Abstract
In-vitro chemical characterization and mosquitocidal screening of essential oil derived from Mikania scandens (L.) Willd. (Asteraceae) (CVO-MS) against the malarial vector Anopheles gambiae was investigated. GC-MS analysis evidenced a total of 12 bio-active compounds, and maximum at α-bisabolol (39.34%) followed by stigmasterol (13.45%) respectively. The larvicidal activity of CVO-MS against the malarial vector An. gambiae evidenced that the mortality rate was prominent at the maximum dosage of 1000 ppm. The Median Lethal Concentration (LC50) of CVO-MS was established at 488 ± 2.45 ppm, respectively. Enzyme inhibition assay showed that the CVO-MS delivers a significant upsurge in the level of CYP450, GST, and a decline in the level of α-β carboxylesterase activity in both the third and fourth instar (p ≤ 0.0001). The gut-histological examination of CVO-MS showed that there is severe damage in the mid-gut tissues, especially epithelial layer (EL), gut-lumen (GL), and peritrophic membrane (pM). The non-target screening against the mosquito predators (A. bouvieri, D. indicus, and third instar larvae of Tx. splendens) suggests that CVO-MS showed less toxicity as compared to Pestanal® (1 ppm) respectively. The present study has paved a new insight in understanding the bioactivity of CVO-MS as a potential larvicidal agent of malarial vector and non-toxic against mosquito predators.
Introduction
The genus Mikania are plants of a climber in nature that belongs to the family of Asteraceae and is found throughout Central America and Asia-Pacific regions (Ismail and Mah Citation1993). To date, 430 subspecies have been reported from the Mikania genus, and particularly 55 (13%) of them provide more than 300 different chemical constituents (Rufatto et al. Citation2012). The Mikania has been listed as one of the world's noxious weeds by the Invasive Species Specialist Group of the International Union for Conservation of Nature (Ismail and Mah Citation1993). In Malaysia, annual expenditure spent on controlling the Mikania (weed) in plantation crops (cocoa, oil palm, and rubber) has been estimated to be around 8–10 million US Dollars (Samsulrizal et al. Citation2013).
Anopheles gambiae Giles, generally referred to as the African malaria mosquito, is the supreme vector spreading human malaria in the tropical and sub-tropical regions (Centre for Disease Prevention and Control Citation2010). Anopheles gambiae is recognized as the crucial vector for spreading malarial disease due to their disposition to host as humans, proneness to the parasite, and their indoor-feeding behavior (Lija-Escaline et al. Citation2015). Controlling this disease is conceivable only through vector control for declining the burden of malaria (World Health Organization Citation2018). Chemical pesticides like organophosphates are displaying a negative impact on non-targets, especially human beings, once it is sprayed under in-door conditions. Also, synthetic chemicals generate high operational costs, and the up-surging rate of insecticidal resistance to malarial vector species has reduced the usage of organophosphates significantly under indoor residual spraying (Pradeepa et al. Citation2015; Senthil-Nathan Citation2020). Essential Oils (EOs) extracted from the botanical source have an extensive history as pest repellents and are also utilized in traditional folk medicines; they also endure receiving greater attraction as promising bio-active substances managing mosquito vectors. Moreover, the present scenario of insect toxicological research highly intensified detecting and evaluating the bioactive substances derived from botanicals for delivering dynamic natural insecticides. This drove us to carry out the present research in green-based insecticides focused on chemical characterization and mosquitocidal activity of CVO-MS against the mosquito vector.
Among the different Mikania species, Mikania scandens (L.) Willd. have been widely utilized as a folk medicine for the treatment of asthma, bronchitis, cough, fever, and itching, and they are also reported to have diverse biological activities such as analgesic, anti-coagulant, anti-diabetic, antifungal, anti-inflammatory, antimicrobial, antioxidant, anti-parasitic, antispasmodic, anti-tumor, anti-ulcer, and anti-viral and wound healing properties (Rufatto et al. Citation2012; Samsulrizal et al. Citation2013). In general, Mikania scandens is distinguished easily in the crop field with its unique physical structure that includes sagittate leaves, along with whitish flower heads. Moreover, this weedy plant can be grown rapidly and lengthy (Siddiqui et al. Citation2017). Mikania scandens has seasonal visitors of different flying insect species across Asian countries (Siddiqui et al. Citation2017). Despite this, the larvicidal activity of M. scandens against medically challenging arthropods remains unexplored.
Thus, the present research was aimed to investigate the chemical characterization and mosquitocidal activity of Essential Oil derived from Mikania scandens (CVO-MS) against the malarial vector An. gambiae and their non-target activity against mosquito predators.
Methodology
Plant harvesting and CVO extraction
The fresh plant leaves of M. scandens (Commonly known as climbing hemp-weed) were collected from the fields of Southern Western Ghats, Theni District, Tamil Nadu, India, in the early morning hours (9°40′58.49′′N; 77°15′01.35′′E) in the month of October 2020. The washed leaves were further air-dried using sterile filter paper. The identification of the plant species was authenticated with voucher specimen number (MS084SPK12) by a taxonomical scientist and deposited in the herbarium of (SPKCEESH), M.S. University, Alwarkurichi, TamilNadu, India. Further, the leaves were finely sliced and lay open to a steam distillation unit for CVO extraction using a rotary evaporator. The CVO-MS was parted and collected in sterile glass containers. The water drops were detached using anhydrous sodium sulfate, and the CVO was preserved at 4°C and utilized for the experiments.
Chemical pesticide
Temephos, an organophosphate larvicide (SIGMA-ALDRICH Pestanal®, analytical standard) was used for all biological assays as a reference compound.
Chemical characterization of CVO-MS
The isolated CVO-MS were further liquefied with ethyl alcohol in the ratio of (1:1). Further, two microliter botanical samples were dissolved using HPLC-grade ethanol and exposed to GC, and MS JEOL GC mate was fortified with an ancillary electron multiplier (JEOL GCMATE II GC-MS) (Agilent Technologies 6890-N Network). The HP-5 column was bonded with silica (50 m × 0.25 mm) I.D. Characterization conditions were fixed with 20 min (100°C), for column 3 min (235°C) temperature, and for injector temperature was set with 240°C, with helium gas, a carrier, with 5:4 split ratio was maintained. The one microliter botanical sample CVO-MS was evaporated with the split-less setting on the injector with the set temperature of 300°C at 22 min run time. Finally, the phytochemicals were characterized through gas chromatography fixed with mass spectrometry. The empirical formula, weight, and structure of the phytochemicals of CVO-MS were determined by elucidation using a mass spectrum of GC-MS and assessed with the National Institute Standard and Technology (NIST) database.
Mosquito rearing
Anopheles gambiae (s.s) culture has been maintained in the BET Lab, SPK Centre for Excellence in Environmental Sciences, without exposure to pesticides at 26 ± 1°C and 70–80% RH under a 15:10 L/D photoperiod and provided Brewer‘s yeast, dog biscuits (Choo Stix Biskies), and pool collected algae in a ratio of 3:2, respectively were given as diet for larvae. The primary mosquito strains were collected from the natural habitat pond located near the SPKCES center. The first-generation larvae were used for conducting an experiment.
Larvicidal assay
Larvicidal bioassay of CVO-MS was performed against the second, third, and fourth instar larvae of the malarial vector, which were placed into 250 ml sterile plastic cups containing 25 ml of the CVO-MS with discriminating dosages (250, 300, 400, 450, and 500 ppm), Temephos (1.0 ppm) as a biological standard and incubated at 27°C. Larvae were measured dead when they were unable to reach the surface of the solution when the cups were disturbed. The dead larvae were recorded post 24 as larval mortality. The whole setup was kept uninterrupted for added 24 h, and mortality counts were recorded post 48 h of treatment. The number of dead larvae number was resolute at the start of the experiment (0, 24, and 48 h). The larvicidal tests with more than 20% mortality in controls and if pupae formed were discarded and repeated again. The treatments were replicated five times, and each treatment contained 25 larvae, and each replicated set contained one control.
Bio-marker enzyme assay
The activity of enzyme assays (α and β-carboxylesterase) pre-treated with CVO-MS was performed according to the previous methodology adapted from Vasantha-Srinivasan et al. (Citation2018). Anopheles gambiae second, third and fourth instars were homogenized in 250 μl of 50 mM sodium phosphate buffer (pH 7.2) before being centrifuged at 15,000 × g at 4οC for 20 min. The Sigma-Aldrich (Catalog 0410, Bangalore) assay kit was used to evaluate the enzyme–substrate. The enzyme assay of GST and CYP450 was performed based on the previous protocol (Thanigaivel et al. Citation2017).
Gut-histological assay
The CVO-MS treated fourth instar larvae and control were stabilized overnight in Bouin's solution, de-hydrated, and attached to paraffin blocks of wax. Tissues of larval blocks were sectioned utilizing a microtome (Cryo-Cut 1800, Leica, Germany) mounted on a sterile glass slides sterile glass slide and stained with eosin and hematoxylin, and eosin (1.5 mL) and observed under a bright-field microscope (Optika vision lite 2.0 ML) linked to a laptop and the larval midgut tissues of the treated and control An. gambiae larvae were photographed using a digital camera connected with the microscope and compared with the control.
Non-target assay
The non-target toxicity effect was studied on the A. bouvieri, D. indicus, and third instar larvae of Tx. splendens a natural aquatic predator following the adapted methodology by Yogarajalakshmi et al. (Citation2020). The strains of non-targets A. bouvieri, D. indicus and Tx. splendens larvae were isolated in the same habitat field of An. gambiae (As mentioned under Mosquito rearing) and cultured separately in the containers (45 cm diameter and 14 cm depth) with sterile water at 26 ± 2°C −85% relative humidity (RH), and fed with II and III instars of An. gambiae. All the bio-monitoring organisms were exposed to the lethal dosage (200, 400, 800, and 1000 ppm) of CVO-MS and Pestanal®(1.0 ppm). For each treatment concentration, ten replicates were performed along with the five replicates of untreated controls. The mortality rate was recorded post 24 h of treatment.
Data analysis
Statistical values from the larvicidal assays were subjected to analysis of variance (ANOVA of arcsine, logarithmic and square root transformed percentages). Differences between the treatments were determined by Tukey's Kramer HSD test (P = 0.05) (Snedecor and Cochran, Citation1989). Statistical differences between each larvicidal treatment group were analyzed by Tukey's multiple range test (significance at p < 0.05) using the Minitab®17 program, and Microcal Software (Sigma plot 11) was used to plot the graph.
Results and discussion
Malaria is a significant disease that spreads through mosquitoes, mainly responsible for morbidity and mortality rates across African and Asian countries. The chief agent responsible for spreading this disease is An. gambiae (s.s); controlling mosquito vectors using chemical pesticides creates less impact due to indicative resistance (Pradeepa et al. Citation2015, Citation2016; Vasantha-Srinivasan et al. Citation2018). Green extracts, with their active phytochemicals, which deliver insecticidal actions against medically challenging arthropods, may help overcome problems of chemical insecticide resistance (Vasantha-Srinivasan et al. Citation2017; Luz et al. Citation2020). Phyto-compounds deliver a better expansion of eco-friendly products which are less expensive and highly effective against the harmful pests (Senthil-Nathan et al. Citation2005; Senthil-Nathan Citation2013, Citation2015; Pradeepa et al. Citation2015, Citation2016; Pavela et al. Citation2019).
The genus Mikania Willd. is considered to be an invasive botanical widely covers more than 430 species dispersed across the nations and they are largely distributed and exist with diverse biological compounds with higher pharmacological actions like antimicrobial, antioxidant, antipyretic, anti-inflammatory, and analgesic properties. Previous literature screening of phytochemicals derived from different Mikania species and their diverse biological actions were displayed (Table ). Mikania scandens is commonly known as Climbing Hemp-vine commonly available in tropical regions of Asian countries like India, Sri Lanka, Mauritius, Malaysia, Pakistan, and the Philippines (Wei et al. Citation2004; Moreira et al. Citation2016). The major phyto-derivatives of this M. scandens prominently include triterpenes, flavonoids, sesquiterpene kaurane diterpene, lactones, and also phytosterols (Moreira et al. Citation2016). Our present chemical characterization delivers eleven major derivatives, and the highest peak area displayed in α-bisabolol (39.34%), followed by stigmasterol (13.45%). Apart from that the CVO-MS also exhibit other phyto-compounds includes myrtenol (3.412%), naphthalene, 1,2,3,4-tetrahydro-1,6-dime (3.234%), ɤ-muurolene (7.865%), caryophyllene oxide (1.313%), taraxerol (3.216%), t-muurolol (2.65%),1,2-benzenedicarboxylic acid (3.21%), provincialin (9.87%), and phthalic acid (7.65%) respectively (Table ). All these compounds synergistically react together and might be responsible for larvicidal and enzyme inhibition activity. The extract and essential oils of climbing hemp-vine are widely utilized in the treatment of gastrointestinal ulcers and also other inflammatory ailments (Moreira et al. Citation2016). Also, extracts and CVO of M. scandens exhibit antipyretic, vulnerary, and anti-carcinogenic activities.
Table 1. Literature screening of phyto-chemicals derived from the Mikania species and their diverse biological activity.
Table 2. GC-MS characterization of crude volatile oil of Mikania scandens (L.) Willd and their chemical structure.
The larvicidal screening CVO-MS delivered a significant mortality rate at the different treatment dosages against the fourth instar larvae of the malarial mosquito vector. The mortality rate of second instar was profound in the 500 ppm dosage (97.88%- F4,20 = 23.13, p ≤ 0.0001) of MS-CVO and it is significant with 450 ppm-(92.35%- F4,20 = 23.13, p ≤ 0.0001), followed by 400 ppm-(83.92%- F4,20 =23.13, p ≤ 0.0001), 350 ppm-(76.54%-F4,20 = 23.13, p ≤0.0001), 300 ppm-(54.33%- F4,20 = 23.13, p ≤0.0001) and 250 ppm-(43.88%-F4,20 = 23.13, p ≤ 0.0001) and control – (6.43% F4,20 = 23.13, p ≤0.0001) respectively. Similar trends were observed in the third and fourth instars, respectively. Despite this, the larval mortality rate was profound in the second instars as compared to other instars. Moreover, there is no significant difference between the control and all other treatment dosages (250–500 ppm) of CVO-MS (Figure ). The Lethal Concentration (LC50) of CVO-MS against second, third and fourth instars of An. gambiae was tabulated (Suppl. Table 1). In contrast, Leeja et al. (Citation2020) research on water extracts of M. scandens showed no larval mortality against the filarial vector Culex quinquefasciatus. But, it is vital to consider that Essential Oils (EOs) are rich in bio-active compounds with diverse biological actions as compared to crude extracts, and these might generate CVO-MS significant larvicidal actions against the malarial vector.
Figure 1. Percentage mortality of second, third and fourth instar larvae of An. gambiae post treatment with crude volatile oil of M. scandens (CVO-MS). Means (SEM±) followed by the same letters above bars indicate no significant difference (P ≤ 0.05) according to a Tukey’s test.
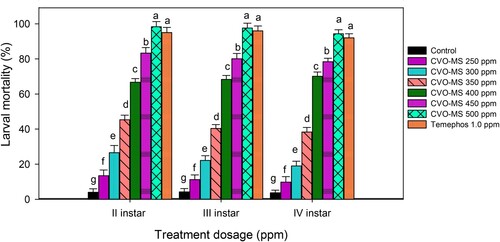
Detoxifying enzymes like carboxylesterase, GST, and CYP450 are considered the major bio-marker enzymes for analyzing the toxicity and resistance of any chemicals in the insect pests (Senthil-Nathan et al., Citation2007; Barlow et al. Citation2012; Regnault-Roger et al. Citation2012; Pavela Citation2015; Selin-Rani et al. Citation2016; Chellappandian et al. Citation2018). The enzyme regulation of the second, third and fourth instars of An. gambiae against the sub-lethal dosages of CVO-MS delivered that the level of carboxylesterase (α and β) activity declined significantly in dose–response respectively, and it was profound in the second instars as compared to third and fourth instars, respectively. The α-carboxylesterase level in second instars declined maximum at 250 ppm (0.482 mg, F4,20 = 21.44, p ≤ 0.0001) as compared to other treatment dosage 200 ppm (0.5210 mg, p ≤ 0.0001), 150 ppm (0.5921 mg, p ≤ 0.0001), 100 ppm (0.6612 mg, p ≤ 0.0001) and 50 ppm (0.6871 mg, p ≤ 0.0001) respectively (Figure A). Similar trends were observed in the third and fourth instar larvae. In parallel, the β – carboxylesterase regulation declined maximum at 250 ppm dosage of CVO-MS (0.6700 mg-F4,20 = 18.32, p ≤ 0.0001) as compared to the treatment dosages of 200 ppm (0.9800 mg, p ≤ 0.0001), 150 ppm (1.1320 mg, p ≤ 0.0001), 100 ppm (1.3400 mg, p ≤ 0.0001) and 50 ppm (1.6000 mg, p ≤ 0.0001) respectively (Figure B). Despite this, the level of GST (glutathione S-transferase) activity was significantly increased in dose–response in all the larval instars. The higher dosage of 250 ppm (F4,20 = 12.90, p ≤ 0.0001) showed increased enzyme levels in second instar larvae as compared to other dosages of 200 ppm (0.6432 mg, p ≤ 0.0001), 150 ppm (0.6121 mg, p ≤ 0.0001), 100 ppm (0.5613 mg, p ≤0.0001) and 50 ppm (0.4321 mg, p ≤ 0.0001) respectively (Figure C). Moreover, the level of CYP450 enzyme is also gets uplifted in the maximum sub-lethal dosage of 250 ppm (7.9456 mg, F4,20 = 23.13, p ≤ 0.0001) as compared to other treatments of 200 ppm (7.5430 mg, p ≤ 0.0001), 150 ppm (7.8910 mg, p ≤ 0.0001), 100 ppm (6.4320 mg, p ≤0.0001) and 50 ppm (5.4530 mg, p ≤ 0.0001) in An. gambiae second instar respectively (Figure D). Previously, it has been stated that the phytochemicals derived from plants generally deliver lethal actions in the mid-gut epithelial cells of the insect body and which in turn decline the metabolic function and deliver uneven enzyme activity (Prachayasittikul et al. Citation2015; Amala et al. Citation2021). The above statement is well parallel with our present research; the peri-tropic membrane (pM) and gut lumen (GL) also gets collapse in the CVO-MS treatment as compared to the control fourth instars of An. gambiae (Figure A). Whereas the sub-lethal dosage of CVO-MS (250 ppm) showed significant dysregulation in the fourth instar mid-gut cells as the epithelial layer (EL) get damaged heavily in the CVO-MS treatment (Figure B).
Figure 2. (A) α-carboxylesterase (B) β-carboxylesterase, (C) GST and (D) CYP450 enzyme activity of second, third and fourth instars of An. gambiae after treatment with crude volatile oil of M. scandens (CVO-MS). The data were fitted on polynomial (regression) model, whereas vertical bars indicate standard error (±SEM).
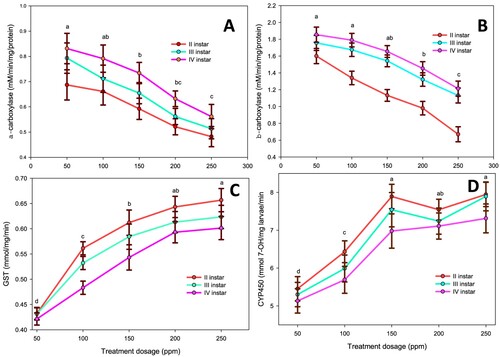
Figure 3. Gut-histological evaluation of An. gambiae instar post treatment with crude volatile oil of M. scandens (CVO-MS), (A) control, (B) Second instar, (C) third instar and (D) fourth instar. Whereas GL: Gut lumen; EL: Epithelial Layer; and pM: peritropic Membrane.
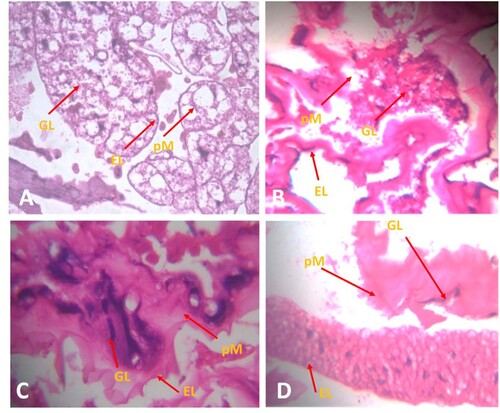
The mosquito predators Toxorhynchites species mosquitoes are conversant as possible natural biocontrol agents of mosquito vectors commonly known as ‘elephant mosquitoes’ (Pradeepa et al. Citation2015, Citation2016). Similarly, the bio-monitoring species like A. bouvieri and D. indicus are largely recognized as natural predators of mosquitoes sharing the same ecological niche of the malarial vector (Vasantha-Srinivasan et al., Citation2018; Yogarajalakshmi et al. Citation2020). It is suggested that the chemical toxins, including plant extracts toxicity against beneficial species, signify the targeted efficacy of that compound against the target pests and helps for effective formulations (Karthi et al. Citation2020). The non-target activity of CVO-MS against the bio-monitoring species Tx. splendens A. bouvieri and D. indicus displayed they are less toxic or harmless even at the maximum dosage treatment (1000 ppm). The larval mortality of mosquito predators was observed to be minimal at CVO-MS 1000 ppm dosage at Tx. splendens-(10.23%- F4,20 = 16.49, p ≤ 0.0001), A. bouvieri (7.67.66%- F4,20 = 23.29, p ≤ 0.0001), and D. indicus (6.88%- F4,20 = 32.11, p ≤ 0.0001) respectively as compared to the Pestanal® (1 ppm) showed maximum mortality rate at Tx. splendens-(97.53%- F4,20 = 27.79, p ≤ 0.0001), A. bouvieri (88.45%- F4,20 = 13.59, p ≤ 0.0001), and D. indicus (94.5%- F4,20 = 38.68, p ≤ 0.0001) respectively (Figure ). Moreover, the enzyme inhibition activity of CVO-MS against the non-targets displayed that α (F4,20 = 25.21, p ≤ 0.0001), β-carboxylesterase (F4,20 = 18.11, p ≤ 0.0001), GST (F4,20 = 22.13, p ≤ 0.0001) and CYP450 (F4,20 = 14.44, p ≤ 0.0001) level was normal even at the maximum treatment dosage of 500 ppm and it was dose-dependent (Figure. ). Thus, our current results deliver that the bio-active compounds of CVO-MS are ecologically safe and can be introduced into the Anopheles habitat to control the arthropod larvae.
Figure 4. Effects of crude volatile oil of M. scandens (CVO-MS) against non-target organism Tx. splendens A. bouvieri, and D. indicus Means (SEM±) followed by the same letters above bars indicate no significant difference (P ≤ 0.05) by using Probit analysis.
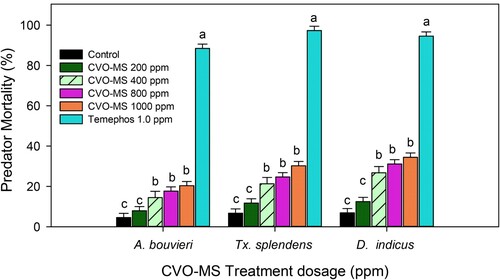
Figure 5. (A) α-carboxylesterase (B) β-carboxylesterase, (C) GST and (D) CYP450 enzyme activity of non-target organism Tx. splendens A. bouvieri, and D. indicus after treatment with crude volatile oil of M. scandens (CVO-MS). The data were fitted on polynomial (regression) model, whereas vertical bars indicate standard error (±SEM).
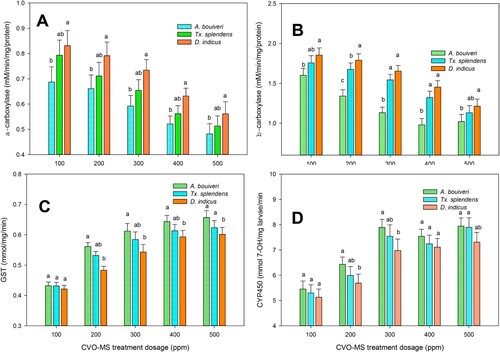
Conclusion
In the present study, it was found that volatile crude oil derived from M. scandens showed eleven major phytochemicals with the highest peak area observed in natural monocyclic sesquiterpene alcohol α – bisabolol. Further, the larvicidal screening of CVO-MS against the malarial vector An. gambiae showed that they are highly lethal at the maximum dosage of 1000 ppm and the sub-lethal dosage of CVO-MS also delivered significant fluctuation in the level of major detoxifying enzymes carboxylesterase, GST, and CYP450. Also, the mid-gut toxicity also showed dysregulation of midgut cells and tissues exposed to the toxic dosage of CVO-MS. Moreover, CVO-MS appears to be target-specific and harmless/less toxic to the aquatic predator's Tx. splendens A. bouvieri and D. indicus and can be utilized as a dynamic substitute for global burden chemical pesticides. Future perspectives of the research include active formulations of bioactive derivatives of Mikania, and their efficacy will be evaluated under field conditions.
Author contributions
Narayanaswam Radhakrishnan: study conception and design, data collection, analysis, and interpretation of results, and draft manuscript preparation.
Sengodan Karthi: study conception and design, data collection, analysis, and interpretation of results, and draft manuscript preparation.
Pandiyan Raghuraman: study conception and design and interpretation of results.
Raja Ganesan: data collection, analysis, and interpretation of results, and draft manuscript preparation.
Kumaraswamy Srinivasan: data analysis and interpretation of results, and draft manuscript preparation.
Edward-Sam Edwin: data analysis and interpretation of results, and draft manuscript preparation.
Selvaraj Ganesh-Kumar: data collection, analysis, and interpretation of results, and draft manuscript preparation.
Norhaizan Mohd Esa: data analysis, and interpretation of results, and draft manuscript preparation.
Sengottayan Senthil-Nathan: data analysis, and interpretation of results, and draft manuscript preparation.
Prabhakaran Vasantha-Srinivasan: data analysis, and interpretation of results, and draft manuscript preparation.
Patcharin Krutmuang: data analysis, and interpretation of results, and drafted manuscript preparation.
Mona S Alwahibi: analysis, and interpretation of results, and drafted manuscript preparation.
Mohamed Soliman Elshikh: analysis, and interpretation of results, and drafted manuscript preparation. All authors reviewed the results and approved the final version of the manuscript.
Ethical statement
The research presented in this manuscript involves experiment of non-target aquatic insects and invertebrates and the experimental species are not under current regulations of ethical approval.
Supplemental Material
Download MS Excel (11.8 KB)Acknowledgments
We would like to thank the Division of Bio-pesticides and Environmental Toxicology, Sri Paramakalyani Centre for Excellence in Environmental Sciences, Manonmaniam Sundaranar University, Tirunelveli, India for providing the infrastructural facility for carrying out this research work and also, this work was partially supported Post-Doctoral Program, Chiang Mai University, Thailand. In addition, the first author (NR) would like to thank the Research Management Center (RMC) of Universiti Putra Malaysia (UPM) for supporting this research. The authors extend their appreciation to the Researchers supporting project number (RSP2023R173), King Saud University, Riyadh, Saudi Arabia. This research work was partially supported by Chiang Mai University, Thailand.
Disclosure statement
No potential conflict of interest was reported by the author(s).
Data availability statement
The data are not publicly available due to the funding agency contract. Since the present research was financially supported by the Early Career Research Award funded by the Science and Engineering Research Board [DST-SERB], Government of India, data cannot be made publicly available.
Additional information
Funding
References
- Amala K, Ganesan R, Kathi S, Senthil-Nathan S, Chellappandian M, Krutmunag P, et al. 2021. Larval and gut enzyme toxicity of n-hexane extract Epaltes pygmaea DC. against the arthropod vectors and its non-toxicity against aquatic predator. Toxin Rev. C40(4):681–691.
- Amorin M, Paula JPD, Silva RZD, Farago PV, Budel JM. 2014. Pharmaco-botanical study of the leaf and stem of Mikania lanuginosa for its quality control. Revista Brasileira de Farmacognosia. 24(5):531–537.
- Barlow DJ, et al. 2012. In-silico studies in Chinese herbal medicines’ research: evaluation of in-silico methodologies and phytochemical data sources, and a review of research to date. J Ethnopharmacol. 140:526–534.
- Bolina RC, et al. 2009. Estudo comparativo da composição química das espécies vegetais Mikania glomerata Sprengel e Mikania laevigata Schultz Bip. ex Baker. Revista Brasileira de Farmacognosia. 19:294–298.
- Borghi AA, et al. 2019. Damage and drying modify the composition of Mikania glomerata and Mikania laevigata leaves. Revista Brasileira de Farmacognosia. 29:793–797.
- Centre for Disease Prevention and Control. 2010. Anopheles Mosquitoes. Malaria. [accessed 2019 June 14] https://www.cdc.gov/malaria/about/biology/#tabs-1-5.
- Chellappandian M, et al. 2018. Toxicological effects of Sphaeranthus indicus Linn. (Asteraceae) leaf essential oil against human disease vectors, Culex quinquefasciatus Say and Aedes aegypti Linn., and impacts on a beneficial mosquito predator. Environmental Science and Pollution Research. 25(11):10294–10306.
- Chowdhury JU, et al. 2007. Aromatic plants of Bangladesh: constituents of the leaf and flowers oils of Mikania cordata (Burm.f.) Rob. Indian Perfumer. 51:56–59.
- Ferreira FP, de Oliveira DCR. 2010. New constituents from mikania laevigata shultz Bip. ex baker. Tetrahedron Lett. 51(52):6856–6859.
- Ismail BS, Mah LS. 1993. Effects of Mikania micrantha HBK on germination and growth of weed species. Plant Soil. 157:107–113.
- Jiang RW, et al. 2001. A novel 1: 1 complex of potassium Mikanin-3-O-sulfate with methanol. Chem Pharm Bull. 49(9):1166–1169.
- Karthi S, Vinothkumar M, Karthic U, Manigandan V, Saravanan R, Vasantha-Srinivasan P, et al. 2020. Biological effects of Avicennia marina (Forssk.) vierh. extracts on physiological, biochemical, and antimicrobial activities against three challenging mosquito vectors and microbial pathogens. Environmental Science and Pollution Research. 27(13):15174–15187.
- Laurella LC, Cerny N, Bivona AE, et al. 2017. Assessment of sesquiterpene lactones isolated from mikania plants species for their potential efficacy against Trypanosoma cruzi and Leishmania sp. PLoS Negl Trop Dis. 11(9):e0005929.
- Leeja L., Sulakshana G. N., Vemeethar V., Praniha P., Megha M., Nivedhitha V., Sumi D., Gayathri T. S., Vishnuram S. 2020. Larvicidal Activity and Phytochemical Analysis of Some Selected Plant Extracts Against Filarial Vector Culex Quinquefasciatus Say (Diptera: Culicidae).
- Lija-Escaline J, Senthil-Nathan S, Thanigaivel A, Pradeepa V, Vasantha-Srinivasan P, Ponsankar A, Edwin ES, Selin-Rani S, Abdel-Megeed A. 2015. Physiological and biochemical effects of botanical extract from Piper nigrum Linn (Piperaceae) against the dengue vector Aedes aegypti Liston (Diptera: Culicidae). Parasitol Res. 114(11):4239–4249.
- Limberger R, et al. 1998. Chemical composition of essential oils from three southern Brazilian species of Mikania (Asteraceae). J Essent Oil Res. 10(4):363–367.
- Luz TRSA, de Mesquita LSS, do Amaral FMM, Coutinho DF. 2020. Essential oils and their chemical constituents against Aedes aegypti L. (Diptera: Culicidae) larvae. Acta Trop. 212:105705.
- Moreira MR, et al. 2016. ent-Kaurenoic acid-rich extract from Mikania glomerata: In vitro activity against bacteria responsible for dental caries. Fitoterapia. 112:211–216.
- Oliveira PA, Turatti ICC, Oliveira DCO. 2006. Comparative analysis of triterpenoids from Mikania cordifolia collected from four different locations. Revista Brasileira de Ciências Farmacêuticas. 42:547–552.
- Pavela R. 2015. Essential oils for the development of eco-friendly mosquito larvicides: A review. Ind Crops Prod. 76:174–187.
- Pavela R, Maggi F, Iannarelli R, Benelli G. 2019. Plant extracts for developing mosquito larvicides: from laboratory to the field, with insights on the modes of action. Acta Trop. 193:236–271.
- Pedroso ADD, Sheila AAS, Santos F, Deschamps A, Barison F, Campos MW, Biavatti MW. 2008. Isolation of syringaldehyde from Mikania laevigata medicinal extract and its influence on the fatty acid profile of mice. Revista Brasileira de Farmacognosia. 18(1):63–69.
- Prachayasittikul V, et al. 2015. Computer-aided drug design of bioactive natural products. Curr Top Med Chem. 15:1780–1800.
- Pradeepa V, Sathish-Narayanan S, Kirubakaran SA, Thanigaivel A, Senthil-Nathan S. 2015. Toxicity of aristolochic acids isolated from Aristolochia indica Linn (Aristolochiaceae) against the malarial vector Anopheles stephensi Liston (Diptera: Culicidae). Exp Parasitol. 153:8–16.
- Pradeepa V, Senthil-Nathan S, Sathish-Narayanan S, Selin-Rani S, Vasantha-Srinivasan P, Thanigaivel A, Ponsankar A, Edwin ES, Sakthi-Bagavathy M, Kalaivani K, Murugan K. 2016. Potential mode of action of a novel plumbagin as a mosquito repellent against the malarial vector Anopheles stephensi,(Culicidae: Diptera). Pestic Biochem Physiol. 1134:84–93.
- Regnault-Roger C, Vincent C, Arnason JT. 2012. Essential oils in insect control: low-risk products in a high-stakes world. Annu Rev Entomol. 57:405–424.
- Rufatto LC, Finimundy TC, Roesch-Ely M, Moura S. 2013. Mikania laevigata: chemical characterization and selective cytotoxic activity of extracts on tumor cell lines. Phytomedicine. 20(10):883–889.
- Rufatto LC, Gower A, Schwambach J, Moura S. 2012. Genus Mikania: chemical composition and phytotherapeutical activity. Revista Brasileira de Farmacognosia. 22(6):1384–1403.
- Samsulrizal N, Suhaimi NA, Sopian NFA, Hatta SKM, Jamil NM. 2013. Hypoglycaemic, antioxidant and wound healing activities of Mikania micrantha leaves extract in normal and alloxan-induced diabetic rats. Focus Scope. 7:6–10.
- Santos SC, et al. 2006. Lc characterisation of guaco medicinal extracts, Mikania laevigata and M. glomerata, and their effects on allergic pneumonitis. Planta Med. 72(08):679–684.
- Selin-Rani S, Senthil-Nathan S, Thanigaivel A, et al. 2016. Toxicity and physiological effect of quercetin on generalist herbivore, Spodoptera litura Fab. and a non-target earthworm Eisenia fetida Savigny. Chemosphere. 165:257–267.
- Senthil-Nathan S, et al. 2005. Effects of neem limonoids on the malaria vector Anopheles stephensi Liston (Diptera: Culicidae). Acta Trop. 96:47–55.
- Senthil-Nathan S. 2013. Physiological and biochemical effect of neem and other Meliaceae plants secondary metabolites against Lepidopteran insects. Front Physiol. 4(359):1–17.
- Senthil-Nathan S. 2015. A review of bio pesticides and their mode of action against insect pests. In: Environmental sustainability- role of green technologies. Springer-Verlag, India.; p. 49–63.
- Senthil-Nathan S. 2020. A review of resistance mechanisms of synthetic insecticides and botanicals, phytochemicals, and essential oils as alternative larvicidal agents against mosquitoes. Front. Physiol. 1591:1–21. https://www.frontiersin.org/articles/10.3389/fphys.2019.01591/full.
- Senthil-Nathan S, Choi MY, Paik CH, Seo HY. 2007. Food consumption, utilization, and detoxification enzyme activity of the rice leaffolder larvae after treatment with Dysoxylum triterpenes. Pestic Biochem Physiol. 88(3):260–267.
- Siddiqui SA, Islam R, Islam R, Jamal AHM, Parvin T, Rahman A. 2017. Chemical composition and antifungal properties of the essential oil and various extracts of Mikania scandens (L.) Willd. Arabian Journal of Chemistry. 10:S2170–S2174.
- Snedecor G. W., Cochran W. G. 1989. Statistical Methods. 8th edition. Ames, IA: Iowa State University Press.
- Thanigaivel A, et al. 2017. Chemicals isolated from Justicia adhatoda Linn reduce fitness of the mosquito. Arch Insect Biochem Physiol. 94(4):e21384.
- Vasantha-Srinivasan P., Senthil-Nathan S., Ponsankar A., Thanigaivel A., Chellappandian M., Edwin E. S., Selin-Rani S., Kalaivani K., Hunter W. B., Duraipandiyan V., Al-Dhabi N. A. 2018. Acute toxicity of chemical pesticides and plant-derived essential oil on the behavior and development of earthworms, Eudrilus eugeniae (Kinberg) and Eisenia fetida (Savigny). Environmental science and pollution research. 25(11):10371–10382.
- Vasantha-Srinivasan P., Senthil-Nathan S., Ponsankar A., Thanigaivel A., Edwin E. S., Selin-Rani S., Chellappandian M., Pradeepa V., Lija-Escaline J., Kalaivani K., Hunter W. B. 2017. Comparative analysis of mosquito (Diptera: Culicidae: Aedes aegypti Liston) responses to the insecticide Temephos and plant derived essential oil derived from Piper betle L. Ecotoxicology and environmental safety. 139:439–446.
- Vasantha-Srinivasan P, et al. Toxicological effects of chemical constituents from Piper against the environmental burden Aedes aegypti Liston and their impact on non-target toxicity evaluation against biomonitoring aquatic insects. Environ Sci Pollut Res. 25:10434–10446.
- Vidal LHI, et al. 2006. Qualidade de mudas de guaco produzidas por estaquia em casca de arroz carbonizada com vermicomposto. Horticultura Brasileira. 24:26–30.
- Wei X, Huang H, Wu P, Cao H, Ye W. 2004. Biochem Syst Ecol. 32:1091–1096.
- World Health Organization. 2018. World malaria report. Geneva.: World Health Organization.
- Yogarajalakshmi P, Poonguzhali TN, Ganesan R, Karthi S, Senthil-Nathan S. et al. 2020. Toxicological screening of marine red algae Champia parvula (C. Agardh) against the dengue mosquito vector Aedes aegypti (Linn.) and its non-toxicity against three beneficial aquatic predators. Aquat Toxicol 222: 105474.