inhibit the germination of non-dormant seeds (Wang et al. Citation2020), JA-ILE accumulation may negatively affect ABA biosynthesis. There are negative regulators of JA, and ABA (Garrido-Bigotes et al. Citation2018), which is consistent with the negative regulation between JA-Ile and ABA found in seeds. However, in the pericarp, JA, JA-ILE, and ME-JA have a significant positive correlation with ABA, and their functions need further investigation.
Conclusion
In summary, the seed kernel and pericarp of C. chekiangoleosa fruit, from the slow growth stage to the cessation of growth, have different types of hormones in terms of content. The pericarp has more content in salicylic and jasmonic acid. Gibberellins and salicylic acid have a close relationship between seed kernel and pericarp. Meanwhile, there are not closely related to abscisic, auxin, jasmonic, and cytokinin between seed kernel and pericarp. The content of ICA and IBA in the pericarp was higher than in the seed kernel, and IBA was only found in the pericarp. The GAs content in the seed kernel was higher than that in pericarp, and GA3 and GA15 may play a critical role in regulating pericarp development. CTK, IAA, and GAs contents in seed kernel and pericarp gradually decreased to very low, while ABA and ICAld gradually increased. SA and ABA were significantly negatively correlated in seed kernel and highly positively correlated with ABA in the pericarp. The content of JAs decreased gradually in general. JA and JAILE were higher in the seed kernel and pericarp, and H2JA and ME-JA were found in the seed kernel and pericarp, respectively.
Author contributions
Conceptualization, C.Y.; data curation, G.H. and K.W.; formal analysis, W.L. and X.Y.; investigation, C.Y., G.H. and W.L.; methodology, X.Y. and K.W.; writing (original draft preparation), C.Y. and W.L.; writing (review and editing), W.L. and C.Y.; funding acquisition, W.L.
Supplemental Material
Download Zip (24.9 KB)Acknowledgements
Data summarized in this paper has been generated through the work of several authors and we would like to thank them for their continuous efforts which contribute to the emergence of the C. chekiangoleosa.
Disclosure statement
No potential conflict of interest was reported by the author(s).
Data availability statement (DAS)
Data for the manuscript is available at https://dx.doi.org/10.6084/m9.figshare.21220898
Additional information
Funding
References
- Bednarek P, Schneider B, Svatos A, Svatoš A, Oldham NJ, Hahlbrock K, et al. 2005. Structural complexity, differential response to infection, and tissue specificity of indolic and phenylpropanoid secondary metabolism in arabidopsis roots. Plant Physiol. 138(2):1058–1070.
- Cheng X, Ruyter-Spira C, Bouwmeester H. 2013. The interaction between strigolactones and other plant hormones in the regulation of plant development. Front Plant Sci. 4:199–215.
- Cui KY, Lin YY, Zhou X, Li SC, Liu H, Zeng F, Zhu F, Ouyang G, Zeng Z, et al. 2015. Comparison of sample pretreatment methods for the determination of multiple phytohormones in plant samples by liquid chromatography–electrospray ionization-tandem mass spectrometry. Microchem J. 121:25–31.
- Eriksson S, BöHlenius H, Moritz T, Nilsson O, et al. 2006. GA4 is the active Gibberellin in the regulation ofLEAFYTranscription and arabidopsis floral initiation. Plant Cell. 18(9):2172–2181.
- Floková K, Tarkowská D, Miersch O, Strnad M, Wasternack C, Novák O, et al. 2014. UHPLC-MS/MS based target profiling of stressinduced phytohormones. Phytochemistry. 105:147–157.
- Gan LJ, Song MY, Wang XC, Yang N, Li H, Liu X, Li Y. 2022. Cytokinins are involved in regulation of tomato pericarp thickness and fruit size. Hortic. Res. 9:uhab041.
- Garrido-Bigotes A, Figueroa PM, Figueroa CR. 2018. Jasmonate metabolism and its relationship with abscisic acid during strawberry fruit development and ripening. J Plant Growth Regul. 37:101–113.
- He H, Yamamuro C. 2022. Interplays between auxin and GA signaling coordinate early fruit development. Hortic Res 9:uhab078.
- He J, Chen Q, Xin P, Yuan J, Ma Y, Wang X, Xu M, Chu J, Peters RJ, Wang G, et al. 2019. CYP72A enzymes catalyse 13-hydrolyzation of gibberellins. Nat Plants. 5:1057–1065.
- He JH, Ma FW, Shu HR. 2012. The change of endogenous hormones in ‘Jinhuang’ mango fruit with normal and aborted embryo. Acta. Hortic. Sin. 39(6):1167–1174. (in chinese).
- He YC, Wu MJ, Dong L. 2020. Analysis of kernel oil content and variation of fatty acid composition of Camellia chekiangoleosa in the main producing areas. Nonwood Forest Research. 38(3):37–45. (in chinese).
- Huang B, Wang Z, Huang J, Li X, Zhu H, Wen Q, Xu L-a, et al. 2022. Population genetic structure analysis reveals significant genetic differentiation of the endemic species Camellia chekiangoleosa Hu. with a narrow geographic range. Forests. 13(2):234.
- Iven T, König S, Singh S, Braus-Stromeyer SA, Bischoff M, Tietze LF, Braus GH, Lipka V, Feussner I, Dröge-Laser W. 2012. Transcriptional activation and production of tryptophan-derived secondary metabolites in Arabidopsis roots contributes to the defense against the fungal vascular pathogen Verticillium longisporum. Mol Plant. 5(6):1389–1402.
- Javanmardi J, Akbari N. 2016. Salicylic acid at different plant growth stages affects secondary metabolites and phisico-chemical parameters of greenhouse tomato. Advances. Horticultural. Sci. 30(3):151–158.
- Kumar D, Mishra DS, Chakraborty B, Kumar P, et al. 2013. Pericarp browning and quality management of litchi fruit by antioxidants and salicylic acid during ambient storage. J Food Sci Technol. 50(4):797–802.
- Li L, Su SC, Kou YR. 2020. Fruit-set and early fruit development in chestnut are associated with endogenous hormones contents. J. Northeast. For. Univ. 48(5):55–61. (in chinese).
- Liu Q, Gao HZ, Yao XH. 2015. Variation of tree growth traits of Camellia chekiangoleosa Hu. clones in low altitude area. Hubei Agric. Sci. 54(5):1105–1108. (in chinese).
- Liu Q, Yao XH, Wang KL. 2015. Flowering phenology of Camellia chekiangoleosa clone in low altitude area. For Res 28(2):249–254. (in chinese).
- Liu ZL, Yang SP, Yao XH. 2007. Variation and correlativity analysis of fruit shape of Camellia chekiangoleosa. For. Res. 20(2):263–266. (in chinese).
- Liu ZL, Yao XH, Yang SP, et al. 2007. Study of economic character variation in Camellia chekiangoleosa Hu. J. Southwest. Univ.(Natural Science Edition). 29(4):83–88. (in chinese).
- Olsen EJ, Junttila O, Moritz T. 1997. Long-day induced bud break in salix pentandra is associated with transiently elevated levels of ga1 and gradual increase in indole-3-acetic acid. Plant Cell Physiol. 38(5):536–540.
- Šimura J, Antoniadi I, Široká J, Tarkowská D, Strnad M, Ljung K, Novák O, et al. 2018. Plant hormonomics: multiple phytohormone profiling by targeted metabolomics. Plant Physiol. 177(2):476–489.
- Su YN, Wang RJ, Cai JB. 2020. Effects of jasmonic acid pretreatment on perennial ryegrass seed germination under heat stress. Pratacultural. Sci. 37(4):658–668. (in chinese).
- Tang J, Feng JL, Yang ZJ. 2005. Changes of endogenous hormones in fruit and their effects on the fruit development of camellia oleifera. J. For. Envir. 35(004):331–336.
- Torres CA, Sepúlveda G, Kahlaoui B. 2017. Phytohormone interaction modulating fruit responses to photooxidative and heat stress on apple (Malus domestica Borkh.). Front Plant Sci. 8:2129.
- Wang KL, Cao FL, Yao XH. 2011. Chemical composition of fatty acid from Camellia chekiangoleosa Hu. J. Nanjing. For. Univ. (Natural Science Edition). 35(2):131–134. (in chinese).
- Wang Q, Hu J, Yang T, Chang S. 2021. Anatomy and lignin deposition of stone cell in Camellia oleifera shell during the young stage. Protoplasma. 258:361–370.
- Wang Y, Hou Y, Qiu J, Tang L, Tong X, Zhang J. 2020. Abscisic acid promotes jasmonic acid biosynthesis via a ‘SAPK10-bZIP72- AOC’ pathway to synergistically inhibit seed germination in rice (Oryza sativa). New Phytol. 228(4):1336–1353.
- Wang Z, Huang B, Ye J, Tang S, Wen Q, et al. 2022. Comparative transcriptomic analysis reveals genes related to the rapid accumulation of oleic acid in camellia chekiangoleosa, an oil tea plant with early maturity and large fruit. Plant Physiol Biochem. 171:95–104. in chinese.
- Wei T, Dong L, Zhong S, Jing H, Deng Z, Wen Q, Li J. 2022. Chemical composition of Camellia chekiangoleosa Hu. seeds during ripening and evaluations of seed oils quality. Ind Crops Prod. 177:114499.
- Wei ZJ, Niu BJ, Wang YX. 2020. Effect of methyl jasmonate on seed germination and seedling growth of Medicago sativa ‘Pianguan’ under salt stress. Acta. Agrestia. Sinica. 28(4):998–1005. (in chinese).
- Xie Y, Wang X. 2018. Comparative transcriptomic analysis identifies genes responsible for fruit count and oil yield in the oil tea plant Camellia chekiangoleosa. Sci Rep 8(1):1–10.
- Yan C, Yao XH, Yin HF. 2020. Fruit development dynamics and lignin accumulation law of oil tea. Acta. Agric. Univ. Jiangxiensis (Natural Sciences Edition). 42(4):788–801. (in chinese).
- Yin X, Li T, Huang B, Xu L, Wen Q, et al. 2021. Complete chloroplast genome of camellia chekiangoleosa (Theaceae), a shrub with gorgeous flowers and rich seed oil. Mitochondrial DNA Part B. 6(3):840–841.
- Zhou WC, Xiao XY, Shen JL, et al. 2019. Review on germplasm resources and breeding strategy for camellia chekiangoleosa. Nanfang. For. Sci. 47(6):20–24. (in chinese).