Abstract
The rhizosphere of grown tea (Camellia sinensis (L.) O. Kuntze) becomes acidic, and aluminium (Al) toxicity has been identified as a key constraint on plant development in acidic soils. The current study investigated the composition and functions of rhizosphere bacteria as well as soil enzyme activity under different Al stress conditions. The effect of soil pH, soil enzyme activities, the diversity and structure of the rhizosphere bacterial population under various Al concentrations were studied by the pot experiment. All analyses demonstrated that substantial alterations in the activity of soil enzymes and the composition of the bacterial communities in the rhizosphere could only be seen under conditions of extreme Al stress. Soil enzymes were more concentrated in Al concentrations of 100, 150 and 250 µM, respectively. More than 82,000 valid reads were obtained for each replicate, and the abundance of certain genera in the aluminium treated groups were greater than the control group. Lower Al stress attracted Al-tolerant bacteria such as Burkholderia to increase Al stress resistance. These findings revealed that soil enzyme activities and the structure of the rhizosphere bacterial population in tea orchard soil provided tolerance or resistance capability to tea plants for their development under Al stress.
Introduction
Tea trees (Camellia sinensis (L.) O. Kuntze) are among the most important economic trees in the world, as an important economic crop, which is widely planted in acidic soil in the tropical and sub-tropical zones of China (Liu et al. Citation2019). Meanwhile, tea is an aluminium (Al) accumulating plant that grows well in highly acidic soils with high levels of Al. However, extensive and long-term cultivation of tea plants causes soil acidification and worsens acidification as cultivation time passes (Yang et al. Citation2018; Sun et al. Citation2020; Zhou, Chen et al. Citation2022). At soil pH levels of 5 or lower, the octahedral hexahydrate Al (H2O)3+, also known as A13+, is solubilized into the soil solution, limiting root development and function and lowering crop yields, according to research (Chen and Liao Citation2016). Long-term monoculture of tea bushes has resulted in significant yield loss and soil degradation, which have become major issues in the sustainable production of tea orchards (Murni Citation2020; Yu et al. Citation2020). Several studies have well documented the soil degradation of tea orchards in terms of physicochemical characteristics, such as decreasing soil pH, Al accumulation and nutrient leaching (Yan et al. Citation2020). Meanwhile, extensive research on Al toxicity, soil biota loss and organic amendment has been conducted in tea orchard systems (Ruan et al. Citation2004; Han et al. Citation2007; Peng et al. Citation2018; Liu et al. Citation2022).
Plant growth promoting rhizobacteria (PGPR) belong to a group of useful bacteria that live in close association with plant rhizosphere and are very important participators in nutrient cycling (Basu et al. Citation2021), also promote plant growth and adaptability to the environment (Khan et al. Citation2021) and escape from pathogenic bacteria (Fallah et al. Citation2021). When fenugreek plants were treated with nanocompounds and PGPR, a substantial increase (1.5–2 times) in plant height, leaf number, leaf area and fresh weight over control was seen (Kumari et al. Citation2020). The best bioinoculants are PGPR and they may create an environment that is conducive to plant growth; the rhizospheres’ microbiome has a greater microbial population than bulk soil and has a major impact on the quality of both plants and soil (Chaudhary et al. Citation2021, Citation2022). Some common PGPR viz. Pseudomonas fluorescens, Bacillus spp., Pantoea agglomerans, Burkholderia spp. etc. are reported to be involved in solubilization of essential minerals, production of plant hormones and antimicrobials and developing resistance in plants (Baas et al. Citation2016; Chaudhary and Sharma Citation2019; Khati et al. Citation2019). Chaudhary et al. (Citation2021) suggested that combined application of nanogypsum and Pseudomonas taiwanensis in maize help in improving the structure and function of soil which affects the plant health without causing any toxic effect. Currently, study is concentrated on the functional diversity of the soil bacteria, fungus, actinomycetes and arbuscular mycorrhizal fungi from the rhizosphere of tea plants (Santhanam et al. Citation2015). Additionally, other researchers have studied how environmental conditions affect the soil bacteria in the tea tree’s rhizosphere. Studies have shown that the lengthening of planting seasons can intensify soil acidification, and that soil pH influences the build-up of Al, which in turn impacts the variety and number of rhizosphere soil microbes (Wan et al. Citation2018; Wang et al. Citation2018). Therefore, understanding the dynamic influence of Al on the microbial community in rhizosphere of tea plants is essential to improve the growth of tea plants.
The quantity of root exudates secreted from stress-tolerant and stress-sensitive genotypes is exceptional underneath Al stress (Chen and Liao Citation2016). Many of the plant growth-promoting microorganisms and fungi recruited by way of the root, such as Rhizobium, Pseudomonas and Fusarium, produce compounds that notably decorate the secretion of exudate in the roots and in consequence have an effect on the tolerance of flora to Al toxicity (Jiang et al. Citation2021; Chai and Schachtman Citation2022). Some Al-tolerant bacteria in the soil, such as Klebsiella, Stenotrophomonas, Serratia and Enterobacter, alleviate Al stress via the formation of Al-siderophore complexes and enhance efficiency (Mora et al. Citation2017). Meanwhile, Al severely impeded the capability of the soil microbe, particularly Pseudomonas fluorescens, to perform oxidative phosphorylation. Most research of Al outcomes on tea plants have focused on the stimulatory effects of Al on plant growth (Devi et al. Citation2020; Pan et al. Citation2020; Hao et al. Citation2022). However, little information is accessible on rhizosphere bacterial community in tea plant handled with Al. Therefore, the study of the effects of Al toxicity on the rhizosphere microorganisms of tea trees is helpful to understand the adaptation of tea trees to Al toxicity.
As 99% of the microbial population is unculturable due to some restrictions in culturing methods, the microbial community can be focused on in depth using a metagenomics approach. It gives access to an uncharted world of diverse microorganisms from odd settings (Agri et al. Citation2022). Therefore, this study used Illumina MiSeq sequencing to evaluate the effects of different doses of Al on soil pH, enzyme activity and microbial characteristics. In this paper, we propose a scientific hypothesis that Al in the pH range improves soil acidification and stimulates soil microbial activity. This study suggests that it may want to be utilized as a good-sized administration strategy, and will furnish insights into the interior mechanisms whereby tea plant cultivation led to soil acidification.
Materials and methods
Plant cultivation
Two-year-old rooted cuttings of tea cultivar ‘Fuding Dabaicha’ (Camallia sinensis (L.) O. Kuntze cv. ‘Fuding Dabaicha’), from a tea plantation in Fuding, Fujian Province, China (GPS: 25°5′N, 118°13′E, Altitude: 65 m), were chosen for the present study. The cuttings were cleaned free of soil by gently removing the soil around the roots to minimize root damage and washing the roots three times with tap water, followed by three washes with distilled water. Uniform plants were selected and mounted in plastic lids placed on 1-L bucket, with nine plants per bucket. The buckets contained nutrient solution (Table S1) as per protocol of Wan et al. (Citation2012). Tea plants were soaked in tap water for three days before being subjected to a one-third-strength nutrient solution missing Al for one week, a half-strength nutritional solution for one week, a three-quarter-strength nutrient solution for one week, and a full-strength nutrient solution for one week. When new white roots had developed, the plants were moved from the nutrient solution to the soil. The groups of N-Soil, O-Soil, F-Soil, T-Soil and R-Soil were treated with 0, 50, 100, 150 and 250 µM of AlCl3 solution respectively, and the same volume of solution was applied to the soil. The culture experiments were 72 h in a room with three replicates per treatment.
A cubic 15 cm steel box was used to gather soil samples at random. All soils were agitated in a tray to separate them into rhizosphere and bulk soils (Chen et al. Citation2015). The soil attached within 4 mm of roots after gentle hand-shaking is defined as rhizosphere soil (Liu et al. Citation2013). The rhizosphere soils were immediately returned to the laboratory, where they were separated into two duplicates, air-dried at room temperature, and kept in a refrigerator at 4°C. The air-dried soil was sifted through a 0.2-mm screen and kept in a plastic bottle until the soil general characteristics and Al fractions were determined. The bacterial community in new soil samples was studied.
Soil enzyme activity characterization
Soil urease, invertase and cellulose activities were analysed according to a previous report (Sun et al. Citation2014). Soil urease activity was determined using improved sodium phenate and sodium hypochlorite colorimetry. Invertase (Antonious et al. Citation2020) and cellulase (Wang et al. Citation2011) activities were estimated calorimetrically by determining the reduction of 3,5-dinitrosalicylic acid from reducing sugar after the soil was incubated with a buffered sucrose and sodium carboxymethyl-cellulose solution and toluene at 37°C for 24 and 72 h, respectively.
Soil DNA extraction
For one treatment, three duplicate samples were chosen at random and utilized for DNA extraction. Soil DNA was extracted from 1 g of sieved soil using a Fast DNA SPIN Kit for soil (MP Biomedicals, Santa Ana, CA), as directed by the manufacturer. The extracted soil DNA was dissolved in 100 ml TE buffer, quantified by NanoDrop and stored at −70°C before use (Sun et al. 2014).
Bacterial 16S rRNA gene amplification and Illumina Sequencing
We employed the procedure outlined in Caporaso et al. (Citation2010) to examine the diversity and composition of the rhizosphere bacterial communities in each of these samples. The 515f/806r primer pair, which amplifies the V4 region of the 16S rDNA gene, was used for PCR amplifications. The primer set was chosen because it has low biases and should produce reliable phylogenetic and taxonomy information. The reverse primer contained a 6-bp error-correcting barcode unique to each sample. DNA was amplified following the protocol described previously (Magoc and Salzberg Citation2011). Amplicon pyrosequencing was performed on the Illumina MiSeq platforms at Biomarker Technologies Co., Ltd., Beijing, China. Pairs of reads from the original DNA fragments were merged by using FLASH tool (Caporaso et al. Citation2010) which was designed to merge pairs of reads when the original DNA fragments were shorter than twice the length of reads. Sequencing reads were assigned to each sample according to the unique barcode of each sample. Sequences were analysed with the QIIME (Wang et al. Citation2010) software package (Quantitative Insights Into Microbial Ecology) and UPARSE pipeline (Edgar Citation2013), in addition to custom Perl scripts to analyse alpha (within sample) and beta (between sample) diversity. Then we used UPARSE pipeline to pick operational taxonomic units (OTUs) through making OTUs table. Sequences were assigned to OTUs at 97% similarity. We picked a representative sequence for each OTU and used the ribosomal database project (RDP) classifier (Caporaso et al. Citation2011) to assign taxonomic data to each representative sequence. We rarified the OTU database and derived three measures to quantify alpha diversity. The Chao1 measure indicates richness, while the Observed OTUs metric simply counts the number of distinct OTUs observed in the sample and the Shannon index.
Statistical and bioinformatics analysis
The R environment v.4.1.2 was employed to process the raw sequencing data to get the heatmap figures. A principal component analysis (PCA) was conducted using Canoco 4.5. Analysis of variance and Spearman’s rank correlations were performed using SPSS Statistics 18. Genetic functions of the bacterial communities were predicted by mothur (http://www.mothur.org/). The histogram was created using Microsoft Excel 2010. Significance was accepted at P < .05, unless otherwise noted.
Results
pH and enzyme activity of different rhizosphere soils
After 72 h of incubation period, the pH value of all treatment soils except O-Soil was significantly lower than N-Soil (P < .05), with the lowest value of 3.53 in the group of R-Soil (Table ). Invertase activity was highest in T-Soil, which was significantly higher than that in N-Soil (4.57-fold), O-Soil (1.77-fold) and R-Soil (1.29-fold). Urease activity in F-Soil, T-Soil and R-Soil soils was significantly higher compared to that in N-Soil and O-Soil. Whereas, no significant differences in urease activity in F-Soil, T-Soil and R-Soil which were 64.71%, 69.5% and 67.6% higher than that in N-Soil were observed (Table ). Cellulase activities in N-Soil and O-Soil were lower than in F-Soil, T-Soil and R-Soil. Moreover, cellulase activity in T-Soil was significantly higher than that in R-Soil. Collectively, the concentration of Al affects pH, invertase activity and urease activity.
Table 1. The effects of soil pH, invertase activity, urease activity and cellulase activity in different treatments.
Richness of rhizosphere bacterial community
More than 82,000 valid reads were obtained for each replicate through a sequence optimization process, and the bacterial community richness index was calculated as shown in Table . After quality filtering, median sequence length of each read was 450 bp. Tags with 97% similarity were then grouped into OTUs by the RDP classifier method. 1104 (N-Soil), 1623 (O-Soil), 1449 (F-Soil), 1363 (T-Soil) and 1406 (R-Soil) OTUs were obtained, and 905 existed in all groups (Figure ). However, some microbes might have remained undetermined. OTUs were significantly different in O-Soil sample. More than 260 additional OTUs were observed compared with T-Soil. Higher ACE estimator and Chao 1 indices in O-Soil sample indicated the higher diversity of bacterial community. Whereas, Shannon indices in treatment soils were not significantly different, indicating OTU abundances among community members were approximately equally distributed and no significant difference (Table ).
Table 2. Comparison of the estimated richness, diversity indexes and Pielou’s evenness at the operational taxonomic unit (OTU) level.
Taxonomic coverage of bacterial community
All sequences were classified into 29 phyla by the mother programme. The overall bacterial composition in the different samples was similar, while the distribution of each phylum varied (Figure ). In all samples >85% of the five most dominant phyla such as Proteobacteria, Acidobacteria, Chloroflexi, Actinobacteria and Gemmatimonadetes were observed. Compared to other samples, O-Soil and F-Soil had a significantly higher percentage of Bacteroidetes (1.61–4.11 fold), Gemmatimonadetes (1.66–6.85 fold), Nitrospirae (3.80–12.25 fold), Verrucomicrobia (1.94–5.69 fold), and a lower percentage of Acidobacteria (F-Soil: 17.84%, O-Soil: 22.71%, R-Soil: 27.34%, T-Soil: 28.89%, N-Soil: 29.40%) and WD272 (F-Soil: 0.01%, O-Soil: 0.56%, R-Soil: 2.32%, T-Soil: 2.75%, N-Soil: 1.69%). The maximum percentage of Actinobacteria was found in F-Soil, and the minimum percentage (0.56%) of Gemmatimonadetes (0.56%) was obtained in T-Soil.
Figure 2. Comparison of the bacterial communities at the phylum level (‘Other’ means sequences that could not be classified into any known group).
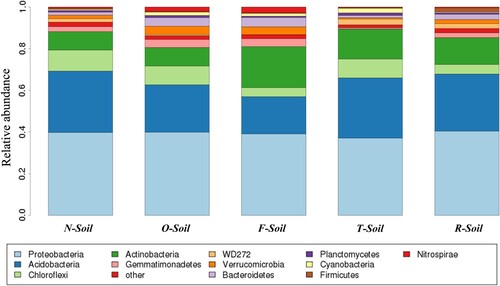
At the genus level, 209 genera were shared by the five samples. The dominant genera were Acidothermus and Rhizomicrobium. And a greater abundance of certain genera within DA111, Acidobacteriaceae Subgroup, Bradyrhizobiaceae, Xanthobacteraceae and Xanthomonadaceae were noted, however, these genera were not grouped into known genera within these families.
Twenty-six genera with significant differences between the samples were listed in Table . In all treatments, Al application had a significant effect on the structure of bacterial community. The abundance of Acidothermus was significantly higher in T-Soil. The abundances of Streptomyces, Haliangium and Rhodanobacter were significantly higher in F-Soil. The abundances of Gemmatimonas, Nitrospira, Dongia and Sphingomonas were higher in O-Soil. The abundances of Rhizomicrobium and Pseudolabrys were higher in R-Soil. Compared with the N-Soil sample, other samples with Al application also resulted in a greater abundance of certain genera within Cytophagaceae, Comamonadaceae, Acetobacteraceae, Nitrosomonadaceae and Xanthomonadaceae, while the abundances of the genera within Acidobacteriaceae Subgroup, Thermosporotrichaceae and Alphai were opposite.
Table 3. The significant different genera among all treatments.
The NMDS at the genus level was performed to further compare the microbiota among the different treatments (Figure ). Data are presented as a 2D plot to better illustrate the relationship. The microbial structures in T-Soil and R-Soil were relatively similar. N-Soil had a significantly lower NMDS value, F-Soil had NMDS positive value, but O-Soil had NMDS2 negative value (Figure ).
LEfSe uses LDA to estimate the effect size of each differentially abundant feature (Segata et al. Citation2011). LEfSe detected 35 bacterial clades showing statistically significant and biologically consistent differences in five treatments. O-Soil had 13 bacterial clades, which was the richest of all treatments, and F-soil had eight bacterial clades as seen in T-Soil. However, N-Soil and R-soil had fewest bacterial clades, at only 3 (Figure (a)). Statistics from order to genera classification showed 23 kinds of significant difference in bacteria had existed on all groups, which were 14 types at order level, 7 types at family level and 2 types at genera level.
Figure 4. LEfSe results on soil bacterial communities. (a) Histogram of the LDA scores computed for features differentially abundant on different site of tea soil. LEfSe scores can be interpreted as the degree of consistent difference in relative abundance between features of analysed bacterial communities. The histogram thus identifies which clades among all those detected as statistically and biologically differential explain the greatest differences between communities. (b) Taxonomic representation of statistically and biologically consistent differences in different site of tea soil. Differences are represented in the colour of the most abundant class (yellow non-significant, other significantly different). Each circle’s diameter is proportional to the taxon’s abundance.
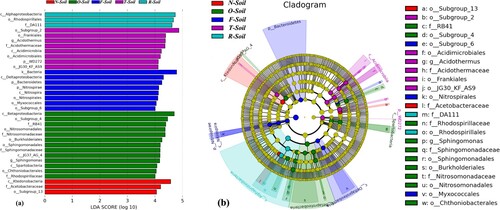
Discussion
Tea is an Al-accumulating plant and grows well in strongly acidic soils containing high levels of Al. Long-term tea cultivation can result in the decline of pH and the accumulation of Al toxicity and antimicrobial substances (Li et al. Citation2016). When the pH of the solution is less than 4.0, the soil will change from a proton buffer system to an Al buffer system, resulting in a sharp increase in the Al content in the soil solution (Szurman-Zubrzycka et al. Citation2021). The pH value in all treatments except O-Soil was significantly lower than that in N-Soil, and there was no significant difference when the Al concentrations were 150 and 250 µM. These results indicated that Al enhanced the accumulation of most nutrient elements in tea plant tissues, and could result in an excess of cations, and the uptake of excess cations over anions per unit whole-plant biomass increased with increasing Al concentration. In previous studies, Al-induced proton release from tea plant roots, leading to acidification in soil. The increase of the initial Al concentration in the culture solutions will lead to the increase of protons released (Wan et al. Citation2012).
Soil enzymes participate in biological cycling and the development of fertility, and are crucial indicators of the soil biochemistry. Previous study suggests that the application of mineral fertilizers improves activities of soil enzymes and physicochemical properties of soil, and macro and micronutrient contents (Jabborova, Sulaymanov et al. Citation2021). Soil invertase is an important factor affecting hydrolysis of sucrose into glucose and fructose. And soil cellulase is involved in breaking down cellulose (Zhang et al. Citation2022). Urease was the key indicator of soil health, as it can catalyze the hydrolysis of urea to produce carbamate and ammonia, and is highly correlative to tree growth, no matter whether the soil type was sandy (Zhou, Monreal, et al. Citation2022) or loam (Savin et al. Citation2021). Co-inoculating crops with plant growth-promoting rhizobacteria and nitrogen-fixing bacteria increases drought tolerance and improves plant growth in semiarid or arid areas (Jabborova, Kannepalli, et al. Citation2021). Therefore, soil urease, invertase and cellulase are ideal indicators of tea orchard maturation. In this study, urease and cellulase activity significantly increased in soil by aluminium treatment except O-Soil. These results indicated that the soil in a certain concentration of Al might support a new and different functional microbial community which was responsible for this apparent increase in mineralization and resulted in a better supply of available nutrients.
We used differential OTUs abundance analysis in the treatments and observed high relative abundance indexes of the OTUs enriched in different treatments (Table ). Our results indicated that the soil had a higher bacterial abundance, Shannon diversity and Chao 1 richness at the Al concentration compared with non-Al. One possible explanation for the increases in bacterial abundance and diversity may be related to the different types and amounts of root exudate secreted from tea plant under Al stress (Chen and Liao Citation2016). Root exudates may mobilize more insoluble nutrients by chelating the occluded cations in soil particles and more rhizosphere microorganisms will definitely increase the amount of enzymes produced, which is beneficial to plants and microbes (Li et al. Citation2011; Martins et al. Citation2021). We observed the five most dominant phyla, such as Proteobacteria, Acidobacteria, Chloroflexi, Actinobacteria and Gemmatimonadetes. Previous research has shown that Proteobacteria can use light cooperation to store energy and degrade harmful substances, and can fix nitrogen and various metabolites (Timmermans et al. Citation2017; Buijs et al. Citation2019; Rothwell et al. Citation2022). Acidobacteria are a widely distributed bacterial phylum, which are commonly found in soil with a low nutrient content and pH. Previous research speculates that Acidobacteria are related to the decomposition of biopolymers, the regulation of biogeochemical cycles, the secretion of extracellular polysaccharides and the promotion of plant growth (Kalam et al. Citation2020; Reji and Zhang Citation2022). In most studies, with an increase in soil pH and soil carbon in the cultivated soil or wet winter, the Acidobacteria relative abundance increased. So, Acinetobacter were related to soil properties (Conradie and Jacobs Citation2020). Chloroflexi assimilate various organic acids from the environment, and play important roles in biogeochemical cycles including nitrogen, carbon, sulphur, and so on. (Speirs et al. Citation2019; Rao et al. Citation2022; Zheng et al. Citation2022). Actinobacteria are a widely distributed bacteria phylum, which play an important role in the cycling of organic compounds and have been associated with soil organic matter production and soil humic acid (Mawang et al. Citation2021). Streptomyces corchorusii of Al-tolerant rhizobacteria Actinobacteria were isolated from rhizosphere in acidic soil, and high content of Al in soil has no effect to Actinobacteria (Silva et al. Citation2013). Gemmatimonadetes were one of the nine most abundant bacterial phyla found in soil, and widely distributed of natural bacterial communities in the environment. They can obtain facultative photoheterotroph of light energy and reduce the powerful greenhouse gas N2O. However, the research of their physiology, ecology and importance in environmental processes is poorly understood (Koblížek et al. Citation2020 Zeng et al. Citation2021; Oshiki et al. Citation2022).
The results of NMDS indicated that the bacterial community structure at high Al stress was relatively similar, but not at low Al stress, resulting in the significant differences of the soil bacterial community structure between different Al concentrations. We found increased diversity within the bacterial community in high Al concentration (200–250 µM), which is similar to the pH of 4.43 in Yang’s research (Yang et al. Citation2012), where they found a higher exchangeable Al content (1.45 cmol kg−1) than the low Al concentration (0.6–0.86 cmol kg−1), which may alter the bacterial community structure (Jiang et al. Citation2021). However, the lowest Al concentration (50 µM) treatment had only 13 bacteria, recruited Al-tolerant microorganisms, such as Burkholderia, to improve tea plant tolerance in the rhizosphere to Al stress, which were not found in other treatments. Studies have documented that Burkholderia enhanced the solubilization of fixed soil phosphorus and produced antimicrobial substances (Kuypers et al. Citation2018; Lian et al. Citation2019).
Conclusions
The present study aimed to determine the influence of Al stress on soil enzyme activities and the rhizosphere bacteria of tea plants. The results explained that Al application decreased the soil pH, and significantly stimulated the activities of soil invertase and urease, and soil microbial populations. Al treatment activated Al-tolerant microorganisms, thereby increasing soil enzyme activity, promoting soil microbial growth and improving soil bacterial communities. These results suggested that Al stress derived different soil enzyme activities and bacterial community structure in tea orchard soil, and soil enzymes and several genera of bacteria likely play important roles in improving tea resistance to Al stress. This study also implied the soil bacterial community was easily influenced by other biotic and abiotic factors. In the future, we will cultivate Al-resistant microorganisms in tea garden soil and conduct in-depth research on the Al-resistant mechanism.
Ethical approval
The authors confirm that there are no ethical issues in the publication of the manuscript.
Supplemental Material
Download MS Word (20.1 KB)Disclosure statement
No potential conflict of interest was reported by the author(s).
Data availability statement
Sequence data are deposited in the National Center for Biotechnology Information (NCBI) Sequence Read Archive (SRA) database [https://www.ncbi.nlm.nih.gov/sra] (PRJNA851941). More details are provided in Data S1 and Data S2. The data that support the findings of this study are available at the figshare repository (https://figshare.com/) at http://doi.org/10.6084/m9.figshare.17162711.v6.
Additional information
Funding
References
- Agri U, Chaudhary P, Sharma A, Kukreti B. 2022. Physiological response of maize plants and its rhizospheric microbiome under the influence of potential bioinoculants and nanochitosan. Plant Soil. 474:451–468.
- Antonious GF, Turley ET, Dawood MH. 2020. Monitoring soil enzymes activity before and after animal manure application. Agriculture. 10:166.
- Baas P, Bell C, Mancini LM, Lee MN, Conant RT, Wallenstein MD. 2016. Phosphorus mobilizing consortium Mammoth P™ enhances plant growth. Peer J. 4:e2121.
- Basu A, Prasad P, Das SN, Kalam S, Sayyed RZ, Reddy MS, El Enshasy H. 2021. Plant growth promoting rhizobacteria (PGPR) as green bioinoculants: recent developments, constraints, and prospects. Sustainability. 13:1140.
- Buijs Y, Bech PK, Vazquez-Albacete D, Bentzon-Tilia M, Sonnenschein EC, Gram L, Zhang SD. 2019. Marine Proteobacteria as a source of natural products: advances in molecular tools and strategies. Nat Prod Rep. 36(9):1333–1350.
- Caporaso JG, Kuczynski J, Stombaugh J, Bittinger K, Bushman FD, Costello EK, Fierer N, Pena AG, Goodrich JK, Gordon FI. 2010. QIIME allows analysis of high-throughput community sequencing data. Nat Methods. 7:335–336.
- Caporaso JG, Lauber CL, Walters WA, Berg-Lyons D, Lozupone CA, Turnbaugh PJ, Fierer N, Knight R. 2011. Global patterns of 16S rRNA diversity at a depth of millions of sequences per sample. PNAS. 108(Suppl 1):4516–4522.
- Chai YN, Schachtman DP. 2022. Root exudates impact plant performance under abiotic stress. Trends Plant Sci. 27(1):80–91.
- Chaudhary P, Chaudhary A, Bhatt P, Kumar G, Khatoon H, Rani A, Kumar S, Sharma A. 2022. Assessment of soil health indicators under the influence of nanocompounds and Bacillus spp. in field condition. Front Environ Sci. 9:769871. doi:10.3389/fenvs.2021.769871.
- Chaudhary P, Khati P, Chaudhary A, Maithani D, Kumar G, Sharma A. 2021. Cultivable and metagenomic approach to study the combined impact of nanogypsum and Pseudomonas taiwanensis on maize plant health and its rhizospheric microbiome. PLoS ONE. 16(4):e0250574.
- Chaudhary P, Sharma A. 2019. Response of nanogypsum on the performance of plant growth promotory bacteria recovered from nanocompound infested agriculture field. Envi Ecol. 37(1B):363–372.
- Chen X, Chen FS, Ye SQ, Yu SQ, Fang XM, Hu XF. 2015. Responses of rhizosphere nitrogen and phosphorus transformations to different acid rain intensities in a hilly red soil tea plantation. Chin J Appl Ecol. 26:1–8.
- Chen ZC, Liao H. 2016. Organic acid anions: an effective defensive weapon for plants against aluminum toxicity and phosphorus deficiency in acidic soils. J Genet Genomics. 43:631–638.
- Conradie T, Jacobs K. 2020. Seasonal and agricultural response of acidobacteria present in two fynbos rhizosphere soils. Diversity. 12:277.
- Devi SS, Saha B, Awasthi JP, Regon P, Kumar PS. 2020. Redox status and oxalate exudation determines the differential tolerance of two contrasting varieties of ‘Assam tea’ [Camelia sinensis (L.) O. Kuntz] in response to aluminum toxicity. Horticulture Environ Biotechnol. 61:485–499.
- Edgar RC. 2013. UPARSE: highly accurate OTU sequences from microbial amplicon reads. Nat Methods. 10:996–998.
- Fallah M, Hadi H, Amirnia R, Hassanzadeh-Ghorttapeh A, Zuan ATK, Sayyed RZ. 2021. Eco-friendly soil amendments improve growth, antioxidant activities, and root colonization in lingrain (Linum Usitatissimum L.) under drought conditions. PLoS ONE. 16(12):e0261225.
- Han W, Kemmitt SJ, Brookes PC. 2007. Soil microbial biomass and activity in Chinese tea gardens of varying stand age and productivity. Soil Biol Biochem. 39:1468–1478.
- Hao J, Peng AQ, Li YY, Zuo H, Li P, Wang JS, Yu KK, Liu C, Zhao SC, Wan XC, et al. 2022. Tea plant roots respond to aluminum-induced mineral nutrient imbalances by transcriptional regulation of multiple cation and anion transporters. BMC Plant Biol. 22:203.
- Jabborova D, Kannepalli A, Davranov K, Narimanov A, Enakiev Y, Syed A, Elgorban AM, Bahkali AH, Wirth S, Sayyed RZ, Gafur A. 2021. Co-inoculation of rhizobacteria promotes growth, yield, and nutrient contents in soybean and improves soil enzymes and nutrients under drought conditions. Sci Rep. 11:22081.
- Jabborova D, Sulaymanov K, Sayyed RZ, Alotaibi SH, Enakiev Y, Azimov A, Jabbarov Z, Ansari MJ, Fahad S, Danish S, Datta R. 2021. Mineral fertilizers improves the quality of turmeric and soil. Sustainability. 13:9437.
- Jiang XL, Li WW, Han ML, Chen G, Wu J, Lai SY, Fu ZP, Zhang SX, Deng WW, Gao LP, Tao X. 2021. Aluminum-tolerant, growth-promoting endophytic bacteria as contributors in promoting tea plant growth and alleviating aluminum stress. Tree Physiol. 42:1043–1058.
- Kalam S, Basu A, Ahmad I, Sayyed RZ, El-Enshasy HA, Dailin DJ, Suriani NL. 2020. Recent understanding of soil Acidobacteria and their ecological significance: a critical review. Front Microbiol. 11:580024.
- Khan N, Ali S, Shahid MA, Mustafa A, Sayyed RZ, Cura JA. 2021. Insights into the interactions among roots, rhizosphere, and rhizobacteria for improving plant growth and tolerance to abiotic stresses: a review. Cells. 10:1551.
- Khati P, Chaudhary P, Gangola S, Sharma P. 2019. Influence of nanozeolite on plant growth promotory bacterial isolates recovered from nanocompound infested agriculture field. Envi Ecol. 37(2):521–527.
- Koblížek M, Dachev M, Bína D, Nupur L, Piwosz K, Kaftan D. 2020. Utilization of light energy in phototrophic Gemmatimonadetes. J Photochem Photobiol B-Biol. 213:112085.
- Kumari S, Sharma A, Chaudhary P, Khati P. 2020. Management of plant vigor and soil health using two agriusable nanocompounds and plant growth promotory rhizobacteria in Fenugreek. 3 Biotech. 10(11):461.
- Kuypers MMM, Marchant HK, Kartal B. 2018. The microbial nitrogen-cycling network. Nat Rev Microbiol. 16:263–276.
- Li SX, Wang ZH, Stewart BA. 2011. Differences of some leguminous and nonleguminous crops in utilization of soil phosphorus and responses to phosphate fertilizers. Adv Agron. 110:125–249.
- Li YC, Li Z, Li ZW, Jiang YH, Weng BQ, Lin WX. 2016. Variations of rhizosphere bacterial communities in tea (Camellia sinensis L.) continuous cropping soil by high-throughput pyrosequencing approach. J Appl Microbiol. 121:787–799.
- Lian TX, Ma QB, Shi QH, Cai ZD, Zhang YF, Cheng YB, Nian H. 2019. High aluminum stress drives different rhizosphere soil enzyme activities and bacterial community structure between aluminum-tolerant and aluminum-sensitive soybean genotypes. Plant Soil. 440:409–425.
- Liu JH, Li WH, Walayat N, Liu SL, Ding YT, Song S. 2022. A calcined shell powder used for minimizing aluminum concentration in the soil of tea plantations. Environ Pollut Bioavail. 34(1):190–201.
- Liu SR, Mi XZ, Ran Z, An YL, Zhou QY, Yang TY, Xia XB, Guo R, Wang XW, Wei CL. 2019. Integrated analysis of miRNAs and their targets reveals that miR319c/TCP2 regulates apical bud burst in tea plant (Camellia sinensis). Planta. 250(4):1111–1129.
- Liu Y, Hu XF, Chen FS, Yuan PC. 2013. Temperature sensitivity of CO2 fluxes from rhizosphere soil mineralization and root decomposition in Pinus massoniana and Castanopsis sclerophylla forests. Chi J Appl Ecol. 24:1501–1508.
- Magoc T, Salzberg SL. 2011. FLASH: fast length adjustment of short reads to improve genome assemblies. Bioinformatics. 27:2957–2963.
- Martins NP, Fuchslueger L, Fleischer K, Andersen KM, Assis RL, Baccaro FB, Camargo PB, Cordeiro AL, Adriana G, Hartley IP, et al. 2021. Fine roots stimulate nutrient release during early stages of leaf litter decomposition in a Central Amazon rainforest. Plant Soil. 469:287–303.
- Mawang CI, Azman AS, Fuad ASM, Ahamad M. 2021. Actinobacteria: an eco-friendly and promising technology for the bioaugmentation of contaminants. Biotechnol Rep. 32:e00679.
- Mora MDLL, Demanet R, Acuña JJ, Viscardi S, Jorquera M, Rengel Z, Durán P. 2017. Aluminum-tolerant bacteria improve the plant growth and phosphorus content in ryegrass grown in a volcanic soil amended with cattle dung manure. Appl Soil Ecol. 115:19–26.
- Murni SD. 2020. Characteristic soil chemical properties and water on tea garden Alahan Panjang region. Gontor Agrotech Sci J. 6(3):517.
- Oshiki M, Toyama Y, Suenaga T, Terada A, Yasuhiro K, Yamaguchi T, Nobuo A. 2022. N2o reduction by Gemmatimonas aurantiaca and potential involvement of Gemmatimonadetes bacteria in N2o reduction in agricultural soils. Microb Environ. 37(2):ME21090.
- Pan JT, Li DQ, Zhu JJ, Shu ZF, Ye XL, Xing AQ, Wen B, Ma YC, Zhu XJ, Fang WP, Wang YH. 2020. Aluminum relieves fluoride stress through stimulation of organic acid production in Camellia sinensis. Physiol Mol Biol Plants. 26:1127–1137.
- Peng CY, Zhu XH, Hou RY, Ge GF, Hua RM, Wan XH, Cai HM. 2018. Aluminum and heavy metal accumulation in tea leaves: an interplay of environmental and plant factors and an assessment of exposure risks to consumers. J Food Sci. 83(4):1165–1172.
- Rao MPN, Luo ZH, Dong ZY, Li Q, Liu BB, Guo SX, Nie GX, Li WJ. 2022. Metagenomic analysis further extends the role of Chloroflexi in fundamental biogeochemical cycles. Environ Res. 209:112888.
- Reji L, Zhang XN. 2022. Genome-resolved metagenomics informs the functional ecology of uncultured Acidobacteria in redox oscillated sphagnum peat. Msystems. 7(5). doi:10.1128/msystems.00055-22.
- Rothwell MAR, Zhai YW, Pagan-Medina CG, Perez-Diaz IM. 2022. Growth of γ-Proteobacteria in low salt cucumber fermentation is prevented by lactobacilli and the cover brine ingredients. Microbiol Spectr. 10(3):e01031–21.
- Ruan JY, Ma LF, Shi YZ, Zhang FS. 2004. Effects of incorporation and nitrogen fertilization on the contents of extractable aluminium in the rhizosphere soil of tea plant (Camallia sinensis (L.) O. Kuntze). Plant Soil. 263:283–296.
- Santhanam R, Luu VT, Weinhold A, Goldberg J, Oh Y, Baldwin IT. 2015. Native root-associated bacteria rescue a plant from a sudden-wilt disease that emerged during continuous cropping. Proc Natl Acad Sci U S A. 112(36):5013–5020.
- Savin MC, Daigh ALM, Brye KR, Norman R, Miller D. 2021. Vertical distribution of fertilizer nitrogen from surface water flooding of a silt loam and clay soil used for rice production. Soil Use Manage. 37:406–417.
- Segata N, Izard J, Waldron L, Gevers D, Miropolsky L, Garrett WS, Huttenhower C. 2011. Metagenomic biomarker discovery and explanation. Genome Biol. 12(6):R60.
- Silva MS, Sales AN, Magalhaes-Guedes KT, Dias DR, Schwan RF. 2013. Brazilian cerrado soil Actinobacteria ecology. BioMed Res Int. 2013:503805.
- Speirs LBM, Rice DTF, Petrovski S, Seviour RJ. 2019. The phylogeny, biodiversity, and ecology of the Chloroflexi in activated sludge. Front Microbiol. 10:2015.
- Sun J, Zhang Q, Zhou J, Wei QP. 2014. Pyrosequencing technology reveals the Impact of different manure doses on the bacterial community in apple rhizosphere soil. Applied Soil Ecology. 78:28–36.
- Sun LL, Zhang MS, Liu XM, Mao QZ, Chen S, Kochian LV, Hong L. 2020. Aluminium is essential for root growth and development of tea plants (Camellia sinensis). J Integr Plant Biol. 62(7):984–997.
- Szurman-Zubrzycka M, Chwiałkowska K, Niemira M, Kwaśniewski M, Nawrot M, Gajecka M, Larsen PB, Iwona S. 2021. Aluminum or low pH-which is the bigger enemy of barley? Transcriptome analysis of barley root meristem under al and low pH stress. Front Genet. 12:675260.
- Timmermans ML, Paudel YP, Ross AC. 2017. Investigating the biosynthesis of natural products from marine proteobacteria: a survey of molecules and strategies. Mrine Drugs. 15:235.
- Wan Q, Mi YH, Yang YY, Xu RK, Li XH. 2018. Aluminum-enhanced proton release associated with plasma membrane H+-adenosine triphosphatase activity and excess cation uptake in tea (Camellia sinensis) plant roots. Pedosphere. 28(5):804–813.
- Wan Q, Xu RK, Li XH. 2012. Proton release from tea plant (Camellia sinensis L.) roots induced by Al (III) under hydroponic conditions. Soil Res. 50:482–488.
- Wang B, Liu GB, Xue S, Zhu B. 2011. Changes in soil physico-chemical and microbiological properties during natural succession on abandoned farmland in the Loess Plateau. Environ Earth Sci. 62:915–925.
- Wang H, Chen X, Ding L, Qiu F, Ye J, Jia X, Kong X, He H. 2018. Effect of soil acidity on microbial diversity in rhizospheric soils of tea plants. Chinese J Trop Crop. 39(3):448–454.
- Wang H, Xu RK, Wang N, Li XH. 2010. Soil acidification of Alfisols as influenced by tea plantation in eastern China. Pedosphere. 20:799–806.
- Yan P, Wu LQ, Wang DH, Fu JY, Shen C, Li X, Zhang LP, Zhang L, Fan LC, Han WY. 2020. Soil acidification in Chinese tea plantations. Sci Total Environ. 715:136963.
- Yang T, Liu G, Li Y, Zhu S, Zou A, Qi J. 2012. Rhizosphere microbial communities and organic acids secreted by aluminum-tolerant and aluminum-sensitive soybean in acid soil. Biol Fertil Soils. 48:97–108.
- Yang XD, Ni K, Shi YZ, Yi XY, Zhang QF, Fang L, Ma LF, Ruan JY. 2018. Effects of long-term nitrogen application on soil acidification and solution chemistry of a tea plantation in China. Agric Ecosyst Environ. 252:74–82.
- Yu JL, Lin S, Shaaban M, Ju WL, Fang LC. 2020. Nitrous oxide emissions from tea garden soil following the addition of urea and rapeseed cake. J Soils Sediments. 20(9):3330–3339.
- Zeng YHN, Wu NC, Madsen AM, Chen XH, Gardiner AT, Koblížek M. 2021. Gemmatimonas groenlandica sp. nov. is an aerobic anoxygenic phototroph in the phylum Gemmatimonadetes. Front Microbiol. 11:606612.
- Zhang ZF, Qian F, Xiao CYX, Ding MY, Liang D, Li HT, Liu RM. 2022. Impact of Paenarthrobacter ureafaciens ZF1 on the soil enzyme activity and microbial community during the bioremediation of atrazine-contaminated soils. BMC Microbiol. 22:146.
- Zheng RK, Cai RN, Wang C, Liu R, Sun CM. 2022. Characterization of the first cultured representative of “Candidatus Thermofonsia” clade 2 within Chloroflexi reveals its phototrophic lifestyle. MBIO. 13(2):e00287–22.
- Zhou B, Chen YY, Zeng LT, Cui YY, Li JL, Hao T, Liu JY, Tang JC. 2022. Soil nutrient deficiency decreases the postharvest quality-related metabolite contents of tea (Camellia sinensis (L.) Kuntze) leaves. Food Chem. 377:132003.
- Zhou L, Xu ST, Monreal CM, Mclaughlin NB, Zhao BP, Liu JH, Hao GC. 2022. Bentonite-humic acid improves soil organic carbon, microbial biomass, enzyme activities and grain quality in a sandy soil cropped to maize (Zea mays L.) in a semi-arid region. J Integrat Agri. 21(1):208–221.