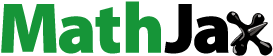
Abstract
Diabetes mellitus (DM) is an enormous public health issue worldwide. Recent data suggest that chronic arsenic exposure is linked to the risk of developing type 1 and type 2 DM, albeit the underlying mechanisms are unclear. This review discusses the role of the immune system as a link to possibly explain some of the mechanisms of developing T1DM or T2DM associated with arsenic exposure in humans, animal models, and in vitro studies. The rationale for the hypothesis includes: (1) Arsenic is a well-recognized modulator of the immune system; (2) arsenic exposures are associated with increased risk of DM; and (3) dysregulation of the immune system is one of the hallmarks in the pathogenesis of both T1DM and T2DM. A better understanding of DM in association with immune dysregulation and arsenic exposures may help to understand how environmental exposures modulate the immune system and how these effects may impact the manifestation of disease.
Introduction
Diabetes mellitus (DM) is a chronic, metabolic disease characterized by elevated levels of blood glucose, which can lead to serious damage to the heart, blood vessels, eyes (vessels of retina), kidneys and peripheral nerves over time. DM is a public health burden affecting 422 million people worldwide, and 1.5 million deaths are directly attributed to DM each year (WHO Citation2022). In all ages of the US population, there is an upward trend in the diagnosis of DM that has increased from 10.3% in 2001 to 13.2% in 2020, with an additional 38.0% of US adults being prediabetic (CDC Citation2022).
There are two major forms of DM, type 1 (T1DM) and type 2 (T2DM). T1DM, also referred to as insulin-dependent diabetes or juvenile diabetes, is characterized by the absence of or diminished production and release of insulin, resulting from autoimmune destruction of the islet beta-cells by autoantibodies and dysregulation of immune cells (Yoon and Jun Citation2005). So T1DM can be defined as a hypoinsulinemic and hyperglycemic state (Figure ). T2DM, also known as non-insulin-dependent diabetes, is a hyperinsulinemic state that occurs initially to compensate for insulin resistance in muscle, liver, and adipose tissues due to obesity, inflammatory responses and endoplasmic reticulum stress that alter insulin sensitivity (da Silva Rosa et al. Citation2020); but over time insulin levels are reduced due to exhaustion of islet beta-cells (Muoio and Newgard Citation2008), while the hyperglycemic state maintains (Figure ).
Figure 1. The process of development of DM. T1DM is characterized as a hypoinsulinemic and hyperglycemic state resulting from the autoimmune destruction of the islet beta-cells. T2DM is a hyperinsulinemic state initially to compensate insulin resistance in muscle, liver, and adipose tissues due to inflammatory responses or altered insulin receptor signaling; but over time insulin levels are reduced due to dysfunction of islet beta-cells, i.e. hypoinsulinemia. Hyperglycemic state remains in T2DM.
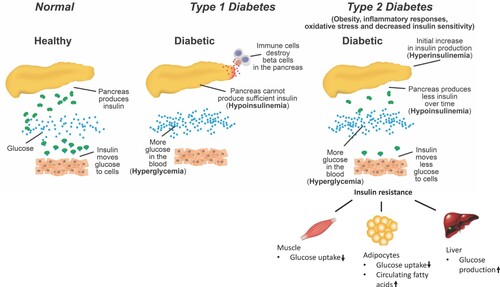
Despite significant advances in blood glucose management by insulin therapy, patients with DM remain at risk for developing lifetime complications. DM is considered to be the fifth-leading cause of death worldwide due to associated comorbidities, and it is one of the most costly diseases requiring life-long chronic treatment (Grandl and Wolfrum Citation2017). DM may be also associated with the COVID-19 pandemic (also known as severe acute respiratory syndrome (SARS)-CoV-2), which began at the end of 2019 and spread extremely quickly around the world. Recent data have shown that DM, cardiovascular diseases and chronic obstructive pulmonary diseases are the most prevalent comorbidities in COVID-19 patients (Sanyaolu et al. Citation2020). Individuals with DM, hypertension, and severe obesity are more likely to be infected and at a higher risk for progression, unfavorable prognosis, and death from COVID-19 (Chang et al. Citation2020; Guan, Ni, et al. Citation2020; Guan, Liang, et al. Citation2020; Muniyappa and Gubbi Citation2020; Onder et al. Citation2020; Yang et al. Citation2020). Additionally, there is a similar increased risk for severe acute respiratory syndrome (SARS) or Middle East Respiratory Syndrome (MERS) in individuals with DM (Muniyappa and Gubbi Citation2020).
The specific etiology of DM is unclear, but important and emerging risk factors include genetics, age, obesity, environment, and lifestyle. Recently, growing evidence supports the role of environmental contaminants such as pesticides, heavy metals and endocrine disruptors as possible risk factors in the development of T2DM (Chen et al. Citation2009; Alonso-Magdalena et al. Citation2011; Evangelou et al. Citation2016). Additionally, many environmental risk factors might also trigger autoimmune mechanisms possibly critical in the etiology of T1DM (Butalia et al. Citation2016; Rewers and Ludvigsson Citation2016). As such, it is particularly important to identify modifiable environmental risk factors for DM and to develop primary prevention strategies.
Arsenic is an environmental heavy metal with ubiquitous human exposure that is present in air, water and soil. Arsenic is biphasic in terms of its role both as a carcinogen and as a therapeutic agent depending upon concentration in a cellular milieu (Prajapati et al. Citation2011). Arsenic exposure has been linked to various pathological conditions including DM, many types of cancer (lung, kidney, liver, and bladder cancers), cardiovascular disease (hypertension and atherosclerosis), neurological disorders, gastrointestinal disturbances, liver disease, renal disease, reproductive health effects, and dermal changes (Jomova et al. Citation2011). In this review, we separately summarize the association of arsenic exposure and the risk of T1DM and T2DM, as well as mechanisms involving the immune system.
Inflammation and DM
T1DM is characterized by the chronic inflammation state of the pancreatic islets, in which the insulin-producing beta-cells are destroyed by auto reactive T-cells, inflammatory cytokines and monocytes (Grunnet and Mandrup-Poulsen Citation2011). A number of proinflammatory cytokines, such as interleukin (IL)-1β, tumor necrosis factor (TNF)-α, IL17, IL21 and IL23, have all been demonstrated to be important for T1DM development (Clark et al. Citation2017), and are upregulated significantly in T1DM patients compared to age-matched healthy control groups (Fatima et al. Citation2016).
T2DM is a chronic, low-grade inflammatory disease characterized by a long-standing imbalance of the immune system, metabolic syndrome, or excess nutrients associated with obesity (Dasu et al. Citation2007; Dasu et al. Citation2008; Shu et al. Citation2012). Cytokines produced by immunocompetent cells influence local or systemic inflammation, which are critical contributors to the development of insulin resistance and T2DM (Ballak et al. Citation2015). The IL1 family can affect the initiation and progression of insulin resistance by the upregulation of proinflammatory cytokines, such as IL1β and TNF-α, which can directly interfere with insulin signaling in adipocytes, hepatocytes, fibroblasts and myocytes (Tseng Citation2004; Koenen et al. Citation2011; Ballak et al. Citation2015). Besides, IL1β can inhibit beta-cell function and destroy beta-cell mass (Koenen et al. Citation2011). Insulin signaling is initiated by binding of insulin to the insulin receptors, then the receptor activates insulin receptor substrates (IRS-1 and IRS-2) through tyrosine phosphorylation. IRS are adaptor molecules in the insulin signaling pathway and dysregulation of IRS proteins may lead to glucose intolerance and insulin resistance (Kaleem et al. Citation2021). It is proposed that IL6 can also induce insulin resistance in adipocytes (Lagathu et al. Citation2003; Rotter et al. Citation2003) by decreasing expression of the beta-subunit of the insulin receptor, insulin receptor substrate 1 (IRS1), and the glucose transporter GLUT4, thereby reducing glucose transport and interfering with insulin receptor signaling (Muoio and Newgard Citation2008; Grunnet and Mandrup-Poulsen Citation2011). TNF-α has been found to decrease insulin sensitivity by increasing serine phosphorylation of IRS1and IRS2, reducing the docking of IRS to the insulin receptor and interfering with downstream signaling pathways (Le Roith and Zick Citation2001). Reactive oxygen species (ROS) resulting from hyperglycemia can induce insulin resistance, impair insulin synthesis and secretion, through its interactions with cytokines and other mediators (Tseng Citation2004; Yan Citation2014). A free fatty acid-bound glycoprotein, fetuin-A, has been shown to be an endogenous ligand for toll-like receptor 4 (TLR4) and can promote lipid-induced insulin resistance through TLR4–NF-κB pathway to produce proinflammatory cytokines (Pal et al. Citation2012).
These findings reveal that several pathogenic cascades of both T1DM and T2DM are mediated by inflammation and proinflammatory cytokines. In addition, hyperglycemia can cause dysfunction of the immune response and vice versa, which increases the incidence of infectious diseases and related comorbidities in patients with DM (Berbudi et al. Citation2020). It has been found that DM-associated inflammation can enhance the progression and be detrimental to the prognosis of patients with COVID-19 (Guo et al. Citation2020). In diabetic patients with COVID-19, serum levels of inflammation-related biomarkers such as IL6, C-reactive protein (CRP), serum ferritin and coagulation index, D-dimer, were all significantly higher than those in non-diabetic patients with COVID-19, indicating that patients with DM are more predisposed to form a hyperinflammatory state ultimately leading to rapid deterioration of COVID-19-infected patients (Guo et al. Citation2020). Also, in patients with DM, compromised innate immunity, pro-inflammatory cytokine milieu, reduced expression of coronavirus surface receptor angiotensin-converting enzyme 2 (ACE2) and use of renin-angiotensin-aldosterone system antagonists exacerbate the severity of COVID-19 (Pal and Bhadada Citation2020). All of the above reveal the close relationship between inflammatory responses and DM.
Arsenic exposure and development of DM
Arsenic characteristics
Arsenic is a ubiquitous environmental contaminant naturally occurring in organic and inorganic forms. Inorganic arsenic (iAs) is the predominant form in surface and groundwater reservoirs and much more toxic than organic arsenic (Sung et al. Citation2015). The most common trivalent iAs compounds are sodium arsenite, arsenic trioxide, and arsenic trichloride; whereas arsenic pentoxide, arsenic acid and arsenates are the most common pentavalent compounds. Arsenic can also occur in organic forms, such as arsanilic acid, dimethylarsinic acid, arsenobetaine, and methylarsonic acid (Straif et al. Citation2009).
Arsenic exposure in humans
People are widely exposed to arsenic compounds through contaminated drinking-water and food, industrial processes (alloying agent, glass, pigments, textiles, paper, metal adhesives, wood preservatives and ammunition, to a limited extent in pesticides and pharmaceuticals) and smoking (Figure ). The range of total arsenic concentrations in natural water is very large, from less than 0.5 µg/L to even more than 5000 µg/L, in which iAs (III) and iAs (V) are generally the dominant species, while organic forms of arsenic are minor (Smedley and Kinniburgh Citation2002). The WHO recommended limit of arsenic is 10 µg/L; however, high groundwater levels of arsenic (100 µg/L, predominantly iAs) affects over 200 million people worldwide, including areas of Bangladesh, India, Pakistan, China, the USA, Canada, Brazil, Vietnam, Indonesia, Hungary, and Mexico (Shahid et al. Citation2018). Small amounts of iAs are also consumed in the diet. Dietary intake of iAs in the US has been estimated to range from 1 to 20 µg/day (ATSDR Citation2007). Normal levels of arsenic in unexposed humans are: < 1µg/L in blood, ≤ 1 mg/L in nails and ≤ 1 mg/L in hair (ATSDR Citation2007). The Agency for Toxic Substances and Disease Registry (ATSDR) in the US has estimated minimal risk levels (MRLs) of iAs 5 µg/kg/day and 0.3 µg/kg/day, respectively for acute-duration (14 days or less) and chronic-duration (365 days or more) oral exposure to iAs (ATSDR Citation2007).
‘Dual-sided personality’ of arsenic in health and disease
Although the ratio of arsenic species exposure varies greatly in different places, globally, chronic exposure to iAs (especially sodium arsenite and arsenic trioxide) from drinking water has been reported to be associated with most health risks, such as cancers, developmental effects, diabetes, pulmonary and cardiovascular disease (Goldman Citation2020).
In addition to its harmful effects, an arsenic derivative, arsenic trioxide (As2O3) has also been used in the treatment of cancers, such as acute promyelocytic leukemia, usually at the dose of 10 mg per day (0.06–0.2 mg per kg body weight/day), which contributes significantly to improving survival rates exceeding 90–95% (Bachleitner-Hofmann et al. Citation2002; Rojewski et al. Citation2004; Fortier et al. Citation2020; Ferrara et al. Citation2021). As2O3 has also shown activity in solid tumors, multiple myeloma, myelodysplasia syndrome, high-grade neuroepithelial tumor of the central nervous system, breast cancer, pancreatic ductal adenocarcinoma and some autoimmune diseases, similar to other chemotherapeutic agents (Baj et al. Citation2002; Goel et al. Citation2012; Kritharis et al. Citation2013; Lang et al. Citation2016; Paret et al. Citation2017). In addition, sodium meta-arsenite has been reported to have anti-tumor effects in human prostate cancer and is a candidate for the treatment of refractory non-Hodgkin’s lymphoma, as well as salvage chemotherapy in refractory biliary tract cancers (Jo et al. Citation2019). The anticancer activity of arsenic is partly dependent on the production of ROS to inhibit cancer cell migration, shorten telomeres, and enhance cell death (Li et al. Citation2006; Liu et al. Citation2011). Besides, based on its immunosuppressive effects, As2O3 might be used as an anti-rejection agent in allotransplant for cardiac disease or T1DM. In a mouse heart transplantation model and islet xenotransplantation, arsenic trioxide was reported to alleviate allograft rejection and prolong xenograft survival in mice (Yan et al. Citation2009; Zhao et al. Citation2018). However, as As2O3 is also a well-known toxicant and carcinogen because of its pro-inflammatory effects, the key point lies in balancing the pros and cons of accurate As2O3 applications (Zhang et al. Citation2018). Research on the ‘dual-sided personality’ of As2O3 can help further elucidate its therapeutic and toxic effects for appropriate use in curing diseases.
Arsenic exposure and the risk of DM
There is existing epidemiological evidence that chronic arsenic exposure, especially iAs and methylated metabolites, monomethylated arsenic (MMA) and dimethylated arsenic (DMA), is associated with DM, primarily T2DM (Navas-Acien et al. Citation2008; Maull et al. Citation2012; Bahadar et al. Citation2014; Currier et al. Citation2014; Huang et al. Citation2014; Brown Citation2015; Martin et al. Citation2015). Numerous epidemiological studies show a strong diabetogenic effect of arsenic in human populations globally, such as Pakistan (Bahadar et al. Citation2014), Mexico (Currier et al. Citation2014; Martin et al. Citation2015), Cambodia (Huang et al. Citation2014), Chile (Castriota et al. Citation2018), Bangladesh (Paul et al. Citation2019) and Canada (Brown Citation2015). People in these regions, regardless of if there is low or high iAs water concentrations, have a higher risk of developing T2DM compared to unexposed populations, but the risk exhibits a dose- and time-response pattern associated with the increase of iAs concentrations in the water and long-term exposure times (Martinez-Castillo et al. Citation2021). A National Toxicology Program (NTP) workshop concluded that arsenic is linked to an increased risk of T2DM in populations exposed to high levels of arsenic (≥ 150 µg/L arsenic in drinking water), including iAs, MMA and DMA (Maull et al. Citation2012). Moreover, investigators have also reported that long-term exposure to low-level arsenic (< 10 µg/L arsenic in drinking water) also contributes to the development of T2DM (Bahadar et al. Citation2014). A study from rural Bangladesh explored the dose-dependent association of drinking water containing low-to-moderate levels of arsenic (50.01–150 µg/L) confers a greater risk of hyperglycemia than safe drinking water (As ≤ 10 µg/L) (Paul et al. Citation2019). In the 2003–2004 National Health and Nutrition Examination Survey (NHANES), 788 adults were studied and found that participants with T2DM had a 26% higher level of total arsenic in their urine than those without T2DM (Navas-Acien et al. Citation2008). Similarly, researchers pointed out that workers from high arsenic exposure occupations, such as copper smelters and the art glass industry, had a higher prevalence of DM in long-term follow-up studies (Rahman and Axelson Citation1995; Rahman et al. Citation1996). A meta-analysis of dose–response indicated that T2DM risk increased by 13% for every 100 µg/L increment of iAs in drinking water (Wang et al. Citation2014).
In addition, particular subpopulations especially children may be at increased risk for health effects resulting from arsenic exposure, due to altered metabolism or underlying genetic predispositions, life stages, and cultural dietary factors (Lai et al. Citation2015). The fetus and young children may be at particular risk as a result of developmental windows of unique sensitivity to arsenic toxicity (Miller et al. Citation2002). In a SEARCH for Diabetes in a Youth Case–Control (SEARCH-CC) study, arsenic metabolism, characterized by the relative proportions of iAs% versus MMA% and DMA% in plasma, was associated with a higher odds of T1DM in children and adolescents (Grau-Perez et al. Citation2017). A growing number of epidemiology studies also support a potential role of maternal arsenic exposure in the development of gestational diabetes mellitus (GDM) (Salmeri et al. Citation2020). GDM is a common metabolic disease that develops during pregnancy and usually resolves after delivery. However, GDM is detrimental for both mothers and their children, with a higher possibility for mothers to develop T2DM later in life (Bellamy et al. Citation2009), and for children to exhibit impaired fetal endothelial function and have greater risks of developing T2DM (Dabelea et al. Citation2008; Kelstrup et al. Citation2013; Sultan et al. Citation2015). Farzan found that for every 5 µg/L increase in total arsenic in a woman’s household well water, there was a nearly 10% greater risk of GDM (Farzan, Gossai, et al. Citation2016). Another study of maternal-infant and birth cohort found that in utero arsenic exposure was associated with cord blood DNA methylation of genes involved in signaling pathways important in T1DM and T2DM development, as several DNA methylation patterns positively correlated with elevated low-density lipoprotein (LDL) levels later in life, one characteristic of diabetic dyslipidemia (Kaushal et al. Citation2017). Epidemiological evidence from different countries/regions has suggested the positive correlation between maternal urinary arsenic concentrations and GDM (Ashley-Martin et al. Citation2018; Wang et al. Citation2019; Wang et al. Citation2020). The detrimental effects of arsenic on glucose homeostasis have also been found in animal studies. In pregnant rats, sodium arsenite exposure from drinking water alters glucose homeostasis by altering beta-cell function, and increasing the risk of GDM development; in pups, it induces low body weight from birth to 8 weeks of age, and glucose intolerance in females (Bonaventura et al. Citation2017).
Humans are susceptible to extended exposures to multiple toxic metals daily, so sometimes it is rather difficult to find the exact mechanism through which each individual heavy metal causes DM (Javaid et al. Citation2021). Many epidemiological studies have investigated the associations between multiple heavy metal exposures in combination with arsenic and the impact on DM risk. A retrospective nested case–control study of 1359 pregnant women in China measured concentration of heavy metals (including arsenic, mercury, lead, cadmium, and chromium) in their newborns’ meconium samples and found that arsenic, cadmium, and chromium were positively associated with GDM prevalence in a dose-dependent manner (Peng et al. Citation2015). In occupational worker populations, a large prospective cohort study demonstrated that multiple urinary metals, particularly arsenic, nickel, zinc and cobalt, were associated with elevated glucose and dysglycemia among occupational workers in the metal industry (Yang et al. Citation2017).
Arsenic and immune responses
Dual adverse effects of arsenic on immune responses
The immune system is a protective complex of cells, special organs, and chemical mediators that prevent cellular or tissue damage in response to various pathogens or toxic insults. Increasing lines of evidence indicate that arsenic may adversely affect the immune system, by inducing proinflammatory or immunosuppressive effects, which we will discuss separately below.
In populations chronically exposed to low levels of arsenic (in the range above the WHO-recommended limit of 10 µg/L but ≤ 50 µg/L) in drinking water, arsenic has been found to stimulate the immune response (Sinha et al. Citation2014; Dutta et al. Citation2015). Prenatal and childhood exposure to arsenic (iAs + MMA + DMA) were found to be significantly associated with the occurrence of asthma and allergic rhinitis in children (Tsai et al. Citation2021). iAs, mainly sodium arsenite, has been well-documented to be associated with inflammatory responses in bronchial epithelial cells (Luo et al. Citation2013), urothelial cells (Liu, Sun, et al. Citation2014), skin (Li et al. Citation2011), atherosclerotic lesions (Srivastava et al. Citation2009), vascular (Kesavan et al. Citation2014), keratinocytes (Lu et al. Citation2015), and pulmonary tissue and also systemically in populations with histories of low level arsenic exposures (Sinha et al. Citation2014; Dutta et al. Citation2015). In mice prenatally exposed to sodium arsenite, the concentrations of arsenic in liver reached 1.2 mg/kg, and the mice showed altered developmental programming in fetal liver and a proinflammatory state postnatally (States et al. Citation2012). Also, it has been shown that chronic low-level arsenic-exposure in women can evoke systemic inflammation by upregulating proinflammatory mediators such as TNF-α, IL6, IL8, IL12, and CRP (Prasad and Sinha Citation2017), many of which have been proposed as important regulators of insulin resistance in T2DM. The inflammatory state, in the long run, may contribute towards the onset of chronic diseases including atherosclerosis, rheumatoid arthritis, cancers and DM.
Alternatively, increasing literature from epidemiological and experimental studies have reported that arsenic exposure is immunosuppressive and enhances susceptibility to infections or induces immune tolerance (Rahman et al. Citation2011; Smith et al. Citation2011; Ramsey, Foong, et al. Citation2013; Farzan, Li, et al. Citation2016; Li, Guo, et al. Citation2021). In some prospective study cohorts, prenatal exposure to iAs through maternal drinking water was associated with a higher morbidity in respiratory infections, diarrhea during infancy or hepatitis B seroprevalence (Rahman et al. Citation2011; Farzan, Li, et al. Citation2016; Cardenas et al. Citation2018). Also, arsenic exposure increased susceptibility to opportunistic infections like tuberculosis (Smith et al. Citation2011), or other diseases associated with immunosuppression, such as skin cancers in HIV-positive or organ transplantation populations (Soto-Pena et al. Citation2006). Mechanisms proposed for arsenic-induced immunosuppression has been changing CD4/CD8 T cell ratio (Soto-Pena et al. Citation2006) and interference with local immunity (Ramsey, Bosco, et al. Citation2013), altered microRNA patterns involved in innate and adaptive immune pathways (Rager et al. Citation2014), as well as decreased numbers of antigen presenting dendritic cells (DCs) in lymph nodes (Kozul et al. Citation2009) or induced expression of Heme oxygenase-1 (HO-1) protein to assist arsenic-suppressed production of pro-inflammatory factors and Th1/Th17-inducible cytokines in DCs (Li, Guo, et al. Citation2021) and inhibited proteolysis in antigen processing (Harrison and McCoy Citation2001).
Taken together, these findings suggest that arsenic exposure can result in immune stimulation or suppression. It is unclear if these immunotoxicological effects of arsenic are time-dependent or dose-dependent. An in vivo study of chronic sodium arsenite exposure in mice via drinking water showed that chronic arsenic exposure induces a time-dependent modulation of the inflammatory response at the initial stage of exposure and immunosuppression at a later stage of treatment in the spleen (Yan et al. Citation2020). There are in vitro studies showing that arsenic can either induce suppression or proliferation of immune cells in a dose-dependent manner (Meng and Meng Citation2000), but not all reflected a clear dose-dependent effect (Soto-Pena and Vega Citation2008). For example, the immunosuppressive effects were pronounced in male cotton rats exposed to 5 mg/L sodium arsenite in drinking water for 6 weeks (Savabieasfahani et al. Citation1998); whereas in another study, 0.5 mg/L sodium arsenite exposure in drinking water for 5 weeks in C57BL/6 mice enhanced inflammatory-induced angiogenesis (Straub et al. Citation2007). The reasons for these differences may be related to gender, species, strain, cell types, arsenic concentration or metabolic capacities of systems evaluated.
Mechanisms of arsenic-induced dual effects on innate and adaptive immunity
Dual effects of arsenic on innate immunity
Innate immunity serves as the first line of defense to protect against deleterious microorganisms or foreign materials or substances. Arsenic has been shown to have dual immunosuppressive and proinflammatory effects on innate immunity. On one hand, arsenic is increasingly being recognized as a suppressor of innate immunity (Kozul et al. Citation2009; Xu et al. Citation2018; Medina et al. Citation2020). Arsenic (mainly iAs) exposures from drinking water have been implicated in persistent opportunistic bacterial and viral infections in adults and in children exposed early in life (Mazumder et al. Citation2000; Smith et al. Citation2006; Raqib et al. Citation2009). One of the major components of innate immunity is the phagocytic leukocytes, mainly neutrophils and macrophages. Human macrophages are the major targets of arsenic-mediated immunotoxicity (Lemarie et al. Citation2006). Several studies as shown below have indicated that iAs can induce cell apoptosis and functional suppression of macrophages. Macrophages from arsenic-exposed people with skin lesions and whose urine arsenic levels averaged 642 µg/L, show cell rounding accompanied by significantly reduced adhesion, decrease in nitric oxide production, and impaired phagocytosis (Banerjee et al. Citation2009). Functional suppression of macrophages induced by environmentally relevant concentrations of iAs (50-500 nM sodium arsenite) in THP-1 derived macrophages is manifested by increased cell apoptosis, suppression of two pro-inflammatory cytokines, IL-1β and TNF-α, as well as inhibition of nitric oxide production (Xu et al. Citation2018). Epidemiologic evidence also shows the altered suppressive effects on innate immune function in children exposed to low-dose arsenic early in life (Parvez et al. Citation2019). In an experimental mouse model of bacteremic Staphylococcus aureus (S. aureus) infection, sodium arsenite (0.5 mg/kg/d) exposure via i.p. injection for 15 days significantly increased bacterial load in the blood, decreased adhesion properties and phagocytic activity of splenic macrophages, and delayed bacterial clearance by the spleen (Bishayi and Sengupta Citation2003). Another mouse study found iAs exposure was immunotoxic to intraepithelial lymphocytes (IELs) and innate immune cells in the small intestine of male and female mice following chronic drinking water exposures to sodium arsenite (Medina et al. Citation2020). Exposure to iAs resulted in a reduction of small intestinal CD4+ IELs (TCRαβ+, CD8αα+) and a decrease in mature macrophages of innate immune cell subsets (Medina et al. Citation2020).
Another major component of innate immunity is the inflammatory response which involves the release of various cytokines. Arsenic can impair the ability of macrophages to produce cytokines. Expression of macrophagic surface markers is also affected altering endocytosis and phagocytosis (Abdul et al. Citation2015). Microarray-based genome-wide expression studies of peripheral blood mononuclear cells (PBMC) from populations have shown the association between arsenic exposure and expression of inflammatory genes, such as IL2RA, IL1B, IL6 and TNF (Dangleben et al. Citation2013).
Alternatively, there are reports that arsenic can promote innate immune responses. The microglial cell is the key cell type involved in innate immune responses in the CNS, and its activation is linked to inflammation and neurotoxicity (Chen et al. Citation2016). In cultured microglial cells treated with arsenic trioxide from 0.5 to 20 nm, the expression and secretion of IL6 was increased in a dose- and time-dependent manner (Chen et al. Citation2016). In another in vitro study, treatment with sodium arsenate (0.5–100 µm) in a murine keratinocyte cell line, arsenic induced overproduction of cytokine IL1α which promotes skin hyperkeratosis and cancer (Corsini et al. Citation1999). In pregnant mice exposed to 100 µg/L sodium arsenite via drinking water from gestation day 8 until birth, genes related to mucus production and innate immunity were increased in the lungs of their offspring (Ramsey, Bosco, et al. Citation2013).
Dendritic cells play a critical role that link innate and adaptive immunity. They are central in the initiation of immune responses that include processes such as phagocytosis, antigen presentation and processing, in addition to T-cell activation and cytokine secretion. Innate and adaptive responses are orchestrated by dendritic cells, and minimal alterations in their function and stability and can majorly impact the immune system (Bahari and Salmani Citation2017). Many responses of dendritic cells are decreased in the presence of very low and non-cytotoxic concentrations of arsenic, through decreased secretions of the key cytokines like IL2, that reduce dendritic cell maturation and cellular immune responses (Macoch et al. Citation2013; Macoch et al. Citation2015).
Studies summarizing the effects of arsenic on the innate immune response are shown in Table . So far, the present studies may not draw a conclusion to elucidate whether there is commonality in the immunosuppressive or proinflammatory effects of arsenic on innate immunity. Further multidisciplinary studies including molecular epidemiologic investigations are needed.
Table 1. Effects of arsenic on the immune system.
Dual effects of arsenic on adaptive immunity
Adaptive immunity involves specific T-lymphocytes and B-lymphocytes, which respond to different antigens during our lifetime. Arsenic has been shown to have dual immunosuppressive and proinflammatory effects on adaptive immunity. One of the major ways arsenic interferes with the adaptive immune system is through its immunosuppressive effects by impairing the development, activation, proliferation and function of lymphocytes and B- and T-cell subpopulations (Abdul et al. Citation2015). In a cross-sectional study from a high arsenic polluted region in India, arsenic exposure through drinking water at the average level of 189.65 µg/L suppressed T-cell proliferation and decreased the levels of cytokines (TNF-, IFN
, IL2, IL10, IL5 and IL4) secreted by the T cells (Biswas et al. Citation2008). In children with a mean arsenic concentration (186.7 µg/L in urine) of iAs and metabolites MMA and DMA, CD4 subpopulation proportions, CD4/CD8 ratio, and IL2 secretion levels were all reduced, as well as a tendency of increased incidences of immune-related conditions (asthma, allergies, and parasitic infections) were noted among individuals with arsenic values higher than 50 µg/L in their urine (Soto-Pena et al. Citation2006). Chronic arsenic exposure in copper smelter workers also induces lymphocyte oxidative damage showing a positive correlation between urinary arsenic levels and oxidative stress markers (Escobar et al. Citation2010). Additionally, impaired T cell functions have also been reported in experimental animals exposed to arsenic. In mice following a two-week inhalation exposure to arsenic trioxide at concentrations of 50 µg/m3 and 1 mg/m3, the T-dependent humoral immune response was highly susceptible to arsenic suppression (Burchiel et al. Citation2009). In vitro experiments also show that sodium arsenite reduces T cell proliferation induced by phytohemaglutinin (PHA), and interferes with expression of several immune and stress genes (Martin-Chouly et al. Citation2011).
Additionally, it has been found that arsenic can stimulate the adaptive immune system into a proinflammatory state. Long-term exposure to arsenic increased gene expression of inflammatory molecules (IL1B, IL6, CCL2/MCP1, MMP1, etc.) in circulating lymphocytes of people with intermediate (4.64–9.00 µg/L), and high (9.60–46.5 µg/L) levels of blood arsenic (Wu et al. Citation2003). In male workers from nonferrous plants located in the northeastern part of China with exposure to arsenic, urinary IL8, TNF- and TGF-
levels are also positively associated with urinary iAs concentrations (Liu, Sun, et al. Citation2014). Arsenic can promote inflammation in vivo (Srivastava et al. Citation2009; Li et al. Citation2011; Kesavan et al. Citation2014) and in vitro (Luo et al. Citation2013; Lu et al. Citation2015), all of which provide evidence of a proinflammatory response induced by arsenic. Studies characterizing the effects of arsenic on the adaptive immune system are summarized in Table .
Mechanisms of arsenic-induced DM through immunity
Based on the above associations between arsenic, the immune system and DM, which includes: (1) Arsenic is a well-recognized modulator of the immune system; (2) dysregulation of the immune system is one of the hallmarks in the pathogenesis of DM; and (3) iAs exposure increases the risk of development of DM, we suggest that iAs exposure predisposes to dysfunctional immune responses, thereby alters the expression and secretion of inflammatory cytokines and mediators that could possibly initiate the development of T1DM or T2DM (as illustrated in Figures and , respectively). Therefore, we review the role of immune system as a link to explain potential mechanisms of iAs-induced DM in human populations, animal models, and in vitro studies.
Figure 3. Mechanisms of arsenic-induced effects on immunity in T1DM. Arsenic can promote the development of T1DM through direct pancreatic beta-cell destruction, gut microbiome alterations inducing abnormal autoimmune or inflammatory responses.
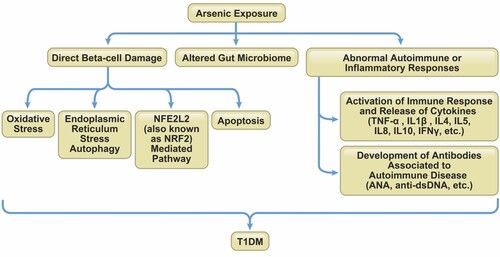
Figure 4. Mechanisms of arsenic interference of immunity in T2DM. Arsenic-induced T2DM is mainly through insulin resistance due to impairing the insulin signaling cascade, affecting oxidative stress and antioxidant pathways and inducing chronic inflammation; pancreatic beta-cell damage and dysfunction due to ROS production and enzymatic impairment; stimulation of liver gluconeogenesis due to Pck1 expression, among which the immune system is implicated to be involved in these processes.
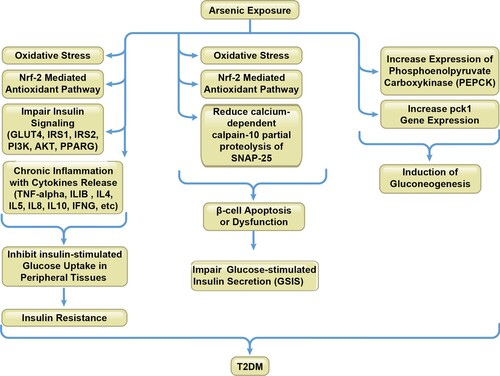
Mechanisms of arsenic-induced effects on immunity in development of T1DM
T1DM is primarily characterized by impaired insulin secretion as a result of an autoimmune assault on pancreatic islet beta-cells. There are few experimental animal studies or epidemiological studies that have directly evaluated the role of arsenic in T1DM. An epidemiological study supports the role of arsenic metabolism in T1DM, showing that low iAs% versus a high MMA% and DMA% in plasma of youth was associated with a higher odds of T1DM (Grau-Perez et al. Citation2017).
Several points of evidence of mechanistic data that supports an association between arsenic and T1DM have been proposed and includes the following.
Direct beta-cells damage. Pancreatic beta-cells are recognized as a target of iAs and methylated metabolites (MMA and DMA) in humans, animals, and in in vitro models (Fu et al. Citation2010; Douillet et al. Citation2013; Wu et al. Citation2018), by inducing the release of TNF-α and ILs, activating oxidative stress and transcription factor NFE2L2 (Nuclear factor, erythroid 2 like 2; also known as NRF2)-mediated antioxidant response, and eliciting a pancreatic islet-autonomous effect by an endoplasmic reticulum stress-autophagy pathway, which all play an important role in islet beta-cell destruction in T1DM (Tseng Citation2004; Chen et al. Citation2009; Khurana et al. Citation2018; Wu et al. Citation2018). Low-level sodium arsenite can also induce apoptosis by inhibiting TrxR activity in pancreatic beta-cells (Yao et al. Citation2015). These direct undesirable effects on beta-cell function and enhanced apoptosis may be possible mechanisms of T1DM development due to arsenic exposure (Tseng Citation2004).
Altered gut microbiome. The gut and intestinal microbiome composition and metabolic profile can be altered by chronic arsenic exposure during prenatal development and early life in children (Dong et al. Citation2017) and by acute/chronic sodium arsenite exposure in adult mice (Lu et al. Citation2014; Li, Yang, et al. Citation2021). In a recent study of mice exposed to sodium arsenite (50 mg/L) for 6 months, long-term exposure to iAs changed the structure of intestinal tissues, affected the Beta diversity of intestinal flora, altered the composition of the intestinal microbiota from phylum to species, and changed the composition of metabolites (Li, Yang, et al. Citation2021). The changes in the gut/intestinal microbiome and metabolome induced by arsenic may potentially influence susceptibility to metabolic diseases such as T1DM. Some human population studies have observed an altered microbiota in T1DM patients (de Goffau et al. Citation2014; Pellegrini et al. Citation2017; Vatanen et al. Citation2018). The association between T1DM and the gut microbiome is that microbiome dysbiosis in individuals may lead to abnormal immunoregulation that enables autoimmune destruction of islet beta-cell. Also, the loss of epithelial barrier integrity augments gut/intestinal permeability, and may ultimately trigger beta-cell autoimmunity by activating islet-reactive T cells, release of cytokines, beta-cell destruction with induction of T1DM (Butalia et al. Citation2016; Zhang et al. Citation2021).
Abnormal autoimmune or inflammatory responses. iAs may impair the immune system in humans and animals, especially interfering with the adaptive immune system by altering the development, activation, proliferation and function of subpopulations of lymphocytes (Dangleben et al. Citation2013; Abdul et al. Citation2015). Chronic iAs exposure from drinking water increases serum levels of antinuclear antibodies (ANA) and anti-doubled stranded DNA (dsDNA) antibodies, thereby possibly increasing the incidence of autoimmune diseases like T1DM (Das et al. Citation2012). Improper activation of the immune system may lead to allergic responses or trigger autoimmunity, and exposure to arsenic has been also reported to be associated with asthma and/or allergies in an epidemiological study (Das et al. Citation2014). Most likely, chronic long-term arsenic exposure enhances immune responses against self-antigens within the islet beta-cells and disrupts beta-cell function, thereby exacerbating the severity of the autoimmune diabetogenic process.
The mechanisms of arsenic interference on immunity in T1DM are summarized in Figure and associated studies are shown in Table .
Table 2. Molecular mechanisms of arsenic-induced immune dysfunction in T1DM.
Molecular mechanisms of arsenic interference of immunity in development of T2DM
Many epidemiological studies have reported an association between arsenic exposure and T2DM (Navas-Acien et al. Citation2008; Maull et al. Citation2012; Brauner et al. Citation2014), with the major mechanisms including: (1) inhibition of insulin-dependent glucose uptake (insulin resistance) due to inhibition of the insulin-activated signaling cascade and glucose transport system; (2) pancreatic beta-cell damage and dysfunction due to oxidative stress and enzymatic impairment; (3) stimulation of liver gluconeogenesis due to expression of phosphoenolpyruvate carboxykinase (PCK1, also known as PEPCK), among which the immune system is involved in the above processes (Martin et al. Citation2017). The mechanisms of arsenic interference in immunity and development of T2DM are summarized in Figure and associated studies are shown in Table .
Table 3. Molecular mechanisms of arsenic-induced immune dysfunction in T2DM.
Arsenic-induced insulin resistance and inhibition of insulin-stimulated glucose uptake (ISGU)
A major mechanism involved in the development of insulin resistance and pancreatic beta-cell dysfunction is oxidative stress, and this process is thought to be critical in the pathogenesis of T2DM (Pi et al. Citation2010). One of mechanisms of arsenic-associated T2DM is inhibition of insulin-dependent glucose uptake through generation of ROS and upregulation of antioxidant pathways including NFE2L2, leading to insulin resistance through decreasing ISGU in insulin sensitive peripheral tissues such as adipose and muscle (Xue et al. Citation2011; Padmaja Divya et al. Citation2015). In adipocytes and myotubes, sodium arsenite induces insulin resistance via oxidative stress-regulated mitochondrial Sirt3-FOXO3a signaling pathway (Padmaja Divya et al. Citation2015). In primary mouse pancreatic beta-cells and hepatocytes, sodium arsenite produced cyto-degenerative effects and ROS accumulation, and in vivo, sodium arsenite impaired insulin signaling by reducing expression levels of GLUT4, IRS1, IRS2, PI3 K, AKT, and PPAR signaling molecules (Chakraborty et al. Citation2012). Moreover, excessive levels of ROS result in upregulation of antioxidant pathways. In mice, iAs exposure stimulates generation of ROS and activates the NFE2L2-mediated cellular antioxidant pathway in the immune system (Duan et al. Citation2015). In 3T3-L1 adipocytes, sodium arsenite exposure resulted in upregulation of NFE2L2 and the subsequent increase in antioxidant enzyme expression, which blunted insulin-stimulated ROS signaling and thus impairing ISGU (Xue et al. Citation2011). Also, sodium arseniteinduced significant induction of inflammatory response genes and decreased expression of GLUT4, which meant decreased transport and utilization of the glucose, suggesting the involvement of chronic inflammation and reduction of GLUT4 in iAs-induced insulin resistance (Xue et al. Citation2011).
Interestingly, the exact mechanisms underlying NFE2L2’s role in insulin resistance have not been fully clarified. Most studies found that increased NFE2L2 inhibited insulin signaling and aggravated insulin resistance, while NFE2L2 deletion ameliorated insulin resistance; however, some conflicting results have been reported based on the different approaches and animal models (Li et al. Citation2020). Therefore, more research is needed to elucidate the role of NFE2L2 in arsenic-induced insulin resistance.
Another possible mechanism for arsenic-induced T2DM is the inhibition of ISGU by disrupting insulin-stimulated signal transduction pathway PKB/Akt-dependent mobilization of GLUT4 transporters in adipocytes. A series of studies from Dr Miroslav Styblo’s group have shown that trivalent arsenicals, arsenite and methylarsonous acid decreased ISGU in 3T3-L1 adipocytes by inhibiting PKB/Akt phosphorylation (Walton et al. Citation2004), and they further identified the key mechanism responsible for this effect was by the inhibition of the PDK-1/PKB/Akt-mediated transduction step (Paul et al. Citation2007). Follow-up studies in male C57BL/6 mice chronically exposed to arsenite or methylarsonous acid in drinking water confirmed that arsenite could induce impaired glucose tolerance in mice, but only at a higher concentration (50 mg/L) than that in humans (Paul et al. Citation2008). This may because of the higher rate of iAs metabolism and clearance in mice compared to humans.
In addition, arsenic may induce a status of insulin resistance through its molecular effects on gene expression of certain cytokines, which may contribute to arsenic-induced T2DM. Arsenic has been demonstrated to increase the production of cytokines involved in inflammation. Expression of inflammatory genes IL1B and IL6 were elevated in association with arsenic exposure in human subjects (Wu et al. Citation2003). In a US pregnancy cohort in New Hampshire, where arsenic levels exceeded the current EPA maximum contaminant level of 10 µg/L, in utero arsenic exposure has shown greater IL1β expression in the placenta and changes of T cell phenotypes and T cell function in cord blood, which may alter fetal immune system development and lead to immune dysregulation (Nadeau et al. Citation2014). Another study in Bangladesh showed that maternal exposure to iAs during pregnancy increased oxidative stress and proinflammatory cytokines (IL1β, TNF-α, and IFNγ) in the placenta and altered cord blood cytokines (IL1β, IL8, IFNγ, TNF-α) (Ahmed et al. Citation2011). Abundant evidence has confirmed TNF-α, IL6 and IL1β are mediators of insulin resistance due to their interference with insulin receptor signaling in adipocytes, hepatocytes, fibroblasts and myocytes (Le Roith and Zick Citation2001; Lagathu et al. Citation2003; Rotter et al. Citation2003; Koenen et al. Citation2011; Ballak et al. Citation2015), supporting that chronic arsenic exposure can lead to insulin resistance by inducing cytokines and regulating related genes, thereby promoting the development of T2DM.
Arsenic-induced beta-cell dysfunction and impaired insulin secretion
Pancreatic beta-cells can be targeted and damaged by oxidative stress induced by arsenic in the development of T2DM. Studies report that arsenic can increase ROS generation, which impairs insulin expression or glucose-stimulated insulin secretion (GSIS), and induces beta-cell dysfunction and apoptosis (Fu et al. Citation2010; Liu, Guo, et al. Diaz-Villasenor et al. Citation2006; Izquierdo-Vega et al. Citation2006; Lu et al. Citation2011; Citation2014; Yao et al. Citation2015; Pan et al. Citation2016). In primary rat pancreatic beta-cells, 5 µM sodium arsenite exposure contributed to the development of DM by impairing beta-cell function, mainly in insulin synthesis and secretion (Diaz-Villasenor et al. Citation2006). Subchronic oral exposure of rats to sodium arsenite (1.7 mg/kg) for 90 days produced oxidative stress-induced pancreatic damage, which could activate stress-sensitive signaling pathways to induce insulin resistance and also impair insulin secretion due to cellular damage (Izquierdo-Vega et al. Citation2006). Another in vivo study showed that iAs exposure could cause pre-diabetic effects in normal mice and worsen the diabetic effects in diabetic mice, by altering lipid metabolism, gluconeogenesis and insulin secretion, and causing oxidative damage and inflammation in the pancreas (Liu, Guo, et al. Citation2014).
Numerous in vitro studies also consistently demonstrated that iAs can increase ROS generation to reduce insulin synthesis and/or secretion, and induce beta-cell apoptosis (Fu et al. Citation2010; Lu et al. Citation2011; Yao et al. Citation2015; Pan et al. Citation2016). In rat INS-1 cells exposed to 10 µm of sodium arsenite for 72 h, iAs induced pancreatic beta-cell apoptosis through activation of a lysosome-mitochondrial pathway by increasing ROS level (Pan et al. Citation2016). Additionally, exposure to low levels of sodium arsenite in INS-1 cells also enhanced NFE2L2 and other antioxidant enzymes levels, which are inclined to dampen ROS signaling in GSIS, thus disturbing beta-cell function (Fu et al. Citation2010). Interestingly, similar to NFE2L2’s role in insulin resistance, some studies have reported contradictory results on the role of NFE2L2 in arsenic-induced beta-cell damage. In mouse MIN6 cells or islets with stable knockdown of Nfe2l2, deficiency of NFE2L2 rendered beta-cells vulnerable to iAs-induced cell damage, and pretreatment of MIN6 cells with NFE2L2 activator protected the cells from iAs-induced cell damage in a NFE2L2-dependent fashion (Yang et al. Citation2012). It’s not clear if NFE2L2 plays paradoxical roles in beta-cell dysfunction induced by environmental arsenic exposures. Further studies are needed to investigate the precise role of NFE2L2 in arsenic-induced beta-cell dysfunction. In addition to causing cell death, the accumulation of ROS has been shown to participate in chronic inflammation which results in pancreatitis in vivo, leading to the development of DM (Yen et al. Citation2007).
There is another mechanism reported by A. Díaz-Villaseñor et al, by which arsenic impairs insulin secretion without altering its synthesis. They found that arsenite-impaired insulin secretion in RINm5F rat pancreatic beta-cells was due to the reduced levels of free intracellular calcium, thus decreasing calpain-10-mediated proteolysis of SNAP-25, a member in the insulin secretory machinery, and limiting insulin exocytosis (Diaz-Villasenor, Burns, et al. Citation2008). Since calpains play an important role in insulin secretion and action as nonlysosomal calcium-dependent cysteine proteases, they compared the activity and expression in lymphocytes of individuals with or without T2DM, and showed that the activity of calpain-10 in lymphocytes was glucose-dependent and decreased in diabetic patients (Diaz-Villasenor, Hiriart, et al. Citation2008). This indicates the important role of enzymatic impairment in the development of T2DM, which not only occurs in tissues involved in glucose-uptake and metabolism such as the pancreas, adipose, liver, and skeletal muscle which directly regulate glucose homeostasis; but also can arise in white blood cells such as lymphocytes, which contain insulin receptors involved in insulin clearance and metabolism.
Arsenic-associated stimulation of gluconeogenesis
In addition to insulin resistance and insulin secretion alteration, arsenic can induce hepatic gluconeogenesis, which is the pathway for synthesis of glucose from noncarbohydrate sources during fasting. PCK (also known as PEPCK) is a key rate-limiting enzyme in hepatic gluconeogenesis, resulting in fasting hyperglycemia (Friedman et al. Citation1993). Sodium arsenite has been shown to increase both basal and hormone-induced Pck1 gene expression in chick embryos in vivo and in cultured rat hepatoma H411E cells at non-overtly toxic doses (Hamilton et al. Citation1998). Increased expression of Pck1 is also found in mice exposed to arsenic trioxide (Huang et al. Citation2015). Sodium arsenite can also upregulate Pck1 gene expression, whose overexpression can increase gluconeogenesis in liver and blood glucose levels in mice (Liu, Guo, et al. Citation2014). These data collectively, suggest arsenic-induced hepatic gluconeogenesis which may result in hyperglycemia and DM.
Mitigation strategies for arsenic exposure
A major threat to public health posed by arsenic is from drinking water and dietary intake of food crops irrigated by contaminated water worldwide (WHO Citation2006). Therefore, mitigation strategies focus primarily on reducing consumption of arsenic-rich drinking-water and arsenic-contaminated water sources. Various solutions for reduction in sources of arsenic contamination fall into two broad categories: alternative arsenic-safe water sources and treatment of arsenic-contaminated water supplies (Sanyal et al. Citation2020). Alternative water sources include groundwater (deep tube wells, shallow shrouded tube wells, or infiltration gallies), cleaning up of surface water (community filtration systems, household filters), and rainwater harvesting (Krupoff et al. Citation2020; Sanyal et al. Citation2020). Drinking water highly contaminated with arsenic can be mitigated in several different ways, such as sedimentation, oxidation/filtration to remove iron and manganese, enhanced coagulation/filtration and lime softening, as well as membrane processes including reverse osmosis, electrodialysis, ultrafiltration, and nanofiltration (WHO Citation2006; Sanyal et al. Citation2020).
In regards to dietary absorption of arsenic, crops irrigated with arsenic-contaminated water pose another major source of human exposure. Recent studies have reported that the adaptation of different agronomic techniques can effectively reduce bioaccumulation of arsenic in rice grains, such as rice grown aerobically, sprinkler irrigation, and genetically modified rice (Xu et al. Citation2008; Moreno-Jimenez et al. Citation2014; Chen et al. Citation2017).
In addition, several microorganisms have been isolated as they are arsenate-resistant or use arsenic to ‘breathe’, which may be used as a way of arsenic removal from water (Oremland and Stolz Citation2005; Dey et al. Citation2016). Some experimental studies have found that dietary supplements like vitamin C, different spectra of medicinal plants, and drugs can reduce arsenic toxicity through antioxidant effects, although these haven’t been assessed in humans (Bhattacharya Citation2017; Ling et al. Citation2017).
Conclusions
In summary, both experimental and epidemiological studies have demonstrated that arsenic exposure may potentiate the development of DM through dysfunctional immune responses. Arsenic exposure can adversely affect the immune system by inducing dual proinflammatory or immunosuppressive effects on both the innate and adaptive immune systems. Arsenic exposure predisposes to dysfunctional immune responses, which alters the expression and secretion of inflammatory cytokines and mediators that could possibly initiate the development of T1DM or T2DM.
The mechanisms of T1DM development due to arsenic exposure may include direct beta-cells damage, altered gut microbiome and abnormal autoimmune or inflammatory responses induced by arsenic. However, the role of arsenic in the development of T1DM needs further exploration due to lack of enough direct supportive evidence from epidemiologic, in vitro, or animal studies. For example, if arsenic exposure truly predisposes individuals to enhanced autoimmunity, associations with other autoimmune diseases, such as rheumatoid arthritis, lupus etc., might be detected in arsenic exposed populations and should be explored. Clearly, additional research needs to be done to address these concerns.
In the development of T2DM, arsenic exposure can induce the major mechanisms involved in T2DM initiation including: Inhibition of insulin-dependent glucose uptake (insulin resistance); induction of pancreatic beta-cell damage and dysfunction; stimulation of liver gluconeogenesis, among all of which the immune system is involved in the above processes. In regards to the association of multiple heavy metal exposures and DM, especially T2DM and GDM, some results are conflicting. Large prospective cohort studies of well-characterized populations, standardization across study designs, and consideration of molecular mechanisms informed by model systems studies are needed for further investigation of combined exposures of arsenic and other metals as a risk factor for DM.
The role of the immune system in DM and the association between arsenic exposure and DM have important clinical implications. First, specific molecules involved in arsenic-activated immune responses may hold the promise of potential biomarkers, and even preventive targets for arsenic-associated DM. Second, great efforts are underway to prospectively evaluate the relationship between arsenic exposure and other chronic low-grade inflammatory diseases, which are commonly associated with DM, such as atherosclerosis and stroke. Nevertheless, it is crucial to further understand the potential mechanisms of how arsenic regulates immune responses in the setting of DM. This could undoubtedly contribute to preventive approaches in the management of diabetes and define environmental risk factors for the disease. Therefore, it is imperative that further in vitro and in vivo studies are needed, in addition to epidemiologic studies to further define underserved populations that may be overly burdened with contaminants, to determine how pollutants like arsenic can induce DM or other diseases through perturbation of the immune system.
Acknowledgements
The authors greatly appreciate the assistance of Mr Paul Cacioppo in the compilation of the figures. The authors sincerely thank Dr Jun Wang for his critical review and comments on the manuscript. JL, TH and XG wrote the paper. DD and HX revised and approved the version to be published. All authors agree to be accountable for all aspects of the work.
Disclosure statement
No potential conflict of interest was reported by the author(s).
Data availability statement
No data were generated for this review manuscript.
Additional information
Funding
References
- Abdul KS, Jayasinghe SS, Chandana EP, Jayasumana C, De Silva PM. 2015. Arsenic and human health effects: a review. Environ Toxicol Pharmacol. 40(3):828–846.
- Ahmed S, Mahabbat-e Khoda S, Rekha RS, Gardner RM, Ameer SS, Moore S, Ekstrom EC, Vahter M, Raqib R. 2011. Arsenic-associated oxidative stress, inflammation, and immune disruption in human placenta and cord blood. Environ Health Perspect. 119(2):258–264.
- Alonso-Magdalena P, Quesada I, Nadal A. 2011. Endocrine disruptors in the etiology of type 2 diabetes mellitus. Nat Rev Endocrinol. 7(6):346–353.
- Ashley-Martin J, Dodds L, Arbuckle TE, Bouchard MF, Shapiro GD, Fisher M, Monnier P, Morisset AS, Ettinger AS. 2018. Association between maternal urinary speciated arsenic concentrations and gestational diabetes in a cohort of Canadian women. Environ Int. 121(Pt 1):714–720.
- ATSDR. 2007. Toxicological profile for arsenic. Atlanta, GA: U.S. Department of Health and Human Services, Public Health Services.
- Bachleitner-Hofmann T, Kees M, Gisslinger H. 2002. Arsenic trioxide: acute promyelocytic leukemia and beyond. Leuk Lymphoma. 43(8):1535–1540.
- Bahadar H, Mostafalou S, Abdollahi M. 2014. Growing burden of diabetes in Pakistan and the possible role of arsenic and pesticides. J Diabetes Metab Disord. 13(1):117.
- Bahari A, Salmani V. 2017. Environmentally relevant dose of arsenic interferes in functions of human monocytes derived dendritic cells. Toxicol Lett. 275:118–122.
- Baj G, Arnulfo A, Deaglio S, Mallone R, Vigone A, De Cesaris MG, Surico N, Malavasi F, Ferrero E. 2002. Arsenic trioxide and breast cancer: analysis of the apoptotic, differentiative and immunomodulatory effects. Breast Cancer Res Treat. 73(1):61–73.
- Ballak DB, Stienstra R, Tack CJ, Dinarello CA, van Diepen JA. 2015. IL-1 family members in the pathogenesis and treatment of metabolic disease: focus on adipose tissue inflammation and insulin resistance. Cytokine. 75(2):280–290.
- Banerjee N, Banerjee S, Sen R, Bandyopadhyay A, Sarma N, Majumder P, Das JK, Chatterjee M, Kabir SN, Giri AK. 2009. Chronic arsenic exposure impairs macrophage functions in the exposed individuals. J Clin Immunol. 29(5):582–594.
- Bellamy L, Casas JP, Hingorani AD, Williams D. 2009. Type 2 diabetes mellitus after gestational diabetes: a systematic review and meta-analysis. Lancet. 373(9677):1773–1779.
- Berbudi A, Rahmadika N, Tjahjadi AI, Ruslami R. 2020. Type 2 diabetes and its impact on the immune system. Curr Diabetes Rev. 16(5):442–449.
- Bhattacharya S. 2017. Medicinal plants and natural products in amelioration of arsenic toxicity: a short review. Pharm Biol. 55(1):349–354.
- Bishayi B, Sengupta M. 2003. Intracellular survival of Staphylococcus aureus due to alteration of cellular activity in arsenic and lead intoxicated mature Swiss albino mice. Toxicology. 184(1):31–39.
- Biswas R, Ghosh P, Banerjee N, Das JK, Sau T, Banerjee A, Roy S, Ganguly S, Chatterjee M, Mukherjee A, Giri AK. 2008. Analysis of T-cell proliferation and cytokine secretion in the individuals exposed to arsenic. Hum Exp Toxicol. 27(5):381–386.
- Bonaventura MM, Bourguignon NS, Bizzozzero M, Rodriguez D, Ventura C, Cocca C, Libertun C, Lux-Lantos VA. 2017. Arsenite in drinking water produces glucose intolerance in pregnant rats and their female offspring. Food Chem Toxicol. 100:207–216.
- Brauner EV, Nordsborg RB, Andersen ZJ, Tjonneland A, Loft S, Raaschou-Nielsen O. 2014. Long-term exposure to low-level arsenic in drinking water and diabetes incidence: a prospective study of the diet, cancer and health cohort. Environ Health Perspect. 122(10):1059–1065.
- Brown C. 2015. Arsenic exposure linked to diabetes risk in Canada. CMAJ. 187(15):E438–E439.
- Burchiel SW, Mitchell LA, Lauer FT, Sun X, McDonald JD, Hudson LG, Liu KJ. 2009. Immunotoxicity and biodistribution analysis of arsenic trioxide in C57Bl/6 mice following a 2-week inhalation exposure. Toxicol Appl Pharmacol. 241(3):253–259.
- Butalia S, Kaplan GG, Khokhar B, Rabi DM. 2016. Environmental risk factors and type 1 diabetes: past, present, and future. Can J Diabetes. 40(6):586–593.
- Cardenas A, Smit E, Welch BM, Bethel J, Kile ML. 2018. Cross sectional association of arsenic and seroprevalence of hepatitis B infection in the United States (NHANES 2003–2014). Environ Res. 166:570–576.
- Castriota F, Acevedo J, Ferreccio C, Smith AH, Liaw J, Smith MT, Steinmaus C. 2018. Obesity and increased susceptibility to arsenic-related type 2 diabetes in Northern Chile. Environ Res. 167:248–254.
- CDC. 2022. National diabetes statistics report: estimates of diabetes and its burden in the United States. Centers for Disease Control and Prevention.
- Chakraborty D, Mukherjee A, Sikdar S, Paul A, Ghosh S, Khuda-Bukhsh AR. 2012. [6]-Gingerol isolated from ginger attenuates sodium arsenite induced oxidative stress and plays a corrective role in improving insulin signaling in mice. Toxicol Lett. 210(1):34–43.
- Chang D, Xu H, Rebaza A, Sharma L, Dela Cruz CS. 2020. Protecting health-care workers from subclinical coronavirus infection. Lancet Respir Med. 8(3):e13.
- Chen G, Mao J, Zhao J, Zhang Y, Li T, Wang C, Xu L, Hu Q, Wang X, Jiang S, et al. 2016. Arsenic trioxide mediates HAPI microglia inflammatory response and the secretion of inflammatory cytokine IL-6 via Akt/NF-kappaB signaling pathway. Regul Toxicol Pharmacol. 81:480–488.
- Chen Y, Han YH, Cao Y, Zhu YG, Rathinasabapathi B, Ma LQ. 2017. Arsenic transport in rice and biological solutions to reduce arsenic risk from rice. Front Plant Sci. 8:268.
- Chen YW, Yang CY, Huang CF, Hung DZ, Leung YM, Liu SH. 2009. Heavy metals, islet function and diabetes development. Islets. 1(3):169–176.
- Clark M, Kroger CJ, Tisch RM. 2017. Type 1 diabetes: a chronic anti-self-inflammatory response. Front Immunol. 8:1898.
- Corsini E, Asti L, Viviani B, Marinovich M, Galli CL. 1999. Sodium arsenate induces overproduction of interleukin-1alpha in murine keratinocytes: role of mitochondria. J Invest Dermatol. 113(5):760–765.
- Currier JM, Ishida MC, Gonzalez-Horta C, Sanchez-Ramirez B, Ballinas-Casarrubias L, Gutierrez-Torres DS, Ceron RH, Morales DV, Terrazas FA, Del Razo LM, et al. 2014. Associations between arsenic species in exfoliated urothelial cells and prevalence of diabetes among residents of Chihuahua, Mexico. Environ Health Perspect. 122(10):1088–1094.
- Dabelea D, Mayer-Davis EJ, Lamichhane AP, D'Agostino, Jr. RB, Liese AD, Vehik KS, Narayan KM, Zeitler P, Hamman RF. 2008. Association of intrauterine exposure to maternal diabetes and obesity with type 2 diabetes in youth: the SEARCH Case-Control Study. Diabetes Care. 31(7):1422–1426.
- Dangleben NL, Skibola CF, Smith MT. 2013. Arsenic immunotoxicity: a review. Environ Health. 12(1):73.
- Das D, Bindhani B, Mukherjee B, Saha H, Biswas P, Dutta K, Prasad P, Sinha D, Ray MR. 2014. Chronic low-level arsenic exposure reduces lung function in male population without skin lesions. Int J Public Health. 59(4):655–663.
- Das N, Paul S, Chatterjee D, Banerjee N, Majumder NS, Sarma N, Sau TJ, Basu S, Banerjee S, Majumder P, et al. 2012. Arsenic exposure through drinking water increases the risk of liver and cardiovascular diseases in the population of West Bengal, India. BMC Public Health. 12:639.
- da Silva Rosa SC, Nayak N, Caymo AM, Gordon JW. 2020. Mechanisms of muscle insulin resistance and the cross-talk with liver and adipose tissue. Physiol Rep. 8(19):e14607.
- Dasu MR, Devaraj S, Jialal I. 2007. High glucose induces IL-1beta expression in human monocytes: mechanistic insights. Am J Physiol Endocrinol Metab. 293(1):E337–E346.
- Dasu MR, Devaraj S, Zhao L, Hwang DH, Jialal I. 2008. High glucose induces toll-like receptor expression in human monocytes: mechanism of activation. Diabetes. 57(11):3090–3098.
- de Goffau MC, Fuentes S, van den Bogert B, Honkanen H, de Vos WM, Welling GW, Hyoty H, Harmsen HJ. 2014. Aberrant gut microbiota composition at the onset of type 1 diabetes in young children. Diabetologia. 57(8):1569–1577.
- Dey U, Chatterjee S, Mondal NK. 2016. Isolation and characterization of arsenic-resistant bacteria and possible application in bioremediation. Biotechnol Rep. 10:1–7.
- Diaz-Villasenor A, Burns AL, Salazar AM, Sordo M, Hiriart M, Cebrian ME, Ostrosky-Wegman P. 2008. Arsenite reduces insulin secretion in rat pancreatic beta-cells by decreasing the calcium-dependent calpain-10 proteolysis of SNAP-25. Toxicol Appl Pharmacol. 231(3):291–299.
- Diaz-Villasenor A, Hiriart M, Cebrian ME, Zacarias-Castillo R, Ostrosky-Wegman P. 2008. The activity of calpains in lymphocytes is glucose-dependent and is decreased in diabetic patients. Blood Cells Mol Dis. 40(3):414–419.
- Diaz-Villasenor A, Sanchez-Soto MC, Cebrian ME, Ostrosky-Wegman P, Hiriart M. 2006. Sodium arsenite impairs insulin secretion and transcription in pancreatic beta-cells. Toxicol Appl Pharmacol. 214(1):30–34.
- Dong X, Shulzhenko N, Lemaitre J, Greer RL, Peremyslova K, Quamruzzaman Q, Rahman M, Hasan OS, Joya SA, Golam M, et al. 2017. Arsenic exposure and intestinal microbiota in children from Sirajdikhan, Bangladesh. PLoS One. 12(12):e0188487.
- Douillet C, Currier J, Saunders J, Bodnar WM, Matousek T, Styblo M. 2013. Methylated trivalent arsenicals are potent inhibitors of glucose stimulated insulin secretion by murine pancreatic islets. Toxicol Appl Pharmacol. 267(1):11–15.
- Duan X, Li J, Zhang Y, Li W, Zhao L, Nie H, Sun G, Li B. 2015. Activation of NRF2 pathway in spleen, thymus as well as peripheral blood mononuclear cells by acute arsenic exposure in mice. Int Immunopharmacol. 28(2):1059–1067.
- Dutta K, Prasad P, Sinha D. 2015. Chronic low level arsenic exposure evokes inflammatory responses and DNA damage. Int J Hyg Environ Health. 218(6):564–574.
- Escobar J, Varela-Nallar L, Coddou C, Nelson P, Maisey K, Valdes D, Aspee A, Espinosa V, Rozas C, Montoya M, et al. 2010. Oxidative damage in lymphocytes of copper smelter workers correlated to higher levels of excreted arsenic. Mediators Inflamm. 2010:403830.
- Evangelou E, Ntritsos G, Chondrogiorgi M, Kavvoura FK, Hernandez AF, Ntzani EE, Tzoulaki I. 2016. Exposure to pesticides and diabetes: a systematic review and meta-analysis. Environ Int. 91:60–68.
- Farzan SF, Gossai A, Chen Y, Chasan-Taber L, Baker E, Karagas M. 2016. Maternal arsenic exposure and gestational diabetes and glucose intolerance in the New Hampshire birth cohort study. Environ Health. 15(1):106.
- Farzan SF, Li Z, Korrick SA, Spiegelman D, Enelow R, Nadeau K, Baker E, Karagas MR. 2016. Infant infections and respiratory symptoms in relation to in utero arsenic exposure in a U.S. cohort. Environ Health Perspect. 124(6):840–847.
- Fatima N, Faisal SM, Zubair S, Ajmal M, Siddiqui SS, Moin S, Owais M. 2016. Role of pro-inflammatory cytokines and biochemical markers in the pathogenesis of type 1 diabetes: correlation with age and glycemic condition in diabetic human subjects. PLoS One. 11(8):e0161548.
- Ferrara F, Molica M, Bernardi M. 2021. Drug treatment options for acute promyelocytic leukemia. Expert Opin Pharmacother. 23:117–127.
- Fortier H, Gies V, Variola F, Wang C, Zou S. 2020. Probing arsenic trioxide (ATO) treated leukemia cell elasticities using atomic force microscopy. Anal Methods. 12(39):4734–4741.
- Friedman JE, Yun JS, Patel YM, McGrane MM, Hanson RW. 1993. Glucocorticoids regulate the induction of phosphoenolpyruvate carboxykinase (GTP) gene transcription during diabetes. J Biol Chem. 268(17):12952–12957.
- Fu J, Woods CG, Yehuda-Shnaidman E, Zhang Q, Wong V, Collins S, Sun G, Andersen ME, Pi J. 2010. Low-level arsenic impairs glucose-stimulated insulin secretion in pancreatic beta cells: involvement of cellular adaptive response to oxidative stress. Environ Health Perspect. 118(6):864–870.
- Goel A, Spitz DR, Weiner GJ. 2012. Manipulation of cellular redox parameters for improving therapeutic responses in B-cell lymphoma and multiple myeloma. J Cell Biochem. 113(2):419–425.
- Goldman RH. 2020. Arsenic exposure and poisoning. https://www.uptodate.com/contents/arsenic-exposure-and-poisoning.
- Grandl G, Wolfrum C. 2017. Hemostasis, endothelial stress, inflammation, and the metabolic syndrome. Semin Immunopathol. 40:215–224.
- Grau-Perez M, Kuo CC, Spratlen M, Thayer KA, Mendez MA, Hamman RF, Dabelea D, Adgate JL, Knowler WC, Bell RA, et al. 2017. The association of arsenic exposure and metabolism with type 1 and type 2 diabetes in youth: the SEARCH Case-Control Study. Diabetes Care. 40(1):46–53.
- Grunnet LG, Mandrup-Poulsen T. 2011. Cytokines and type 1 diabetes: a numbers game. Diabetes. 60(3):697–699.
- Guan WJ, Liang WH, Zhao Y, Liang HR, Chen ZS, Li YM, Liu XQ, Chen RC, Tang CL, Wang T, et al. 2020. Comorbidity and its impact on 1590 patients with COVID-19 in China: a nationwide analysis. Eur Respir J. 55(5):2000547.
- Guan WJ, Ni ZY, Hu Y, Liang WH, Ou CQ, He JX, Liu L, Shan H, Lei CL, Hui DSC, et al. 2020. Clinical characteristics of coronavirus disease 2019 in China. N Engl J Med. 382(18):1708–1720.
- Guo W, Li M, Dong Y, Zhou H, Zhang Z, Tian C, Qin R, Wang H, Shen Y, Du K, et al. 2020. Diabetes is a risk factor for the progression and prognosis of COVID-19. Diabetes Metab Res Rev. 36:3319.
- Hamilton JW, Kaltreider RC, Bajenova OV, Ihnat MA, McCaffrey J, Turpie BW, Rowell EE, Oh J, Nemeth MJ, Pesce CA, Lariviere JP. 1998. Molecular basis for effects of carcinogenic heavy metals on inducible gene expression. Environ Health Perspect. 106(Suppl 4):1005–1015.
- Harrison MT, McCoy KL. 2001. Immunosuppression by arsenic: a comparison of cathepsin L inhibition and apoptosis. Int Immunopharmacol. 1(4):647–656.
- Huang CF, Yang CY, Chan DC, Wang CC, Huang KH, Wu CC, Tsai KS, Yang RS, Liu SH. 2015. Arsenic exposure and glucose intolerance/insulin resistance in estrogen-deficient female mice. Environ Health Perspect. 123(11):1138–1144.
- Huang JW, Cheng YY, Sung TC, Guo HR, Sthiannopkao S. 2014. Association between arsenic exposure and diabetes mellitus in Cambodia. Biomed Res Int. 2014:683124.
- Izquierdo-Vega JA, Soto CA, Sanchez-Pena LC, De Vizcaya-Ruiz A, Del Razo LM. 2006. Diabetogenic effects and pancreatic oxidative damage in rats subchronically exposed to arsenite. Toxicol Lett. 160(2):135–142.
- Javaid A, Akbar I, Javed H, Khan U, Iftikhar H, Zahra D, Rashid F, Ashfaq UA. 2021. Role of heavy metals in diabetes: mechanisms and treatment strategies. Crit Rev Eukaryot Gene Expr. 31(3):65–80.
- Jo JH, Kang H, Lee HS, Chung MJ, Park JY, Bang S, Park SW, Song SY. 2019. KML001, an arsenic compound, as salvage chemotherapy in refractory biliary tract cancers: a prospective study. Hepatobiliary Pancreat Dis Int. 18(1):62–66.
- Jomova K, Jenisova Z, Feszterova M, Baros S, Liska J, Hudecova D, Rhodes CJ, Valko M. 2011. Arsenic: toxicity, oxidative stress and human disease. J Appl Toxicol. 31(2):95–107.
- Kaleem A, Javed S, Rehman N, Abdullah R, Iqtedar M, Aftab MN, Hoessli DC, Haq IU. 2021. Phosphorylated and O-GlcNAc modified IRS-1 (Ser1101) and -2 (Ser1149) contribute to human diabetes type II. Protein Pept Lett. 28(3):333–339.
- Kaushal A, Zhang H, Karmaus WJJ, Everson TM, Marsit CJ, Karagas MR, Tsai SF, Wen HJ, Wang SL. 2017. Genome-wide DNA methylation at birth in relation to in utero arsenic exposure and the associated health in later life. Environ Health. 16(1):50.
- Kelstrup L, Damm P, Mathiesen ER, Hansen T, Vaag AA, Pedersen O, Clausen TD. 2013. Insulin resistance and impaired pancreatic beta-cell function in adult offspring of women with diabetes in pregnancy. J Clin Endocrinol Metab. 98(9):3793–3801.
- Kesavan M, Sarath TS, Kannan K, Suresh S, Gupta P, Vijayakaran K, Sankar P, Kurade NP, Mishra SK, Sarkar SN. 2014. Atorvastatin restores arsenic-induced vascular dysfunction in rats: modulation of nitric oxide signaling and inflammatory mediators. Toxicol Appl Pharmacol. 280(1):107–116.
- Khurana A, Tekula S, Godugu C. 2018. Nanoceria suppresses multiple low doses of streptozotocin-induced type 1 diabetes by inhibition of Nrf2/NF-kappaB pathway and reduction of apoptosis. Nanomedicine. 13(15):1905–1922.
- Koenen TB, Stienstra R, van Tits LJ, de Graaf J, Stalenhoef AF, Joosten LA, Tack CJ, Netea MG. 2011. Hyperglycemia activates caspase-1 and TXNIP-mediated IL-1beta transcription in human adipose tissue. Diabetes. 60(2):517–524.
- Kozul CD, Ely KH, Enelow RI, Hamilton JW. 2009. Low-dose arsenic compromises the immune response to influenza A infection in vivo. Environ Health Perspect. 117(9):1441–1447.
- Kritharis A, Bradley TP, Budman DR. 2013. The evolving use of arsenic in pharmacotherapy of malignant disease. Ann Hematol. 92(6):719–730.
- Krupoff M, Mobarak AM, van Geen A. 2020. Evaluating strategies to reduce arsenic poisoning in South Asia: a view from the social sciences. Asian Dev Rev. 37(2):21–44.
- Lagathu C, Bastard JP, Auclair M, Maachi M, Capeau J, Caron M. 2003. Chronic interleukin-6 (IL-6) treatment increased IL-6 secretion and induced insulin resistance in adipocyte: prevention by rosiglitazone. Biochem Biophys Res Commun. 311(2):372–379.
- Lai PY, Cottingham KL, Steinmaus C, Karagas MR, Miller MD. 2015. Arsenic and rice: translating research to address health care providers’ needs. J Pediatr. 167(4):797–803.
- Lang M, Wang X, Wang H, Dong J, Lan C, Hao J, Huang C, Li X, Yu M, Yang Y, et al. 2016. Arsenic trioxide plus PX-478 achieves effective treatment in pancreatic ductal adenocarcinoma. Cancer Lett. 378(2):87–96.
- Lemarie A, Morzadec C, Bourdonnay E, Fardel O, Vernhet L. 2006. Human macrophages constitute targets for immunotoxic inorganic arsenic. J Immunol. 177(5):3019–3027.
- Le Roith D, Zick Y. 2001. Recent advances in our understanding of insulin action and insulin resistance. Diabetes Care. 24(3):588–597.
- Li C, Xu J, Li F, Chaudhary SC, Weng Z, Wen J, Elmets CA, Ahsan H, Athar M. 2011. Unfolded protein response signaling and MAP kinase pathways underlie pathogenesis of arsenic-induced cutaneous inflammation. Cancer Prev Res. 4(12):2101–2109.
- Li D, Yang Y, Li Y, Li Z, Zhu X, Zeng X. 2021. Changes induced by chronic exposure to high arsenic concentrations in the intestine and its microenvironment. Toxicology. 456:152767.
- Li J, Guo Y, Duan X, Li B. 2021. Heme oxygenase-1 (HO-1) assists inorganic arsenic-induced immune tolerance in murine dendritic cells. Chemosphere. 264(Pt 2):128452.
- Li JJ, Tang Q, Li Y, Hu BR, Ming ZY, Fu Q, Qian JQ, Xiang JZ. 2006. Role of oxidative stress in the apoptosis of hepatocellular carcinoma induced by combination of arsenic trioxide and ascorbic acid. Acta Pharmacol Sin. 27(8):1078–1084.
- Li S, Eguchi N, Lau H, Ichii H. 2020. The role of the Nrf2 signaling in obesity and insulin resistance. Int J Mol Sci. 21(18):6973.
- Ling S, Shan Q, Liu P, Feng T, Zhang X, Xiang P, Chen K, Xie H, Song P, Zhou L, et al. 2017. Metformin ameliorates arsenic trioxide hepatotoxicity via inhibiting mitochondrial complex I. Cell Death Dis. 8(11):e3159.
- Liu S, Guo X, Wu B, Yu H, Zhang X, Li M. 2014. Arsenic induces diabetic effects through beta-cell dysfunction and increased gluconeogenesis in mice. Sci Rep. 4:6894.
- Liu S, Sun Q, Wang F, Zhang L, Song Y, Xi S, Sun G. 2014. Arsenic induced overexpression of inflammatory cytokines based on the human urothelial cell model in vitro and urinary secretion of individuals chronically exposed to arsenic. Chem Res Toxicol. 27(11):1934–1942.
- Liu Y, Zhang W, Zhang X, Qi Y, Huang D, Zhang Y. 2011. Arsenic trioxide inhibits invasion/migration in SGC-7901 cells by activating the reactive oxygen species-dependent cyclooxygenase-2/matrix metalloproteinase-2 pathway. Exp Biol Med. 236(5):592–597.
- Lu K, Abo RP, Schlieper KA, Graffam ME, Levine S, Wishnok JS, Swenberg JA, Tannenbaum SR, Fox JG. 2014. Arsenic exposure perturbs the gut microbiome and its metabolic profile in mice: an integrated metagenomics and metabolomics analysis. Environ Health Perspect. 122(3):284–291.
- Lu TH, Su CC, Chen YW, Yang CY, Wu CC, Hung DZ, Chen CH, Cheng PW, Liu SH, Huang CF. 2011. Arsenic induces pancreatic beta-cell apoptosis via the oxidative stress-regulated mitochondria-dependent and endoplasmic reticulum stress-triggered signaling pathways. Toxicol Lett. 201(1):15–26.
- Lu X, Luo F, Liu Y, Zhang A, Li J, Wang B, Xu W, Shi L, Liu X, Lu L, Liu Q. 2015. The IL-6/STAT3 pathway via miR-21 is involved in the neoplastic and metastatic properties of arsenite-transformed human keratinocytes. Toxicol Lett. 237(3):191–199.
- Luo F, Xu Y, Ling M, Zhao Y, Xu W, Liang X, Jiang R, Wang B, Bian Q, Liu Q. 2013. Arsenite evokes IL-6 secretion, autocrine regulation of STAT3 signaling, and miR-21 expression, processes involved in the EMT and malignant transformation of human bronchial epithelial cells. Toxicol Appl Pharmacol. 273(1):27–34.
- Macoch M, Morzadec C, Fardel O, Vernhet L. 2013. Inorganic arsenic impairs differentiation and functions of human dendritic cells. Toxicol Appl Pharmacol. 266(2):204–213.
- Macoch M, Morzadec C, Genard R, Pallardy M, Kerdine-Romer S, Fardel O, Vernhet L. 2015. Nrf2-dependent repression of interleukin-12 expression in human dendritic cells exposed to inorganic arsenic. Free Radic Biol Med. 88(Pt B):381–390.
- Martin E, Gonzalez-Horta C, Rager J, Bailey KA, Sanchez-Ramirez B, Ballinas-Casarrubias L, Ishida MC, Gutierrez-Torres DS, Hernandez Ceron R, Viniegra Morales D, et al. 2015. Metabolomic characteristics of arsenic-associated diabetes in a prospective cohort in Chihuahua, Mexico. Toxicol Sci. 144(2):338–346.
- Martin EM, Styblo M, Fry RC. 2017. Genetic and epigenetic mechanisms underlying arsenic-associated diabetes mellitus: a perspective of the current evidence. Epigenomics. 9(5):701–710.
- Martin-Chouly C, Morzadec C, Bonvalet M, Galibert MD, Fardel O, Vernhet L. 2011. Inorganic arsenic alters expression of immune and stress response genes in activated primary human T lymphocytes. Mol Immunol. 48(6-7):956–965.
- Martinez-Castillo M, Garcia-Montalvo EA, Arellano-Mendoza MG, Sanchez-Pena LDC, Soria Jasso LE, Izquierdo-Vega JA, Valenzuela OL, Hernandez-Zavala A. 2021. Arsenic exposure and non-carcinogenic health effects. Hum Exp Toxicol. 40(12_suppl):S826–S850.
- Maull EA, Ahsan H, Edwards J, Longnecker MP, Navas-Acien A, Pi J, Silbergeld EK, Styblo M, Tseng CH, Thayer KA, Loomis D. 2012. Evaluation of the association between arsenic and diabetes: a national toxicology program workshop review. Environ Health Perspect. 120(12):1658–1670.
- Mazumder DN, Haque R, Ghosh N, De BK, Santra A, Chakraborti D, Smith AH. 2000. Arsenic in drinking water and the prevalence of respiratory effects in West Bengal, India. Int J Epidemiol. 29(6):1047–1052.
- Medina S, Lauer FT, Castillo EF, Bolt AM, Ali AS, Liu KJ, Burchiel SW. 2020. Exposures to uranium and arsenic alter intraepithelial and innate immune cells in the small intestine of male and female mice. Toxicol Appl Pharmacol. 403:115155.
- Meng ZQ, Meng NY. 2000. Effects of arsenic on blast transformation and DNA synthesis of human blood lymphocytes. Chemosphere. 41(1–2):115–119.
- Miller MD, Marty MA, Arcus A, Brown J, Morry D, Sandy M. 2002. Differences between children and adults: implications for risk assessment at California EPA. Int J Toxicol. 21(5):403–418.
- Moreno-Jimenez E, Meharg AA, Smolders E, Manzano R, Becerra D, Sanchez-Llerena J, Albarran A, Lopez-Pinero A. 2014. Sprinkler irrigation of rice fields reduces grain arsenic but enhances cadmium. Sci Total Environ. 485–486:468–473.
- Muniyappa R, Gubbi S. 2020. COVID-19 pandemic, coronaviruses, and diabetes mellitus. Am J Physiol Endocrinol Metab. 318(5):E736–E741.
- Muoio DM, Newgard CB. 2008. Mechanisms of disease: molecular and metabolic mechanisms of insulin resistance and beta-cell failure in type 2 diabetes. Nat Rev Mol Cell Biol. 9(3):193–205.
- Nadeau KC, Li Z, Farzan S, Koestler D, Robbins D, Fei DL, Malipatlolla M, Maecker H, Enelow R, Korrick S, Karagas MR. 2014. In utero arsenic exposure and fetal immune repertoire in a US pregnancy cohort. Clin Immunol. 155(2):188–197.
- Navas-Acien A, Silbergeld EK, Pastor-Barriuso R, Guallar E. 2008. Arsenic exposure and prevalence of type 2 diabetes in US adults. JAMA. 300(7):814–822.
- Onder G, Rezza G, Brusaferro S. 2020. Case-fatality rate and characteristics of patients dying in relation to COVID-19 in Italy. JAMA. 323(18):1775–1776.
- Oremland RS, Stolz JF. 2005. Arsenic, microbes and contaminated aquifers. Trends Microbiol. 13(2):45–49.
- Padmaja Divya S, Pratheeshkumar P, Son YO, Vinod Roy R, Andrew Hitron J, Kim D, Dai J, Wang L, Asha P, Huang B, et al. 2015. Arsenic induces insulin resistance in mouse adipocytes and myotubes via oxidative stress-regulated mitochondrial Sirt3-FOXO3a signaling pathway. Toxicol Sci. 146(2):290–300.
- Pal D, Dasgupta S, Kundu R, Maitra S, Das G, Mukhopadhyay S, Ray S, Majumdar SS, Bhattacharya S. 2012. Fetuin-A acts as an endogenous ligand of TLR4 to promote lipid-induced insulin resistance. Nat Med. 18(8):1279–1285.
- Pal R, Bhadada SK. 2020. COVID-19 and diabetes mellitus: an unholy interaction of two pandemics. Diabetes Metab Syndr. 14(4):513–517.
- Pan X, Jiang L, Zhong L, Geng C, Jia L, Liu S, Guan H, Yang G, Yao X, Piao F, Sun X. 2016. Arsenic induces apoptosis by the lysosomal-mitochondrial pathway in INS-1 cells. Environ Toxicol. 31(2):133–141.
- Paret C, Russo A, Otto H, Mayer A, Zahnreich S, Wagner W, Samuel D, Scharnhorst D, Solomon DA, Dhall G, et al. 2017. Personalized therapy: CNS HGNET-BCOR responsiveness to arsenic trioxide combined with radiotherapy. Oncotarget. 8(69):114210–114225.
- Parvez F, Akhtar E, Khan L, Haq MA, Islam T, Ahmed D, Eunus HM, Hasan AR, Ahsan H, Graziano JH, Raqib R. 2019. Exposure to low-dose arsenic in early life alters innate immune function in children. J Immunotoxicol. 16(1):201–209.
- Paul DS, Devesa V, Hernandez-Zavala A, Adair BM, Walton FS, Drobna Z, Thomas DJ, Styblo M. 2008. Environmental arsenic as a disruptor of insulin signaling. Met Ions Biol Med. 10:1–7.
- Paul DS, Harmon AW, Devesa V, Thomas DJ, Styblo M. 2007. Molecular mechanisms of the diabetogenic effects of arsenic: inhibition of insulin signaling by arsenite and methylarsonous acid. Environ Health Perspect. 115(5):734–742.
- Paul SK, Islam MS, Hasibuzzaman MM, Hossain F, Anjum A, Saud ZA, Haque MM, Sultana P, Haque A, Andric KB, et al. 2019. Higher risk of hyperglycemia with greater susceptibility in females in chronic arsenic-exposed individuals in Bangladesh. Sci Total Environ. 668:1004–1012.
- Pellegrini S, Sordi V, Bolla AM, Saita D, Ferrarese R, Canducci F, Clementi M, Invernizzi F, Mariani A, Bonfanti R, et al. 2017. Duodenal mucosa of patients with type 1 diabetes shows distinctive inflammatory profile and microbiota. J Clin Endocrinol Metab. 102(5):1468–1477.
- Peng S, Liu L, Zhang X, Heinrich J, Zhang J, Schramm KW, Huang Q, Tian M, Eqani SA, Shen H. 2015. A nested case-control study indicating heavy metal residues in meconium associate with maternal gestational diabetes mellitus risk. Environ Health. 14:19.
- Pi J, Zhang Q, Fu J, Woods CG, Hou Y, Corkey BE, Collins S, Andersen ME. 2010. ROS signaling, oxidative stress and Nrf2 in pancreatic beta-cell function. Toxicol Appl Pharmacol. 244(1):77–83.
- Prajapati V, Kale RK, Singh RP. 2011. Arsenic and its combinations in cancer therapeutics. Ther Deliv. 2(6):793–806.
- Prasad P, Sinha D. 2017. Low-level arsenic causes chronic inflammation and suppresses expression of phagocytic receptors. Environ Sci Pollut Res Int. 24(12):11708–11721.
- Rager JE, Bailey KA, Smeester L, Miller SK, Parker JS, Laine JE, Drobna Z, Currier J, Douillet C, Olshan AF, et al. 2014. Prenatal arsenic exposure and the epigenome: altered microRNAs associated with innate and adaptive immune signaling in newborn cord blood. Environ Mol Mutagen. 55(3):196–208.
- Rahman A, Vahter M, Ekstrom EC, Persson LA. 2011. Arsenic exposure in pregnancy increases the risk of lower respiratory tract infection and diarrhea during infancy in Bangladesh. Environ Health Perspect. 119(5):719–724.
- Rahman M, Axelson O. 1995. Diabetes mellitus and arsenic exposure: a second look at case-control data from a Swedish copper smelter. Occup Environ Med. 52(11):773–774.
- Rahman M, Wingren G, Axelson O. 1996. Diabetes mellitus among Swedish art glass workers – an effect of arsenic exposure? Scand J Work Environ Health. 22(2):146–149.
- Ramsey KA, Bosco A, McKenna KL, Carter KW, Elliot JG, Berry LJ, Sly PD, Larcombe AN, Zosky GR. 2013. In utero exposure to arsenic alters lung development and genes related to immune and mucociliary function in mice. Environ Health Perspect. 121(2):244–250.
- Ramsey KA, Foong RE, Sly PD, Larcombe AN, Zosky GR. 2013. Early life arsenic exposure and acute and long-term responses to influenza A infection in mice. Environ Health Perspect. 121(10):1187–1193.
- Raqib R, Ahmed S, Sultana R, Wagatsuma Y, Mondal D, Hoque AM, Nermell B, Yunus M, Roy S, Persson LA, et al. 2009. Effects of in utero arsenic exposure on child immunity and morbidity in rural Bangladesh. Toxicol Lett. 185(3):197–202.
- Rewers M, Ludvigsson J. 2016. Environmental risk factors for type 1 diabetes. Lancet. 387(10035):2340–2348.
- Rojewski MT, Korper S, Schrezenmeier H. 2004. Arsenic trioxide therapy in acute promyelocytic leukemia and beyond: from bench to bedside. Leuk Lymphoma. 45(12):2387–2401.
- Rotter V, Nagaev I, Smith U. 2003. Interleukin-6 (IL-6) induces insulin resistance in 3T3-L1 adipocytes and is, like IL-8 and tumor necrosis factor-alpha, overexpressed in human fat cells from insulin-resistant subjects. J Biol Chem. 278(46):45777–45784.
- Salmeri N, Villanacci R, Ottolina J, Bartiromo L, Cavoretto P, Dolci C, Lembo R, Schimberni M, Valsecchi L, Vigano P, Candiani M. 2020. Maternal arsenic exposure and gestational diabetes: a systematic review and meta-analysis. Nutrients. 12(10):3094.
- Sanyal T, Bhattacharjee P, Paul S, Bhattacharjee P. 2020. Recent advances in arsenic research: significance of differential susceptibility and sustainable strategies for mitigation. Front Public Health. 8:464.
- Sanyaolu A, Okorie C, Marinkovic A, Patidar R, Younis K, Desai P, Hosein Z, Padda I, Mangat J, Altaf M. 2020. Comorbidity and its impact on patients with COVID-19. SN Compr Clin Med. 2(8):1069–1076.
- Savabieasfahani M, Lochmiller RL, Rafferty DP, Sinclair JA. 1998. Sensitivity of wild cotton rats (Sigmodon hispidus) to the immunotoxic effects of low-level arsenic exposure. Arch Environ Contam Toxicol. 34(3):289–296.
- Shahid M, Niazi NK, Dumat C, Naidu R, Khalid S, Rahman MM, Bibi I. 2018. A meta-analysis of the distribution, sources and health risks of arsenic-contaminated groundwater in Pakistan. Environ Pollut. 242(Pt A):307–319.
- Shu CJ, Benoist C, Mathis D. 2012. The immune system's involvement in obesity-driven type 2 diabetes. Semin Immunol. 24(6):436–442.
- Sinha D, Mukherjee B, Bindhani B, Dutta K, Saha H, Prasad P, Ray MR. 2014. Chronic low level arsenic exposure inflicts pulmonary and systemic inflammation. J Cancer Sci Ther. 6(3):62–69.
- Smedley PL, Kinniburgh DG. 2002. A review of the source, behaviour and distribution of arsenic in natural waters. Appl Geochem. 17(5):517–568.
- Smith AH, Marshall G, Yuan Y, Ferreccio C, Liaw J, von Ehrenstein O, Steinmaus C, Bates MN, Selvin S. 2006. Increased mortality from lung cancer and bronchiectasis in young adults after exposure to arsenic in utero and in early childhood. Environ Health Perspect. 114(8):1293–1296.
- Smith AH, Marshall G, Yuan Y, Liaw J, Ferreccio C, Steinmaus C. 2011. Evidence from Chile that arsenic in drinking water may increase mortality from pulmonary tuberculosis. Am J Epidemiol. 173(4):414–420.
- Soto-Pena GA, Luna AL, Acosta-Saavedra L, Conde P, Lopez-Carrillo L, Cebrian ME, Bastida M, Calderon-Aranda ES, Vega L. 2006. Assessment of lymphocyte subpopulations and cytokine secretion in children exposed to arsenic. FASEB J. 20(6):779–781.
- Soto-Pena GA, Vega L. 2008. Arsenic interferes with the signaling transduction pathway of T cell receptor activation by increasing basal and induced phosphorylation of Lck and Fyn in spleen cells. Toxicol Appl Pharmacol. 230(2):216–226.
- Srivastava S, Vladykovskaya EN, Haberzettl P, Sithu SD, D'Souza SE, States JC. 2009. Arsenic exacerbates atherosclerotic lesion formation and inflammation in ApoE-/-mice. Toxicol Appl Pharmacol. 241(1):90–100.
- States JC, Singh AV, Knudsen TB, Rouchka EC, Ngalame NO, Arteel GE, Piao Y, Ko MS. 2012. Prenatal arsenic exposure alters gene expression in the adult liver to a proinflammatory state contributing to accelerated atherosclerosis. PLoS One. 7(6):e38713.
- Straif K, Benbrahim-Tallaa L, Baan R, Grosse Y, Secretan B, El Ghissassi F, Bouvard V, Guha N, Freeman C, Galichet L, Cogliano V. 2009. A review of human carcinogens–part C: metals, arsenic, dusts, and fibres. Lancet Oncol. 10(5):453–454.
- Straub AC, Stolz DB, Vin H, Ross MA, Soucy NV, Klei LR, Barchowsky A. 2007. Low level arsenic promotes progressive inflammatory angiogenesis and liver blood vessel remodeling in mice. Toxicol Appl Pharmacol. 222(3):327–336.
- Sultan SA, Liu W, Peng Y, Roberts W, Whitelaw D, Graham AM. 2015. The role of maternal gestational diabetes in inducing fetal endothelial dysfunction. J Cell Physiol. 230(11):2695–2705.
- Sung TC, Huang JW, Guo HR. 2015. Association between arsenic exposure and diabetes: a meta-analysis. Biomed Res Int. 2015:368087.
- Tsai TL, Lei WT, Kuo CC, Sun HL, Su PH, Wang SL. 2021. Maternal and childhood exposure to inorganic arsenic and airway allergy – a 15-year birth cohort follow-up study. Environ Int. 146:106243.
- Tseng CH. 2004. The potential biological mechanisms of arsenic-induced diabetes mellitus. Toxicol Appl Pharmacol. 197(2):67–83.
- Vatanen T, Franzosa EA, Schwager R, Tripathi S, Arthur TD, Vehik K, Lernmark A, Hagopian WA, Rewers MJ, She JX, et al. 2018. The human gut microbiome in early-onset type 1 diabetes from the TEDDY study. Nature. 562(7728):589–594.
- Walton FS, Harmon AW, Paul DS, Drobna Z, Patel YM, Styblo M. 2004. Inhibition of insulin-dependent glucose uptake by trivalent arsenicals: possible mechanism of arsenic-induced diabetes. Toxicol Appl Pharmacol. 198(3):424–433.
- Wang W, Xie Z, Lin Y, Zhang D. 2014. Association of inorganic arsenic exposure with type 2 diabetes mellitus: a meta-analysis. J Epidemiol Community Health. 68(2):176–184.
- Wang X, Gao D, Zhang G, Zhang X, Li Q, Gao Q, Chen R, Xu S, Huang L, Zhang Y, et al. 2020. Exposure to multiple metals in early pregnancy and gestational diabetes mellitus: a prospective cohort study. Environ Int. 135:105370.
- Wang Y, Zhang P, Chen X, Wu W, Feng Y, Yang H, Li M, Xie B, Guo P, Warren JL, et al. 2019. Multiple metal concentrations and gestational diabetes mellitus in Taiyuan, China. Chemosphere. 237:124412.
- WHO. 2006. Arsenic mitigation for safe groundwater.
- WHO. 2022. Diabetes. World Health Organization.
- Wu MM, Chiou HY, Ho IC, Chen CJ, Lee TC. 2003. Gene expression of inflammatory molecules in circulating lymphocytes from arsenic-exposed human subjects. Environ Health Perspect. 111(11):1429–1438.
- Wu W, Yao X, Jiang L, Zhang Q, Bai J, Qiu T, Yang L, Gao N, Yang G, Liu X, et al. 2018. Pancreatic islet-autonomous effect of arsenic on insulin secretion through endoplasmic reticulum stress-autophagy pathway. Food Chem Toxicol. 111:19–26.
- Xu H, Wang X, Wang W. 2018. Functional suppression of macrophages derived from THP-1 cells by environmentally-relevant concentrations of arsenite. Comp Biochem Physiol C Toxicol Pharmacol. 214:36–42.
- Xu XY, McGrath SP, Meharg AA, Zhao FJ. 2008. Growing rice aerobically markedly decreases arsenic accumulation. Environ Sci Technol. 42(15):5574–5579.
- Xue P, Hou Y, Zhang Q, Woods CG, Yarborough K, Liu H, Sun G, Andersen ME, Pi J. 2011. Prolonged inorganic arsenite exposure suppresses insulin-stimulated AKT S473 phosphorylation and glucose uptake in 3T3-L1 adipocytes: involvement of the adaptive antioxidant response. Biochem Biophys Res Commun. 407(2):360–365.
- Yan LJ. 2014. Pathogenesis of chronic hyperglycemia: from reductive stress to oxidative stress. J Diabetes Res. 2014:137919.
- Yan N, Xu G, Zhang C, Liu X, Li X, Sun L, Wang D, Duan X, Li B. 2020. Chronic arsenic exposure induces the time-dependent modulation of inflammation and immunosuppression in spleen. Cell Biosci. 10:91.
- Yan S, Zhang QY, Zhou B, Xue L, Chen H, Wang Y, Zheng SS. 2009. Arsenic trioxide attenuated the rejection of major histocompatibility complex fully-mismatched cardiac allografts in mice. Transplant Proc. 41(5):1855–1858.
- Yang A, Liu S, Cheng N, Pu H, Dai M, Ding J, Li J, Li H, Hu X, Ren X, et al. 2017. Multiple metals exposure, elevated blood glucose and dysglycemia among Chinese occupational workers. J Diabetes Complications. 31(1):101–107.
- Yang B, Fu J, Zheng H, Xue P, Yarborough K, Woods CG, Hou Y, Zhang Q, Andersen ME, Pi J. 2012. Deficiency in the nuclear factor E2-related factor 2 renders pancreatic beta-cells vulnerable to arsenic-induced cell damage. Toxicol Appl Pharmacol. 264(3):315–323.
- Yang X, Yu Y, Xu J, Shu H, Xia J, Liu H, Wu Y, Zhang L, Yu Z, Fang M, et al. 2020. Clinical course and outcomes of critically ill patients with SARS-CoV-2 pneumonia in Wuhan, China: a single-centered, retrospective, observational study. Lancet Respir Med. 8(5):475–481.
- Yao XF, Zheng BL, Bai J, Jiang LP, Zheng Y, Qi BX, Geng CY, Zhong LF, Yang G, Chen M, et al. 2015. Low-level sodium arsenite induces apoptosis through inhibiting TrxR activity in pancreatic beta-cells. Environ Toxicol Pharmacol. 40(2):486–491.
- Yen CC, Lu FJ, Huang CF, Chen WK, Liu SH, Lin-Shiau SY. 2007. The diabetogenic effects of the combination of humic acid and arsenic: in vitro and in vivo studies. Toxicol Lett. 172(3):91–105.
- Yoon JW, Jun HS. 2005. Autoimmune destruction of pancreatic beta cells. Am J Ther. 12(6):580–591.
- Zhang J, Zhang Y, Wang W, Li C, Zhang Z. 2018. Double-sided personality: effects of arsenic trioxide on inflammation. Inflammation. 41(4):1128–1134.
- Zhang S, Cai Y, Meng C, Ding X, Huang J, Luo X, Cao Y, Gao F, Zou M. 2021. The role of the microbiome in diabetes mellitus. Diabetes Res Clin Pract. 172:108645.
- Zhao B, Xia JJ, Wang LM, Gao C, Li JL, Liu JY, Cheng QJ, Dai C, Ma QL, Qi ZQ, Zhao BH. 2018. Immunosuppressive effect of arsenic trioxide on islet xenotransplantation prolongs xenograft survival in mice. Cell Death Dis. 9(3):408.