Abstract
Urothelial carcinoma (UC) is the tenth most diagnosed cancer in humans worldwide. Dogs are a robust model for invasive UC as tumor development and progression is similar in humans and dogs. Recent studies in humans have revealed alterations in urine microbiota in individuals with UC; however, microbial alterations in dogs with UC have not been evaluated. The objective of this pilot study was to compare the urine and fecal microbiota of dogs with UC and matched healthy controls. DNA was extracted from urine and fecal samples followed by 16S rRNA sequencing and analyses using QIIME2 and R. Dogs with UC had significantly decreased microbial diversity (Kruskal–Wallis; Shannon, p = 0.048) and altered microbial composition (PERMANOVA: Unweighted UniFrac, p = 0.011) in urine, but not fecal samples. The relative abundance of Fusobacterium was also increased, although not significantly, in urine and fecal samples of dogs with UC. A comparison of canine and human urine microbiota further revealed similarities in dominant microbial taxa across both host species. This study supports the value of dogs as a model for studies on bladder cancer and urine microbiota, and it provides a foundation for future work exploring host-microbe dynamics in UC carcinogenesis, prognosis, and treatment.
Introduction
Bladder cancer is the tenth most diagnosed cancer worldwide (World Bladder Cancer Patient Coalition. GLOBOCAN (Citation2020)). In 2020, the International Agency for Research on Cancer estimated over 573,000 new bladder cancer diagnoses would be confirmed worldwide (Sung et al. Citation2021). Urothelial carcinoma (UC), also known as transitional cell carcinoma, is the most common type of bladder cancer. Age (being over age 55), race (white), sex (male), and some heritable mutations (Randi et al. (Citation2007); Aben et al. (Citation2002); Murta-Nascimento et al. (Citation2007); Mueller et al. (Citation2008); Chu et al. (Citation2013); Mucci et al. (Citation2016); Martin et al. (Citation2018); Aveyard et al. (Citation1999)) are established risk factors for bladder cancer (Antoni et al. (Citation2017); American Cancer Society. Key Statistics for Bladder Cancer [Internet] (Citation2021); Wang et al. (Citation2018)). Bladder cancer is also strongly associated with environmental exposures such as smoking (Cumberbatch et al. (Citation2016); Alguacil et al. (Citation2011); Burger et al. (Citation2013); Wu et al. (Citation2018)) or occupational exposure to chemicals like aromatic amines, pesticides, industrial dyes, or diesel fumes (Pesch et al. (Citation2014); Koutros et al. (Citation2016)). However, not all persons exposed to these chemicals develop urothelial carcinoma indicating that there are individualized host-environment interactions that mediate UC risk.
Clear host-environment (diet) interactions mediated through the gut microbiome have emerged in colorectal carcinogenesis (O’Keefe (Citation2016); Sears and Garrett (Citation2014)) and environment-microbiome-carcinogenesis links have also begun emerging in lung cancer (Mao et al. (Citation2018); Ramírez-Labrada et al. (Citation2020)). For example, diets high in animal fat can directly or indirectly impact microbial composition by increasing liver bile acid production and excretion into the intestines. Bile tolerant microbes or microbes that can metabolize primary bile acids expand in this bile-rich environment, and some of these microbes produce pro-inflammatory, cytotoxic, or genotoxic secondary metabolites that can contribute to colorectal carcinogenesis. Work on the gut microbiome has far outpaced and outnumbered studies on the urine / bladder microbiome; however, it has now become apparent that the urine microbiota play a key role in host health and may also be influencing bladder cancer development and progression (Aragon et al. (Citation2018)).
Alterations in urine microbiota have been reported in association with multiple genitourinary diseases including chronic kidney disease (Kramer et al. (Citation2018)), chronic prostatitis, chronic pelvic pain syndrome (Shoskes et al. (Citation2016)), interstitial cystitis (Siddiqui et al. (Citation2012)), sexually transmitted infections (Nelson et al. (Citation2010)), urgency urinary incontinence (Pearce et al. (Citation2014)), urinary tract infections (Magruder et al. (Citation2019)), urinary stone disease (Zampini et al. (Citation2019)), urogenital schistosomiasis (Adebayo et al. (Citation2017)), urogynecologic surgery (Fok et al. (Citation2018)), and vaginosis (Gottschick et al. (Citation2017)). A few recent studies on the urine / bladder microbiome have also revealed subtle but intriguing differences in urine or bladder tissue microbial diversity and composition of individuals with and without UC (Table ) (Wu et al. (Citation2018); Bi et al. (Citation2019); Pederzoli et al. (Citation2020); Liu et al. (Citation2019); Bučević Popović et al. (Citation2018); Chipollini et al. (Citation2020); Mai et al. (Citation2019); Oresta et al. (Citation2021); Xu et al. (Citation2014); Chen et al. (Citation2021); Hussein et al. (Citation2021); Mansour et al. (Citation2020)), but approaches and results in these studies vary widely and require additional examination. The effects of gut microbiota on bladder health is another area that requires further attention, as there are compelling studies demonstrating the links between gut microbiota and urinary tract infections (Magruder et al. (Citation2019); Worby et al. (Citation2022)). Moreover, short chain fatty acids (SCFA) or other metabolites produced by gut microbes may affect inflammation levels in distal organs such as the bladder or lungs (Trompette et al. (Citation2014)). Studies in relevant animal models could advance this research by offering a more controlled environment; however, rodent models of UC have many limitations (Ding et al. (Citation2014)). Canine models of UC, on the other hand, exhibit cancer heterogeneity, molecular features, muscle invasive cancer behavior, and host immunocompetence similar to humans (Connelly et al. (Citation2019); Chaitman et al. (Citation2020); Manchester et al. (Citation2019)). Moreover, humans and dogs share many of the same environmental exposures, and canine UC, like human UC, has been epidemiologically linked to chemical exposures including herbicides and pesticides (De Brot et al. (Citation2018); Glickman et al. (Citation2004)). Notably, the human microbiome is more similar to the dog microbiome compared to other animal models, such as the rodent microbiome (Coelho et al. (Citation2018)), making dogs a more suitable model for studying microbiota in relation to UC.
Table 1. Key findings in 13 publications about the urine / tissue microbiota and urothelial carcinoma.
Here, we used a naturally-occurring canine model of bladder cancer to compare the urine and fecal microbiota of dogs with and without UC. We broadly hypothesize that microbes could be directly or indirectly contributing to bladder cancer pathogenesis by altering the bladder environment (Magruder et al. (Citation2019); Worby et al. (Citation2022); Reid et al. (Citation2001)). Our first step in testing this hypothesis was to characterize the microbiota. We predicted that microbial community profiles would differ between dogs with and without UC.
Materials and methods
Sample collection
Approval for the use of dogs in this study was obtained at Purdue University (IACUC: 1111000169) and Ohio State University (IACUC: 2019A00000005). This study was carried out and reported according to ARRIVE guidelines. All dogs were recruited through Purdue University College of Veterinary Medicine between September 2016 and October 2019. Urine and fecal samples were initially collected from 57 dogs with biopsy-confirmed urothelial carcinoma (UC) and 56 age-, sex-, and breed-matched healthy controls (Figure ). Dogs with active urinary tract infections or other co-morbidities (e.g. diabetes) were excluded. We additionally excluded any dog with a history of chemotherapy (vinblastine, zebularine, vemurafenib, chlorambucil, mitoxantrone, and cyclophosphamide) or a history of antibiotics within the previous three weeks (Montassier et al. (Citation2015); Stringer et al. (Citation2013); Stewardson et al. (Citation2015); Suchodolski et al. (Citation2009); Connelly et al. (Citation2019); Chaitman et al. (Citation2020); Manchester et al. (Citation2019)). We did not exclude dogs on non-steroidal anti-inflammatory drugs (NSAIDs), including piroxicam and deracoxib, which are commonly used in dogs with UC. Healthy dogs underwent physical exams and had no history or indications of gastrointestinal or urogenital disease.
In healthy dogs, urine was collected via mid-stream free catch. In dogs with UC, a variety of urine collection methods were employed as deemed clinically appropriate including: mid-stream free catch, catheter, or cystoscopy. Free catch urine can include bacteria from the bladder, urethra, periurethral skin, prepuce, or vagina, while urine collected via catheterization or cystoscopy primarily includes microbes from the bladder and limits the presence of genital and skin microbes (Oresta et al. (Citation2021); Wolfe et al. (Citation2012); Bajic et al. (Citation2020); Hourigan et al. (Citation2020)). To determine if collection method could potentially influence our results, we compared samples from dogs with UC collected via mid-stream free catch (n = 8) to samples collected via non-free catch methods (catheterization, cystoscopy) (n = 11) (S1 Table; S1-S3 Figs). We observed significant differences in microbial composition but not diversity by collection method (Bray–Curtis PERMANOVA rarefied: p = 0.008; non-rarefied: p = 0.005; S1f and S2f Figs). Moreover, Staphylococcus and Streptococcus – common skin colonizers - were amongst the top genera in mid-stream free catch urine but not amongst the top genera in non-free catch urine (S2 Table). Based on the compositional differences we observed by collection method and on other studies that have reported differences in urine microbiota due to collection method (Oresta et al. (Citation2021); Wolfe et al. (Citation2012); Bajic et al. (Citation2020); Hourigan et al. (Citation2020); Pohl et al. (Citation2020); Chen et al. (Citation2020)), we opted to limit the remainder of our analyses to samples collected via mid-stream free catch only. This allowed us to compare microbiota in urine from healthy dogs and dogs with UC without introducing collection method as a potential confounder.
As such, after exclusions, we analyzed mid-stream free-catch urine samples from a total 7 dogs with UC and 7 age-, sex-, and breed-matched healthy controls (Table ). To determine if bladder cancer affected fecal microbial diversity and composition, we analyzed fecal samples from a subset of the dogs whose urine samples were selected for analysis (4 dogs with UC and 6 healthy dogs) (Magruder et al. (Citation2019); Flores-Mireles et al. (Citation2015); Paalanne et al. (Citation2018)). We also evaluated fecal microbiota in a larger validation cohort of 30 dogs with UC and 30 healthy dogs. All urine and stool samples were placed on ice immediately after collection and then transferred into a −80°C freezer. Samples were transported on dry ice from Purdue (West Lafayette, IN, USA) to the Ohio State University (Columbus, OH, USA), where they were stored in at −80°C until extraction.
Table 2. Demographics of dogs with and without urothelial carcinoma (UC).
Urine samples were collected and analyzed from all dogs. Stool samples were collected and analyzed from a subset of these dogs including 6 healthy (4 females, 2 males), and 4 with UC (3 females, 1 male).
DNA extraction and quantification
Urine samples were extracted using QIAamp® BiOstic® Bacteremia DNA Isolation Kit (Qiagen, Hilden, Germany) as described previously (Mrofchak et al. (Citation2021a)). Fecal samples were extracted using the QIAamp® PowerFecal® DNA Kit (Qiagen, Hilden, Germany) following the manufacturer’s instructions. Negative (no sample) controls were run with each kit used for extraction. DNA concentrations were measured using a Qubit® 4.0 Fluorometer (Invitrogen, Thermo Fisher ScientificTM, Carlsbad, CA, USA) and purity was assessed using Nanodrop One (Thermo Fisher ScientificTM, Carlsbad, CA, USA).
16S rRNA sequencing and sequence processing
Library preparation, PCR amplification, and amplicon sequencing was performed at Argonne National Laboratory (DuPage County, Illinois). Likewise, negative controls underwent the full extraction, library preparation, and sequencing process. We amplified the V4 region of the 16S rRNA gene using primers 515F and 806R, and PCR and sequencing were performed as described previously (2 × 250 bp paired-end reads, on an Illumina Miseq (Lemont, IL, USA)) (Mrofchak et al. (Citation2021a); Caporaso et al. (Citation2012); Caporaso et al. (Citation2011)). Raw, paired-end sequence reads were processed using QIIME2 v. 2020.11 and DADA2 (Bolyen et al. (Citation2019); Callahan et al. (Citation2016)). Taxonomy was assigned in QIIME2 using the Silva 132 99% database and the 515F / 806R classifier (Yilmaz et al. (Citation2014); Quast et al. (Citation2013)). In the analysis comparing urine collection method in dogs with UC, we excluded samples with fewer than 1,000 reads and analyzed the data with rarefaction (at 1,000 reads) and without rarefaction. We included both analyses because rarefaction, especially at low read counts, can increase type 1 errors and mask potential differentially abundant taxa between samples (McMurdie and Holmes (Citation2014)). In the analyses comparing urine and fecal microbiota from dogs with and without UC, samples with fewer than 7,000 reads were excluded; this cutoff allowed us to retain all but two urine samples while excluding all negative controls (Figure ). Urine samples from dogs with and without UC were rarefied at 7,000 reads; fecal samples were rarefied at 9,233 reads, which included all fecal samples. Sequencing data for this project is available in SRA BioProject PRJNA763920.
Prior to analyses, we first removed singletons (Amplicon Sequence Variants (ASVs) with only one read in the dataset). ASVs are roughly equivalent to a microbial species or strain. We then applied the R package decontam to identify and filter out putative contaminant ASVs based on their frequency and prevalence (0.5 threshold) as compared to negative controls (R package, v.1.10.0) (Davis et al. (Citation2018)). In total, we identified and removed 13 putative contaminant ASVs from the urine samples and 8 from the fecal samples (S3 Table). We also removed sequences aligned to chloroplasts, eukaryotes, mammalia, and mitochondria. In addition, in the urine samples, we removed taxa within the phylum Cyanobacteria and the class Chloroflexia. All six negative controls, which contained fewer than 7000 reads, were then removed from subsequent analyses.
Comparing human and canine urine microbiota
To compare canine and human urine microbiota, we downloaded publicly available 16S rRNA sequencing data from the Bučvić Popović et al. study on human urine microbiota in individuals with and without bladder cancer (European Nucleotide Archive: PRJEB22327) (Bučević Popović et al. (Citation2018)). Raw reads from the canine and human studies were merged and processed together as described above (See 16S rRNA sequencing and sequence processing). Data were rarefied to 7000 reads to account for differences in read counts between human and dog samples (average read count – humans: 370,586 ± 31,353 reads; dogs: 20,010 ± 7,329). We selected this study for comparison based on its similarities to our canine study: human urine was collected mid-stream free catch and frozen without a buffer prior to DNA extraction. The Bučvić Popović et al. study also utilized the same hypervariable region (V4), primers (515F-806R), and sequencing platform (MiSeq, Illumina, San Diego, CA) for 16S rRNA sequencing. However, different DNA extraction methods were used in both studies.
Statistical analyses
Data were tested for normality using the Shapiro Wilk Normality Test in R version 3.5.2 (R Core Team (Citation2018)). We then compared DNA concentrations and read numbers between groups using Wilcoxon Rank Sum tests and two-sample t-tests, respectively. All alpha and beta diversity metrics were assessed using the R package phyloseq with a p-value cutoff of 0.05 adjusted using the Benjamini & Hochberg False Discovery Rates (McMurdie and Holmes (Citation2013)). Alpha-diversity metrics included Shannon, Simpson, and Observed Features followed by Kruskal–Wallis Rank Sum Tests to compare metrics by group. Beta-diversity metrics included Bray–Curtis, Unweighted UniFrac, and Weighted UniFrac. Permutational Multivariate Analysis of Variance (PERMANOVA) were implemented in QIIME2 v. 2020.11 to compare bacterial community composition by group. An Analysis of Composition of Microbiome (ANCOM) was used to identify differentially abundant taxa by group.
Results
Urine microbiota in dogs with UC
We compared the urine microbiota of 7 dogs with UC to 7 age-, sex-, and breed-matched healthy controls. The total number of reads across all samples ranged from 7,232–36,692 with a mean of 20,010 ± 7,329 reads. Urine samples contained a total of 21 bacterial phyla, 308 genera, and 187 species. Urine DNA concentrations were significantly higher in dogs with UC as compared to healthy dogs (Figure a: Wilcoxon Rank Sum test, p = 0.002), but there was no significant difference in the number of 16S reads between dogs with and without UC (Figure b: two-sample t-test, p = 0.99).
Figure 2. DNA concentrations and number of 16S reads in the urine samples of dogs with and without urothelial carcinoma (UC). (a) DNA concentrations were significantly greater in dogs with UC than in healthy dogs (Wilcoxon Rank Sum test, p = 0.002). (b) The number of 16S reads did not differ significantly between groups (two-sample t-test, p = 0.99). Error bars denote standard error. Statistical significance is represented by stars: * < 0.05, ** < 0.001, *** < 0.0001.
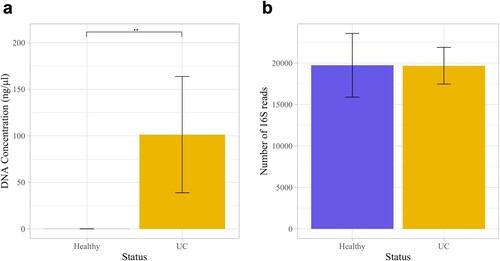
Dogs with UC had significantly lower urine microbial diversity compared to healthy dogs as measured by the Shannon diversity index and Observed Features but not by the Simpson diversity index (Kruskal–Wallis: Shannon, p = 0.048; Observed Features, p = 0.025; Simpson, p = 0.133; Figure a, S4a and S4b Figs). Dogs with UC also had significantly different urine microbial composition than healthy dogs based on an Unweighted UniFrac distance matrix (Figure b; PERMANOVA, p = 0.011); although, no significant differences were observed by Bray Curtis (p = 0.888) or Weighted UniFrac (p = 0.168) distance matrices (S4c and S4d Figs). At the phylum level, Firmicutes (healthy: 61.1%; UC: 79.5%) Proteobacteria (healthy: 18.0%; UC: 15.6%), and Actinobacteria (healthy: 12.5%; UC: 4.26%) were the three most abundant phyla in the urine of healthy dogs and dogs with UC (Figure a). (Note, percentages represent relative abundance of a microbial taxon compared to all taxa.) At the family level, Staphylococcaceae (healthy 42.6%; UC 48.6%) and Streptococcaceae (healthy 5.99%; UC 14.8%) were amongst the most abundant taxa (Figure b; For genus and order level taxa see S5 Fig). Interestingly, Fusobacterium was present in the urine of dogs with UC but not in the urine of healthy dogs (relative abundance of Fusobacterium in healthy dogs: 0%; in dogs with UC: 0.167%). There were no differentially abundant taxa between healthy dogs and dogs with UC at the phylum, genus, or ASV levels.
Figure 3. Microbial diversity and composition in the urine of dogs with and without UC. (a) Healthy dogs had a significantly higher microbial diversity compared to dogs with UC as measured by the Shannon diversity index (Kruskal-Wallis, p = 0.048). (b) Microbial composition between healthy dogs and dogs with UC also differed significantly (Unweighted UniFrac, PERMANOVA, p = 0.011). Error bars denote standard error. Statistical significance is represented by stars: * < 0.05, ** < 0.001, *** < 0.0001.
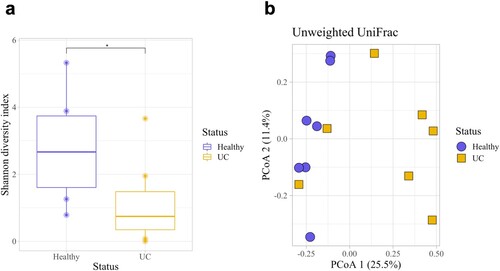
Fecal microbiota in dogs with UC
We compared the fecal microbiota of a subset of dogs from the urine analyses for which we also had fecal samples: four dogs with and six dogs without UC. The total number of reads across all fecal samples ranged from 9,233–28,345 with a mean of 19,196 ± 6,100 reads. Fecal samples contained a total of 8 bacterial phyla, 92 genera, and 45 species. There was no significant difference in fecal DNA concentrations or number of 16S reads in dogs with UC as compared to healthy dogs (DNA concentration: Wilcoxon Rank Sum Test, p = 0.136; 16S reads: Two-sample t-test, p = 0.322; Figure ).
Figure 5. DNA concentrations and number of 16S reads in the fecal samples of dogs with and without UC. (a) DNA concentrations in dogs with UC as compared to healthy dogs (Wilcoxon Rank Sum Test, p = 0.136). (b) The number of 16S reads did not differ significantly between groups (two-sample t-test, p = 0.322). Error bars denote standard error.
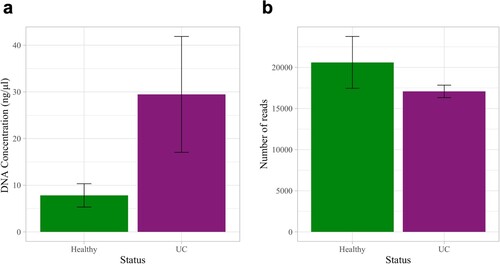
Fecal microbial diversity and composition did not differ significantly in dogs with and without UC (Kruskal–Wallis: Shannon, p = 0.67; Unweighted UniFrac PERMANOVA, p = 0.252; Figure , S6 Fig). The top three most abundant phyla across all fecal samples were Firmicutes (healthy: 72.6%; UC: 32.9%), Bacteroidetes (healthy: 10.6%, UC 31.9%) and Fusobacteria (healthy: 11.3%, UC: 31.1%) (Figure ; S7 Fig). At the family and genera levels, Fusobacterieacea (healthy: 11.4%, UC: 31.7%) and Fusobacterium (healthy: 12.0%, UC: 33.1%) were the most abundant taxa in UC but not healthy samples, respectively; although, these differences were not statistically significant. Only one Bacteroides spp. was significantly increased in relative abundance in dogs with UC compared to healthy dogs (ANCOM, W = 25).
Figure 6. Microbial diversity and composition of fecal samples in dogs with and without UC. (a) Fecal microbial diversity did not differ significantly between dogs with and without UC (Kruskal-Wallis, p = 0.67). (b) Microbial composition also did not differ significantly between healthy dogs and dogs with UC (Unweighted UniFrac, PERMANOVA, p = 0.252). Error bars denote standard error.

Figure 7. Taxa bar plots of fecal samples in dogs with and without UC. (a) Microbial phyla and (b) family relative abundances.
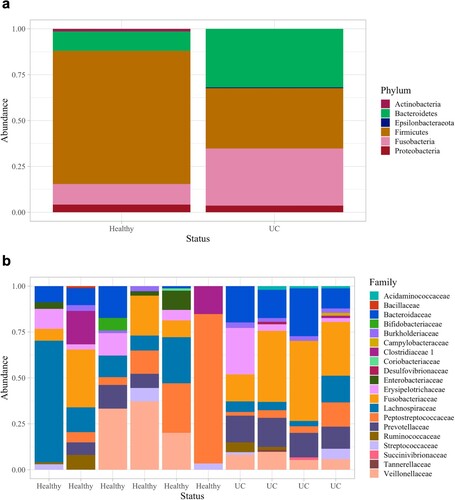
To determine how results from this subset of fecal samples compared to a larger sample set, we then analyzed the fecal microbiota in a validation cohort of 30 dogs with UC and 30 age-, sex-, and breed-matched healthy controls (S4 Table). All of these dogs also met our inclusion/exclusion criteria. Fecal DNA concentrations, 16S reads, and fecal microbial diversity and microbial composition again did not differ significantly between groups (DNA concentration: Wilcoxon Rank Sum test, p = 0.515; 16S reads: two-sample t-test, p = 0.0697; S8 Fig; S5 Table). Firmicutes, Bacteroidetes, and Fusobacteria also remained the most abundant phyla across both groups, and interestingly, Fusobacteriaceae (healthy: 17.4%; UC: 28%) and Fusobacterium (healthy: 18.5%; UC: 29.2%) were still the most abundant family and genus in the fecal samples of dogs with UC (S9 Fig); although, this difference was still not significant. In fact, no taxa were differentially abundant at the phylum, genus, or ASV levels between groups in the larger sample set (S5 Table), suggesting that that Bacteroides spp. identified as differentially abundant in the subset was likely an artifact of small sample size.
Microbiota identified in both fecal and urine samples
As the gut can be a source for microbes in the urinary tract (Magruder et al. (Citation2019); Paalanne et al. (Citation2018)), we then combined urine and fecal data to determine what ASVs were present in both urine and fecal samples. There were a total of 1,204 ASVs across all urine and fecal samples combined. Sixty-six ASVs were identified in both urine and fecal samples from any dog (S6 Table). The most common taxa found in both urine and fecal samples included taxa in the genera Streptococcus and Blautia. Notably, Fusobacterium spp., Porphyromonas spp., Campylobacter spp., Helicobacter spp., and Clostridiodes difficile were also found in both urine and fecal samples. Further, nine ASVs were identified in urine and fecal samples from the same dogs (S7 Table). These ASVs included two Escherichia or Shigella spp., two Streptococcus spp., a Clostridium sensu stricto 1 spp., Actinomyces coleocanis, Streptococcus minor, an Enterococcus spp., and an uncultured Peptoclostridium spp.
Comparing human and canine urine microbiota
To further validate the use of dogs as model organisms for bladder cancer, we compared dog urine microbiota from our study to samples from the Bučvić Popović et al. study, which analyzed urine samples from 12 humans with UC and 11 healthy humans (Bučević Popović et al. (Citation2018)) (Table ). Although overall microbial composition differed significantly between dogs and humans, clear overlap between dog and human samples on a Weighted UniFrac Principal Coordinates Analysis plot (Figure a; S8 Table) demonstrated strong similarity between canine and human urine microbial community profiles. The wider differences observed in Unweighted UniFrac and Bray–Curtis plots indicate the presence of host-species specific microbial taxa (S10 Fig, S8 Table). However, these taxa were rarer compared to more dominant taxa that were similar between dogs and humans (Figure b). Urine microbial diversity was also similar between healthy dogs and humans (Figure c).
Figure 8. Comparing human and dog urine microbiota. (a) Urine microbial composition (Weighted UniFrac) and (b) taxonomic profiles demonstrated clear similarities between canine and human samples despite statistical differences (Wilcoxon Rank pairwise comparisons - all p < 0.003). (c) Urine microbial diversity (Shannon Diversity Index) was similar between healthy dogs and humans.
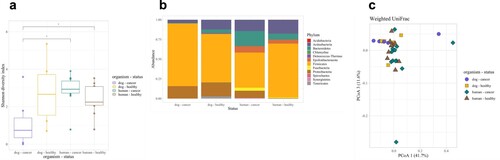
Discussion
The purpose of our study was to characterize the urine and fecal microbiota in a naturally-occurring canine model of UC. We report decreased urine microbial diversity and altered urine microbial composition in dogs with UC compared to healthy controls. We did not detect significant differences in fecal microbiota between dogs with and without UC; although, Fusobacterium was increased in dogs with UC. We also report on the similarities between human and canine urine microbiota. These results provide a foundation for further exploring the role of microbes in UC in a highly relevant animal model.
Urine and fecal microbiota associated with UC
The higher concentrations of DNA found in urine from dogs with UC is likely host DNA from epithelial or tumor cells being sloughed into the urine. Notably, urine microbial read numbers did not differ significantly between dogs with and without UC indicating similar amplicon sequencing depths despite differences in DNA concentrations. (Notably, efforts to remove host DNA from UC urine samples prior to sequencing may be beneficial in future microbiome studies employing shotgun metagenomics to ensure that the run is not overwhelmed with host sequences.)
Besides DNA concentrations, we also observed significant differences in urine microbial diversity (Shannon) and composition (Unweighted UniFrac) between dogs with and without UC. In this study, urine microbial diversity was greater in healthy dogs as compared to dogs with UC, a finding that aligns with several studies on urine microbiota in humans with UC (Liu et al. (Citation2019); Chipollini et al. (Citation2020)). However, there are also studies in humans that report no differences in microbial diversity or decreased diversity in urine from healthy individuals as compared to those with UC (Wu et al. (Citation2018); Bi et al. (Citation2019); Pederzoli et al. (Citation2020); Bučević Popović et al. (Citation2018); Xu et al. (Citation2014); Hussein et al. (Citation2021); Zeng et al. (Citation2020)). Differences in microbial composition (Unweighted UniFrac) have also been reported in previous human studies on UC (Pederzoli et al. (Citation2020); Bučević Popović et al. (Citation2018); Chen et al. (Citation2021); Hussein et al. (Citation2021)). In this study, the four most abundant phyla in urine were Firmicutes, Actinobacteria, Bacteroides, and Proteobacteria. These phyla also dominate the urine microbiota in humans (Wu et al. (Citation2018); Pederzoli et al. (Citation2020); Bučević Popović et al. (Citation2018); Mai et al. (Citation2019); Hussein et al. (Citation2021); Mansour et al. (Citation2020)) and have been reported in previous studies on healthy dog urine (Burton et al. (Citation2017); Melgarejo et al. (Citation2021)). In humans, taxa associated with UC vary widely across studies, but Acinetobacter and Actinomyces have been found at increased abundances in patients with UC across at least three studies (Bi et al. (Citation2019); Xu et al. (Citation2014); Hussein et al. (Citation2021)). In this study, we did not see Acinetobacter or Actinomyces spp. increased in relation to UC, which may be due to small sample sizes and reduced power to detect differentially abundant taxa, or differences between human and canine urine microbiota, or lack of a true link between these taxa and UC.
In relation to fecal microbiota, we did not observe any significant differences in dogs with and without UC. However, intriguingly, Fusobacterium was increased in relative abundance (although not significantly) in urine and fecal samples of dogs with UC. One previous study on bladder cancer also reported increased Fusobacterium in the urine of individuals (human) with UC (Bučević Popović et al. (Citation2018)). Importantly, taxa in the phyla Fusobacteria are considered normal inhabitants of the canine gastrointestinal tract (Pilla and Suchodolski (Citation2020)); although, they are more typically associated with disease in humans. Studies in colorectal cancer have demonstrated direct links between Fusobacteria (Fusobacterium nucleatum) and carcinogenesis. Specifically, Fusobacterium nucleatum Fap2 protein can bind to host factor Gal-GalNAc which is overexpressed on tumor cells (Abed et al. (Citation2016)) - thereby localizing to tumors where Fap2 can impair host anti-tumor immunity (Abed et al. (Citation2016)). Fusobacterium nucleatum can also induce the host Wnt / beta-catenin pathway resulting in upregulated host cellular proliferation (Rubinstein et al. (Citation2019)). Future studies are needed to elucidate the potential role of Fusobacterium in bladder cancer.
Limitations of the current study include small sample size and varying diets and NSAID use amongst the dogs included in this study. While these factors could impact the microbiota, we limited the influence of many other variables by rigorously controlling for antibiotic use, history of chemotherapy or radiation, and co-morbidities including metabolic, gastrointestinal, and urogenital diseases and infections. We also age-, sex-, and breed-matched the dogs in our analyses to limit the impact of these demographic variables on the microbiota. Future studies with larger sample sizes and evaluating the impact of diet and NSAID use on urine and fecal microbiota will be important.
Microbiota shared between urine and fecal samples
Communication and migration of microbes between the gut and bladder can increase a host’s risk of UTIs and bacteriuria (Magruder et al. (Citation2019)). Microbes may migrate and ascend into the urogenital tract externally from the rectum / anus, or internally via the blood stream (Meštrović et al. (Citation2020); Łaniewski et al. (Citation2020)). In this study, 66 ASVs were shared between urine and fecal samples. Interestingly, ∼ 59% of those ASVs (39 / 66) are likely spore-formers (Bacilli, Clostridia, Negativicutes) suggesting that spore formation may more readily enable exchange of microbes between body niches (Galperin (Citation2013); Tetz and Tetz (Citation2017)). Among the microbes (ASVs) found in both urine and fecal samples, there were multiple potentially pathogenic taxa: Campylobacter spp., Helicobacter canis, Clostridiodes difficile, Clostridium baratii, Escherichia / Shigella spp., and Enterococcus spp. There were also a few taxa that have been associated with tumors or directly linked with tumor development or progression in gastrointestinal, oral, and genital cancers: Fusobacterium spp. and Porphyromonas spp. (Kostic et al. (Citation2013); Hale et al. (Citation2018); Mitsuhashi et al. (Citation2015); Ha et al. (Citation2015); Atanasova and Yilmaz (Citation2014); Walther-António et al. (Citation2016)). The shared presence of two Fusobacterium ASVs between urine and fecal samples is particularly of interest given the role of Fusobacterium in colorectal cancer.
Dogs as a model for studies on human urine microbiota
Dogs are already considered effective models for studies on bladder cancer (Knapp et al. (Citation2014); Knapp et al. (Citation2020)) and on the gut microbiota (Coelho et al. (Citation2018); Ericsson (Citation2019)). Dogs also offer many advantages over rodent models – from immune competency, to naturally-occurring cancer, to gut microbial responses that mirror human responses. Additionally, there is clear evidence that dogs and humans share microbes including microbes that can colonize the gut, and microbies that can cause urinary tract infections in both host species (Johnson and Clabots (Citation2006); Johnson et al. (Citation2008); Johnson et al. (Citation2008); Murray et al. (Citation2004); Song et al. (Citation2013); Misic et al. (Citation2015); Johnson et al. (Citation2000)). However, there is no study, to our knowledge, that has directly compared urine microbial communities between dogs and humans. Our novel results demonstrate that human and dog urine is dominated by similar taxa; although, host-specific taxa are also observed. This key finding bolsters the value of dogs as models for studying urine microbiota, and demonstrates that dogs and humans exhibit similar microbial niches within the urinary tract.
Conclusions
This pilot study is a novel investigation of urine and fecal microbiota in a canine model of UC. The dominant microbial taxa identified in canine urine and fecal samples were similar to those reported in humans, further supporting the value of dogs as a model for studies on bladder cancer and on the microbiota. Also, as in humans, altered microbial diversity and composition were observed in dogs with UC as compared to healthy controls. This suggests that the microbiota could play a role in UC development, progression, prognosis, or response to treatment, as has been observed in other cancers. Moreover, Fusobacterium was increased – albeit not significantly – in both urine and fecal samples of dogs with UC. Fusobacterium ASVs were also shared between urine and fecal samples. Taken together, these results provide support for the use of dogs as a model in UC microbiome studies. Additionally, these findings suggest that future work evaluating the role of Fusobacterium in UC, and the gut as a potential source of this Fusobacterium, may be warranted.
Author contributions
Conceptualization: Vanessa L. Hale, Deborah W. Knapp, and William C. Kisseberth.
Clinical sample collection, clinical care / monitoring, clinical data extraction: Deborah W. Knapp, Deepika Dhawan, and William C. Kisseberth.
DNA extraction: Chris Madden, Ryan Mrofchak, and Morgan V. Evans.
Data processing, analysis: Ryan Mrofchak, Morgan, V. Evans, Chris Madden, and Deborah W. Knapp.
Data interpretation and conclusions: Ryan Mrofchak, Vanessa L. Hale, Chris Madden, and Deborah W. Knapp.
Manuscript writing: Ryan Mrofchak, Vanessa L. Hale, and Chris Madden.
Manuscript editing: Chris Madden, Deepika Dhawan, Deborah W. Knapp, and William C. Kisseberth.
Preprint availability
The article has been published in BioRvix with the title ‘Urine and Fecal Microbiota in a Canine Bladder Cancer Model’ (Mrofchak et al. (Citation2021b)).
Supplemental Material
Download Zip (23.7 MB)Acknowledgements
We are grateful to all individuals involved in sample collection at Purdue University College of Veterinary Medicine (West Lafayette, IN, USA) and to the dogs and dog owners who participated in this study. We also acknowledge the Ohio Supercomputer Center (Columbus, Ohio, USA, established 1987) for computing resources used in this study.
Data accessibility
The data that support the findings of this study are openly available in SRA BioProject PRJNA763920 [https://www.ncbi.nlm.nih.gov/sra/?term=PRJNA763920] and figshare [https://doi.org/10.6084/m9.figshare.19775449.v2].
Additional information
Funding
References
- Abed J, Emgård JE, Zamir G, Faroja M, Almogy G, Grenov A, Sol A, Naor R, Pikarsky E, Atlan KA, Mellul A. 2016 Aug. Fap2 mediates fusobacterium nucleatum colorectal adenocarcinoma enrichment by binding to tumor-expressed Gal-GalNAc. Cell Host Microbe. 20(2):215–225. Available from: https://linkinghub.elsevier.com/retrieve/pii/S1931312816303055.
- Aben KK, Witjes JA, Schoenberg MP, Hulsbergen-van de Kaa C, Verbeek AL, Kiemeney LA. 2002 Mar 10. Familial aggregation of urothelial cell carcinoma. Int J Cancer. 98(2):274–278. doi:10.1002/ijc.10191.
- Adebayo AS, Survayanshi M, Bhute S, Agunloye AM, Isokpehi RD, Anumudu CI, Shouche YS. 2017. The microbiome in urogenital schistosomiasis and induced bladder pathologies. PLoS Negl Trop Dis. 11(8):e0005826.
- Alguacil J, Kogevinas M, Silverman DT, Malats N, Real FX, García-Closas M, Tardón A, Rivas M, Tora M, García-Closas R, Serra C. 2011 Jun. Urinary pH, cigarette smoking and bladder cancer risk. Carcinogenesis. 32(6):843–847. doi:10.1093/carcin/bgr048.
- American Cancer Society. Key Statistics for Bladder Cancer [Internet]. 2021. Available from: https://www.cancer.org/cancer/bladder-cancer/about/key-statistics.html.
- Antoni S, Ferlay J, Soerjomataram I, Znaor A, Jemal A, Bray F. 2017 Jan. Bladder cancer incidence and mortality: A global overview and recent trends. Eur Urol. 71(1):96–108. Available from: https://linkinghub.elsevier.com/retrieve/pii/S0302283816302809.
- Aragon IM, Herrera-Imbroda B, Queipo-Ortuño MI, Castillo E, Del Moral JSG, Gomez-Millan J, Yucel G, Lara MF. 2018. The urinary tract microbiome in health and disease. Eur Urol Focus. 4(1):128–138.
- Atanasova KR, Yilmaz Ö. 2014 Apr. Looking in the porphyromonas gingivalis cabinet of curiosities: the microbium, the host and cancer association. Mol Oral Microbiol. 29(2):55–66. doi:10.1111/omi.12047.
- Aveyard JS, Skilleter A, Habuchi T, Knowles MA. 1999 May 23. Somatic mutation of PTEN in bladder carcinoma. Br J Cancer. 80(5–6):904–908. Available from: http://www.nature.com/articles/6690439.
- Bajic P, Van Kuiken ME, Burge BK, Kirshenbaum EJ, Joyce CJ, Wolfe AJ, Branch JD, Bresler L, Farooq AV. 2020. Male bladder microbiome relates to lower urinary tract symptoms. Eur Urol Focus. 6(2):376–382.
- Bi H, Tian Y, Song C, Li J, Liu T, Chen Z, Chen C, Huang Y, Zhang Y. 2019 Oct 1. Urinary microbiota – a potential biomarker and therapeutic target for bladder cancer. J Med Microbiol. 68(10):1471–1478. doi:10.1099/jmm.0.001058.
- Bolyen E, Rideout JR, Dillon MR, Bokulich NA, Abnet CC, Al-Ghalith GA, Alexander H, Alm EJ, Arumugam M, Asnicar F, Bai Y. 2019. Reproducible, interactive, scalable and extensible microbiome data science using QIIME 2. Nat Biotechnol. 37(8):852–857.
- Bučević Popović V, Šitum M, Chow CET, Chan LS, Roje B, Terzić J. 2018. The urinary microbiome associated with bladder cancer. Sci Rep. 8(12157):1–8.
- Burger M, Catto JW, Dalbagni G, Grossman HB, Herr H, Karakiewicz P, Kassouf W, Kiemeney LA, La Vecchia C, Shariat S, Lotan Y. 2013 Feb. Epidemiology and risk factors of urothelial bladder cancer. Eur Urol. 63(2):234–241. Available from: https://linkinghub.elsevier.com/retrieve/pii/S0302283817306620.
- Burton EN, Cohn LA, Reinero CN, Rindt H, Moore SG, Ericsson AC. 2017. Characterization of the urinary microbiome in healthy dogs. PLoS One. 12(5):e0177783. doi:10.1371/journal.pone.0177783.
- Callahan BJ, McMurdie PJ, Rosen MJ, Han AW, Johnson AJA, Holmes SP. 2016. DADA2: High-resolution sample inference from illumina amplicon data. Nat Methods. 13:581–583. doi:10.1038/nmeth.3869.
- Caporaso JG, Lauber CL, Walters WA, Berg-Lyons D, Huntley J, Fierer N, Owens SM, Betley J, Fraser L, Bauer M, Gormley N. 2012. Ultra-high-throughput microbial community analysis on the illumina HiSeq and MiSeq platforms. ISME J. 6:1621–1624. doi:10.1038/ismej.2012.8.
- Caporaso JG, Lauber CL, Walters WA, Berg-Lyons D, Lozupone CA, Turnbaugh PJ, Fierer N, Knight R. 2011. Global patterns of 16S rRNA diversity at a depth of millions of sequences per sample. Proc Natl Acad Sci USA. 18(Supplement 1):4516–4522. doi:10.1037/pnas.1000080107.
- Chaitman J, Ziese AL, Pilla R, Minamoto Y, Blake AB, Guard BC, Isaiah A, Lidbury JA, Steiner JM, Unterer S, Suchodolski JS. 2020 Apr 16. Fecal microbial and metabolic profiles in dogs with acute diarrhea receiving either fecal microbiota transplantation or oral metronidazole. Front Vet Sci. 7(192):1–12. doi:10.3389/fvets.2020.00192.
- Chen C, Huang Z, Huang P, Li K, Zeng J, Wen Y, Li B, Zhao J, Wu P. 2021. Profiling the urinary microbiota in men with positive versus negative PD-L1 expression for non-muscle invasive bladder cancer. Res Sq. 8(167):1–10.
- Chen YB, Hochstedler B, Pham TT, Alvarez MA, Mueller ER, Wolfe AJ. 2020 Aug. The urethral microbiota: a missing link in the female urinary microbiota. J Urol. 204(2):303–309. doi:10.1097/JU.0000000000000910.
- Chipollini J, Wright JR, Nwanosike H, Kepler CY, Batai K, Lee BR, Spiess PE, Stewart DB, Lamendella R. 2020 Jul. Characterization of urinary microbiome in patients with bladder cancer: results from a single-institution, feasibility study. Urol Oncol Semin Orig Investig. 38(7):615–621. doi:10.1016/j.urolonc.2020.04.014.
- Chu H, Wang M, Zhang Z. 2013 May 30. Bladder cancer epidemiology and genetic susceptibility. J Biomed Res. 27(3):170–178. doi:10.7555/JBR.27.20130026.
- Coelho LP, Kultima JR, Costea PI, Fournier C, Pan Y, Czarnecki-Maulden G, Hayward MR, Forslund SK, Schmidt TSB, Descombes P, Jackson JR. 2018 Dec 19. Similarity of the dog and human gut microbiomes in gene content and response to diet. Microbiome. 6(1):72. doi:10.1186/s40168-018-0450-3.
- Connelly S, Fanelli B, Hasan NA, Colwell RR, Kaleko M. 2019. Low dose oral beta-lactamase protects the gut microbiome from oral beta-lactam-mediated damage in dogs. AIMS Public Heal. 6(4):477–487. doi:10.3934/publichealth.2019.4.477.
- Cumberbatch MG, Rota M, Catto JWF, La Vecchia C. 2016 Sep. The role of tobacco smoke in bladder and kidney carcinogenesis: A comparison of exposures and meta-analysis of incidence and mortality risks. Eur Urol. 70(3):458–466. doi:10.1016/j.eururo.2015.06.042.
- Davis NM, Proctor DM, Holmes SP, Relman DA, Callahan BJ. 2018 Dec 17. Simple statistical identification and removal of contaminant sequences in marker-gene and metagenomics data. Microbiome. 6(1):226. doi:10.1186/s40168-018-0605-2.
- De Brot S, Robinson BD, Scase T, Grau-Roma L, Wilkinson E, Boorjian SA, Gardner D, Mongan NP. 2018 May 30. The dog as an animal model for bladder and urethral urothelial carcinoma: comparative epidemiology and histology. Oncol Lett. 16:1641–1649. doi:10.3892/ol.2018.8837.
- Ding JIE, Xu D, Pan C, Ye M, Kang J, Bai Q, Qi J. 2014 Sep. Current animal models of bladder cancer: awareness of translatability (review). Exp Ther Med. 8(3):691–699. doi:10.3892/etm.2014.1837.
- Ericsson AC. 2019 Jun 17. The use of non-rodent model species in microbiota studies. Lab Anim. 53(3):259–270. doi:10.1177/0023677219834593.
- Flores-Mireles AL, Walker JN, Caparon M, Hultgren SJ. 2015 May 8. Urinary tract infections: epidemiology, mechanisms of infection and treatment options. Nat Rev Microbiol. 13(5):269–284. doi:10.1038/nrmicro3432.
- Fok CS, Gao X, Lin H, Thomas-White KJ, Mueller ER, Wolfe AJ, Dong Q, Brubaker L. 2018 Dec 16. Urinary symptoms are associated with certain urinary microbes in urogynecologic surgical patients. Int Urogynecol J. 29(12):1765–1771. doi:10.1007/s00192-018-3732-1.
- Galperin MY.. 2013. Genome Diversity of Spore-Forming Firmicutes. Microbiol Spectrum. 1(2):TBS-0015-2012. doi:10.1128/microbiolspectrum.TBS-0015-2012.
- Glickman LT, Raghavan M, Knapp DW, Bonney PL, Dawson MH. 2004. Herbicide exposure and the risk of transitional cell carcinoma of the urinary bladder in Scottish terriers. J AM Vet Med Assoc. 224(8):1290–1297. doi:10.2460/javma.2004.224.1290.
- Gottschick C, Deng ZL, Vital M, Masur C, Abels C, Pieper DH, Wagner-Döbler I. 2017. The urinary microbiota of men and women and its changes in women during bacterial vaginosis and antibiotic treatment. Microbiome. 5(1):99. doi:10.1186/s40168-017-0305-3.
- Ha NH, Woo BH, Kim DJ, Ha ES, Choi JI, Kim SJ, Park BS, Lee JH, Park HR. 2015 Dec 16. Prolonged and repetitive exposure to porphyromonas gingivalis increases aggressiveness of oral cancer cells by promoting acquisition of cancer stem cell properties. Tumor Biol. 36(12):9947–9960. doi:10.1007/s13277-015-3764-9.
- Hale VL, Jeraldo P, Chen J, Mundy M, Yao J, Priya S, Keeney G, Lyke K, Ridlon J, White BA, French AJ. 2018 Dec 31. Distinct microbes, metabolites, and ecologies define the microbiome in deficient and proficient mismatch repair colorectal cancers. Genome Med. 10(1):78. doi:10.1186/s13073-018-0586-6.
- Hourigan SK, Zhu W, SW Wong W, Clemency NC, Provenzano M, Vilboux T, Niederhuber JE, Deeken J, Chung S, McDaniel-Wiley K, Trump D. 2020. Studying the urine microbiome in superficial bladder cancer: samples obtained by midstream voiding versus cystoscopy. BMC Urol. 20(5):1–8. doi:10.1186/s12894-020-0576-z.
- Hussein AA, Elsayed AS, Durrani M, Jing Z, Iqbal U, Gomez EC, Singh PK, Liu S, Smith G, Tang L, Guru KA. 2021. Investigating the association between the urinary microbiome and bladder cancer: An exploratory study. Urol Oncol Semin Orig Investig. 39(6):370.e9–370.e19. doi:10.1016/j.urolonc.2020.12.011.
- Johnson JR, Clabots C. 2006 Nov 15. Sharing of virulent escherichia coli clones among household members of a woman with acute cystitis. Clin Infect Dis. 43(10):e101–e108. doi:10.1086/508541.
- Johnson JR, Clabots C, Kuskowski MA. 2008a Dec. Multiple-Host sharing, long-term persistence, and virulence of escherichia coli clones from human and animal household members. J Clin Microbiol. 46(12):4078–4082. doi:10.1128/JCM.00980-08.
- Johnson JR, O’Bryan TT, Low DA, Ling G, Delavari P, Fasching C, Russo TA, Carlino U, Stell AL. 2000 Jun. Evidence of commonality between canine and human extraintestinal pathogenic escherichia coli strains that express papG allele III. O’Brien AD, editor. Infect Immun. 68(6):3327–3336. doi:10.1128/IAI.68.6.3327-3336.2000.
- Johnson JR, Owens K, Gajewski A, Clabots C. 2008b Jan 15. Escherichia coli colonization patterns among human household members and pets, with attention to acute urinary tract infection. J Infect Dis. 197(2):218–224. doi:10.1086/524844.
- Knapp DW, Dhawan D, Ramos-Vara JA, Ratliff TL, Cresswell GM, Utturkar S, Sommer BC, Fulkerson CM, Hahn NM. 2020 Jan 21. Naturally-Occurring invasive urothelial carcinoma in dogs, a unique model to drive advances in managing muscle invasive bladder cancer in humans. Front Oncol. 9. doi:10.3389/fonc.2019.01493/full.
- Knapp DW, Ramos-Vara JA, Moore GE, Dhawan D, Bonney PL, Young KE. 2014 Apr 1. Urinary bladder cancer in dogs, a naturally occurring model for cancer biology and drug development. ILAR J. 55(1):100–118. doi:10.1093/ilar/ilu018.
- Kostic AD, Chun E, Robertson L, Glickman JN, Gallini CA, Michaud M, Clancy TE, Chung DC, Lochhead P, Hold GL, El-Omar EM. 2013 Aug. Fusobacterium nucleatum potentiates intestinal tumorigenesis and modulates the tumor-immune microenvironment. Cell Host Microbe. 14(2):207–215. doi:10.1016/j.chom.2013.07.007.
- Koutros S, Silverman DT, Alavanja MC, Andreotti G, Lerro CC, Heltshe S, Lynch CF, Sandler DP, Blair A, Beane Freeman LE. 2016 Jun. Occupational exposure to pesticides and bladder cancer risk. Int J Epidemiol. 45(3):792–805. doi:10.1093/ije/dyv195.
- Kramer H, Kuffel G, Thomas-White K, Wolfe AJ, Vellanki K, Leehey DJ, Bansal VK, Brubaker L, Flanigan R, Koval J, Wadhwa A. 2018 Jun 12. Diversity of the midstream urine microbiome in adults with chronic kidney disease. Int Urol Nephrol. 50(6):1123–1130. doi:10.1007/s11255-018-1860-7.
- Łaniewski P, Ilhan ZE, Herbst-Kralovetz MM. 2020 Apr 18. The microbiome and gynaecological cancer development, prevention and therapy. Nat Rev Urol. 17(4):232–250. doi:10.1038/s41585-020-0286-z.
- Liu F, Liu A, Lu X, Zhang Z, Xue Y, Xu J, Zeng S, Xiong Q, Tan H, He X, Xu W. 2019. Dysbiosis signatures of the microbial profile in tissue from bladder cancer. Cancer Med. 8(16):6904–6914. doi:10.1102/cam4.2419.
- Magruder M, Sholi AN, Gong C, Zhang L, Edusei E, Huang J, Albakry S, Satlin MJ, Westblade LF, Crawford C, Dadhania DM. 2019 Dec 4. Gut uropathogen abundance is a risk factor for development of bacteriuria and urinary tract infection. Nat Commun. 10(1):5521. doi:10.1038/s41467-019-13467-w.
- Mai G, Chen L, Li R, Liu Q, Zhang H, Ma Y. 2019 Jun 19. Common core bacterial biomarkers of bladder cancer based on multiple datasets. Biomed Res Int. 2019:1–8. doi:10.1155/2019/4824909.
- Manchester AC, Webb CB, Blake AB, Sarwar F, Lidbury JA, Steiner JM, Suchodolski JS. 2019 Nov. Long-term impact of tylosin on fecal microbiota and fecal bile acids of healthy dogs. J Vet Intern Med. 33(6):2605–2617. doi:10.1111/jvim.15635.
- Mansour B, Monyók Á, Makra N, Gajdács M, Vadnay I, Ligeti B, Juhász J, Szabó D, Ostorházi E. 2020 Dec 6. Bladder cancer-related microbiota: examining differences in urine and tissue samples. Sci Rep. 10:11042. doi:10.1038/s41598-020-67443-2.
- Mao Q, Jiang F, Yin R, Wang J, Xia W, Dong G, Ma W, Yang Y, Xu L, Hu J. 2018 Feb. Interplay between the lung microbiome and lung cancer. Cancer Lett. 415:40–48. doi:10.1016/j.canlet.2017.11.036.
- Martin C, Leiser CL, O’Neil B, Gupta S, Lowrance WT, Kohlmann W, Greenberg S, Pathak P, Smith KR, Hanson HA. 2018 May 1. Familial cancer clustering in urothelial cancer: A population-based case–control study. JNCI J Natl Cancer Inst. 110(5):527–533. Available from: https://academic.oup.com/jnci/article/110/5/527/4698132.
- McMurdie PJ, Holmes S. 2013. Phyloseq: An R package for reproducible interactive analysis and graphics of microbiome census data. PLoS One. 8(4):e61217. doi:10.1371/journal.pone.0061217.
- McMurdie PJ, Holmes S. 2014. Waste not, want not: Why rarefying microbiome data is inadmissible. PLoS Comput Biol. 10(4):e1003531. doi:10.1371/journal.pcbi.1003531.
- Melgarejo T, Oakley BB, Krumbeck JA, Tang S, Krantz A, Linde A. 2021 Mar 19. Assessment of bacterial and fungal populations in urine from clinically healthy dogs using next-generation sequencing. J Vet Intern Med. jvim.16104. doi:10.1111/jvim.16104.
- Meštrović T, Matijašić M, Perić M, Čipčić Paljetak H, Barešić A, Verbanac D. 2020 Dec 22. The role of gut, vaginal, and urinary microbiome in urinary tract infections: from bench to bedside. Diagnostics. 11(1):7. doi:10.3390/diagnostics11010007.
- Misic AM, Davis MF, Tyldsley AS, Hodkinson BP, Tolomeo P, Hu B, Nachamkin I, Lautenbach E, Morris DO, Grice EA. 2015 Dec 23. The shared microbiota of humans and companion animals as evaluated from staphylococcus carriage sites. Microbiome. 3(1):2. doi:10.1186/s40168-014-0052-7.
- Mitsuhashi K, Nosho K, Sukawa Y, Matsunaga Y, Ito M, Kurihara H, Kanno S, Igarashi H, Naito T, Adachi Y, Tachibana M. 2015 Mar 30. Association of fusobacterium species in pancreatic cancer tissues with molecular features and prognosis. Oncotarget. 6(9):7209–7220. doi:10.18632/oncotarget.3109.
- Montassier E, Gastinne T, Vangay P, Al-Ghalith GA, Bruley des Varannes S, Massart S, Moreau P, Potel G, de La Cochetière MF, Batard E, Knights D. 2015 Sep. Chemotherapy-driven dysbiosis in the intestinal microbiome. Aliment Pharmacol Ther. 42(5):515–528. doi:10.1111/apt.13302.
- Mrofchak R, Madden C, Evans MV, Hale VL. 2021a Jul 9. Evaluating extraction methods to study canine urine microbiota. PLoS One. 16(7):e0253989. Available from: doi:10.1371/journal.pone.0253989.
- Mrofchak R, Madden C, Evans MV, Kisseberth WC, Dhawan D, Knapp DW, Hale VL. 2021b. Urine and fecal microbiota in a canine model of bladder cancer. bioRvix. doi:10.1101/2021.12.20.472715.
- Mucci LA, Hjelmborg JB, Harris JR, Czene K, Havelick DJ, Scheike T, Graff RE, Holst K, Möller S, Unger RH, McIntosh C. 2016 Jan 5. Familial risk and heritability of cancer among twins in Nordic countries. JAMA. 315(1):68. doi:10.1001/jama.2015.17703.
- Mueller CM, Caporaso N, Greene MH. 2008 Sep. Familial and genetic risk of transitional cell carcinoma of the urinary tract. Urol Oncol Semin Orig Investig. 26(5):451–464. doi:10.1016/j.urolonc.2008.02.016.
- Murray AC, Kuskowski MA, Johnson JR. 2004 May 18. Virulence factors predict escherichia coli colonization patterns among human and animal household members. Ann Intern Med. 140(10):848. doi:10.7326/0003-4819-140-10-200405180-00032.
- Murta-Nascimento C, Silverman DT, Kogevinas M, García-Closas M, Rothman N, Tardón A, García-Closas R, Serra C, Carrato A, Villanueva C, Dosemeci M. 2007 Aug. Risk of bladder cancer associated with family history of cancer: do low-penetrance polymorphisms account for the increase in risk? Cancer Epidemiol Biomarkers Prev. 16(8):1595–1600. doi:10.1158/1055-9965.EPI-06-0743.
- Nelson DE, Van Der Pol B, Dong Q, Revanna KV, Fan B, Easwaran S, Sodergren E, Weinstock GM, Diao L, Fortenberry JD. 2010. Characteristic male urine microbiomes associate with asymptomatic sexually transmitted infection. PLoS One. 5(11):e14116. doi:10.1371/journal.pone.0014116.
- O’Keefe SJD. 2016 Dec 16. Diet, microorganisms and their metabolites, and colon cancer. Nat Rev Gastroenterol Hepatol. 13(12):691–706. Available from: http://www.nature.com/articles/nrgastro.2016.165.
- Oresta B, Braga D, Lazzeri M, Frego N, Saita A, Faccani C, Fasulo V, Colombo P, Guazzoni G, Hurle R, Rescigno M. 2021 Jan. The microbiome of catheter collected urine in males with bladder cancer according to disease stage. J Urol. 205(1):86–93. doi:10.1097/JU.0000000000001336.
- Paalanne N, Husso A, Salo J, Pieviläinen O, Tejesvi MV, Koivusaari P, Pirttilä AM, Pokka T, Mattila S, Jyrkäs J, Turpeinen A. 2018 Oct 13. Intestinal microbiome as a risk factor for urinary tract infections in children. Eur J Clin Microbiol Infect Dis. 37(10):1881–1891. doi:10.1007/s10096-018-3322-7.
- Pearce MM, Hilt EE, Rosenfeld AB, Zilliox MJ, Thomas-White K, Fok C, Kliethermes S, Schreckenberger PC, Brubaker L, Gai X, Wolfe AJ. 2014. The female urinary microbiome: A comparison of women with and without urgency urinary incontinence. MBio. 5(4):e01283–14. doi:10.1128/mBio.01283-14.
- Pederzoli F, Ferrarese R, Amato V, Locatelli I, Alchera E, Lucianò R, Nebuloni M, Briganti A, Gallina A, Colombo R, Necchi A. 2020. Sex-specific alterations in the urinary and tissue microbiome in therapy-naïve urothelial bladder cancer patients. Eur Urol Oncol. 3(6):784–788. doi:10.1016/j.euo.2020.04.002.
- Pesch B, Taeger D, Johnen G, Gawrych K, Bonberg N, Schwentner C, Wellhäußer H, Kluckert M, Leng G, Nasterlack M, Lotan Y. 2014 Oct 16. Screening for bladder cancer with urinary tumor markers in chemical workers with exposure to aromatic amines. Int Arch Occup Environ Health. 87(7):715–724. Available from: http://link.springer.com/10.1007s00420-013-0916-3.
- Pilla R, Suchodolski JS. 2020. The role of the canine gut microbiome and metabolome in health and gastrointestinal disease. Front Vet Sci. 6(498):1–12. doi:10.3389/fvets.2019.00498.
- Pohl HG, Groah SL, Pérez-Losada M, Ljungberg I, Sprague BM, Chandal N, Caldovic L, Hsieh M. 2020. The urine microbiome of healthy men and women differs by urine collection method. Int Neurol J. 24(1):41–51. doi:10.5213/inj.1938244.122.
- Quast C, Pruesse E, Yilmaz P, Gerken J, Schweer T, Yarza P, Peplies J, Glöckner FO. 2013. The SILVA ribosomal RNA gene database project: improved data processing and web-based tools. Nucleic Acids Res. 41(Database Issue):D590–D596. doi:10.1093/nar/gks1219.
- Ramírez-Labrada AG, Isla D, Artal A, Arias M, Rezusta A, Pardo J, Gálvez EM. 2020 Feb. The influence of lung microbiota on lung carcinogenesis, immunity, and immunotherapy. Trends Cancer. 6(2):86–97. Available from: https://linkinghub.elsevier.com/retrieve/pii/S2405803319302651.
- Randi G, Pelucchi C, Negri E, Talamini R, Galeone C, Franceschi S, La Vecchia C. 2007 Dec 15. Family history of urogenital cancers in patients with bladder, renal cell and prostate cancers. Int J Cancer. 121(12):2748–2752. doi:10.1002/ijc.23037.
- R Core Team. 2018. R: a langauge and environment for statistical computing. Vienna, Austria: R foundation for Statistical Computing.
- Reid G, Bruce AW, Fraser N, Heinemann C, Owen J, Henning B. 2001 Feb. Oral probiotics can resolve urogenital infections. FEMS Immunol Med Microbiol. 30(1):49–52. doi:10.1111/j.1574-695X.2001.tb01549.x.
- Rubinstein MR, Baik JE, Lagana SM, Han RP, Raab WJ, Sahoo D, Dalerba P, Wang TC, Han YW. 2019 Apr 4. Fusobacterium nucleatum promotes colorectal cancer by inducing Wnt/β-catenin modulator annexin A1. EMBO Rep. 20(4). doi:10.15252/embr.201847638.
- Sears CL, Garrett WS. 2014 Mar. Microbes, microbiota, and colon cancer. Cell Host Microbe. 15(3):317–328. Available from: https://linkinghub.elsevier.com/retrieve/pii/S1931312814000651.
- Shoskes DA, Altemus J, Polackwich AS, Tucky B, Wang H, Eng C. 2016. The urinary microbiome differs significantly between patients with chronic prostatitis/chronic pelvic pain syndrome and controls as well as between patients with different clinical phenotypes. Urology. 92:26–32. doi:10.1016/j.urology.2016.02.043.
- Siddiqui H, Lagesen K, Nederbragt AJ, Jeansson SL, Jakobsen KS. 2012. Alterations of microbiota in urine from women with interstitial cystitis. BMC Microbiol. 13(12):205. doi:10.1186/1471-2180-12-205.
- Song SJ, Lauber C, Costello EK, Lozupone CA, Humphrey G, Berg-Lyons D, Caporaso JG, Knights D, Clemente JC, Nakielny S, Gordon JI. 2013 Apr 16. Cohabiting family members share microbiota with one another and with their dogs. Elife. 2:e00458. https://elifesciences.org/articles/00458.
- Stewardson AJ, Gaïa N, Francois P, Malhotra-Kumar S, Delemont C, de Tejada BM, Schrenzel J, Harbarth S, Lazarevic V, S WP, Groups WS. 2015 Apr. Collateral damage from oral ciprofloxacin versus nitrofurantoin in outpatients with urinary tract infections: a culture-free analysis of gut microbiota. Clin Microbiol Infect. 21(4):344.e1–344.e11. Available from: https://linkinghub.elsevier.com/retrieve/pii/S1198743X14001025.
- Stringer AM, Al-Dasooqi N, Bowen JM, Tan TH, Radzuan M, Logan RM, Mayo B, Keefe DM, Gibson RJ. 2013 Jul 10. Biomarkers of chemotherapy-induced diarrhoea: a clinical study of intestinal microbiome alterations, inflammation and circulating matrix metalloproteinases. Support Care Cancer. 21(7):1843–1852. doi:10.1007/s00520-013-1741-7.
- Suchodolski JS, Dowd SE, Westermarck E, Steiner JM, Wolcott RD, Spillmann T, Harmoinen JA. 2009. The effect of the macrolide antibiotic tylosin on microbial diversity in the canine small intestine as demonstrated by massive parallel 16S rRNA gene sequencing. BMC Microbiol. 9(1):210. doi:10.1186/1471-2180-9-210.
- Sung H, Ferlay J, Siegel RL, Laversanne M, Soerjomataram I, Jemal A, Bray F. 2021 May 4. Global cancer statistics 2020: GLOBOCAN estimates of incidence and mortality worldwide for 36 cancers in 185 countries. CA Cancer J Clin. 71(3):209–249. doi:10.3322/caac.21660.
- Tetz G, Tetz V. 2017 Dec 30. Introducing the sporobiota and sporobiome. Gut Pathog. 9(1):38. doi:10.1186/s13099-017-0187-8.
- Trompette A, Gollwitzer ES, Yadava K, Sichelstiel AK, Sprenger N, Ngom-Bru C, Blanchard C, Junt T, Nicod LP, Harris NL, Marsland BJ. 2014 Feb 5. Gut microbiota metabolism of dietary fiber influences allergic airway disease and hematopoiesis. Nat Med. 20(2):159–166. Available from: http://www.nature.com/articles/nm.3444.
- Walther-António MR, Chen J, Multinu F, Hokenstad A, Distad TJ, Cheek EH, Keeney GL, Creedon DJ, Nelson H, Mariani A, Chia N. 2016 Dec 25. Potential contribution of the uterine microbiome in the development of endometrial cancer. Genome Med. 8(1):122. doi:10.1186/s13073-016-0368-y.
- Wang Y, Chang Q, Li Y. 2018 Dec 21. Racial differences in urinary bladder cancer in the United States. Sci Rep. 8(1):12521. Available from: http://www.nature.com/articles/s41598-018-29987-2.
- Wolfe AJ, Toh E, Shibata N, Rong R, Kenton K, FitzGerald M, Mueller ER, Schreckenberger P, Dong Q, Nelson DE, Brubaker L. 2012. Evidence of uncultivated bacteria in the adult female bladder. J Clin Microbiol. 50(4):1376–1383. doi: 10.1128/JCM.05852-11.
- Worby CJ, Olson BS, Dodson KW, Earl AM, Hultgren SJ. 2022 Mar 1. Establishing the role of the gut microbiota in susceptibility to recurrent urinary tract infections. J Clin Invest. 132(5):e158497. doi:10.1172/JCI158497.
- World Bladder Cancer Patient Coalition. GLOBOCAN. 2020. Bladder cancer 10th most common diagnosed worldwide [Internet]. Lyon, France; 2020. Available from: https://worldbladdercancer.org/news_events/globocan-2020-bladder-cancer-10th-most-commonly-diagnosed-worldwide/.
- Wu P, Zhang G, Zhao J, Chen J, Chen Y, Huang W, Zhong J, Zeng J. 2018 May 31. Profiling the urinary microbiota in male patients with bladder cancer in China. Front Cell Infect Microbiol. 8(167). doi:10.3389/fcimb.2018.00167/full.
- Xu W, Yang L, Lee P, Huang WC, Nossa C, Ma Y, Deng FM, Zhou M, Melamed J, Pei Z. 2014. Mini-review: perspective of the microbiome in the pathogenesis of urothelial carcinoma. Am J Clin Exp Urol. 2(1):57–61.PMCID: PMC4219294.
- Yilmaz P, Parfrey LW, Yarza P, Gerken J, Pruesse E, Quast C, Schweer T, Peplies J, Ludwig W, Glöckner FO. 2014. The SILVA and “all-species living tree project (LTP)” taxonomic frameworks. Nucleic Acids Res. 42(Database Issue):D643–D648. doi:10.1093/nar/gkt1209.
- Zampini A, Nguyen AH, Rose E, Monga M, Miller AW. 2019 Dec 1. Defining dysbiosis in patients with urolithiasis. Sci Rep. 9(1):5425. Available from: http://www.nature.com/articles/s41598-019-41977-6.
- Zeng J, Zhang G, Chen C, Li K, Wen Y, Zhao J, Wu P. 2020 Dec 15. Alterations in urobiome in patients with bladder cancer and implications for clinical outcome: a single-institution study. Front Cell Infect Microbiol. 15(10):555508. doi:10.3389/fcimb.2020.555508.