Abstract
Enterohemorrhagic Escherichia coli (EHEC) are important pathogenic E. coli characterized by the production of Shiga toxin, intimin, and enterohemolysin, which are important virulence factors for pathogenesis. These virulence factors are the cause of enterocyte extinction and affect both intestinal cells and various extraintestinal cells such as lymphocytes, granulocytes, erythrocytes, and renal cells. The resultant severe bloody diarrhea and colonic infections are associated with life-threatening extraintestinal complications, leading to high morbidity and mortality worldwide. Although EHEC has been responsible for large outbreaks around the world, research into effective therapies to prevent and treat the infection in a timely manner and affordable diagnostic tests remains a challenge due to the complicated nature of the bacteria. This review addresses previous studies, the current status, and prospects regarding the severity, treatment, and diagnosis of EHEC with a focus on a new finding on the use of simple point of care diagnostic procedures.
Introduction
Several pathogenic Escherichia coli strains can cause infections in the intestine and a variety of extraintestinal sites such as the urinary tract, meninges, and bloodstream in animals and humans (Ruciaka Citation2017; Sarowska et al. Citation2019; Govindarajan and Kandaswamy Citation2022). These strains are evolved by obtaining virulence genes from plasmids, transposons, bacteriophages or pathogenicity islands (Javadi et al. Citation2017). According to their serogroups, pathogenicity mechanisms, clinical symptoms, and virulence characteristics, pathogenic E. coli strains are divided into numerous groups (Ori et al. Citation2019; Govindarajan et al. Citation2020). Among the pathogenic E. coli bacteria, enterohemorrhagic E. coli (EHEC) are important bacteria that cause life-threatening hemolytic uremic syndrome (HUS) and hemorrhagic colitis (HC) (Mühlen et al. Citation2020). These bacteria are emerging zoonotic pathogens and are transmitted through contaminated food and water (Igwaran et al. Citation2018). They primarily colonize the human large and small intestine, causing illness through attaching and effacing (A/E) lesions in the intestinal epithelium (Slater and Frankel Citation2020) and producing bloody diarrhea (Cabrera-Sosa and Ochoa Citation2020). EHEC strains release a very potent toxin called Shiga toxin (Stx), with the O157 (O157:H7) antigen-producing E. coli bacteria being the most common. In particular, O157:H7 serotype has been associated with global outbreaks of food and waterborne illness (Katani Citation2016; Ubeda et al. Citation2017; Hwang et al. Citation2021; Shumi et al. Citation2021), both in developed and developing countries including: Germany and France in 2011 (Köckerling et al. Citation2017); EU/EEA from 2012 to 2017 (Biohaz Panel Citation2020); Sweden in 2005 (Söderström et al. Citation2005); Canada in 1995 (Welinder-Olsson et al. Citation2004); Japan from 2006 to 2010 (Watahiki et al. Citation2014); Korea in 2004 (Kwun and Lee Citation2007); and South Africa in 2003 (Hunter Citation2003).
The incidence and type of toxins in pathogenic strains acquired from a bacteriophage are determined by the Stx1 and Stx2 genes (Onuoha Citation2017; Daryaei et al. Citation2018; Karmali Citation2018; Akindolire Citation2019; Fitzgerald et al. Citation2019; Maniha and Noor Citation2020). The severity of EHEC is caused by the production of Stx which induces an inflammatory response in the gut and translocates to the epithelial barrier of the gut. The bacterial virulence factors intimin and Stx, as well as a type III secretion system (T3SS), are associated with symptoms and intestinal damage leading to HUS, thrombocytopenia, microangiopathic hemolytic anemia and renal damage (Delahoy et al. Citation2018; D’Accolti et al. Citation2021).
To date, several studies have identified different interventional mechanisms, including dialysis (hemodialysis or peritoneal dialysis), or a comparison of two or more interventions were examined. Interventions examined have included heparin, aspirin/dipyridamole, prostanoids, ticlopidine, vincristine, fresh frozen plasma (FFP) infusion, plasmapheresis with FFP or cryosupernatant plasma, systemic corticosteroids, Stx-binding agents, or immunosuppressive agents of HUS in humans, but specific treatment options are not yet available (Michael et al. Citation2009). Intravenous fluids and volume expansion are non-specific interventions used to some extent to treat this disease. Antibiotics are generally used as therapeutics for bacterial infections. However, antibiotic therapy is not recommended for patients with EHEC due to the lack of benefit, and even harm that may result. When O157:H7 infection is suspected or confirmed, the use of antibiotics is contraindicated because of the possibility of increased risk of HUS due to induction of Stx-encoding bacteriophages (Garzón et al. Citation2020).
Various approaches to pathogen detection, such as selective culture media, enzyme immunoassays, microfluidic systems and PCR assays, have been developed (Rajapaksha et al. Citation2019; Sivaramakrishnan et al. Citation2020). To promptly report outbreaks and prevent further cases, design of rapid EHEC detection methods with high sensitivity and specificity for pathogen identification is of utmost importance and benefit. The current recommendation for EHEC diagnosis in humans by clinical laboratories is culture isolation, which remains the gold standard for identification. Nevertheless, to reduce the analysis time, various cost effective and rapid immunological and molecular detection systems have been developed and used. Effective detection of Stx has been performed using PCR-ELISA, loop-mediated isothermal amplification (LAMP), monoclonal and polyclonal immunoassays, qPCR, multiplex-PCR, PCR-less DNA co-polymerization and with DNA linked gold nanoparticles (AuNPs) and magnetic microparticles (Saeedi et al. Citation2017). Moreover, this review discusses in detail various advanced and novel EHEC intervention and diagnostic methods, their potential benefits and challenges for application.
Mechanisms of pathogenesis
According to Jubelin et al. (Citation2018), the pathogenesis of EHEC is a multistep process that involves interactions between a number of different bacterial and host components. In humans, it encodes many virulence genes that cause disease (Baranzoni et al. Citation2016). EHEC are bacteria that cause bloody and watery diarrhea, as well as HC, HUS, and thrombotic thrombocytopenic purpura (TTP) in humans (Shahreza et al. Citation2017). EHEC O157 has been identified as one of the major causative agents of these diseases in humans. In the past two decades, E. coli O157:H7 has been one of the most prominent foodborne pathogens, causing enormous losses in the human population (Yang et al. Citation2017). The production of Stx causing HUS, and colonization of the intestinal epithelium through the formation of A/E lesions are the main features of EHEC pathogenesis (Shanmugasundarasamy et al. Citation2022).
Attaching and effacing adherence
The significant attaching and effacing that causes the formation of a histopathological lesion occurs at the time of colonization of the large bowel and intestinal epithelial cells by EHEC (Estrada-Garcia et al. Citation2013; Xue-Han et al. Citation2013). EHEC exploits a pedestal-like structure or ‘actin pedestal’ on the apical surface of the intestinal epithelium for adhesion and colonization, and then remains there to cause infection, microvillus disruption at the brush border, and polymerized actin buildup. For virulence and the emergence of A/E lesions, the locus of enterocyte effacement (LEE), a chromosomal pathogenicity island present in EHEC, is required (Desvaux et al. Citation2020; Hentschel et al. Citation2021).
An important bacterial structure that promotes adherence is the fimbriae, thread-like structures that extend from the surface of bacteria. There are three types of fimbriae responsible for adherence (Lukaszczyk et al. Citation2019). This includes type I fimbriae that cause intimate adherence and A/E lesion formation; P fimbriae that recognize a disaccharide from uroepithelial cells and human erythrocytes; and S fimbriae that mediate adherence to endothelial cells, urinary tract epithelial cells, and brain endothelial cells (Pal and Ayele Citation2017). The virulence factor produced by this bacterium gets transmitted to the host through a T3SS.
Virulence of Shiga toxin
The ability to produce Stx is the most important pathogenic feature of EHEC. Stx (Stx1 and Stx2) is the prototype toxin of Shigella dysenteriae serotype 1 (Paletta et al. Citation2020), and consists of two major subunits, the A subunit and the B subunit. The A subunit suppresses cell relevant protein synthesis in the gene expression process and promotes apoptosis by acting as an RNA N-glycosidase against the 28S rRNA, resulting in removal of a single adenine base from this rRNA (Menge Citation2020). The B subunit of Stx causes disease by creating a pentamer that binds to the globotriaosylceramide-3 molecule (Gb3), located and expressed in the outer layer of the cell membrane. After EHEC colonizes the human large intestine and establishes pedestal-like structures on intestine epithelial cells (Nkiiri Citation2017), Stx is then released as it binds to endothelial cells that express Gb3, allowing it to enter the bloodstream and spread to other organs (Lee and Tesh Citation2019), while cleaving a single adenine residue from the eukaryotic ribosomal 60S subunit's 28S rRNA component (Hall et al. Citation2017). Moreover, according to Bowen (Citation2020), the toxin causes disease by: (1) an intestine epithelial barrier-crossing mechanism; (2) causing a gut inflammatory infiltration that is neutrophil-rich; and (3) being transported by neutrophils through the circulation, which mediates the transport to tissues with high-prevalence of Stx (Khadem Citation2019; Pienaar Citation2019; Jia and Zhang Citation2020; Panwar et al. Citation2020) (Figure ).
Figure 1. Sequences of events leading to diseases of EHEC (Source: Gerba Citation2009).
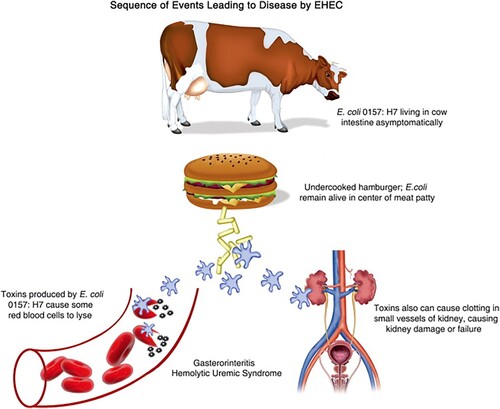
Clinical severity
EHEC outbreaks have been recorded worldwide in both developing and developed countries (Pal et al. Citation2019). Some people recover without treatment, but others develop HC in a few days (Grantina-Ievina and Rodze Citation2020). HC is characterized by profuse bloody diarrhea, abdominal soreness, and, in most cases, severe stomach cramps, as well as nausea, vomiting, and dehydration to some extent. Sometimes, individuals may experience a low-grade fever (Kumar et al. Citation2017; Travert et al. Citation2021). Many occurrences of HC are self-limiting, with patients healing in a week or less, but severe cases might cause intestinal necrosis, perforation, or the formation of colonic strictures (Lianou et al. Citation2017; Surendran Nair Citation2017; Bhunia Citation2018; Akindolire Citation2019; Dias et al. Citation2019).
HUS causes significant kidney damage, anemia, and thrombocytopenia in immunocompromised individuals. It develops within two weeks of the onset of diarrhea, but occasionally cases without prodromal diarrhea do occur (Swelam et al. Citation2017). While some HUS patients have significant renal disease but no thrombocytopenia or little hemolysis, other HUS patients have hemolytic anemia and/or thrombocytopenia with little to no renal disease.
Lethargy, irritability, convulsions, paresis, stroke, cerebral edema, or coma are only a few examples of extrarenal symptoms; others include respiratory syndromes, elevated pancreatic enzymes or pancreatitis, deep abscesses or myocardial involvement (Bateman and Conrad Citation2016). TTP is the most common form of HUS in adults, particularly in the elderly, and kidney damage is typically less common in TTP than in children, but neurologic symptoms are more common (Kappler et al. Citation2017). Although most children recover from HUS without any lasting damage, some patients may experience long-term renal complications of varying severity (Khalid and Andreoli Citation2019). Extrarenal issues can also include pancreatic insufficiency, temporary or persistent insulin-dependent diabetic mellitus, and neurologic deficits such poor fine-motor coordination (Khalid and Andreoli Citation2019).
Clinical cases of EHEC can occur at any time. In various countries, the estimated annual incidence of EHEC O157:H7 ranges from 0.5 to 50 cases per 100,000 people. EHEC O157:H7 infections arise in temperate climates during summer months due to animal shading (Smith and Fratamico Citation2018). However, not all EHEC infections follow the same seasonal trend and may peak at different times. Mortality rates for clinical cases vary by disease. HC is self-limiting in most cases but can be fatal. It is estimated that EHEC-associated HUS/TTP kills 1–10% of adolescents and up to 50% of the elders (Nkiiri Citation2017).
Antibiotic treatment and potential preventive agents
HUS includes acute renal failure causing fluid and electrolyte imbalance, hemolysis, and stroke risk, as well as the formation of toxins and complement complexes. To counteract this, a multidrug approach is used. Antiplatelet and thrombolytic medicines, as well as thrombin inhibitors, antimicrobials, probiotics, toxin neutralizers, and antibodies against critical elements of the pathogenetic pathway, are used to halt pathological processes necessary for disease elimination and treatment (Charnaya and Moudgil Citation2017; Song et al. Citation2019).
Antibiotic therapy is not usually used in EHEC to avoid release of toxic substances by dead pathogenic bacterial cells. Monitoring hemoglobin, hematocrit, and platelet count is also important to control hematologic problems (Garzón et al. Citation2020). Fluid and electrolyte imbalances are corrected with intravenous fluid administration. Renal replacement therapy, apheresis, and antihypertensives can be used to treat acute renal failure. A new EHEC treatment method involves binding Stx in the gastrointestinal system using ligands such as Synsorb Pk, a synthetic analog of the Stx receptor (Gb3), to bind to Stx in the gastrointestinal tract and prevent the spread of the toxin to extraintestinal sites (Detzner et al. Citation2022). Antibodies are used for therapeutic purposes by neutralizing the toxin. Stx1 monoclonal antibodies targeting the epitopes of the A subunit of Stx1 have been shown to neutralize the toxin (Mühlen et al. Citation2020; Mühlen and Dersch Citation2020). Orally administered immunoglobulin, such as for rotavirus, have been used for gastrointestinal infections (Jasion and Burnett Citation2015).
On the other hand, the use of recombinant secretory immunoglobulin A (sIgA) in passive mucosal immunotherapy is common for pre-harvest intervention of EHEC. Customized intervention strategies will be required to prevent pre-harvest E. coli O157 colonization, which should lower carcass contamination and improve public health. Specific direct-fed microbial strains, vaccine technology, sodium chlorate, and neomycin sulfate are a few pre-harvest interventions that have significant promise, while brown seaweed and water chlorination have little to no discernible benefit (Loneragan and Brashears Citation2005). Thus, a sIgA directed against intimin would serve as a drug to prevent the interaction of luminal EHEC cells with the host epithelium and eliminate them by entrapment in the mucosal layer and subsequent fecal shedding. The mechanism in the gastrointestinal tract is therefore that sIgA functions primarily to eliminate pathogens by immune exclusion (Saberianfar et al. Citation2019). Saberianfar et al. (Citation2019) produced a chimeric antibody (VHH10-sIgA) with sIgA functionality against three EHEC strains: O26:H11, O111: Hnm, and O157:H7. Because antibiotic treatment of EHEC is controversial or has adverse effects that may aggravate the course of the disease, particularly by triggering Stx production, currently available antibiotics cannot be used as therapy. New antibiotic drugs such as sIgA and lactoferrin (LF) are needed. Studies have shown that LF has potential as a therapeutic agent in EHEC infections that can be used for treatment (Flores-Villaseñor et al. Citation2012). This iron binding protein has shown broad spectrum of antimicrobial activity both in vivo and in vitro (Farnaud and Evans Citation2003; Orsi Citation2004; Zimecki et al. Citation2004). LF bactericidal activity sets in after the host cell membrane has been penetrated and damaged (Haney et al. Citation2007; Flores-Villaseñor et al. Citation2012). Short cationic peptides with amphipathic structures, known as antimicrobial peptides (AMPs), which are mainly found in animals and plants, play an important role in the defense against pathogens. For example, cecropin A and LL37 can trigger anti-inflammatory effects (Ishida et al. Citation2016); a study using mice as a model of intestinal inflammation showed that a hybrid peptide of cecropin A and LL37 (C-L peptide) can provide effective therapy against intestinal inflammation and the impairment of epithelial barrier function caused by EHEC O157:H7 (Wei et al. Citation2020). Another study using a mouse model of EHEC O157:H7 infection shows that porcine-derived AMP PR39 alleviates inflammation levels, maintains intestinal barrier function and immune regulation, and balances the gut microbiota, making this drug a potential treatment for EHEC O157:H7 (Haiwen et al. Citation2019).
Probiotics for the treatment of EHEC infections
Probiotics that block host cells and the bacterial interaction are another promising option for treating EHEC. Probiotics can balance the composition of the intestinal population and control the intestinal microbiota (Wang et al. Citation2020). They are harmless engineered bacteria that express a surface molecule resembling the host cell receptor, enticing the pathogen to attach to the probiotic cell rather than the host cell receptor. For instance, supernatant from cultures of Bifidobacterium longum HY8001 inhibits the action of Stx by interfering with the B subunit of Stx in binding to Gb3 (Goldwater and Bettelheim Citation2012). One study showed that the probiotic Clostridium butyricum MIYAIRI strain 588 inhibited the growth and toxin production of EHEC O157:H7 and had prophylactic and therapeutic effects on EHEC O157:H7 infections in gnotobiotic mice (Takahashi et al. Citation2004). Dini et al. (Citation2016) isolated the podophage CA933P and the probiotic Lactobacillus plantarum strain CIDCA 83114, which successfully inhibited EHEC pathogenesis in vitro, and they are promising candidates for the biocontrol of EHEC (Dini et al. Citation2016). By reducing intestinal inflammation, enhancing intestinal barrier function, and managing the intestinal microbiota in infected female mice, Pediococcus pentosaceus IM96 protects against EHEC O157:H7 and may be an alternative therapy to antibiotics for treating EHEC O157:H7 (Wang et al. Citation2020). Taken together, probiotics and their combination with prebiotics, which are non-digestible nutrients that are degraded by intestinal microbiota, beneficially influencing their growth and activity, may be a promising alternative treatment approach to improve and maintain host health (Tabashsum et al. Citation2019).
EHEC vaccines
Novel alternatives for treatment include the use of attenuated live bacteria, such as Salmonella spp., as carriers for vaccine proteins against mucosal diseases such as EHEC, as well as protein-based and DNA vaccines (Nimmanapalli and Gupta Citation2020). Several vaccine strategies have been demonstrated in animal models. The production of attenuated and recombinant vaccine against EHEC is another promising novel approach to eradicate this disease (Goumari et al. Citation2020). Vaccines can be produced by using recombinant virulence proteins containing Stx, intimin, and E. coli secreted protein A (EspA), an important part of the T3SS that is needed for EHEC pathogenesis, peptides such as Stx2 subunit A-Stx1 subunit B, and EHEC O157:H7 pathogenic host cells (Nesta and Pizza Citation2018). Cattle vaccines against EHEC O157:H7 can reduce shedding and have received full or conditional approval in some countries, including the United States and Canada, but are not widely used (Mesele and Abunna Citation2019). A recent study shows that vaccine formulated with the EHEC antigens LomW, EscC, LpfA1, or LpfA2 and administered using AuNPs can elicit robust antigen-specific cellular and humoral responses associated with reduced EHEC colonization in a mouse model (Sanchez-Villamil et al. Citation2022).
Detection of EHEC
The identification of biomarkers or unique genes and antigens as biomarkers for Stx-producing strains, including EHEC, is beneficial for diagnosis, epidemiological surveillance, and phylogenetic investigation, especially during outbreaks. To this end, several studies have been conducted to develop a new marker and methods to distinguish EHEC from other pathogenic and non-pathogenic E.coli (McMahon et al. Citation2017). The current evolution of EHEC epidemiology requires the development of genetic markers to increase the efficiency of diagnostic tests. For the detection of E.coli from environmental samples the most common genetic markers such as 23S rRNA and lacZ are used with the established PCR method. Most PCR assays amplify the virulence genes of EHEC, such as eaeA, Stx1, and Stx2, or phenotypic genes, such as rfbE (O antigen), fliC (H antigen), uidA and lacZ which are commonly shared (Fagan et al. Citation1999; Sharma Citation2006; Madic et al. Citation2010; Deshmukh and Roy Citation2021). Additionally, the orphan gene yaiO, which encodes an outer membrane protein, and the xanQ gene can be used to detect EHEC (Srivastava et al. Citation2018; Rajapaksha et al. Citation2019; Ricke et al. Citation2019; Deshmukh and Roy Citation2021). However, the above genetic markers do not play a significant role in distinguishing EHEC pathogenic E. coli from Shigella spp. Recently, z3276 and Stx genes were identified to specifically detect E. coli O157:H7 and screen for non-O157 Stx-producing E. coli (STEC) (Stx1 and Stx2) (Deshmukh and Roy Citation2021).
In addition to the unique gene markers and antigen markers of EHEC, cytokines and chemokines could play an important role as biomarkers in diagnosing EHEC pathogenesis (Alakrea et al. Citation2022). Studies have shown that 174 serum cytokines have been detected, and five serum biomarkers have been identified to predict the severity of EHEC-induced HUS (Shimizu et al. Citation2012). Among them are, angiopoietin (Ang-1 and -2), which competitively bind to the endothelial Tie-2 receptor and play a crucial role in regulating endothelial cell function (Eklund and Saharinen Citation2013). Another study shows that sensory protein indicators can also be used as biomarkers for the diagnosis of a disease or the outcome of a therapeutic intervention (Bhar et al. Citation2020). Taken all together, distinguishing the different EHEC isotypes is not an easy task, and various molecular methods have been developed to distinguish Shigella spp. from EHEC. Nowadays, clinical laboratories use matrix-assisted laser-desorption ionization time-of-flight mass spectrometry (MALDI-TOF MS) in routine diagnostics to identify bacteria (Van den Beld et al. Citation2022). The other commercially available databases like VITEK® MS, MALDI biotyper®, MALDI Security-Relevant (SR) library can distinguish Shigella spp. and E. coli from other Enterobacteriaceae, but they cannot differentiate among Shigella spp. and E. coli, including the EHEC pathotype (Van den Beld et al. Citation2022).
Culture-dependent detection methods
Over a long period of time, culture methods were referred to as a gold standard for the detection of EHEC in clinical and environmental samples. These techniques often used vancomycin, cefsulodin, and cefixime to limit the growth of Aeromonas spp. and Proteus spp., whereas selective novobiocin or acriflavin were employed to inhibit the growth of Gram-positive organisms. However, the most common biochemical detection method is sorbitol MacConkey agar (SMAC). Because EHEC strains have lost their sorbitol fermenting gene, they are unable or slow to ferment SMAC (Blackburn and McCarthy Citation2000; Perry Citation2017). Similarly, because of lack of β-glucuronidase or 4-methylumbelliferyl-b-D-glucuronidase activity of EHEC strains, selective culture media called chromogenic media (CHROMagar O157 or Colorex™ O157 Agar) exploit a substrate for β-glucuronidase to detect E.coli as common urinary pathogens (Ebrahimi et al. Citation2016; Perry Citation2017). The advent of numerous chromogenic agars and antibody-bead retrieval systems has improved the diagnosis of EHEC, and they are still the method of choice for many diagnostic laboratories (Jia et al. Citation2021). Even though many modified EHEC methods have been investigated, the culture method has disadvantages, such as the inability to detect nonviable organisms, the possibility that normal flora may mask STEC growth, the relatively low detection limit, the length of time (24–48 h) for a definitive diagnosis, and the additional procedures required to obtain serotype, virulotyping or antimicrobial resistance (AMR) information (Naylor et al. Citation2007; Newell and La Ragione Citation2018; Bizot et al. Citation2021). Moreover, because the culture method is labor intensive, expensive and time-consuming, there is an urgent need for rapid, economical testing for EHEC.
Immunology based detection methods
Immunology based detection methods have advantages for clinical laboratories because they have high efficiency (specificity and sensitivity), straightforward execution, and can be performed quickly (Moges et al. Citation2022). There are several commercial immunoassays, such as ELISA immunoassay (EIA): ProSpecT™ Shiga Toxin E. coli, Premier® EHEC, Ridascreen® Verotoxin test; Shiga toxin Check™ and Shiga Toxins, EIA with Reflex to E coli O157; lateral flow assay (LFA): Duopath®Verotoxins, ImmunoCard STAT!®EHEC, Ridascreen® Quick Verotoxin/O157 and Shiga toxin Quick Check™; immunomagnetic separation, such as the Rapid Check®Confirm™STEC; and an optical immunoassay, such as the Biostar OIA Shigatoxin (Silva et al. Citation2019). However, the aforementioned commercially available assays are not used as point of care tests (POCT), i.e. they are neither laboratory diagnostics performed close to the patient, nor affordable to implement in routine clinical laboratories in low and middle-income countries. Therefore, several studies have been conducted to find a new immunological target for the development of a low cost EHEC diagnostic test. Shiga et al. (Citation2020) developed a latex agglutination test (LAT), lateral flow assay (LFA), and capture ELISA (cEIA) using Stx1 and Stx2 monoclonal antibodies with high efficiency (96%), which could robustly diagnose EHEC. Latex agglutination test using recombinant single-chain antibody fragments (scFvStx1 and scFvStx2) coupled to nanoparticles like latex particles can be used as a routine laboratory or POCT, with low cost and short execution time (Shiga et al. Citation2020). The rabbit antiserum specific for STEC strain VT3 (Stx1, Stx2 and eae) prepared by Hajra et al. (Citation2007) and used for the latex agglutination test, was approximately 98% efficient. Therefore, efforts should be directed toward the development of affordable POCTs to provide rapid and accurate pathogen detection tests with maximum sensitivity.
DNA amplification detection methods
Various methods have been developed worldwide for the detection O157:H7 (e.g. PCR, selective culture, immunological techniques, LAMP, and molecular approaches) (Zhao et al. Citation2019). The methods for detecting EHEC O157:H7 do not distinguish between EHEC O157:H7 and non-EHEC O157, which are biochemically like other E. coli and ferment sorbitol (Mercanoglu Taban and Aytac Citation2019). Stx production is frequently used to identify non-O157 EHEC, which is then sent to a reference lab for additional characterization. Additionally, traditional biochemically based selective culture techniques are time-consuming and typically take up to 72 h. Therefore, the creation of quick detection methods based on genetic and immunological targets has drawn attention (Baker Citation2015). Rapid immunological tests are exploited to detect O and H antigens or various genes associated with EHEC. These tests are utilized with human clinical or food samples, but some validated kits are not sensitive enough to test fecal samples from animals (Newell and La Ragione Citation2018). Because of their lack of specificity, approaches based on the detection of somatic O157 and flagellar H7 antigens are also inadequate (Jyoti and Tomar Citation2018; Umesha and Manukumar Citation2018).
Nowadays DNA-based techniques are used to identify whether EHEC is present in a fecal specimen or food sample (Forsythe Citation2020). Molecular methods such as real-time polymerase chain reaction (PCR) testing have been used to detect foodborne diseases (such as E. coli O157:H7) over the past decade by amplifying many key genes (Liu et al. Citation2017). For instance, multiplex PCR, CRISPR-directed real-time PCR, commercial real-time PCR kits, as well as droplet digital PCR (ddPCR) technology and the geneFields® EHEC/SS kit using marker genes (Stx1,Stx2, eaeA, and EHEC hlyA gene, fliCh7, rfbE) were developed to advance the specificity and sensitivity, rapidity, affordability and reliability of this diagnostic method (Fagan et al. Citation1999; Sharma and Dean-Nystrom Citation2003; Madic et al. Citation2010; Delannoy et al. Citation2012; Sadhu et al. Citation2018; Mercanoglu Taban and Aytac Citation2019; Nihayati et al. Citation2020). Despite the need for trained personnel, operating space, and sophisticated instrumentation, post-amplification procedures such as electrophoresis take longer (3–4 h) to determine the result, require extracted DNA from the sample, and are sensitive to contaminants or PCR inhibitors (World Health Organization Citation2020).
As a result, the demand for simple and cost-effective molecular testing assay is increasing. LAMP is a ground-breaking nucleic acid amplification technique based on auto-cycling strand displacement DNA synthesis using Bst DNA polymerase (Srividya et al. Citation2019; Yinur, Citation2019). Since the beginning of molecular biology, technologies such as multiplex PCR, real-time PCR, reverse transcription PCR, quantitative PCR, immunocapture PCR, PCR-ELISA, and other molecular detection methods have been developed (Dhama et al. Citation2014; Indraswari et al. Citation2021). Each of these methods has its own set of drawbacks. Of the above techniques, LAMP is most likely superior because it is efficient, rapid, cheap and easy for the detection of EHEC (Dhama et al. Citation2014; Ravan et al. Citation2020).
Conclusion
Diarrhea remains a serious public health concern and is now considered one of the leading causes of death. Since no effective therapies for HUS disease is available, ceasing primary infection by Stx-producing bacteria and quick identification are crucial; early separation of infected individuals is needed to abolish secondary infections. In addition to the culture method (SMAC), the use of molecular techniques (PCR and LAMP) to diagnose EHEC is recommended, because they can distinguish EHEC from Shigella spp. and non-EHEC pathogenic E.coli by determining the presence or absence of certain virulence factors. Efforts should be focused towards the development of point of care diagnostic assays because the diagnosis of disease plays a significant role in its treatment and control. Taken all together, to step up the development of new strategies to control, prevent and treat EHEC infection and disease, multidisciplinary research efforts in EHEC pathogenesis, epidemiology and pathophysiology should be applied.
Acknowledgments
The authors would like to thank the University of Gondar for allowing us to work in this area. Both authors have substantially contributed to the work reported. To list individual author contributions: DY was involved in conceptualizing, original draft writing, writing review and editing; YM was involved in writing review and editing, revising critically, data curating, validating. Both authors have commented, read, and approved the final manuscript as well as agreed to be accountable for all aspects of the work.
Disclosure statement
No potential conflict of interest was reported by the author(s).
Data availability statement
Data sharing is not applicable to this article as no new data were created or analyzed in this study.
Additional information
Funding
References
- Akindolire MA. 2019. Molecular characterisation of Escherichia coli O157: H7 specific bacteriophages from cattle faeces. Diss. North-West University (South Africa).
- Alakrea MAA, Hussein HM, Gomaa MA-S, Ibrahem AMA. 2022. Diagnostic value of angiopoietin-1 and-2 as markers for disease severity in hemolytic uremic syndrome in children. Egypt J Hosp Med. 86(1):513–517.
- Baker CA. 2015. Shiga Toxin-Producing Escherichia coli (STEC) Detection Strategies with Formalin-Fixed STEC Cells. Diss. University of Arkansas.
- Baranzoni GM, Fratamico PM, Gangiredla J, Patel I, Bagi LK, Delannoy S, Fach P, Boccia F, Anastasio A, Pepe T. 2016. Characterization of Shiga toxin subtypes and virulence genes in porcine Shiga toxin-producing Escherichia coli. Front Microbiol. 7:574.
- Bateman L, Conrad K. 2016. Inpatient medicine. In: Absolute hospital medicine review. Cham: Springer; p. 1–105.
- Bhar S, Bose T, Mande SS. 2020. Can pathogenic and nonpathogenic bacteria be distinguished by sensory protein abundance? Appl Environ Microbiol. 86(14):e00478-20.
- Bhunia AK. 2018. Foodborne microbial pathogens: mechanisms and pathogenesis. Springer.
- Biohaz Panel. 2020. Pathogenicity assessment of Shiga toxin-producing Escherichia coli (STEC) and the public health risk posed by contamination of food with STEC. EFSA J. 18(1):e05967.
- Bizot E, Cointe A, Smadja N, Sergentet D, Lefèvre S, et al. 2021. Improved molecular diagnosis and culture of the emerging heteropathotype enterohemorrhagic Escherichia coli O80: H2 using Its Non-melibiose-fermenting and antibiotic-resistance properties. J Clin Microbiol. 60(1):e01530-21.
- Blackburn CdW, McCarthy JD. 2000. Modifications to methods for the enumeration and detection of injured Escherichia coli O157:H7 in foods. Int J Food Microbiol. 55(1-3):285–290.
- Bowen EE. 2020. The Role of the Podocyte in Shiga Toxin Associated Haemolytic Uraemic Syndrome. Diss. University of Bristol.
- Cabrera-Sosa L, Ochoa TJ. 2020. Escherichia coli diarrhea. In: Hunter’s tropical medicine and emerging infectious diseases. Elsevier; p. 481–485.
- Charnaya O, Moudgil A. 2017. Hypertension in the pediatric kidney transplant recipient. Front Pediatr. 5:86.
- D’Accolti M, Soffritti I, Mazzacane S, Caselli E. 2021. Bacteriophages as a potential 360-degree pathogen control strategy. Microorganisms. 9(2):261.
- Daryaei H, Peñaloza W, Hildebrandt I, Krishnamurthy K, Thiruvengadam P, et al. 2018. Heat inactivation of Shiga toxin-producing Escherichia coli in a selection of low moisture foods. Food Control. 85:48–56.
- Delahoy MJ, Wodnik B, McAliley L, Penakalapati G, Swarthout J, et al. 2018. Pathogens transmitted in animal feces in low-and middle-income countries. Int J Hyg Environ Health. 221(4):661–676.
- Delannoy S, Beutin L, Fach P. 2012. Use of clustered regularly interspaced short palindromic repeat sequence polymorphisms for specific detection of enterohemorrhagic Escherichia coli strains of serotypes O26:H11, O45:H2, O103:H2, O111:H8, O121:H19, O145:H28, and O157:H7 by real-time PCR. J Clin Microbiol. 50(12):4035–4040.
- Deshmukh R, Roy U. 2021. Molecular Diagnostic Platforms for Specific Detection of Escherichia coli. Escherichia coli - Old and New Insights. IntechOpen.
- Desvaux M, Dalmasso G, Beyrouthy R, Barnich N, Delmas J, et al. 2020. Pathogenicity factors of genomic islands in intestinal and extraintestinal Escherichia coli. Front Microbiol. 11:2065.
- Detzner J, Pohlentz G, Müthing J. 2022. Enterohemorrhagic Escherichia coli and a fresh view on Shiga toxin-binding glycosphingolipids of primary human kidney and colon epithelial cells and their toxin susceptibility. Int J Mol Sci. 23(13):6884.
- Dhama K, Karthik K, Chakraborty S, Tiwari R, Kapoor S, et al. 2014. Loop-mediated isothermal amplification of DNA (LAMP): a new diagnostic tool lights the world of diagnosis of animal and human pathogens: a review. Pak J Biol Sci. 17(2):151–166.
- Dias D, Caetano T, Torres RT, Fonseca C, Mendo S. 2019. Shiga toxin-producing Escherichia coli in wild ungulates. Sci Total Environ. 651:203–209.
- Dini C, Bolla PA, de Urraza PJ. 2016. Treatment of in vitro enterohemorrhagic Escherichia coli infection using phage and probiotics. J Appl Microbiol. 121(1):78–88.
- Ebrahimi M-MS, Dohm N, Müller M, Jansen B, Schönherr H. 2016. Self-reporting hydrogels rapidly differentiate among enterohemorrhagic Escherichia coli (EHEC) and non-virulent Escherichia coli (K12). Eur Polym J. 81:257–265.
- Eklund L, Saharinen P. 2013. Angiopoietin signaling in the vasculature. Exp Cell Res. 319(9):1271–1280.
- Estrada-Garcia T, Hodges K, Hecht GA, Tarr PI. 2013. Chapter 8. Escherichia coli. In: Foodborne infections and intoxications. Elsevier.
- Fagan PK, Hornitzky MA, Bettelheim KA, Djordjevic SP. 1999. Detection of Shiga-like toxin (stx1 and stx2), intimin (eaeA), and enterohemorrhagic Escherichia coli (EHEC) hemolysin (EHEC hlyA) genes in animal feces by multiplex PCR. Appl Environ Microbiol. 65(2):868–872.
- Farnaud S, Evans RW. 2003. Lactoferrin—a multifunctional protein with antimicrobial properties. Mol Immunol. 40(7):395–405.
- Fitzgerald SF, Beckett AE, Palarea-Albaladejo J, McAteer S, Shaaban S, et al. 2019. Shiga toxin sub-type 2a increases the efficiency of Escherichia coli O157 transmission between animals and restricts epithelial regeneration in bovine enteroids. PLoS Pathog. 15(10):e1008003.
- Flores-Villaseñor H, Canizalez-Román A, Velazquez-Roman J, Nazmi K, Bolscher JGM, et al. 2012. Protective effects of lactoferrin chimera and bovine lactoferrin in a mouse model of enterohaemorrhagic Escherichia coli O157: H7 infection. Biochem Cell Biol. 90(3):405–411.
- Forsythe SJ. 2020. The microbiology of safe food. Wiley.
- Garzón V, Bustos R-H, Pinacho DG. 2020. Personalized medicine for antibiotics: the role of nanobiosensors in therapeutic drug monitoring. J Pers Med. 10(4):147.
- Gerba CP. 2009. Environmentally transmitted pathogens. In: Environmental microbiology. Academic Press; p. 445–484.
- Goldwater PN, Bettelheim KA. 2012. Treatment of enterohemorrhagic Escherichia coli (EHEC) infection and hemolytic uremic syndrome (HUS). BMC Med. 10(1):1–8.
- Goumari MM, Farhani I, Nezafat N, Mahmoodi S. 2020. Multi-epitope vaccines (MEVs), as a novel strategy against infectious diseases. Curr Proteomics. 17(5):354–364.
- Govindarajan DK, Kandaswamy K. 2022. Virulence factors of uropathogens and their role in host pathogen interactions. Cell Surface. 8:100075.
- Govindarajan DK, Viswalingam N, Meganathan Y, Kandaswamy K. 2020. Adherence patterns of Escherichia coli in the intestine and its role in pathogenesis. Med Microecol. 5:100025.
- Grantina-Ievina L, Rodze I. 2020. Survival of pathogenic and antibiotic-resistant bacteria in vermicompost, sewage sludge, and other types of composts in temperate climate conditions. Biol Composts. 58:107.
- Haiwen Z, Rui H, Bingxi Z, Qingfeng G, Beibei W, et al. 2019. Cathelicidin-derived PR39 protects enterohemorrhagic Escherichia coli O157: H7 challenged mice by improving epithelial function and balancing the microbiota in the intestine. Sci Rep. 9(1):1–13.
- Hajra TK, Bag PK, Das SC, Mukherjee S, Khan A, et al. 2007. Development of a simple latex agglutination assay for detection of Shiga toxin-producing Escherichia coli (STEC) by using polyclonal antibody against STEC. Clin Vaccine Immunol. 14(5):600–604.
- Hall G, Kurosawa S, Stearns-Kurosawa DJ. 2017. Shiga toxin therapeutics: beyond neutralization. Toxins (Basel). 9(9):291.
- Haney EF, Lau F, Vogel HJ. 2007. Solution structures and model membrane interactions of lactoferrampin, an antimicrobial peptide derived from bovine lactoferrin. Biochim Biophys Acta (BBA)-Biomem. 1768(10):2355–2364.
- Hentschel V, Arnold F, Seufferlein T, Azoitei N, Kleger A, et al. 2021. Enteropathogenic infections: organoids go bacterial. Stem Cells Int. 2021.
- Hunter PR. 2003. Drinking water and diarrhoeal disease due to Escherichia coli. J Water Health. 1(2):65–72.
- Hwang S-B, Chelliah R, Kang JE, Rubab M, Banan-MwineDaliri E, et al. 2021. Role of recent therapeutic applications and the infection strategies of Shiga toxin-producing Escherichia coli. Front Cell Infect Microbiol. 11:450.
- Igwaran A, Iweriebor BC, Okoh AI. 2018. Molecular characterization and antimicrobial resistance pattern of Escherichia coli recovered from wastewater treatment plants in Eastern Cape South Africa. Int J Environ Res Public Health. 15(6):1237.
- Indraswari A, Suardana IW, Haryanto A, Widiasih DA. 2021. Molecular analysis of pathogenic Escherichia coli isolated from cow meat in Yogyakarta, Indonesia using 16S rRNA gene. Biodiversitas J Biol Divers. 22(10).
- Ishida W, Harada Y, Fukuda K, Fukushima A. 2016. Inhibition by the antimicrobial peptide LL37 of lipopolysaccharide-induced innate immune responses in human corneal fibroblasts. Invest Ophthalmol Visual Sci. 57(1):30–39.
- Jasion VS, Burnett BP. 2015. Survival and digestibility of orally-administered immunoglobulin preparations containing IgG through the gastrointestinal tract in humans. Nutr J. 14(1):22.
- Javadi M, Bouzari S, Oloomi M. 2017. Horizontal gene transfer and the diversity of Escherichia coli. In: Escherichia coli. Recent advances on physiology, pathogenesis and biotechnological applications. p. 317–331.
- Jia S, Zhang X. 2020. Biological HRPs in wastewater. In: High-Risk pollutants in wastewater. Elsevier; p. 41–78.
- Jia Z, Müller M, Le Gall T, Riool M, Müller M, et al. 2021. Multiplexed detection and differentiation of bacterial enzymes and bacteria by color-encoded sensor hydrogels. Bioact Mater. 6(12):4286–4300.
- Jubelin G, Desvaux M, Schüller S, Etienne-Mesmin L, Muniesa M, et al. 2018. Modulation of enterohaemorrhagic Escherichia coli survival and virulence in the human gastrointestinal tract. Microorganisms. 6(4):115.
- Jyoti A, Tomar RS. 2018. Biosensors: a potential tool for detection of microbial contaminants for food safety. In: Sustainable biological systems for agriculture. Apple Academic Press; p. 143–156.
- Kappler S, Ronan-Bentle S, Graham A. 2017. Thrombotic microangiopathies (TTP, HUS, HELLP). Hematology/Oncology Clinics. 31(6):1081–1103.
- Karmali MA. 2018. Factors in the emergence of serious human infections associated with highly pathogenic strains of shiga toxin-producing Escherichia coli. Int J Med Microbiol. 308(8):1067–1072.
- Katani R. 2016. Identification of the microbial factors contributing to super-shedding amongst isolates of Shiga toxin-producing Escherichia coli O157: H7. The Pennsylvania State University.
- Khadem EAH. 2019. The Impact of Bacterial Spoilage and Foodborne Pathogens on Beef Industry and Application of Antimicrobial Interventions.
- Khalid M, Andreoli S. 2019. Extrarenal manifestations of the hemolytic uremic syndrome associated with Shiga toxin-producing Escherichia coli (STEC HUS). Pediatr Nephrol. 34(12):2495–2507.
- Köckerling E, Karrasch L, Schweitzer A, Razum O, Krause G. 2017. Public health research resulting from one of the world’s largest outbreaks caused by entero-hemorrhagic Escherichia coli in Germany 2011: a review. Front Public Health. 5:332.
- Kumar Y, Bansal S, Jaiswal P. 2017. Loop-mediated isothermal amplification (LAMP): A rapid and sensitive tool for quality assessment of meat products. Compr Rev Food Sci Food Saf. 16(6):1359–1378.
- Kwun JW, Lee CH. 2007. Trends of recent food-borne disease outbreaks in Korea. J Korean Med Assoc. 50(7):573–581.
- Lee M-S, Tesh VL. 2019. Roles of Shiga toxins in immunopathology. Toxins (Basel). 11(4):212.
- Lianou A, Panagou EZ, Nychas G-JE. 2017. Meat safety—I Foodborne pathogens and other biological issues. In: Lawrie’s meat science. Woodhead; p. 521–552.
- Liu H, Dong H, Chen Z, Lin L, Chen H, et al. 2017. Magnetic nanoparticles enhanced microarray detection of multiple foodborne pathogens. J Biomed Nanotechnol. 13(10):1333–1343.
- Loneragan GH, Brashears MM. 2005. Pre-harvest interventions to reduce carriage of E. coli O157 by harvest-ready feedlot cattle. Meat Sci. 71(1):72–78.
- Lukaszczyk M, Pradhan B, Remaut H. 2019. The biosynthesis and structures of bacterial pili. In: Bacterial cell walls and membranes. p. 369–413.
- Madic J, de Garam CP, Vingadassalon N, Oswald E, Fach P, et al. 2010. Simplex and multiplex real-time PCR assays for the detection of flagellar (H-antigen) fliC alleles and intimin (eae) variants associated with enterohaemorrhagic Escherichia coli (EHEC) serotypes O26:H11, O103:H2, O111:H8, O145:H28 and O157:H7. J Appl Microbiol. 109(5):1696–1705.
- Maniha SM, Noor R. 2020. Genetic makeup and associated virulence posed by the enteropathogenic Escherichia coli and the enterotoxigenic Escherichia coli pathotypes. Biomed Biotechnol Res J. 4(4):280.
- McMahon TC, Blais BW, Wong A, Carrillo CD. 2017. Multiplexed single intact cell droplet digital PCR (MuSIC ddPCR) method for specific detection of enterohemorrhagic E. coli (EHEC) in food enrichment cultures. Front Microbiol. 8:332.
- Menge C. 2020. Molecular biology of Escherichia coli Shiga toxins’ effects on mammalian cells. Toxins (Basel). 12(5):345.
- Mercanoglu Taban B, Aytac SA. 2019. An evaluation of immunomagnetic separation-real-time PCR (IMS-RTiPCR) combined assay for rapid and specific detection of Escherichia coli O157: H7 in raw milk and ground beef. Food Sci Technol. 39:955–961.
- Mesele F, Abunna F. 2019. Escherichia coli O157: H7 in foods of animal origin and its food safety implications. Adv Biol Res (Rennes). 13(4):134–145.
- Michael M, Elliott EJ, Craig JC, Ridley G, Hodson EM. 2009. Interventions for hemolytic uremic syndrome and thrombotic thrombocytopenic purpura: a systematic review of randomized controlled trials. Am J Kidney Dis. 53(2):259–272.
- Moges B, Yinur D, Hassen A, Tessema TS. 2022. Designing of immunodiagnostic assay using polyclonal antibodies for detection of Shiga toxin producing pathogenic E. coli (STEC) strains. World J Microbiol Biotechnol. 38(11):1–14.
- Mühlen S, Dersch P. 2020. Treatment strategies for infections with Shiga toxin-producing Escherichia coli. Front Cell Infect Microbiol. 10:169.
- Mühlen S, Ramming I, Pils MC, Koeppel M, Glaser J, et al. 2020. Identification of antibiotics that diminish disease in a murine model of enterohemorrhagic Escherichia coli infection. Antimicrob Agents Chemother. 64(4):e02159–19.
- Naylor SW, Nart P, Sales J, Flockhart A, Gally DL, et al. 2007. Impact of the direct application of therapeutic agents to the terminal recta of experimentally colonized calves on Escherichia coli O157:H7 shedding. Appl Environ Microbiol. 73(5):1493–1500.
- Nesta B, Pizza M. 2018. Vaccines against Escherichia coli. Curr Top Microbiol Immunol. 213–242.
- Newell DG, La Ragione RM. 2018. Enterohaemorrhagic and other Shiga toxin-producing Escherichia coli (STEC): where are we now regarding diagnostics and control strategies? Transbound Emerg Dis. 65:49–71.
- Nihayati K, Rachmawati Y, Rokhim S, Prasetyaning L. 2020. Detection of Enterohemorrhagic Escherichia coli (EHEC) in Consumption Water Source using Multiplex PCR Method.
- Nimmanapalli R, Gupta V. 2020. Vaccines the tugboat for prevention-based animal production. In: Genomics and biotechnological advances in veterinary, poultry, and fisheries. Academic Press; p. 469–504.
- Nkiiri KG. 2017. Prevalence and Detection of Virulence Genes of Shigatoxigenic Escherichia coli Serotypes Isolated From Cattle Carcasses in Slaughter Houses in Nairobi, Kenya. Diss. COHES, JKUAT.
- Onuoha MN. 2017. Studies on the Extra-Human Distribution of Enterohaemorrhagic Escherichia Coli From Borno and Enugu States of Nigeria. Diss.
- Ori EL, Takagi EH, Andrade TS, Miguel BT, Cergole-Novella MC, et al. 2019. Diarrhoeagenic Escherichia coli and Escherichia albertii in Brazil: pathotypes and serotypes over a 6-year period of surveillance. Epidemiol Infect. 147:e10.
- Orsi N. 2004. The antimicrobial activity of lactoferrin: current status and perspectives. Biometals. 17(3):189–196.
- Pal M, Ayele Y. 2017. Public health significance of verotoxin-producing Escherichia coli O157:H7. EC Microbiol. 16:257–263.
- Pal RR, Baidya AK, Mamou G, Bhattacharya S, Socol Y, et al. 2019. Pathogenic E. coli extracts nutrients from infected host cells utilizing injectisome components. Cell. 177(3):683–696.
- Paletta ACC, Castro VS, Conte-Junior CA. 2020. Shiga toxin-producing and enteroaggregative Escherichia coli in animal, foods, and humans: pathogenicity mechanisms, detection methods, and epidemiology. Curr Microbiol. 77(4):612–620.
- Panwar H, Rokana N, Aparna SV, Kaur J, Singh A, et al. 2020. Gastrointestinal stress as innate defence against microbial attack. J Appl Microbiol. 130(4):1035–1061.
- Perry JD. 2017. A decade of development of chromogenic culture media for clinical microbiology in an era of molecular diagnostics. Clin Microbiol Rev. 30(2):449–479.
- Pienaar JA. 2019. Escherichia coli Survival Strategies in Simulated Gastric Fluid and the Possible Impact on Calculated Human Infectious Doses. University of Johannesburg (South Africa).
- Rajapaksha P, Elbourne A, Gangadoo S, Brown R, Cozzolino D, et al. 2019. A review of methods for the detection of pathogenic microorganisms. Analyst. 144(2):396–411.
- Ravan H, Norouzi A, Sanadgol N, Hosseinzadeh E. 2020. Colorimetric nanoplatform for visual determination of cancer cells via target-catalyzed hairpin assembly actuated aggregation of gold nanoparticles. Mikrochim Acta. 187(7):1–8.
- Ricke SC, Feye KM, Evan Chaney W, Shi Z, Pavlidis H, et al. 2019. Developments in rapid detection methods for the detection of foodborne campylobacter in the United States. Front Microbiol. 9:3280.
- Ruciaka PK. 2017. Isolation and molecular characterization of Escherichia coli from selected drinking water sources in Njoro sub county, Kenya. Diss. Egerton University.
- Saberianfar R, Chin-Fatt A, Scott A, Henry KA, Topp E, et al. 2019. Plant-produced chimeric VHH-sIgA against enterohemorrhagic E. coli intimin shows cross-serotype inhibition of bacterial adhesion to epithelial cells. Front Plant Sci. 10:270.
- Sadhu MJ, Bloom JS, Day L, Siegel JJ, Kosuri S, et al. 2018. Highly parallel genome variant engineering with CRISPR–Cas9. Nat Genet. 50(4):510–514.
- Saeedi P, Yazdanparast M, Behzadi E, Salmanian AH, Mousavi SL, et al. 2017. A review on strategies for decreasing E. coli O157:H7 risk in animals. Microb Pathog. 103:186–195.
- Sanchez-Villamil JI, Tapia D, Torres AG. 2022. Optimization of multivalent gold nanoparticle vaccines eliciting humoral and cellular immunity in an in vivo model of enterohemorrhagic Escherichia coli O157: H7 colonization.”. mSphere. 7(1):e00934–21.
- Sarowska J, Futoma-Koloch B, Jama-Kmiecik A, Frej-Madrzak M, Ksiazczyk M, et al. 2019. Virulence factors, prevalence and potential transmission of extraintestinal pathogenic Escherichia coli isolated from different sources: recent reports. Gut Pathog. 11(1):1–16.
- Shahreza S, Hossein M, Rahimi E, Momtaz H. 2017. Shiga-toxigenic Escherichia coli in ready-to-eat food staffs: prevalence and distribution of putative virulence factors. Microbiol Res (Pavia). 8(2):7244.
- Shanmugasundarasamy T, Govindarajan DK, Kandaswamy K. 2022. A review on pilus assembly mechanisms in Gram-positive and Gram-negative bacteria. Cell Surf. 8:100077.
- Sharma VK. 2006. Real-time reverse transcription-multiplex PCR for simultaneous and specific detection of rfbE and eae genes of Escherichia coli O157: H7. Mol Cell Probes. 20(5):298–306.
- Sharma VK, Dean-Nystrom EA. 2003. Detection of enterohemorrhagic Escherichia coli O157: H7 by using a multiplex real-time PCR assay for genes encoding intimin and Shiga toxins. Vet Microbiol. 93(3):247–260.
- Shiga EA, Guth BEC, Piazza RMF, Luz D. 2020. Comparative analysis of rapid agglutination latex test using single-chain antibody fragments (scFv) versus the gold standard Vero cell assay for Shiga toxin (Stx) detection. J Microbiol Methods. 175:105965.
- Shimizu M, Kuroda M, Sakashita N, Konishi M, Kaneda H, et al. 2012. Cytokine profiles of patients with enterohemorrhagic Escherichia coli O111-induced hemolytic-uremic syndrome. Cytokine. 60(3):694–700.
- Shumi E, Tolosa T, Abdurahaman M, Olani A, Lekew M, et al. 2021. Phenotypic characterization, antimicrobial susceptibility patterns profile and risk factors of Escherichia coli o157: H7 isolated from cattle meat at Jimma Ethiopia. Am J Biosci Bioeng. 9(2):40–48.
- Silva MA, Santos ARR, Rocha LB, Caetano BA, Mitsunari T, et al. 2019. Development and validation of Shiga toxin-producing Escherichia coli immunodiagnostic assay. Microorganisms. 7(9):276.
- Sivaramakrishnan M, Kothandan R, Govindarajan DK, Meganathan Y, Kandaswamy K. 2020. Active microfluidic systems for cell sorting and separation. Curr Opin Biomed Eng. 13:60–68.
- Slater SL, Frankel G. 2020. Advances and challenges in studying type III secretion effectors of attaching and effacing pathogens. Front Cell Infect Microbiol. 10:337.
- Smith JL, Fratamico PM. 2018. Emerging and re-emerging foodborne pathogens. Foodborne Pathog Dis. 15(12):737–757.
- Söderström A, Lindberg A, Andersson Y. 2005. EHEC o157 outbreak in Sweden from locally produced lettuce, August-September 2005. Weekly Releases (1997–2007). 10(38):2794.
- Song H, Zhang J, Qu J, Liu J, Yin P, et al. 2019. Lactobacillus rhamnosus GG microcapsules inhibit Escherichia coli biofilm formation in coculture. Biotechnol Lett. 41(8):1007–1014.
- Srivastava S, Singh PK, Vatsalya V, Karch RC. 2018. Developments in the diagnostic techniques of infectious diseases: rural and urban prospective. Adv Infect Dis. 8(3):121.
- Srividya A, Maiti B, Chakraborty A, Chakraborty G. 2019. Loop mediated isothermal amplification: a promising tool for screening genetic mutations. Mol Diagn Ther. 23(6):723–733.
- Surendran Nair M. 2017. Controlling Enterohemorrhagic E. coli O157: H7 using Selenium and Rutin. Diss. University of Connecticut.
- Swelam S, Amin A, Amer AA, ElMakarem H. 2017. Isolation of some food poisoning microorganisms from Raw milk with special reference to verocytotoxigenic E. coli. Alex J Vet Sci. 54(2).
- Tabashsum Z, Peng M, Bernhardt C, Patel P, Carrion M, et al. 2019. Synbiotic-like effect of linoleic acid overproducing Lactobacillus casei with berry phenolic extracts against pathogenesis of enterohemorrhagic Escherichia coli. Gut Pathog. 11(1):1–11.
- Takahashi M, Taguchi H, Yamaguchi H, Osaki T, Komatsu A, et al. 2004. The effect of probiotic treatment with Clostridium butyricum on enterohemorrhagic Escherichia coli O157: H7 infection in mice. FEMS Immunol Med Microbiol. 41(3):219–226.
- Travert B, Rafat C, Mariani P, Cointe A, Dossier A, et al. 2021. Shiga toxin-associated hemolytic uremic syndrome: specificities of adult patients and implications for critical care management. Toxins (Basel). 13(5):306.
- Ubeda C, Djukovic A, Isaac S. 2017. Roles of the intestinal microbiota in pathogen protection. Clin Transl Immunology. 6(2):e128.
- Umesha S, Manukumar HM. 2018. Advanced molecular diagnostic techniques for detection of food-borne pathogens: current applications and future challenges. Crit Rev Food Sci Nutr. 58(1):84–104.
- Van den Beld MJC, Rossen JWA, Evers N, Kooistra-Smid MAMD, Reubsaet FAG. 2022. MALDI-TOF MS using a custom-made database, biomarker assignment, or mathematical classifiers does Not differentiate Shigella spp. and Escherichia coli. Microorganisms. 10(2):435.
- Wang G, Huang S, Cai S, Yu H, Wang Y, et al. 2020. Lactobacillus reuteri ameliorates intestinal inflammation and modulates gut microbiota and metabolic disorders in dextran sulfate sodium-induced colitis in mice. Nutrients. 12(8):2298.
- Watahiki M, Isobe J, Kimata K, Shima T, Kanatani J-i, et al. 2014. Characterization of enterohemorrhagic Escherichia coli O111 and O157 strains isolated from outbreak patients in Japan. J Clin Microbiol. 52(8):2757–2763.
- Wei X, Zhang L, Zhang R, Koci M, Si D, et al. 2020. A novel cecropin-LL37 hybrid peptide protects mice against EHEC infection-mediated changes in gut microbiota, intestinal inflammation, and impairment of mucosal barrier functions. Front Immunol. 11:1361.
- Welinder-Olsson C, Stenqvist K, Badenfors M, Brandberg A, Florén K, et al. 2004. EHEC outbreak among staff at a children's hospital–use of PCR for verocytotoxin detection and PFGE for epidemiological investigation. Epidemiol Infect. 132(1):43–49.
- World Health Organization. 2020. GLASS whole-genome sequencing for surveillance of antimicrobial resistance.
- Xue-Han Z, Qing Y, Ya-Dong L, Bin L, Renata I, et al. 2013. Development of a LAMP assay for rapid detection of different intimin variants of attaching and effacing microbial pathogens. J Med Microbiol. 62:1665–1672.
- Yang S-C, Lin C-H, Aljuffali IA, Fang J-Y. 2017. Current pathogenic Escherichia coli foodborne outbreak cases and therapy development. Arch Microbiol. 199(6):811–825.
- Yinur D. 2019. Application and evaluation of LAMP assay for detection of enterohemorrhagic Escherichia coli (O157:H7). MSc. Thesis, Addis Ababa University, Addis Ababa.
- Zhao X, Li M, Liu Y. 2019. Microfluidic-based approaches for foodborne pathogen detection. Microorganisms. 7(10):381.
- Zimecki M, Artym J, Chodaczek G, Kocieba M, Kruzel ML. 2004. Protective effects of lactoferrin in Escherichia coli-induced bacteremia in mice: relationship to reduced serum TNF alpha level and increased turnover of neutrophils. Inflammation Res. 53(7):292–2296.