Abstract
Osteosarcoma (OS), a common bone malignancy, is highly metastatic and featured by poor prognosis. SUMOylation is deeply involved in tumorigenesis and cancer progression. SUMO-activating enzyme (SAE) inhibitor has been found to play an anti-proliferative role in multiple cancers. However, their functions in osteosarcoma remain unknown. Based on this, it was hypothesized that ML792, an SAE inhibitor, might be an anti-tumor candidate against osteosarcoma in this paper. The role of ML792 in the treatment of osteosarcoma was investigated in vitro and in vivo. The results showed ML792 significantly arrested human osteosarcoma cells (HOCs) at the G2/M phase, inhibited the proliferation of HOCs, and elicited apoptosis. To better assess the inhibitory role of ML792 in human osteosarcoma, we induced HOCs with TGF-β1. Our study showed that ML792 significantly suppressed the migration, invasion, and EMT of HOCs induced by TGF-β1. Moreover, ML792 inhibited TGF-β1-induced phosphatidylinositol- 3-kinase (PI3K) and AKT activation, as well as Smad2/3 phosphorylation in HOCs. In vivo, ML792 inhibits the growth of osteosarcoma. Thus, ML792 is a promising new antitumor agent to suppress the progress of OS cells undergoing proliferation and EMT by targeting the PI3K/Akt and TGF-β1/Smad pathway and may prevent or reduce the growth and metastasis of osteosarcoma.
Introduction
Osteosarcoma (OS) is the most common primary sarcoma of bone, primarily diagnosed in children, adolescents, and young adults. Although it is rare, due to the early metastasis and drug resistance of osteosarcoma, the 5-year survival rate after recurrence and metastasis is <20% (Eccles and Welch Citation2007; Bielack et al. Citation2009). Despite the continuous advances in treatment for osteosarcoma such as chemotherapy (Bishop et al. Citation2016) and radical resection, outcomes of osteosarcoma cases are still poor, and the 5-year overall survival rate of patients with osteosarcoma is still low (Dean et al. Citation2018). For this reason, it is necessary to find novel molecular targeted compounds for cases with this disease.
TGF-β, a secretory cytokine, has been revealed to play important roles in tumor progression via regulating cell apoptosis, migration, and proliferation. Recent studies have shown that TGF- β also acts as a tumor promoter by stimulating epithelial-mesenchymal transition (EMT) (Hao et al. Citation2019). EMT is a physiological process that is involved in cancer chemoresistance and metastasis (Du and Shim Citation2016). The TGF-β cytokine family covers 3 subtypes, TGF-β3, TGF-β2, and TGF-β1, each of which has a specific expression pattern and performs different functions. For instance, TGF-β1 enhances the metastatic and invasive properties of various tumor types. Several studies have shown that high TGF-β1 expression is positively correlated with cancer progression and metastasis in prostate cancer, breast cancer, colorectal cancer, and other tumor types (Padua and Massagué Citation2009), suggesting that TGF-β1 induces EMT in cancer cells (Xu et al. Citation2009). In addition, TGF-β1 can activate cell metastasis via Smad signaling (Roberts et al. Citation2006; Moustakas and Heldin Citation2007; Xu et al. Citation2009) and non-Smad signaling pathway, such as PI3K (phosphoinositide 3-kinase)/AKT (Bakin et al. Citation2000; Baek et al. Citation2017), which is one of the most frequently activated pathways in cancer (Lu et al. Citation2003; Knight and Shokat Citation2007). Moreover, previous studies have shown that TGF-β1 levels are elevated in osteosarcoma (Tu et al. Citation2014). Therefore, it may be possible to effectively inhibit the EMT of osteosarcoma by inhibiting TGF-β1/Smad and PI3K/AKT signaling.
Small ubiquitin-like modifiers (SUMOs) are a class of post-translational modification proteins conjugated to many enzymes which are related to the regulation of cellular activities. These enzymes cover E3 ligase, Ubc9 (E2-conjugated enzyme), and SAE (SUMO-activating enzyme E1), facilitating SUMO transfer from Ubc9 to specific proteins (Seeler and Dejean Citation2017). A great number of studies had shown that the disorder of SUMOylation or deSUMOylation process is related to the development and progression of cancer (Geiss-Friedlander and Melchior Citation2007; Hendriks et al. Citation2017). Of note, noticeably increased expressions of SUMO pathway components are found in several human tumors (Wu et al. Citation2009; Zhao et al. Citation2012), suggesting that SUMOylation is required to initiate or sustain tumorigenesis. In recent years, a series of studies have found that SUMOylation is closely related to TGF-β1/Smad signaling pathway. For example, SUMOylation enhances the affinity of TbRI kinase to Smad protein and facilitates phosphorylation and activation of Smad2/3 under the action of TGF-β1 (Kim and Baek Citation2009). Moreover, SMAD4 mainly mediates TGF-β/SMAD signaling, and Smad4 SUMOylation may prevent Smad4 from ubiquitylation, thus enhancing TGF-β1/Smad signaling (Xu et al. Citation2016). Similarly, several studies have indicated critical roles of SUMOylation events in the regulation of PI3K/AKT signaling pathway (Reidick et al. Citation2015). Thus, the regulation of SUMOylation in tumor cells may be a novel and feasible treatment.
ML792, a potent and selective inhibitor of SAE, potentially inhibits SUMOylation, which might be used to treat some MYC-amplified malignant tumors (He et al. Citation2017; Schneekloth Citation2017), such as breast cancer. Nevertheless, the role of ML792, as a SUMO scavenger, in osteosarcoma cell lines remains to be investigated, and the PI3K/AKT and TGF-β/SMAD pathway may be regulated by SUMOylation. Hence, the objective of this study was to evaluate the possible effects of ML792 on osteosarcoma cells, especially TGF-β1-induced EMT, and to investigate its acting mechanism(s).
Methods
Cell culture
Human osteosarcoma cells (HOCs)-HOS and U2OS were provided by The National Collection of Authenticated Cell Cultures (Shanghai, China). The U2OS cells were cultured in RPMI-1640, and HOS cells were cultured in Dulbecco’s Modified Eagle Medium (DMEM) which contained FBS (fetal bovine serum; 10%) and streptomycin-penicillin (1%) at thirty-seven centigrade degrees in an atmosphere of 5% carbon dioxide. The medium was renewed twice a week until the cells were used in the following procedures.
Cell proliferation assay
Using Cell Counting Kit-8 (CCK-8), the cell proliferation and viability were determined. U2OS and HOS cells were added onto a 96-well plate (5 × 103 cells in each well), respectively. After 24 h, ML792 (0, 0.07, 0.156, 0.312, 0.625, 1.25, 2.5, 5, 10 and 20 μmol/L) was added into cells and incubated for an indicated period (24 and 48 h) in a 37°C, 5% CO2 incubator. Then, the cells were incubated with CCK-8 (10 µl/well) for 1 h at 37°C, the optical density value was detected at 450 nm. All assays were performed in triplicate.
Colony formation
Onto a 6-well plate cells were added (1,000 cells in each well). After one day, ML792 (0.25, 0.5, or 1.0 μmol/L) was added into cells for treatment, which were then incubated for half a month until colony formation. Paraformaldehyde (4%) was used to fix the cells for twenty minutes after PBS washing twice, and crystal violet (0.1%) was used to stain the cells for half an hour. The colony number was determined using microscopy.
Flow cytometry
HOS and U2OS cells were added into a 6-well plate (5 × 105 cells in each well), respectively. One day later, ML792, or control medium was added into the cells for 24-hour treatment. Digestion of the cells was performed, followed by centrifugation and washing twice. Then, the cells were fixed using cold ethanol (75%) for ≥2 h. The cells were subjected to incubation with RNase A (100 μg/mL) and propidium iodide (PI; 50 μg/mL) for half an hour in the dark after PBS washing twice. Accuri C6 (provided by BD Biosciences) was used to determine cell distribution in diverse cell cycles. For the determination of apoptosis, adherent cells and the cells in the medium were collected. The cells were washed with PBS twice and incubated with PI and FITC-conjugated Annexin V for fifteen minutes in the dark. Accuri C6 was used to analyze apoptosis.
Scratch wound healing
HOS and U2OS cells were added to a 6-well plate, respectively. When confluency of about 80% was reached, the dishes were scraped straightly using a pipet (p200) tip, and then rinsed with PBS three times for debris removal, followed by incubation with fresh culture medium (including1% FBS) and treatment with ML792 or TGF-β1 for one day. At 0 and 24 h, images of cell migration were captured, respectively. The wound area was observed under an optical microscope. The wound-healing index (percentage) was determined using 5 distances (randomly selected) across the wound at 0 and 24 h, which was divided by the distance measured at 0 h.
Transwell assay
A 24-well Transwell chamber with Matrigel was used to assess cell invasion and migration. In brief, U2OS and HOS cells were seeded (density, 1 × 106 cells/well) on the upper surface of the Transwell chamber (8.0 mm pores; Corning, NY, USA), and treated with ML792 or TGF-β1 for 24 h. We removed the cells in the upper chamber. The Paraformaldehyde Fix Solution (4%) was used to fix invaded cells, which were next stained by crystal violet and counted under a high-power microscope. And the number of invaded cells was counted in 5 randomly selected fields.
Western blot
Onto a 6-well plate cells were added, followed by culture in the complete medium until reaching confluency. The total proteins from HOS and U2OS cells were resolved using SDS-PAGE (Bio-Rad, Hercules, America). After membrane transfer (PVDF; provided by Millipore, Billerica, America), dry nonfat milk (5%) in TBS plus Tween (TBS-T, 0.1%) was used to block the membranes at room temperature for two hours, followed by incubation overnight with the primary antibody at four centigrade degree and incubation with the secondary HRP-conjugated antibodies (Abcam, Cambridge, America) at room temperature for one hour. The protein bands were visualized using Western enhanced chemiluminescence substrate and the Odyssey Infrared Imaging System (Li-COR, USA).
Immunofluorescent staining
Onto 24-well plates cells were added, and ML792 was added half an hour before treatment with or without TGF-β1(10 ng/ml). After culture with ML792 (1.0 μmol/L) for one day and subsequent PBS washing three times, the cells were fixed in paraformaldehyde (4%) for one hour. The cells were then penetrated for fifteen minutes and blocked for one hour before being incubated at 4°C overnight with primary antibodies. The cells were incubated with goat anti-mouse IgG (H + L) Alexa Fluor 488 (1:500, Invitrogen, Thermo Fisher, USA) for one hour. DAPI (Beyotime, China) was used to stain nuclei for five minutes at room temperature. Fluorescence microscopy (Nikon 80i, Japan) was used to observe the cells.
Tumor xenografts in vivo
6-week-old female BALB/c-nude mice were purchased from SLAC Laboratory Animal CO. LTD (Shanghai, China). Hos cells were suspended in culture media at a density of 1.0 × 106/ml and subcutaneously transplanted onto the nude mice (200 ul). After weighing, the nude mice were randomly divided into three groups (6 in each group): control group, 50 mg/kg ML792, and 100 mg/kg ML792. Treatment begins when subcutaneous tumors form. The intraperitoneal administration regimen was two days a week. Tumors were measured weekly using calipers. The tumor volume (V) was calculated by applying the following equation: v = 0.5 × (L × W2). The nude mice were killed before the tumor reached ethical size. All animal experiments have been approved by the animal ethics committee of Taizhou hospital, Zhejiang Province.
Statistics
SPSS 26.0 statistical software was used for analysis, and mean ± SD was used to describe the related data. The student’s t-test was used for unpaired data. P < 0.05 indicated significant difference.
Results
ML792 suppresses the proliferation of HOCs
HOCs were treated with various concentrations of ML792 (0, 0.078, 0.156, 0.312, 0.625, 1.25, 2.5, 5, 10 or 20μmol/L) for 24 and 48 h, respectively. The results demonstrated that the IC50 value for ML792 was 0.21 μmol/L at 24 h and 0.70 µmol/L at 48 h in HOS cells, and 0.40 µmol/L at 24 h and 0.34 µmol/L at 48 h in U2OS cells, respectively (Figure A and B). Moreover, we found that ML792 can inhibit the colony-forming ability of OS cells in a dose-dependent manner (0.25, 0.5 and 1.0 µmol/L) (Figure C and D). These observations demonstrate that ML792 inhibited the proliferation of HOCs.
ML792 induces the apoptosis of HOCs
To study the mechanism of ML792 inhibiting cell viability, the effect of ML792 on apoptosis was analyzed by flow cytometry (Figure E and F). The results showed the number of PI-positive and annexin V-FITC-positive cells was elevated with the rise of ML792 concentrations (0.25, 0.5, 1.0 µmol/L) (Figure E and F). To delineate the role of the Bcl-2 family in ML792-induced apoptosis, the protein expression was analyzed using Western blot (Figure G–I). After 24-hour ML792 treatment, the expression level of anti-apoptotic protein Bcl-2 was significantly down-regulated and the expression level of Pro-apoptotic protein Bax was increased. Exposed to ML792 of 1.0 µmol/L, the expression of Bax in HOS and U2OS cells was increased by 69.8% and 198.7% respectively, and the expression of Bcl-2 was decreased by 53.1% and 44.5%, respectively.
Figure 1. ML792 represses proliferation and induces apoptosis of human osteosarcoma cells (HOCs). (A and B) The anti-proliferative effect of ML792 on HOCs was analyzed using the Cell Counting Kit-8 assays. (C and D) Colony-forming assays of HOCs. (E and F) HOCs were stained with Annexin V- fluorescein isothiocyanate (FITC) / propidium iodide (PI), and analyzed the percentage of the apoptotic cells was determined by flow cytometry. (G-I) The effects of ML792 on the expressions of Bax and Bcl-2 proteins were evaluated by Western blot. *P < 0.05, **P < 0.01 vs ML792 (0 µmol/L) group.
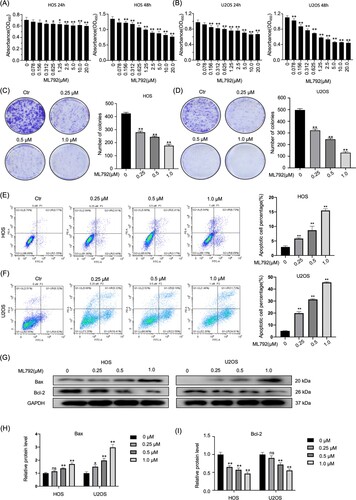
ML792 induces G2 phase arrest of HOCs
Using flow cytometry, we evaluated the distribution of U2OS and HOS cells at diverse stages of the cell cycle with different concentrations (0.25, 0.5, 1.0 µmol/L) of ML792 after 24 h (Figure A and B). It was found that ML792 treatment significantly inhibited osteosarcoma cells at the G2/M phase (Figure A and B). Moreover, expressions of proteins associated with cell cycle were studied by Western blot (Figure C–F), and it was found that ML792 elevated cyclin B1 levels (340% and 351% in HOS and U2OS, respectively), but reduced the expression of CDK4 (56.5% and 57.1% in HOS and U2OS, respectively) and cyclin D1 (48.5% and 74.6% in HOS and U2OS, respectively).
Figure 2. ML792 induces cell cycle arrest in the G2 phase. (A and B) Human osteosarcoma cells (HOCs) were stained with propidium iodide and evaluated the cell cycle by flow cytometry. (C-F) Western blot was used to evaluate the cell cycle-associated proteins. *P < 0.05, **P < 0.01 vs ML792(0 µmol/L) group.
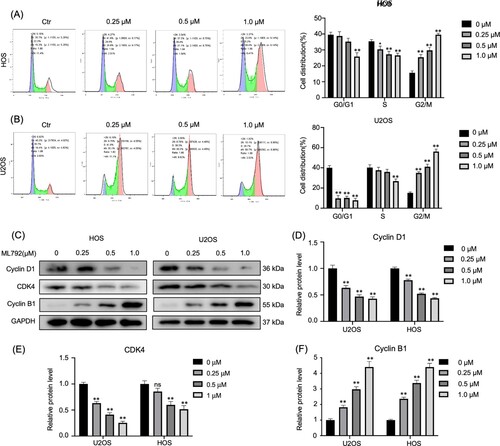
ML792 represses invasion, migration, and EMT phenotype in HOCs
Using ML792 after 24-hour incubation, we evaluated the invasion and migration of HOCs by Transwell and wound-healing assays. The results of wound-healing indicated that ML792 suppressed cell migration in a dose-dependent manner (0.25, 0.5, 1.0 µmol/L) (Figure A and B). Similarly, Transwell analyses indicated that ML792 decreased the relative invasion of HOS and U2OS cells, which was dependent on dose (Figure C and D). Next, we evaluated expressions of protein, which were involved in the invasion and migration of osteosarcoma cells. It was found that ML792 increased E-cadherin levels, and decreased protein levels of vimentin and N-cadherin (mesenchymal markers), slug, MMP2, snail, and MMP9, which was dependent on dose (Figure A–F). Consistent with these results, immunofluorescence analysis revealed that the expression of Vimentin and N-cadherin was decreased and the level of E-cadherin was increased after ML792 treatment (1.0 µmol/L) (Figure G).
Figure 3. ML792 represses the invasion, migration, and epithelial-mesenchymal transition (EMT) of human osteosarcoma cells (HOCs). (A and B) Scratch wound healing was performed for evaluating cell migration. Magnification, ×100. (C and D) Transwell assays were used to analyze cell invasion and migration ability. Magnification, 100x. *P < 0.05, **P < 0.01 vs ML792(0 µmol/L) group.
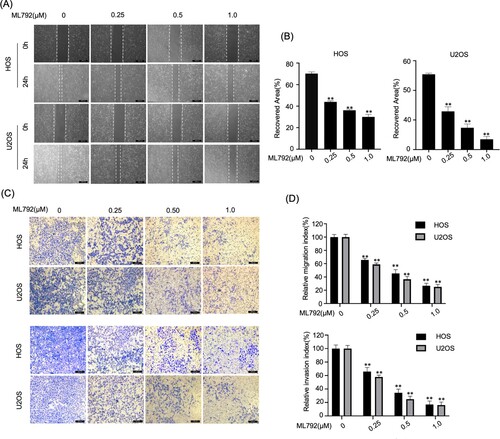
Figure 4. ML792 rebalances epithelial-mesenchymal transition (EMT)-related proteins in human osteosarcoma cells (HOCs). (A–F) Migration, invasion, and EMT-related proteins were analyzed by Western blot. (G) Immunofluorescence was used to analyze the protein expressions of Vimentin, N-cadherin, and E-cadherin in HOCs. Magnification, 200x. *P < 0.05, **P < 0.01 vs ML792(0 µmol/L) group.
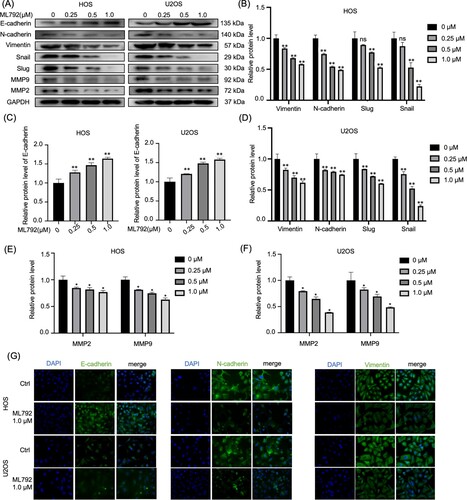
ML792 suppresses invasion, migration, and EMT of HOCs elicited by TGF-β1
Since EMT is mainly facilitated by TGF-β1, the suppressive effect of ML792 on TGF-β1-elicited invasion and migration of HOCs was explored. ML792 (1.0 µmol/L) with or without TGF-β1 (10 ng/ml) was used for the experiments below. It was found that ML792 suppressed the migration of osteosarcoma cells treated by TGF-β1 (Figure A and B). Similarly, ML792 restrained HOCs crossing the chamber under the treatment of TGF-β1 (Figure C and D). To investigate the mechanism of ML792 acting on EMT elicited by TGF-β1, we examined protein levels of E-cadherin, N-cadherin, vimentin, snail, slug, MMP2, and MMP9. The results indicated noticeably reduced protein levels of E-cadherin in the TGF-β1 treatment group (10 ng/ml), and increased levels of Vimentin, N-cadherin, slug, Snail, MMP2, and MMP9, which were rebalanced by ML792 (1.0 µmol/L) (Figure A–H). Moreover, immunofluorescence analysis showed the same results (Figure I).
Figure 5. ML-792 inhibits TGF-β1-induced invasion and migration of human osteosarcoma cells (HOCs). HOCs were treated with or without TGF-β1(10 ng/mL) in the absence or presence of ML792(1 µmol/L) for 24 h. (A and B) Scratch wound healing was used to evaluate cell migration. Magnification, 100x. (C and D) Transwell assays were used to analyze cell invasion ability. Magnification, 100x. *P < 0.05, **P < 0.01. TGF-β1, transforming growth factor-β1.
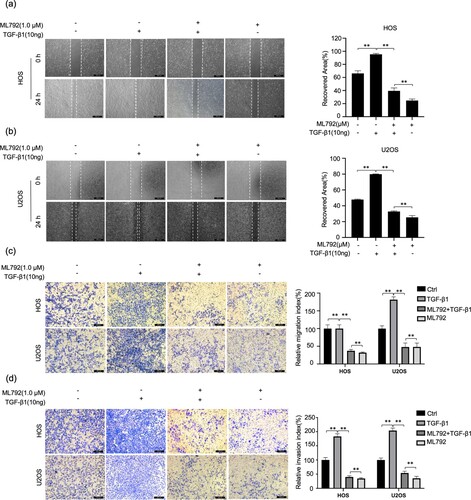
Figure 6. ML-792 rebalances EMT-related proteins in human osteosarcoma cells (HOCs) induced by TGF-β1. HOCs were treated with or without TGF-β1(10 ng/mL) in the absence or presence of ML792(1 µmol/L) for 24 h. (A-H) The expressions of invasion, migration, and EMT proteins were evaluated by Western blot. (I) Immunofluorescence was used to analyze the protein expression of Vimentin, N-cadherin, and E-cadherin in HOCs. Magnification, 200x. *P < 0.05, **P < 0.01. TGF-β1, transforming growth factor-β1.
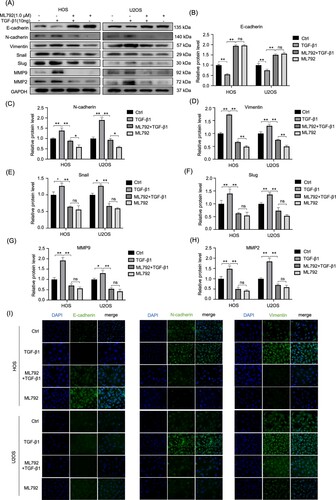
ML792 abrogates the PI3K/AKT pathway in HOCs
It has been reported that PI3K/AKT regulates cell migration and proliferation through a variety of pathways, and TGF-β activates the AKT pathway. Hence, using Western blot, we detected protein expressions of p-PI3K/PI3K and p-AKT/AKT in HOCs cells after treatment of ML792 (1.0 µmol/L) with or without TGF-β1 (10 ng/ml) for one day (Figure A–C). The results showed that ML792 blocked the TGF-β1-activated AKT pathway.
ML792 impaired the TGF-β1/Smad pathway in HOCs
For TGF-β superfamily members, Smads are critical signal transducers. Protein expressions of p-Smad2/3 and Smad2/3 in HOCs were examined after being treated by ML792 (1.0 µmol/L) with or without TGF-β1 (10 ng/ml) for one day, and the effects of ML792 on the TGF-β1/Smad pathway were investigated. TGF-β1 (10 ng/ml) increased the protein level of p-Smad2/3 and Smad4, which was reversed by ML792 (Figure A, D, and E).
ML792 represses the growth of xenograft tumors
To confirm the antitumor effect of ML792, we established a subcutaneous tumorigenic model in nude mice by injecting HOS cells. Our results showed that compared with the control group, ML792 significantly inhibited the growth of tumors (Figure F and G), and the effect of the high concentration group was more obvious.
Figure 7. ML792 abrogates the TGF-β1- elicited PI3K/AKT and TGF-β1/Smad pathway in human osteosarcoma cells (HOCs) and inhibits the growth of osteosarcoma in vivo. HOCs were treated with or without TGF-β1(10 ng/mL) in the absence or presence of ML792(1 µmol/L) for 24 h. (A) The expressions of PI3K/AKT and TGF-β1/Smad pathway-related proteins were analyzed by Western blot. (B-E) Quantitative analyses of Western blots. (F)Tumor size. (G)The change of tumor volume and weight. (H) Chemical structure of ML792. (I) Schematic diagram of the mechanism by which ML792 works. *P < 0.05, **P < 0.01. TGF-β1, transforming growth factor-β1.
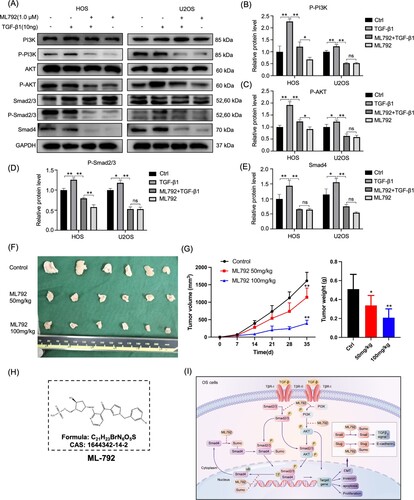
Discussion
ML792 (Figure H) is a SAE1 inhibitor, which has been reported to have potential applications in the treatment of various human tumors such as human breast cancer, human malignant melanoma, and human colon cancer (He et al. Citation2017). However, its role in osteosarcoma has not been reported previously. The present study found that ML792 inhibited the proliferation of HOCs by promoting apoptosis and cell cycle arrest in the G2/M phase. Furthermore, ML792 suppressed the invasion, migration, and EMT of TGF-β1-treated HOCs. Additionally, ML792 inhibited PI3K/AKT signaling and the TGF-β1/Smad pathway in HOCs treated with TGF-β1. Taken together, our results suggest that ML792 may suppress the proliferation and EMT of osteosarcoma cells in part by inhibiting the PI3K/Akt and TGF-β1/Smad pathways.
Rapid cell division is one of the most important hallmarks of tumors. In cells, cell division is promoted by CDKs (cyclin-dependent kinases) interacting with cyclins (Ingham and Schwartz Citation2017). We found that ML792 suppressed the proliferation, elicited cell cycle arrest in the G2/M phase, the levels of cyclin D1 and CDK4, and increased the levels of cyclin B1 (a G2/M arrest marker) in HOCs, suggesting that the anti-proliferative property of ML792 is achieved by arresting mitosis. We also found that ML792 promoted the apoptosis of HOCs, which was dependent on dose. In detail, ML792 decreased the anti-apoptotic protein Bcl-2, which was accompanied by increased pro-apoptotic protein Bax, finally suppressing the proliferation and accelerated apoptosis of HOCs. An increase in the number of apoptotic cells may have been the reason for cell cycle arrest in the G2 phase.
EMT is a process by which epithelial cells acquire mesenchymal properties. In tumors, EMT is linked to malignant progression, tumor initiation, progression, migration, intravasation into the blood vessels, tumor metastasis, and resistance to therapy (Pastushenko and Blanpain Citation2019). The molecular mechanisms regulating EMT are complex. Matrix metalloproteinases (MMPs) are essential for many pathophysiological processes and are necessary for tumor invasion and metastasis. In the tumor microenvironment, raised levels of MMPs, such as MMP-9, can elicit EMT, while EMT itself promotes the secretion of MMP-9 and promotes cell metastasis and invasion (Galindo-Hernandez et al. Citation2014). We found that ML792 inhibited the scratch wound healing of HOS and U2OS cells, which was dependent on concentration, and prevented HOS and U2OS cells from passing through the transwell chamber. After treatment with ML792, the invasion and migration of HOCs elicited by TGF-β1 were also weakened. In line with these results, ML792 reduced the expression of MMP-9 and MMP-2 in HOS and U2OS cells both in the absence and presence of TGF-β1. In addition, we also found that ML792 inhibited the increased Vimentin and N-cadherin in HOS and U2OS cells stimulated by TGF-β1, and the reduced E-cadherin expression. The facts above indicated ML792 inhibited the EMT of HOCs and may reduce the invasion and migration of tumor cells by inhibiting the TGF-β1-induced EMT, which in part may be due to down-regulation of MMP-2 and MMP-9.
The cell adhesion protein E-cadherin is silenced in many malignant tumors, and the loss of E-cadherin expression is a common event in EMT (Mendonsa et al. Citation2018). Several transcription factors related to EMT regulate the expressions of E-cadherin. For example, Slug and Snail suppress E-cadherin expression in tumor cells, leading to EMT (Yang et al. Citation2019b), and both of these transcription factors are involved in TGF-β-induced EMT in osteosarcoma cells. SUMOylation has been suggested to increase the stabilization of Slug and Snail and thereby promoted suppress E-cadherin expression (Chanda et al. Citation2018). Our results showed that ML792 inhibited the down-regulation of E-cadherin expression elicited by TGF-β1, and also reduced expressions of Snail1 and Slug elicited by TGF-β1 in HOS and U2OS cells. Therefore, ML792 may attenuate TGF-β1-induced down-regulation of E-cadherin expression by negatively regulating Snail and Slug, thereby inhibiting EMT in HOCs.
TGF-β1-mediated signal transduction functions to promote EMT in many cancers such as lung cancer (David et al. Citation2016). Extracellular TGF-β acts via binding to its two transmembrane receptors (TGFβ-RI and TGFβ-RII), which recruit diverse signaling pathways including the canonical Smad, GTPase, MAPK, and PI3K/AKT pathways (Brandl et al. Citation2010; Boudreau et al. Citation2012; Lamouille et al. Citation2014; David et al. Citation2016; Meng et al. Citation2016). Smads play a key role in the signaling pathway induced by TGF-β1, leading to EMT (Jiang et al. Citation2019). SUMOylation regulates several components of the TGF-β1 signaling pathway. SUMOylation of the TGFβ-RI (which requires ligand binding as well as the TGFβ-RII) has been reported to enhance downstream signaling (Wang et al. Citation2018). SUMOylation has been shown to increase the affinity of TbRI kinase to Smad protein and facilitate Smad2/3 phosphorylation and activation (Kim and Baek Citation2009). SUMOylation of Smad4 inhibits its ubiquitylation and thereby enhances TGF-β1/Smad signaling (Xu et al. Citation2016), and Smad4 SUMOylation has been implicated in the resistance of glioma cells to ionizing radiation (Wang et al. Citation2018). Additionally, SUMOylation of Smad nuclear interacting protein-1 reverses its inhibitory effects on TGF-β1 signaling to promote TGF-β-regulated cell migration and invasion (Liu et al. Citation2016). In agreement with the above studies, we observed that the expressions of p-Smad2/3 and Smad4 proteins were reduced by ML792 in TGF-β1-treated HOCs. Therefore, our findings support the possibility that ML792 inhibits the SUMOylation of components of the TGF-β1 pathway and thereby reduces TGF-β1-mediated signaling, which in turn suppresses the proliferation, migration, and EMT of osteosarcoma cells. However, the effects of ML792 may also be mediated via other proteins that undergo SUMOylation. For example, SAE1 was reported to promote human glioma progression by enhancing AKT SUMOylation-mediated signaling, and silencing of SAE1 was observed to elicit cell cycle arrest at the G2 phase and apoptosis (Yang et al. Citation2019a).
ML792 also significantly reduced the expressions of p-PI3K and p-AKT in TGF-β1-treated HOS and U2OS cells. AKT signaling is known to be involved in tumorigenesis and affects the growth and apoptosis of cancer cells (Bunney and Katan Citation2010). Furthermore, it has been reported to regulate EMT by regulating the critical signal molecules, e.g. PI3K and AKT (Baek et al. Citation2017; Luo et al. Citation2018). Taken together, our results suggest that ML792 can prevent TGF-β1-elicited invasion and migration of HOCs by suppressing PI3K/AKT and TGF-β1/Smad signaling and inhibiting EMT. Additionally, ML792 may also promote the apoptosis process of HOCs and elicit cell cycle arrest at the G2 phase via its regulation of the TGF-β1/Smad and PI3K/AKT pathways (Figure I).
The same results have also been confirmed in other tumors. For example, Kroonen et al. treated chondrosarcoma cells with ML792 for 24 h and found that they also blocked the G2 / M phase of the cell cycle and inhibited cell proliferation (Kroonen et al. Citation2021). The difference was that they detected the level of SUMOylation in human chondrosarcoma tissue and the level of SUMOylation in chondrosarcoma cells after ML792 treatment. In this experiment, we focus on detecting the changes of cell signaling pathway-related proteins after ml792 treatment, which is a lack of data support from clinical samples, which is one of the limitations of this experiment. In addition, our experiment did not evaluate the correlation between drug resistance and the effect of ML792 on osteosarcoma.
In summary, we found that ML792 suppressed the invasion, migration, and EMT of TGF-β1-treated HOCs. Additionally, ML792 inhibited PI3K/AKT and TGF-β1/Smad signaling in TGF-β1-treated HOCs. Our results suggest that inhibition of SUMOylation by ML792 may attenuate PI3K/Akt and TGF-β1/Smad signaling and thereby suppress the proliferation and EMT of osteosarcoma cells. Although strong inhibition of cellular SUMOylation brings a clear risk, temporary inhibition might elicit tumor-specific weaknesses, such as susceptibility to senescence and/or apoptosis (Seeler and Dejean Citation2017). Therefore, ML792 is potentially used in the targeted treatment of osteosarcoma and other cancer. Our findings remain to be further verified by more experiments.
Author contribution
The work reported in the paper has been performed by the authors, unless clearly specified in the text. Xiangang Jin and Hua Yin: conceptualization. Xiangang Jin and Jiaqian Bao: data curation. Xiangang Jin, Xiaoting Song and Feng Lu: formal analysis. Junbo Liang: funding acquisition. Junbo Liang: supervision. Xiangang Jin: writing – original draft. Hua Yin and Junbo Liang: writing – review & editing.
Acknowledgments
Thanks for the experimental platform provided by the public laboratory of Taizhou Hospital of Zhejiang University (Zhejiang, China)
Data availability statement
That data that supports the findings of this study are available at the figshare repository (https://figshare.com/) at https://doi.org/10.6084/m9.figshare.20218667.v5.
Disclosure statement
No potential conflict of interest was reported by the author(s).
Additional information
Funding
References
- Baek SH, Ko JH, Lee JH, Kim C, Lee H, Nam D, Lee J, Lee S-G, Yang WM, Um J-Y, et al. 2017. Ginkgolic acid inhibits invasion and migration and TGF-β-induced EMT of lung cancer cells through PI3K/Akt/mTOR inactivation. J Cell Physiol. 232(2):346–354. doi:10.1002/jcp.25426.
- Bakin AV, Tomlinson AK, Bhowmick NA, Moses HL, Arteaga CL. 2000. Phosphatidylinositol 3-kinase function is required for transforming growth factor β-mediated epithelial to mesenchymal transition and cell migration. J Biol Chem. 275(47):36803–10. doi:10.1074/jbc.M005912200.
- Bielack S, Carrle D, Casali PG. 2009. Osteosarcoma: ESMO clinical recommendations for diagnosis, treatment and follow-up. Ann Oncol. 20 Suppl 4:iv137–9. doi:10.1093/annonc/mdp154.
- Bishop MW, Janeway KA, Gorlick R. 2016. Future directions in the treatment of osteosarcoma. Curr Opin Pediatr. 28(1):26–33. doi:10.1097/MOP.0000000000000298.
- Boudreau HE, Casterline BW, Rada B, Korzeniowska A, Leto TL. 2012. Nox4 involvement in TGF-beta and SMAD3-driven induction of the epithelial-to-mesenchymal transition and migration of breast epithelial cells. Free Radic Biol Med. 53(7):1489–99. doi:10.1016/j.freeradbiomed.2012.06.016.
- Brandl M, Seidler B, Haller F, Adamski J, Schmid RM, Saur D, Schneider G. 2010. IKKα controls canonical TGFβ–SMAD signaling to regulate genes expressing SNAIL and SLUG during EMT in Panc1 cells. J Cell Sci. 123(Pt 24):4231–9. doi:10.1242/jcs.071100.
- Bunney TD, Katan M. 2010. Phosphoinositide signalling in cancer: beyond PI3K and PTEN. Nat Rev Cancer. 10(5):342–52. doi:10.1038/nrc2842.
- Chanda A, Sarkar A, Bonni S. 2018. The SUMO system and TGFβ signaling interplay in regulation of epithelial-mesenchymal transition: implications for cancer progression. Cancers (Basel). 10(8). doi:10.3390/cancers10080264
- David CJ, Huang YH, Chen M, Su J, Zou Y, Bardeesy N, Iacobuzio-Donahue C, Massagué J. 2016. TGF-β tumor suppression through a lethal EMT. Cell. 164(5):1015–30. doi:10.1016/j.cell.2016.01.009.
- Dean DC, Shen S, Hornicek FJ, Duan Z. 2018. From genomics to metabolomics: emerging metastatic biomarkers in osteosarcoma. Cancer Metastasis Rev. 37(4):719–731. doi:10.1007/s10555-018-9763-8.
- Du B, Shim JS. 2016. Targeting epithelial-mesenchymal transition (EMT) to overcome drug resistance in cancer. Molecules. 21(7). doi:10.3390/molecules21070965
- Eccles SA, Welch DR. 2007. Metastasis: recent discoveries and novel treatment strategies. Lancet. 369(9574):1742–57. doi:10.1016/S0140-6736(07)60781-8.
- Galindo-Hernandez O, Serna-Marquez N, Castillo-Sanchez R, Salazar EP. 2014. Extracellular vesicles from MDA-MB-231 breast cancer cells stimulated with linoleic acid promote an EMT-like process in MCF10A cells. Prostaglandins Leukot Essent Fatty Acids. 91(6):299–310. doi:10.1016/j.plefa.2014.09.002.
- Geiss-Friedlander R, Melchior F. 2007. Concepts in sumoylation: a decade on. Nat Rev Mol Cell Biol. 8(12):947–56. doi:10.1038/nrm2293.
- Hao Y, Baker D, Ten Dijke P. 2019. TGF-β-mediated epithelial-mesenchymal transition and cancer metastasis. Int J Mol Sci. 20(11). doi:10.3390/ijms20112767
- He X, Riceberg J, Soucy T, Koenig E, Minissale J, Gallery M, Bernard H, Yang X, Liao H, Rabino C, et al. 2017. Probing the roles of SUMOylation in cancer cell biology by using a selective SAE inhibitor. Nat Chem Biol. 13(11):1164–1171. doi:10.1038/nchembio.2463.
- Hendriks IA, Lyon D, Young C, Jensen LJ, Vertegaal ACO, Nielsen ML. 2017. Site-specific mapping of the human SUMO proteome reveals co-modification with phosphorylation. Nat Struct Mol Biol. 24(3):325–336. doi:10.1038/nsmb.3366.
- Ingham M, Schwartz GK. 2017. Cell-cycle therapeutics come of age. J Clin Oncol. 35(25):2949–2959. doi:10.1200/JCO.2016.69.0032.
- Jiang X, Zhang Z, Song C, Deng H, Yang R, Zhou L, Sun Y, Zhang Q. 2019. Glaucocalyxin a reverses EMT and TGF-β1-induced EMT by inhibiting TGF-β1/Smad2/3 signaling pathway in osteosarcoma. Chem Biol Interact. 307:158–166. doi:10.1016/j.cbi.2019.05.005.
- Kim KI, Baek SH. 2009. International review of cell and molecular biology. Int Rev Cell Mol Biol. 273:265–311. doi:10.1016/S1937-6448(08)01807-8.
- Knight ZA, Shokat KM. 2007. Chemically targeting the PI3K family. Biochem Soc Trans. 35(Pt 2):245–9. doi:10.1042/BST0350245.
- Kroonen JS, Kruisselbrink AB, Briaire-de Bruijn IH, Olaofe Olaejirinde O, Bovée Judith VMG, Vertegaal Alfred CO. 2021. SUMOylation is associated with aggressive behavior in chondrosarcoma of bone. Cancers (Basel). 13(15). doi:10.3390/cancers13153823
- Lamouille S, Xu J, Derynck R. 2014. Molecular mechanisms of epithelial-mesenchymal transition. Nat Rev Mol Cell Biol. 15(3):178–96. doi:10.1038/nrm3758.
- Liu S, Long J, Yuan B, Zheng M, Xiao M, Xu J, Lin X, Feng X-H. 2016. SUMO modification reverses inhibitory effects of smad nuclear interacting protein-1 in TGF-β responses. J Biol Chem. 291(47):24418–24430. doi:10.1074/jbc.M116.755850.
- Lu Y, Wang H, Mills GB. 2003. Targeting PI3K-AKT pathway for cancer therapy. Rev Clin Exp Hematol. 7(2):205–28.
- Luo J, Yao JF, Deng XF, Zheng X-D, Jia M, Wang Y-Q, Huang Y, Zhu J-H. 2018. 14, 15-EET induces breast cancer cell EMT and cisplatin resistance by up-regulating integrin αvβ3 and activating FAK/PI3K/AKT signaling. J Exp Clin Cancer Res. 37(1):23. doi:10.1186/s13046-018-0694-6.
- Mendonsa AM, Na TY, Gumbiner BM. 2018. E-cadherin in contact inhibition and cancer. Oncogene. 37(35):4769–4780. doi:10.1038/s41388-018-0304-2.
- Meng XM, Nikolic-Paterson DJ, Lan HY. 2016. TGF-β: the master regulator of fibrosis. Nat Rev Nephrol. 12(6):325–38. doi:10.1038/nrneph.2016.48.
- Moustakas A, Heldin CH. 2007. Signaling networks guiding epithelial–mesenchymal transitions during embryogenesis and cancer progression. Cancer Sci. 98(10):1512–20. doi:10.1111/j.1349-7006.2007.00550.x.
- Padua D, Massagué J. 2009. Roles of TGFβ in metastasis. Cell Res. 19(1):89–102. doi:10.1038/cr.2008.316.
- Pastushenko I, Blanpain C. 2019. EMT transition states during tumor progression and metastasis. Trends Cell Biol. 29(3):212–226. doi:10.1016/j.tcb.2018.12.001.
- Reidick C, El Magraoui F, Meyer HE, Stenmark H, Platta H. 2015. Regulation of the tumor-suppressor function of the class III phosphatidylinositol 3-kinase complex by ubiquitin and SUMO. Cancers (Basel). 7(1):1–29. doi:10.3390/cancers7010001.
- Roberts AB, Tian F, Byfield SD, Stuelten C, Ooshima A, Saika S, Flanders K. 2006. Smad3 is key to TGF-β-mediated epithelial-to-mesenchymal transition, fibrosis, tumor suppression and metastasis. Cytokine Growth Factor Rev.17(1-2):19–27. doi:10.1016/j.cytogfr.2005.09.008.
- Schneekloth JS Jr. 2017. Controlling protein SUMOylation. Nat Chem Biol. 13(11):1141–1142. doi:10.1038/nchembio.2496.
- Seeler JS, Dejean A. 2017. SUMO and the robustness of cancer. Nat Rev Cancer. 17(3):184–197. doi:10.1038/nrc.2016.143.
- Tu B, Peng ZX, Fan QM, Du L, Yan W, Tang T-T. 2014. Osteosarcoma cells promote the production of pro-tumor cytokines in mesenchymal stem cells by inhibiting their osteogenic differentiation through the TGF-β/Smad2/3 pathway. Exp Cell Res. 320(1):164–73. doi:10.1016/j.yexcr.2013.10.013.
- Wang Z, Wang K, Wang R, Liu X. 2018. SUMOylation regulates TGF-β1/Smad4 signalling in-resistant glioma cells. Anticancer Drugs. 29(2):136–144. doi:10.1097/CAD.0000000-000000578.
- Wu F, Zhu S, Ding Y, Beck WT, Mo Y-Y. 2009. MicroRNA-mediated regulation of Ubc9 expression in cancer cells. Clin Cancer Res. 15(5):1550–7. doi:10.1158/1078-0432.CCR-08-0820.
- Xu J, Lamouille S, Derynck R. 2009. TGF-β-induced epithelial to mesenchymal transition. Cell Res. 19(2):156–72. doi:10.1038/cr.2009.5.
- Xu P, Lin X, Feng XH. 2016. Posttranslational regulation of Smads. Cold Spring Harb Perspect Biol. 8(12). doi:10.1101/cshperspect.a022087
- Yang Y, Liang Z, Xia Z, Wang X, Ma Y, Sheng Z, Gu Q, Shen G, Zhou L, Zhu H, et al. 2019a. SAE1 promotes human glioma progression through activating AKT SUMOylation-mediated signaling pathways. Cell Commun Signal. 17(1):82. doi:10.1186/s12964-019-0392-9.
- Yang Y, Zhang P, Yan R, et al. 2019b. MnTE-2-PyP attenuates TGF-β-induced epithelial-mesenchymal transition of colorectal cancer cells by inhibiting the Smad2/3 signaling pathway. Oxid Med Cell Longev. 2019:8639791. doi:10.1155/2019/8639791.
- Zhao Z, Tan X, Zhao A, Zhu L, Yin B, Yuan J, Qiang B, Peng X. 2012. microRNA-214-mediated UBC9 expression in glioma. BMB Rep. 45(11):641–6. doi:10.5483/BMBRep.2012.45.11.097.