Abstract
Propofol is widely used as a short-acting intravenous anaesthetic for the induction and maintenance of anaesthetic sedation. The application of propofol is expanding due to its advantages and the increasing demand for painless diagnosis and treatment. However, accumulating clinical and experimental evidence has shown that propofol can potentially be abused and is prone to causing addiction. To further understand the underlying mechanism of propofol addiction, our review places emphasis on explaining the roles of the mesolimbic, noradrenergic, and nitrergic systems in propofol addiction. We have focused on the role of the gamma-aminobutyric acid, glucocorticoid, N-methyl-D-aspartic acid, adenosine A2A, and corticotropin-releasing factor receptors in the mesolimbic system in forming and maintaining propofol addiction. Furthermore, we have attempted to clarify the relationship between the dopaminergic pathway, extracellular signal-regulated kinase signalling pathway, and the ventral tegmental area-nucleus accumbens circuit in the mesolimbic system.
Key Policy Highlights
Although propofol is effective in inducing and maintaining anesthesia, it is prone to cause abuse and addiction.
The mesolimbic, noradrenergic, and nitrergic systems are involved in propofol addiction.
The dopaminergic pathway, extracellular signal-regulated kinase signalling pathway, and the ventral tegmental area-nucleus accumbens circuit in the mesolimbic system may be involved in the maintenance of propofol addiction.
Introduction
Propofol is a widely used anaesthetic for the induction and maintenance of anaesthetic sedation. It can inhibit the central nervous system by activating the GABA receptors, so as to exert its sedative and hypnotic effects. Moreover, propofol can reduce the blood pressure and vascular resistance by inhibiting the circulatory system, with no significant change on heart rate. With the widespread application of propofol, its side effects have gradually emerged. Clinical and animal studies have provided reliable evidence that propofol may cause drug abuse and addiction. Lately, propofol addiction has garnered increasing attention; however, the underlying mechanisms of propofol addiction remain unknown. In this review, we focus on the roles of the mesolimbic, noradrenergic, and nitrergic systems in propofol addiction and explain the different roles of related neurotransmitters and receptors, signal pathways, and neural circuits.
Extensive application of propofol
Propofol is a widely used sedative-hypnotic agent for the induction and maintenance of general anaesthesia. Due to its rapid onset advantages, quick action, rapid metabolism, and fast clearance (Takizawa et al. Citation2005; Wilson et al. Citation2010), clinical anaesthesiologists prefer propofol. With the popularisation of painless procedures, such as endoscopy, colonoscopy, and short-term invasive surgical attempts (Abu Baker et al. Citation2019; Tekeli et al. Citation2020), the application of propofol has expanded. In addition, recent studies have revealed that propofol has antioxidant, anti-inflammatory effects (Haitsma et al. Citation2009) and may be a new agent for the treatment of ischemic stroke (Wang et al. Citation2020) and alleviation of cognitive impairment (Zhou and Qiu Citation2019). In recent years, researchers have focused on the role of propofol in inhibiting different cancer types, such as ovarian, gastric, and cervical cancer (Li et al. Citation2017; Zeng et al. Citation2020; Zhang et al. Citation2020), suggesting that propofol has a wide range of applications in the field of oncology. An emphasis on educating people on the uses and expanding the reach of propofol is recommended to ensure increased access to these benefits, especially in the operating room (Merlo et al. Citation2008).
Abuse potential and addiction properties of propofol
Drug abuse refers to the repeated and extensive use of drugs with dependence characteristics or potential. Drug abuse has become a common societal concern. Although propofol has a wide range of applications in clinical practice, its potential for abuse should not be overlooked. The first reported case of propofol abuse was reported in 1991 by an anaesthetist (Follette and Farley Citation1992). Double-blind, randomised, crossover experiments on healthy volunteers have shown that propofol has the potential for abuse in humans (Zacny et al. Citation1993a, Citation1993b). Most reports have revealed that most propofol abusers are anaesthetists because they have easy access to and exposure to propofol (McAuliffe et al. Citation2006). One study analysing 21 propofol-related deaths revealed that most of these events occurred among anaesthesiologists and nurse anaesthetists (Diaz and Kaye Citation2017). In 2009, Michael Jackson was presumed to have died of propofol abuse, significantly raising awareness of propofol abuse (Monroe et al. Citation2011). In recent years, several propofol abuse-related deaths of healthcare staff have been reported in countries such as Turkey, Germany, Austria, and Switzerland (Köroğlu and Tezcan Citation2015; Maier et al. Citation2017).
Addiction is a chronic, relapsing disorder and is the consequence of drug abuse (Camí and Farré Citation2003). A patient who was repeatedly exposed to propofol at an anaesthetic dose eventually developed an addiction, suggesting that patients who were frequently administered propofol during operations are at a higher risk for propofol addiction (Arias et al. Citation2011). Several patients described a sense of euphoria during endoscopy when anesthetised with propofol (Brechmann et al. Citation2018). Furthermore, a study of propofol abuse among 38 non-healthcare workers in Korea indicated that the main purpose for first-time propofol intake was to seek stress relief, and 97.3% of abusers described a sense of euphoria upon receiving a propofol injection (Kim et al. Citation2015). Thus, seeking a sense of relief and feelings of euphoria are the potential allures that cause propofol abuse, as cravings lead to addiction (Bonnet and Scherbaum Citation2012). Notably, propofol addiction can result in death; respiratory depression is apparently the main cause (Lee Citation2012). The above literature clearly elucidates that an increasing number of people face the risk of propofol addiction consciously or unconsciously. Hence, several organisations have categorised propofol as a controlled substance. Wilson et al. recommended that the United States Drug Enforcement Administration regulate propofol (Wilson et al. Citation2010), after which propofol was included in the proposed rules for controlled substances in 2010 (Stocks Citation2011). Korea was the first country to categorise propofol as a psychotropic substance to reduce propofol abuse and addiction (Han et al. Citation2013).
Mechanism of propofol addiction
Mesolimbic system
Neurotransmitters and receptors
Gamma-aminobutyric acid receptor (GABAR)
Gamma-aminobutyric acid is an important inhibitory neurotransmitter in the central nervous system, and GABARs are classified into three subtypes: GABAAR, GABABR, and GABACR (Bormann and Feigenspan Citation1995). Previous studies have revealed that GABAAR and GABABR are involved in drug addiction (Kumar et al. Citation2013; Stephens et al. Citation2017). Recently, Yang et al. studied the effects of GABAAR and GABABR on propofol reinforcement in the ventral tegmental area (VTA) using the respective antagonists bicuculline and baclofen. They showed that systemic pretreatment and intra-VTA injection with baclofen decreased propofol self-administration, whereas systemic pretreatment with bicuculline promoted propofol self-administration (Yang et al. Citation2011). It is worth noting that propofol can activate GABAAR (Sahinovic et al. Citation2018), and this property may have counteracted the effect of bicuculline in this study. Thus, Yang et al. showed that GABAAR activation played a role in partially maintaining the propofol reward properties and that directly stimulating GABABR within the VTA may adversely affect the propofol reward properties (Yang et al. Citation2011).
Moreover, propofol increases dopamine (DA) release in the nucleus accumbens (NAc) by agonising GABAergic neurons in the VTA, leading to its addiction (Margolis et al. Citation2012). However, the GABAAR subtypes that play a dominant role in the discriminative effects of propofol remain unknown. Among CL218,872 (α1 GABAAR agonist), SL651,498 (α2/3 GABAAR agonist), and L838,417 (α2/3/5 GABAAR agonist), only L838,417 showed the ability to completely negate propofol effects, proving that propofol has the property of addiction and that α5 GABAAR plays a dominant role in the process of propofol discriminative effects (Wang et al. Citation2018a).
Glucocorticoid receptor (GR)
Glucocorticoids (GCs) are members of the steroid hormone family, which regulate the development, growth, metabolism, and immune function in the body. Previous studies have revealed the involvement of GCs and GR in cocaine and morphine addiction (Deroche-Gamonet et al. Citation2003; Dong et al. Citation2006). In recent years, a study by Wu et al. has provided clear evidence that GRs also participate in propofol reward. With systemic pretreatment with dexamethasone (a potent GR agonist) and RU486 (a specific GR antagonist), they observed that both dexamethasone (at a high dose) and RU486 decreased the nose-poke responses, infusions, and dopamine D1 receptor (D1R) expression in the NAc, indicating that both agonists and antagonists reduced the maintenance of propofol addiction and rewarding effects (Wu et al. Citation2016). Interestingly, intra-NAc injection of dexamethasone increased the active-nose poke responses, with higher D1R and c-Fos expression in the NAc (Wu et al. Citation2018). The effects might have been more pronounced if antagonists had been added.
N-methyl-D-aspartic acid (NMDA) receptor
NMDA receptors are subtypes of ionic glutamate receptors that are involved in the process of drug addiction (Liu et al. Citation2016; Morisot and Ron Citation2017). Previous studies have shown that MK-801, a non-competitive NMDA receptor antagonist, inhibits the onset of morphine withdrawal syndrome and cocaine self-administration behaviour (Higgins et al. Citation1992; Schenk et al. Citation1993). Recently, Chen et al. investigated the role of NMDA receptors in the mechanism of propofol addiction using MK-801. A significant difference was observed in the number of infusions per session following MK-801 administration, with a significant decrease in the number of infusions depending on the dose (Chen et al. Citation2020). These results are consistent with those of previous studies. However, the specific role of NMDA receptors in the mechanism of propofol addiction requires further study.
Adenosine A2A receptor (A2AR)
Adenosine A2AR is a subtype of adenosine receptor involved in the maintenance of sleep (Al-Attraqchi et al. Citation2019) and regulation of the heart and cardiovascular system (Reiss et al. Citation2019). Dong et al. investigated whether adenosine A2AR in the NAc modulated propofol addiction. After a stereotaxic injection of AAV-shA2AR and AAV-shCTRL, they found that AAV-shA2AR caused a significant decrease in the A2AR in the NAc, with no difference in the propofol self-administration behaviour. Upon completing the propofol self-administration schedule, the authors withdrew propofol for 14 days and tested the recovery of propofol self-administration behaviour induced by cues on the next day. The results indicated that the AAV-shA2AR group showed more active nose-poke responses than the AAV-shCTRL group. This implies that A2AR inhibition can activate propofol relapse induced by cues in rats. This conclusion was further reinforced by using the A2AR agonist CGS21680 and A2AR antagonist MSX-2. Double-side intra-NAc microinjections of CGS21680 inhibited the active nose-poke responses at a dose of ≥5.0 ng/site, and MSX-3 increased the active nose-poke responses depending on the dose. Interestingly, using the dopamine D2 receptor (D2R) antagonist eticlopride also decreased the recovery from propofol addiction induced by cues, revealing that there might be an interaction between A2AR and D2R in this process (Dong et al. Citation2021a).
Corticotropin-releasing factor receptor (CRFR)
Stress is a risk factor for drug addiction. Previous studies have revealed that corticotropin-releasing factor (CRF) and its receptors CRF1R and CRF2R play important roles in the interaction between stress and drug addiction (Bale and Vale Citation2004; Sinha and Jastreboff Citation2013). Recently, Dong et al. focused on the interaction between stress and propofol addiction and also investigated the role of CRF receptors in maintaining propofol addiction induced by stress. They demonstrated that stress (induced by clipping the rats’ tails) promoted propofol addiction in a propofol-administered model. Through lateral ventricle injection of antalarmin (CRF1R antagonist), antisauvagine 30 (CRF2R antagonist), and RU486, they observed that only antalarmin inhibited the stress-induced propofol self-administration behaviour and D1R expression in the NAc, as analysed by western blotting. The study proved that stress is a high-risk factor for developing propofol addiction and that CRF1R antagonists can inhibit the maintenance of propofol addiction by regulating D1R expression (Dong et al. Citation2021b).
Signal pathways
Dopaminergic pathway
The role of the dopaminergic pathway in drug addiction is well established (Walsh et al. Citation2003). Previous studies have demonstrated that D1R and D2R in the NAc are associated with the maintenance of drug addiction (Seif et al. Citation2011). A study by Lian et al. revealed that only the D1R played a crucial role in the reinforcing properties of propofol addiction in the NAc. Using the D1R antagonist SCH23390, D2R antagonist spiperone, and eticlopride after self-administration, they found that only SCH23390 reduced self-administration in rats, depending on the dose (Lian et al. Citation2013). Wang et al. investigated the potential relationship between propofol cue-induced resorption behaviour and dopamine receptors. After establishing the rat propofol self-administration model, the rats were forced to withdraw from propofol. During the process of propofol withdrawal, the authors tested propofol resorption behaviour induced by cues, revealing that cues related to propofol strongly induced propofol-seeking behaviour. With a systematic injection of SCH23390 and spiperone, the propofol relapse behaviours induced by cues decreased in rats. Furthermore, after an intra-basolateral amygdala (BLA) injection of SCH23390 and spiperone, the cue-induced propofol resorption behaviour was inhibited only by SCH23390, indicating that D1R played a dominant role in propofol resorption behaviour induced by cues in the BLA (Wang et al. Citation2018b). These studies suggested the differences in the role of the dopamine receptor in the maintenance of drug addiction between propofol and other drugs, such as nicotine (Linker et al. Citation2020).
Extracellular signal-regulated kinase (ERK) signalling pathways
The extracellular signal-regulated kinase is a member of the mitogen-activated protein kinase family and an important molecule of signal transduction (Nathan and Charles Citation2019). Previous studies have shown that the ERK and ERK signalling pathways are involved in the progression of cocaine, morphine, and nicotine addiction (Valjent et al. Citation2004; Corbillé et al. Citation2007). Using U0126, a selective ERK inhibitor, Wang et al. found that a specific dose (4 µg/side) of U0126 decreased propofol infusion behaviours in rats. Moreover, ERK expression substantially increased after propofol self-administration and reduced after an infusion of SCH23390. The results showed that the ERK signalling pathway played a role in the rewarding effects of propofol addiction. Furthermore, ERK signalling pathways accompanied by D1R in the NAc may be connected to the maintenance of propofol addiction (Wang et al. Citation2016).
Neural circuits
Ventral tegmental area-nucleus accumbens (VTA-NAc) circuit
Both the VTA and NAc are important components of the mesolimbic system. Previous studies have revealed that the VTA-NAc circuit plays a critical role in the process of drug addiction (Mameli et al. Citation2009; McCutcheon et al. Citation2011). Here, we emphasise the different functions of the VTA-NAc circuit in propofol addiction caused by acute exposure and chronic exposure to propofol.
Propofol addiction caused by acute exposure may be associated with increased dopamine levels. A study by Li et al. suggested that 1 nM propofol increased the activity of the VTA dopamine neurons by enhancing glutamate transmission (Li et al. Citation2008), and VTA dopamine neurons stimulated dopamine release in the NAc (Nestler and Carlezon Citation2006) As for propofol addiction caused by chronic exposure, ΔFosB may play an important role. ΔFosB is a transcription factor induced by addictive drugs. Owing to its stability, ΔFosB can accumulate within neurons in the NAc after repeated administration of addictive drugs (Nestler et al. Citation2001). A study by Xiong et al. suggested that repeated injection of propofol in rats significantly increased ΔFosB expression in the NAc at the mRNA and protein levels, with a significantly increased D1R expression at the mRNA and protein levels (Xiong et al. Citation2011) It is worth noting that D1R is the upstream signal molecule of ERK and ΔFosB, and ERK is the upstream signal molecule of ΔFosB (Wang et al. Citation2016; Wen et al. Citation2020). Hence, it seems that D1R-ERK-ΔFosB signalling may modulate propofol addiction caused by chronic exposure.
Noradrenergic System
The mesolimbic system and locus coeruleus (LC) noradrenergic system both play vital roles in regulating reward behaviour (Du et al. Citation2018). Most current studies have focused on the LC, which is involved in the recovery from propofol general anaesthesia, sleep-wake processes, and neurodegenerative diseases (Havekes et al. Citation2019; Jameie et al. Citation2019; Chen et al. Citation2021). Few studies have targeted the role of LC in the mechanism of propofol addiction. The hedonic effect is characteristic of drug addiction (Eliot, Citation2011). Recently, Chen et al. investigated the role of the VTA in the dopaminergic system and LC neurons in the noradrenergic system in the propofol-induced euphoric state. They injected propofol through the tail vein at doses of 0, 2, 4, 6, and 8 mg/kg and observed that 75% of rats were anaesthetised at a dose of 8 and 4 mg/kg of propofol induced hedonic responses in rats. Using the conditioned place preference (CPP) method, they reported a similar phenomenon; at a dose of 4 mg/kg, rats had the highest locomotor activity and preference for propofol. GCaMP was used as a marker for LC and VTA neurons, and fibre photometry was used to record neuronal activity. They observed that propofol promoted neuronal activity in the LC at a dose of 4 mg/kg, whereas neuronal activity in the VTA did not change. Furthermore, through chemogenetic inhibition, when LC neuronal activity was suppressed, the hedonic effects disappeared at a sub-anaesthetic dose of propofol. This study revealed that LC noradrenergic neurons might play a critical role in the hedonic effects induced by a subanaesthetic dose of propofol (Chen et al. Citation2021), providing evidence for propofol addiction. However, this study did not address specific neurotransmitters and their receptors, and the underlying mechanisms may require further investigation.
Nitrergic System
Nitric oxide (NO) is a gas with biological activities (Robbins and Grisham Citation1997), and its role in nicotine and morphine addiction has been demonstrated in previous studies (Rambali et al. Citation2002; Bhatt and Kumar Citation2015). Shahzadi et al. revealed that an intraperitoneal injection of propofol induced CPP in rats, and L-NAME, an inhibitor of NOS, lowered the effect of propofol-induced bottoming up CPP (Shahzadi et al. Citation2018). Furthermore, an intraperitoneal injection of propofol significantly produced locomotor sensitisation in rats, whereas an intraperitoneal injection of L-NAME significantly reduced this behaviour (Tekeli et al. Citation2020). Their studies further confirmed that propofol is prone to causing addiction and revealed the role of NO in the process of addiction.
Discussion
Propofol has a wide range of applications in clinical anaesthesia and treatment (Takizawa et al. Citation2005; Wilson et al. Citation2010; Abu Baker et al. Citation2019; Tekeli et al. Citation2020). Meanwhile, there have been increasing reports of propofol abuse and addictive potential in recent years (Follette and Farley Citation1992; Arias et al. Citation2011). This potential for propofol abuse and addiction has placed new demands on its clinical applications. In terms of the mechanism of addiction, this review focused on the mesolimbic, noradrenergic, and nitrergic systems to clarify the mechanism of propofol addiction. The mesolimbic system, including the NAc, VTA, and BLA, is strongly related to drug addiction (Xiong et al. Citation2011). Previous studies have indicated that the GABAR, GR, NMDAR, A2AR, and CRFR play important roles in the formation and maintenance of propofol addiction (Yang et al. Citation2011; Wu et al. Citation2016, Citation2018; Wang et al., Citation2018a; Chen et al. Citation2020; Dong et al. Citation2021a, Citation2021b) Meanwhile, the dopaminergic pathway, ERK signalling pathway, and VTA-NAC circuit explain the internal relationship between neurotransmitters and receptors in the brain during the formation and maintenance of addiction (Li et al. Citation2008; Xiong et al. Citation2011; Lian et al. Citation2013; Wang et al. Citation2016, Citation2018b), which will enable people to understand the mechanism of propofol addiction further. In addition, studies have shown that the noradrenergic and nitrergic systems are also involved in the formation and maintenance of propofol addiction (Shahzadi et al. Citation2018; Tekeli et al. Citation2020; Chen et al. Citation2021), although more evidence is still needed to explain this phenomenon. Here, we summarised the relevant neurotransmitters and pathways in Supplemental Table 1.
Despite many published studies, there are still many deficiencies in the research on the mechanism of propofol addiction. The acute use of addictive drugs induces pleasant states or pain relief (Camí and Farré Citation2003), a phenomenon that has been demonstrated in clinical and animal studies and may be related to an acute increase in dopamine and dopamine receptors (Li et al. Citation2008; Kim et al. Citation2015). Repeated use of addictive drugs can lead to adaptive changes in the central nervous system, thus leading to psychological dependence (including seeking, sensation, reward, relapse et al.) and physical dependence (including tolerance, withdrawal syndrome et al.) (Camí and Farré Citation2003). Currently, most studies focus on propofol psychological dependence and explore the role of transmitters and receptors in propofol seeking, sensitisation, reward and relapse through self-administration, CPP, drug discrimination, and behavioural sensitisation experiments (Supplemental Table 1). However, few studies have been done on physical dependence, including tolerance and withdrawal responses. We believe that the study of physical dependence on propofol may have a certain guiding role in clinical drug use. In terms of the mechanism of psychological dependence, micro-RNA has been suggested to play a regulatory role in alcohol dependence and have an influencing relationship with D1R and ΔFosB (Li et al. Citation2013). However, whether this mechanism exists in propofol dependence still needs further investigation.
In terms of neural pathways, the role of the VTA-BLA pathway in propofol addiction is also worth exploring. Nguyen et al. have shown that nicotine promotes the projection of dopamine neurons from VTA to NAc and inhibits the projection of dopamine neurons from VTA to amygdala (Nguyen et al. Citation2021). Moreover, previous studies have revealed that the mPFC-NAc, VP-VTA, and NAc-VP circuits are involved in cocaine seeking (Berglind et al. Citation2009; Stefanik et al. Citation2013; Mahler et al. Citation2014). Here, we depict the proven pathway and potential pathways in Figure . However, whether those effects also occur with propofol is unknown, and few current studies have addressed this aspect.
Figure 1. The role of different neurotransmitters, VTA-NAc circuit, and other possible circuits in propofol addiction. NAc, nucleus accumbens; VTA, ventral tegmental area; BLA, basolateral amygdala; LC, locus coeruleus; VP, ventral pallidum; mPFC, medial prefrontal cortex; vHipp, ventral hippocampus.
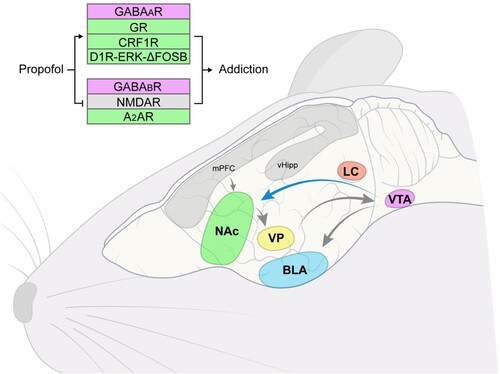
Conclusion
In summary, the combined action of multiple transmitters and receptors, signalling pathways, and neural circuits lead to the formation and maintenance of propofol addiction. Further research on the mechanism of addiction will enable people to better target the characteristics of the specific population prevention and treatment work.
Author contributions
Tianhao Zhong, Bingwu Huang and Ruifeng Zeng involved in the conception and design; Tianhao Zhong and Yuyan Lin drafted the paper, Ruohuai ZhuGe and Yujie Lin revised it critically for intellectual content; Ruifeng Zeng approved the version to be published. All authors agree to be accountable for all aspects of the work.
Supplemental Material
Download MS Word (17.7 KB)Acknowledgements
We acknowledge Editage (www.editage.cn) for English language editing.
Disclosure statement
No potential conflict of interest was reported by the author(s).
Data availability
Data sharing does not apply to this article as no new data were created or analysed in this study.
Additional information
Funding
References
- Abu Baker F, Mari A, Aamarney K, Ras Hakeem A, Ovadia B, Kopelman Y. 2019. Propofol sedation in colonoscopy: from satisfied patients to improved quality indicators. Clin Exp Gastroenterol. 12:105–110. doi:10.2147/CEG.S186393.
- Al-Attraqchi OHA, Attimarad M, Venugopala KN, Nair A, Al-Attraqchi NHA. 2019. Adenosine A2A receptor as a potential drug target – current status and future perspectives. Curr Pharm Des. 25:2716–2740. doi:10.2174/1381612825666190716113444.
- Arias SA, Cohen P, Kwon JS. 2011. Clozapine-induced lymphocytic alveolitis. Am J Psychiatry. 168:210–211. doi:10.1176/appi.ajp.2010.10101494.
- Bale TL, Vale WW. 2004. CRF and CRF receptors: role in stress responsivity and other behaviors. Annu Rev Pharmacol Toxicol. 44:525–557. doi:10.1146/annurev.pharmtox.44.101802.121410.
- Berglind WJ, Whitfield TW, Jr., LaLumiere RT, Kalivas PW, McGinty JF. 2009. A single intra-PFC infusion of BDNF prevents cocaine-induced alterations in extracellular glutamate within the nucleus accumbens. J Neurosci. 29:3715–3719. doi:10.1523/JNEUROSCI.5457-08.2009.
- Bhatt K, Kumar A. 2015. Mechanism of morphine addiction by inhibiting the soluble Guanylate Cyclase–Nitric Oxide (sGC–NO) pathway. Math Biosci. 266:85–92. doi:10.1016/j.mbs.2015.06.004.
- Bonnet U, Scherbaum N. 2012. Craving dominates propofol addiction of an affected physician. J Psychoactive Drugs. 44:186–190. doi:10.1080/02791072.2012.684635.
- Bormann J, Feigenspan A. 1995. GABAc receptors. Trends Neurosci. 18:515–519. doi:10.1016/0166-2236(95)98370-E.
- Brechmann T, Maier C, Kaisler M, Vollert J, Schmiegel W, Pak S, et al. 2018. Propofol sedation during gastrointestinal endoscopy arouses euphoria in a large subset of patients. United European Gastroenterol J. 6:536–546. doi:10.1177/2050640617736231.
- Camí J, Farré M. 2003. Drug addiction. N Engl J Med. 349:975–986. doi:10.1056/NEJMra023160.
- Chen BP, Huang XX, Dong DM, Wu H, Zhu TQ, Wang BF. 2020. The role of NMDA receptors in rat propofol self-administration. BMC Anesthesiol. 20:149. doi:10.1186/s12871-020-01056-0.
- Chen H, Xu D, Zhang Y, Yan Y, Liu J, Liu C, et al. 2021. Neurons in the locus coeruleus modulate the hedonic effects of sub-anesthetic dose of propofol. Front Neurosci. 15:636901. doi:10.3389/fnins.2021.636901.
- Corbillé AG, Valjent E, Marsicano G, Ledent C, Lutz B, Hervé D, et al. 2007. Role of cannabinoid type 1 receptors in locomotor activity and striatal signaling in response to psychostimulants. J Neurosci. 27:6937–6947. doi:10.1523/JNEUROSCI.3936-06.2007.
- Deroche-Gamonet V, Sillaber I, Aouizerate B, Izawa R, Jaber M, Ghozland S, et al. 2003. The glucocorticoid receptor as a potential target to reduce cocaine abuse. J Neurosci. 23:4785–4790. doi:10.1523/JNEUROSCI.23-11-04785.2003.
- Diaz JH, Kaye AD. 2017. Death by propofol. J La State Med Soc. 169:28–32.
- Dong Z, Han H, Wang M, Xu L, Hao W, Cao J. 2006. Morphine conditioned place preference depends on glucocorticoid receptors in both hippocampus and nucleus accumbens. Hippocampus. 16:809–813. doi:10.1002/hipo.20216.
- Dong Z, Huang B, Jiang C, Chen J, Lin H, Lian Q, et al. 2021a. The adenosine A2a receptor activation in nucleus accumbens suppress cue-induced reinstatement of propofol self-administration in rats. Neurochem Res. 46:1081–1091. doi:10.1007/s11064-021-03238-9.
- Dong Z, Zhang G, Xiang S, Jiang C, Chen Z, Li Y, et al. 2021b. The antagonism of corticotropin-releasing factor receptor-1 in brain suppress stress-induced propofol self-administration in rats. Front Behav Neurosci. 15:775209. doi:10.3389/fnbeh.2021.775209.
- Du WJ, Zhang RW, Li J, Zhang BB, Peng XL, Cao S, et al. 2018. The locus coeruleus modulates intravenous general anesthesia of zebrafish via a cooperative mechanism. Cell Rep. 24:3146–3155.e3. doi:10.1016/j.celrep.2018.08.046.
- Eliot LG. 2011. Addiction and brain reward and antireward pathways. Adv Psychosom Med. 30:22–60. doi:10.1159/000324065.
- Follette JW, Farley WJ. 1992. Anesthesiologist addicted to propofol. Anesthesiology. 77:817–818. doi:10.1097/00000542-199210000-00028.
- Haitsma JJ, Lachmann B, Papadakos PJ. 2009. Additives in intravenous anesthesia modulate pulmonary inflammation in a model of LPS-induced respiratory distress. Acta Anaesthesiol Scand. 53:176–182. doi:10.1111/j.1399-6576.2008.01844.x.
- Han E, Jung S, Baeck S, Lee S, Chung H. 2013. Deaths from recreational use of propofol in Korea. Forensic Sci Int. 233:333–337. doi:10.1016/j.forsciint.2013.10.008.
- Havekes R, Heckman PRA, Wams EJ, Stasiukonyte N, Meerlo P, Eisel ULM. 2019. Alzheimer’s disease pathogenesis: The role of disturbed sleep in attenuated brain plasticity and neurodegenerative processes. Cell Signal. 64:109420. doi:10.1016/j.cellsig.2019.109420.
- Higgins GA, Nguyen P, Sellers EM. 1992. The NMDA antagonist dizocilpine (MK801) attenuates motivational as well as somatic aspects of naloxone precipitated opioid withdrawal. Life Sci. 50:PL167–PL172. doi:10.1016/0024-3205(92)90452-U.
- Jameie SB, Mesgar S, Aliaghaei A, Raoofi A, Amini M, Khodagholi F, et al. 2019. Neuroprotective effect of exogenous melatonin on the noradrenergic neurons of adult male rats’ locus coeruleus nucleus following REM sleep deprivation. J Chem Neuroanat. 100:101656. doi:10.1016/j.jchemneu.2019.101656.
- Kim EJ, Kim SH, Hyun YJ, Noh YK, Jung HS, Han SY, et al. 2015. Clinical and psychological characteristics of propofol abusers in Korea: A survey of propofol abuse in 38, non-healthcare professionals. Korean J Anesthesiol. 68:586–593. doi:10.4097/kjae.2015.68.6.586.
- Köroğlu G, Tezcan AH. 2015. A case report of the first propofol addiction in Turkey. Turk J Anesthesiol Reanim. 43:434–436. doi:10.5152/TJAR.2015.54872.
- Kumar K, Sharma S, Kumar P, Deshmukh R. 2013. Therapeutic potential of GABAB receptor ligands in drug addiction, anxiety, depression and other CNS disorders. Pharmacol Biochem Behav. 110:174–184. doi:10.1016/j.pbb.2013.07.003.
- Lee J. 2012. Propofol abuse in professionals. J Korean Med Sci. 27:1451–1452. doi:10.3346/jkms.2012.27.12.1451.
- Li H, Lu Y, Pang Y, Li M, Cheng X, Chen J. 2017. Propofol enhances the cisplatin-induced apoptosis on cervical cancer cells via EGFR/JAK2/STAT3 pathway. Biomed Pharmacother. 86:324–333. doi:10.1016/j.biopha.2016.12.036.
- Li J, Li J, Liu X, Qin S, Guan Y, Liu Y, et al. 2013. MicroRNA expression profile and functional analysis reveal that miR-382 is a critical novel gene of alcohol addiction. EMBO Mol Med. 5:1402–1414. doi:10.1002/emmm.201201900.
- Li KY, Xiao C, Xiong M, Delphin E, Ye JH. 2008. Nanomolar propofol stimulates glutamate transmission to dopamine neurons: a possible mechanism of abuse potential? J Pharmacol Exp Ther. 325:165–174. doi:10.1124/jpet.107.132472.
- Lian Q, Wang B, Zhou W, Jin S, Xu L, Huang Q, et al. 2013. Self-administration of propofol is mediated by dopamine D1 receptors in nucleus accumbens in rats. Neuroscience. 231:373–383. doi:10.1016/j.neuroscience.2012.11.002.
- Linker KE, Gad M, Tawadrous P, Cano M, Green KN, Wood MA, et al. 2020. Microglial activation increases cocaine self-administration following adolescent nicotine exposure. Nat Commun. 11:306. doi:10.1038/s41467-019-14173-3.
- Liu Y, Lin D, Wu B, Zhou W. 2016. Ketamine abuse potential and use disorder. Brain Res Bull. 126:68–73. doi:10.1016/j.brainresbull.2016.05.016.
- Mahler SV, Vazey EM, Beckley JT, Keistler CR, McGlinchey EM, Kaufling J, et al. 2014. Designer receptors show role for ventral pallidum input to ventral tegmental area in cocaine seeking. Nat Neurosci. 17:577–585. doi:10.1038/nn.3664.
- Maier C, Iwunna J, Tsokos M, Mußhoff F. 2017. Deaths from propofol abuse: survey of institutes of forensic medicine in Germany, Austria and Switzerland. Anaesthesist. 66:109–114. doi:10.1007/s00101-016-0260-6.
- Mameli M, Halbout B, Creton C, Engblom D, Parkitna JR, Spanagel R, et al. 2009. Cocaine-evoked synaptic plasticity: persistence in the VTA triggers adaptations in the NAc. Nat Neurosci. 12:1036–1041. doi:10.1038/nn.2367.
- Margolis EB, Toy B, Himmels P, Morales M, Fields HL. 2012. Identification of rat ventral tegmental area GABAergic neurons. PLOS ONE. 7:e42365. doi:10.1371/journal.pone.0042365.
- McAuliffe PF, Gold MS, Bajpai L, Merves ML, Frost-Pineda K, Pomm RM, et al. 2006. Second-hand exposure to aerosolized intravenous anesthetics propofol and fentanyl may cause sensitization and subsequent opiate addiction among anesthesiologists and surgeons. Med Hypotheses. 66:874–882. doi:10.1016/j.mehy.2005.10.030.
- McCutcheon JE, Wang X, Tseng KY, Wolf ME, Marinelli M. 2011. Calcium-permeable AMPA receptors are present in nucleus accumbens synapses after prolonged withdrawal from cocaine self-administration but not experimenter-administered cocaine. J Neurosci. 31:5737–5743. doi:10.1523/JNEUROSCI.0350-11.2011.
- Merlo LJ, Goldberger BA, Kolodner D, Fitzgerald K, Gold MS. 2008. Fentanyl and propofol exposure in the operating room: sensitization hypotheses and further data. J Addict Dis. 27:67–76. doi:10.1080/10550880802122661.
- Monroe T, Hamza H, Stocks G, Scimeca PD, Cowan R. 2011. The misuse and abuse of propofol. Subst Use Misuse. 46:1199–1205. doi:10.3109/10826084.2011.564705.
- Morisot N, Ron D. 2017. Alcohol-dependent molecular adaptations of the NMDA receptor system. Genes Brain Behav. 16:139–148. doi:10.1111/gbb.12363.
- Nathan L, Charles JM. 2019. Extracellular Signal-Regulated Kinase: a regulator of cell growth, inflammation, chondrocyte and bone cell receptor-mediated gene expression. Int J Mol Sci. 20:3792. doi:10.3390/ijms20153792.
- Nestler EJ, Barrot M, Self DW. 2001. Δfosb: A sustained molecular switch for addiction. Proc Natl Acad Sci USA. 98:11042–11046. doi:10.1073/pnas.191352698.
- Nestler EJ, Carlezon WA, Jr. 2006. The mesolimbic dopamine reward circuit in depression. Biol Psychiatry. 59:1151–1159. doi:10.1016/j.biopsych.2005.09.018
- Nguyen C, Mondoloni S, Le Borgne T, Centeno I, Come M, Jehl J, et al. 2021. Nicotine inhibits the VTA-to-amygdala dopamine pathway to promote anxiety. Neuron. 109:2604–2615.e9. doi:10.1016/j.neuron.2021.06.013.
- Rambali B, Vleeming W, Opperhuizen A. 2002. The role of nitric oxide in cigarette smoking and nicotine addiction. Nicotine Tob Res. 4:341–348. doi:10.1080/14622200210142724.
- Reiss AB, Grossfeld D, Kasselman LJ, Renna HA, Vernice NA, Drewes W, et al. 2019. Adenosine and the cardiovascular system. Am J Cardiovasc Drugs. 19:449–464. doi:10.1007/s40256-019-00345-5.
- Robbins RA, Grisham MB. 1997. Nitric oxide. Int J Biochem Cell Biol. 29:857–860. doi:10.1016/S1357-2725(96)00167-7.
- Sahinovic MM, Struys MMRF, Absalom AR. 2018. Clinical pharmacokinetics and pharmacodynamics of propofol. Clin Pharmacokinet. 57:1539–1558. doi:10.1007/s40262-018-0672-3.
- Schenk S, Valadez A, Worley CM, McNamara C. 1993. Blockade of the acquisition of cocaine self-administration by the NMDA antagonist MK-801 (dizocilpine). Behav Pharmacol. 4:652–659. doi:10.1097/00008877-199312000-00011.
- Seif T, Makriyannis A, Kunos G, Bonci A, Hopf FW. 2011. The endocannabinoid 2-arachidonoylglycerol mediates D1 and D2 receptor cooperative enhancement of rat nucleus accumbens core neuron firing. Neuroscience. 193:21–33. doi:10.1016/j.neuroscience.2011.07.055.
- Shahzadi A, Uskur T, Akkan AG, Çevreli B, Uzbay T. 2018. Effects of propofol on conditioned place preference in male rats: involvement of nitrergic system. Am J Drug Alcohol Abuse. 44:167–174. doi:10.1080/00952990.2017.1344681.
- Sinha R, Jastreboff AM. 2013. Stress as a common risk factor for obesity and addiction. Biol Psychiatry. 73:827–835. doi:10.1016/j.biopsych.2013.01.032.
- Stefanik MT, Kupchik YM, Brown RM, Kalivas PW. 2013. Optogenetic evidence that pallidal projections, not nigral projections, from the nucleus accumbens core are necessary for reinstating cocaine seeking. J Neurosci. 33:13654–13662. doi:10.1523/JNEUROSCI.1570-13.2013.
- Stephens DN, King SL, Lambert JJ, Belelli D, Duka T. 2017. GABAA receptor subtype involvement in addictive behaviour. Genes Brain Behav. 16:149–184. doi:10.1111/gbb.12321.
- Stocks G. 2011. Abuse of propofol by anesthesia providers: The case for re-classification as a controlled substance. J Addict Nurs. 22:57–62. doi:10.3109/10884602.2010.545091.
- Takizawa D, Sato E, Hiraoka H, Tomioka A, Yamamoto K, Horiuchi R, et al. 2005. Changes in apparent systemic clearance of propofol during transplantation of living related donor liver. Br J Anaesth. 95:643–647. doi:10.1093/bja/aei243.
- Tekeli AE, Oğuz AK, TunÇdemir YE, Almali N. 2020. Comparison of dexmedetomidine-propofol and ketamine-propofol administration during sedation-guided upper gastrointestinal system endoscopy. Medicine (Baltimore). 99:e23317. doi:10.1097/MD.0000000000023317.
- Valjent E, Pagès C, Hervé D, Girault JA, Caboche J. 2004. Addictive and non-addictive drugs induce distinct and specific patterns of ERK activation in mouse brain. Eur J Neurosci. 19:1826–1836. doi:10.1111/j.1460-9568.2004.03278.x.
- Walsh PD, Abernethy KA, Bermejo M, Beyers R, De Wachter P, Akou ME, et al. 2003. Catastrophic ape decline in western equatorial Africa. Nature. 422:611–614. doi:10.1038/nature01566.
- Wang B, Lv K, Liu H, Su Y, Wang H, Wang S, et al. 2018a. Contribution of the α5 GABAA receptor to the discriminative stimulus effects of propofol in rat. NeuroReport. 29:347–352. doi:10.1097/WNR.0000000000000959.
- Wang B, Yang X, Sun A, Xu L, Wang S, Lin W, et al. 2016. Extracellular signal-regulated kinase in nucleus accumbens mediates propofol self-administration in rats. Neurosci Bull. 32:531–537. doi:10.1007/s12264-016-0066-1.
- Wang S, Wang X, Lin W, Bao S, Wang B, Wu B, et al. 2018b. Dopamine D1 receptor within basolateral amygdala is involved in propofol relapse behavior induced by cues. Neurochem Res. 43:2393–2403. doi:10.1007/s11064-018-2667-8.
- Wang Y, Tian D, Wei C, Cui V, Wang H, Zhu Y, et al. 2020. Propofol attenuates α-synuclein aggregation and neuronal damage in a mouse model of ischemic stroke. Neurosci Bull. 36:289–298. doi:10.1007/s12264-019-00426-0.
- Wen D, Hui R, Liu Y, Luo Y, Wang J, Shen X, et al. 2020. Molecular hydrogen attenuates methamphetamine-induced behavioral sensitization and activation of ERK-ΔFosB signaling in the mouse nucleus accumbens. Prog Neuropsychopharmacol Biol Psychiatry. 97:109781. doi:10.1016/j.pnpbp.2019.109781.
- Wilson C, Canning P, Caravati EM. 2010. The abuse potential of propofol. Clin Toxicol (Phila). 48:165–170. doi:10.3109/15563651003757954.
- Wu B, Liang Y, Dong Z, Chen Z, Zhang G, Lin W, et al. 2016. Glucocorticoid receptor mediated the propofol self-administration by dopamine D1 receptor in nucleus accumbens. Neuroscience. 328:184–193. doi:10.1016/j.neuroscience.2016.04.029.
- Wu B, Lin W, Wang H, Abdullah T, Wang B, Su Y, et al. 2018. Glucocorticoid receptor in rat nucleus accumbens: Its roles in propofol addictions. Neurosci Lett. 662:115–121. doi:10.1016/j.neulet.2017.10.011.
- Xiong M, Li J, Ye JH, Zhang C. 2011. Upregulation of DeltaFosB by propofol in rat nucleus accumbens. Anesth Analg. 113:259–264. doi:10.1213/ANE.0b013e318222af17.
- Yang B, Wang BF, Lai MJ, Zhang FQ, Yang XW, Zhou WH, et al. 2011. Differential involvement of GABAA and GABAB receptors in propofol self-administration in rats. Acta Pharmacol Sin. 32:1460–1465. doi:10.1038/aps.2011.123.
- Zacny JP, Lichtor JL, Thompson W, Apfelbaum JL. 1993a. Propofol at a subanesthetic dose may have abuse potential in healthy volunteers. Anesth Analg. 77:544–552. doi:10.1213/00000539-199309000-00020.
- Zacny JP, Lichtor JL, Zaragoza JG, Coalson DW, Uitvlugt AM, Flemming DC, et al. 1993b. Assessing the behavioral effects and abuse potential of propofol bolus injections in healthy volunteers. Drug Alcohol Depend. 32:45–57. doi:10.1016/0376-8716(93)90021-H.
- Zeng J, Li YK, Quan FF, Zeng X, Chen CY, Zeng T. 2020. Propofol-induced miR-125a-5p inhibits the proliferation and metastasis of ovarian cancer by suppressing LIN28B. Mol Med Rep. 22:1507–1517. doi:10.3892/mmr.2020.11223.
- Zhang YF, Li CS, Zhou Y, Lu XH. 2020. Propofol facilitates cisplatin sensitivity via lncRNA MALAT1/miR-30e/ATG5 axis through suppressing autophagy in gastric cancer. Life Sci. 244:117280. doi:10.1016/j.lfs.2020.117280.
- Zhou Y, Qiu Y. 2019. Propofol alleviates cisplatin-related cognitive impairment. Neurol Sci. 40:1645–1649. doi:10.1007/s10072-019-03895-3.