Abstract
Drought causes a decrease in tobacco yield and quality as well as a reduction in net photosynthetic rate. Salicylic acid (SA) is involved in the regulation of many physiological processes and has been widely used to improve plant drought tolerance. The hydroponic experiment was carried out on flue-cured tobacco variety K326. SA solutions (0.3 mM) were sprayed on the leaves every day for three days, after which 15% PEG-6000 was used to simulate moderate drought. The results showed that drought stress reduced the photosynthetic rate (Pn), stomatal conductance (Gs), and transpiration rate (Tr), while it enhanced the activities of superoxide dismutase (SOD), peroxidase (POD), catalase (CAT), malondialdehyde (MDA), proline and protein content. Compared with PEG drought stress, SA improved the Pn, Gs and Tr by 45.74%, 26.82%, and 52.22% respectively. Besides, the activities of SOD, POD, CAT, protein and proline content increased by 44.27%, 50.18%, 25%, 34.69% and 24.88%, respectively, while the MDA content decreased by 23.89%. Furthermore, SA spraying increased the drought tolerance of tobacco by modulating the expression level of the genes associated with photosynthesis, carbon metabolism, and photosynthesis-antenna proteins. Hence, SA spraying reduced the inhibition of drought on photosynthesis and stimulated the antioxidant system, thus improving the drought resistance in tobacco.
Introduction
Drought is one of the most severe environmental factors that adversely affect plant growth and development, as well as crop productivity and quality. Under drought stress conditions, many physiological and metabolic processes, such as photosynthesis, are negatively affected. Drought causes the accumulation of reactive oxygen species (ROS) in plants, resulting in membrane lipid peroxidation, photosynthetic rate reduction, and even plant death. Plants have evolved multifaceted adaptation mechanisms at the morphological, physiological, biochemical, and molecular levels to rapidly recognize and adapt to drought stress conditions (Shinozaki and Yamaguchi-Shinozaki Citation2006; Qi et al. Citation2018). These internal responses range from changes in photosynthetic activity to the development of antioxidant defenses that enhance drought tolerance (Gao et al. Citation2009). Tobacco (Nicotiana tabacum L.) is a model organism and a valuable economic crop. In recent years, drought stress has become the main factor limiting the productivity and quality of tobacco owing to dramatic climate change (Khan et al. Citation2019). In particular, drought stress occurring during the vigorous growth stage has the greatest impact on the yield and quality of tobacco leaves. During this stage, drought causes a decrease in pigment content, nitrate reductase activity, and net photosynthesis of tobacco leaves, thus leading to a decrease in yield and an increase in nitrate accumulation (Su et al. Citation2017). Therefore, the improvement of tobacco drought resistance and its physiological traits has become one of the main goals of tobacco breeders and agronomists.
Addition of exogenous regulatory substances is known to be an efficient way of improving plant resistance to abiotic stress. Melatonin, brassinosteroids, and methyl jasmonate have been widely used to improve plant stress resistance (Behnamnia et al. Citation2009; Sharma and Zheng Citation2019; Xiong et al. Citation2020). Salicylic acid (SA) is an essential plant growth regulator which plays an important role in regulating physiological processes, such as growth, photosynthesis, and other metabolic processes (Mohammadi et al. Citation2022; Sharma et al. Citation2023). Wang et al. (Citation2021) suggested that SA application remarkably decreased cadmium (Cd) accumulation in rice grains by modulating the genes expression level correlated with Cd translocation and accumulation under Cd stress. It is also reported that SA could alleviate salinity-induced inhibition of leaf growth and increase grain yield of barley and wheat (Pirasteh-Anosheh et al. Citation2022). Pirasteh-Anosheh et al. (Citation2020, Citation2023) also illustrated that SA improved crops production under saline conditions. In addition, SA also participated in relieving Cd stress and arsenic toxicity (Yordanova et al. Citation2017; Kaya et al. Citation2020). Prior research has generally confirmed that plants treated with SA exhibit better resistance to drought stress (Sohag et al. Citation2020; Ahmad et al. Citation2021; Khalvandi et al. Citation2021). Meanwhile, prior literature has shown that plants treated with SA exhibit better resistance to drought stress by increasing antioxidant enzyme activities (Maruri-López et al. Citation2019; Ahmad et al. Citation2021) as well as proline and soluble carbohydrate contents (Demiralay et al. Citation2013). One study reported that exogenous application of SA effectively improved the net photosynthesis rate (Pn), stomatal conductance (Gs), transpiration rate (Tr), and intercellular CO2 concentration (Ci) of Atractylodes lancea (Thumb.) DC., and it also protected its photosynthetic system and improved its photosynthetic efficiency (Wang et al. Citation2020). Another study revealed that foliar application of SA increased plant proline and soluble carbohydrate contents (Demiralay et al. Citation2013). However, to date, no in-depth genome-wide studies on the drought tolerance mechanisms of SA-treated tobacco seedlings at the transcriptional level have been carried out.
Transcriptome sequencing technology has been used to comprehensively study the gene functions of different species and specific biological processes at the mRNA level. It has clear advantages over other existing methods, and numerous studies have applied the RNA-seq method to assess plant drought responses and to explore the mechanism of plant response to drought. For example, global transcriptomic profiles of drought responses of rice (Yoo et al. Citation2017), maize (Lu et al. Citation2017), wheat (Li et al. Citation2019), soybean (Zhang et al. Citation2019), and tobacco (Khan et al. Citation2019) have been published. The objective of the present study was to comprehensively examine the effects of drought stress and SA treatment on the photosynthesis, secondary metabolites, and transcriptome of flue-cured tobacco, aiming to reveal the regulatory mechanism of SA and provide effective methods for increasing tobacco drought tolerance.
Materials and methods
Plant materials and drought treatment
A hydroponic experiment was carried out on substrate culture in a greenhouse located at the National Tobacco Cultivation & Physiology & Biochemistry Research Center of Henan Agricultural University (Zhengzhou, China). The seeds of flue-cured tobacco variety K326, provided by Yunnan Tobacco Company, were sown in a floating system in a greenhouse that maintained a 12/12 h light/dark cycle, 28/19°C light/dark temperature regime, an average photosynthetic photon flux density of 400 μmol m−2 s−1, and relative humidity of 65% – 80%. Six weeks later, well-rooted and same-sized tobacco seedlings (with 5–6 leaves) were selected and cultivated in 25 cm × 30 cm (diameter × depth) plastic pots (one plant per pot). The seedlings were cultivated in a Hoagland solution which contained 1 mmol/L NH4NO3, 1 mmol/L KNO3, 1 mmol/L Ca(NO3)2·4H2O, 1 mmol/L KH2PO4, 0.045 mmol/L H3BO3, 2 mmol/L MgSO4·7H2O, 0.01 µmol/L MnCl2·4H2O, 0.4 µmol/L Na2MoO4·2H2O, 0.8 µmol/L ZnSO4·7H2O, 0.3 µmol/L CuSO4·5H2O, 0.02µmol/L FeSO4·7H2O, and 0.02 µmol/L Na2-EDTA. The nutrient solutions were renewed once every three days. The collection of plant material was in accordance with relevant institutional, national, and international guidelines and legislation.
The seedlings were subjected to the treatment seven days after replanting (this seven-day period was added for their recovery). One-third of the seedlings were treated with SA. Distilled water was used to dissolve the SA. The SA solution was sprayed on the leaves at 6:00 pm every day for three days. Then, polyethylene glycol (PEG)-simulated water stress was applied. After three days, moderate drought stress was simulated at 6:00 pm using 15% (−0.388 MPa) PEG6000 treatment (Michel and Kaufmann Citation1973). The seedlings were grouped into three experimental test sets with 15 plants per treatment: CK (water + Hoagland nutrient solution), D (water + 15% PEG6000-treated nutrient solutions), and D + SA (0.3 mM SA + 15% PEG6000). Sampling and analysis were performed 14 h after the treatment. Fully expanded leaves (length > 5 cm, up to down, fourth leaf from the top) from the same position were sampled from three pots of each treatment, immediately frozen in liquid N2, and stored at – 80°C for RNA sequencing.
Phenotypic observation and photosynthesis parameters measurement
Morphological changes in tobacco plants in the different treatments were observed at 8:00 am (14 h after the treatment). The net Pn, Gs, Tr, and Ci were measured using a portable photosynthesis system (LI-COR Biotechnology, 6400XT, Lincoln, NE, USA) between 9:00 and 11:00 am These parameters were measured under the following conditions: 400 µmol m−2 s−1 photosynthetic photon flux density, leaf temperature of 28 ± 2°C, and relative humidity of 65 ± 5%.
Measurement of antioxidant enzyme activities and osmotic substance contents
Leaf lamina between the sixth and eighth side veins was sliced into small sections to determine the physiological and biochemical indices. Each treatment had three biological replicates. Fresh lamina tissues were cut into 2 mm × 5 mm pieces. The activities of superoxide dismutase (SOD), peroxidase (POD), and catalase (CAT) were measured according to previously described methods (Liu et al. Citation2020). Proline and malondialdehyde (MDA) contents were determined as described in Fan and Liang (Citation1999). About 1.0 g of each sample was frozen in liquid N2 and used to assay the soluble protein content according to Li et al. (Citation2000).
RNA extraction, preparation of cDNA library, and sequencing
Transcriptome sequencing and analysis were conducted by OE Biotech Co., Ltd. (Shanghai, China). Total RNA was extracted using the mirVana miRNA Isolation Kit (Ambion, Waltham, MA, USA) following the manufacturer’s protocol. RNA integrity was evaluated using an Agilent 2100 Bioanalyzer (Agilent Technologies, Santa Clara, CA, USA). The samples with the RNA integrity number (RIN) ≥ 7 were used for subsequent analysis. The libraries were constructed using the TruSeq Stranded mRNA LTSample Prep Kit (Illumina, San Diego, CA, USA) according to the manufacturer’s instructions. These libraries were then sequenced on the Illumina sequencing platform (HiSeqTM2500 or Illumina HiSeq X Ten), and 125 bp/150 bp paired-end reads were generated. Quality control was performed on the remaining reads using the NGS QC Toolkit (Patel and Jain Citation2012). Raw data (raw reads) were processed using the Trimmomatic software (Bolger et al. Citation2014). The reads containing poly-N and low-quality reads were removed to obtain clean reads. The clean reads were then mapped to the reference genome using hisat2 (Kim et al. Citation2015). After removing the low-quality data, the clean reads were mapped to the K326 tobacco reference genome (https://solgenomics.net/organism/Nicotiana_tabacum/genome) using HISAT 2.4 (Kim et al. Citation2015).
RNA-Seq analysis, gene ontology (GO) enrichment, and Kyoto encyclopedia of genes and genomes (KEGG) pathway analyses of differentially expressed genes (DEGs)
The transcript profiles of RNA-seq data were analyzed by calculating the read fragments per kilobase per million mapped reads (FPKM). The FPKM value of each gene was calculated using cufflinks (Trapnell et al. Citation2012). RNAs differential expression analysis was performed by DESeq2 (Love et al. Citation2014) software between two different groups. A p-value < 0.05 and fold change > 2 or < 0.5 were set as the thresholds for significantly differential expression. GO enrichment and KEGG pathway enrichment analyses of the DEGs were performed using R based on hypergeometric distribution (Kanehisa et al. Citation2007).
Statistical analysis
The figures were processed using Adobe Illustrator and GraphPad Prism (v. 8.0.1, GraphPad Software Inc., CA, USA), and correlation analysis and analysis of variance between the treatments were carried out using SPSS 20.0 (IBM, Palo Alto, CA, USA). The SD values of the three replicates were used for the error bars. The LSD multiple range test at p < 0.05 was used to carry out mean comparison among the treatments. All presented data are shown as the mean of three biological replicates (n = 3).
Results
Effect of SA on tobacco photosynthesis parameters
To evaluate the effects of SA on the photosynthesis of flue-cured tobacco seedlings, the Pn, Gs, Tr, and Ci were measured (Figure (a–d)). These parameters were significantly influenced by the imposed drought stress. In comparison with those in the control, Pn, Gs, and Tr were markedly decreased by 45.23%, 40.31%, and 59.67% in 15% polyethylene glycol (PEG)-treated seedlings, respectively, whereas Ci was increased by 9.59%. In contrast, SA-treated leaves had higher Pn, Gs, and Tr by 45.74%, 26.82%, and 61.37%, respectively, and lower Ci by 3.29%, compared with those of the seedlings treated with PEG alone.
Figure 1. (a) Pn. (b) Tr. (c) Gs. (d) Ci. Pn: net photosynthesis rate; Gs: stomatal conductance; Tr: transpiration rate; Ci: intercellular CO2 concentration. CK: water + Hoagland nutrient solution; D: water + 15% PEG6000-treated nutrient solutions; D + SA: 0.3 mmol/L SA + 15% PEG6000. Symbols of lower case letters indicate that the significant differences between treatments are at 0.05.
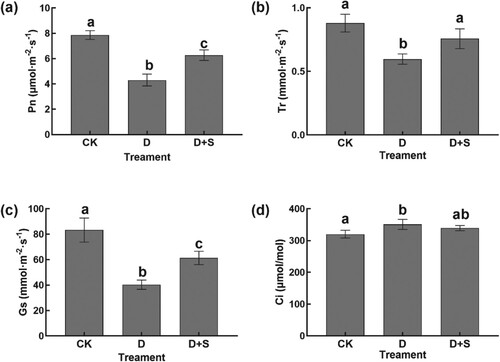
Reduction in MDA and increase in antioxidant enzyme activity and osmotic regulating substances after the SA treatment
Antioxidant enzymes play an important role in ROS scavenging, which has been proven to hinder the synthesis of PSII core D1 (Takahashi and Murata Citation2008). An increase in POD, SOD, and CAT activity, as well as proline and soluble protein contents, was observed in 15% PEG-treated tobacco seedlings compared to those in control (Figure (a–c, e–f)). In contrast, exogenous SA application led to a remarkable increase in POD, SOD, and CAT by 50.18%, 44.27%, and 26.23%, respectively, compared with those under PEG treatment alone (Figure (a–c, e–f)). Moreover, a sharp increase in MDA (the product of lipid peroxidation) was observed under drought stress (Figure (d)). The seedlings under 15% PEG drought stress had an 87.85% increase in MDA content compared to that of the control (Figure (d)). However, a significant reduction in MDA and increase in proline and soluble protein contents were observed in SA-supplemented drought-stressed seedlings compared to those in drought-stressed seedlings without SA supplementation, indicating that SA could improve the cell osmotic function and alleviate the damage caused by drought stress.
Data processing
Nine cDNA libraries were established for RNA-seq analysis. A total of 85.88M, 88.96M, and 89.26M raw reads were identified in the CK, D, and D + S samples, respectively; after data processing, 83.57M (97.31%), 86.43M (97.16%), and 86.91M (97.37%) million clean reads were obtained for further analysis, respectively (Table ). Overall, the mapping rates of all samples to the reference genome were above 95%, and the GC content of all samples was stable, with a distribution ranging from 43.28 to 44.13%. The QC30 values of all samples were above 95%, indicating successful library construction and RNA sequencing.
Table 1. Statistics of transcriptome sequencing data.
Gene expression and correlation between samples
After filtering the raw reads, a high rate of clean reads was obtained for each sample. FPKM expression levels in each sample were calculated. In addition, Pearson’s correlation coefficient and principal component analysis (PCA) of the data profiles from all nine samples revealed a high correlation among all samples (Supplementary Figure S1). These results demonstrated that the sequencing data used in the present study were adequately representative and valid.
Differentially expressed gene (DEG) selection, gene ontology (GO) enrichment, and Kyoto encyclopedia of genes and genomes (KEGG) pathway analyses of the DEGs
We identified DEGs with fold change (FC) > 2 or FC < 0.5 and a p-value < 0.05. A total of 6229 DEGs (2914 upregulated and 3315 downregulated) were identified between the D group and the control group, and 4840 DEGs (1944 upregulated and 2896 downregulated) were identified between the D + S group and the D group (Supplementary Figure S2).
Gene ontology (GO) is a standardized system used for functional classification of genes according to three domains: biological processes, cellular components, and molecular functions. The DEGs in D-vs-CK were further analyzed using GO and Kyoto Encyclopedia of Genes and Genomes (KEGG) analyses (Figure (a–d)). Almost 5.97% of the downregulated genes were correlated with the biological processes (GO-BP) of photosynthesis (GO:0015979), chlorophyll biosynthetic process (GO:0015995), and chloroplast organization (GO:0009658). In the cellular component domain (GO-CC), the downregulated genes were significantly enriched in thylakoid (GO:0009579), chloroplast (GO:0009507), and photosystem II components (GO:0009523). Genes related to molecular function (GO-MF), such as pigment binding (GO:0031409), rRNA binding (GO:0019843), and chlorophyll binding (GO:0016168), were also downregulated. The KEGG pathway analysis also showed that the downregulated DEGs were mostly involved in photosynthesis (ko00195) and porphyrin and chlorophyll metabolism (ko00860). However, in D + S-vs-D, almost all DEGs in the categories of molecular function, biological process, and cellular component were upregulated, which was consistent with the results of the KEGG pathway analyses.
GO enrichment and KEGG pathway analyses of the DEGs in response to SA
To explore the DEGs in tobacco seedlings under drought stress after spraying with SA, a Venn diagram analysis between D-vs-CK down and D + S-vs-D up was conducted (Figure (a)). GO and KEGG analyses were then conducted to examine the potential gene functions and metabolic pathways of the DEGs (Figure (b–c)). The Venn analyses showed that 1098 DEGs were enriched between D-vs-CK down and D + S-vs-D up. Genes involved in biological processes were mostly enriched in photosynthesis (GO:0015979), photosynthesis light reaction (GO:0019684), and photosynthesis light harvesting (GO:0009765). High values of cellular component categories, such as chloroplast (GO:0009507), thylakoid (GO:0009579), photosynthetic membrane (GO:0034357), photosystem I (GO:0009522), and photosystem II (GO:0009523), were observed. Genes involved in molecular functions were mostly enriched in chlorophyll binding (GO:0016168). The KEGG pathway analysis showed that the genes were significantly enriched in the photosynthesis-antenna protein (ko00196), photosynthesis (ko00195), carbon fixation in photosynthetic organisms (ko00710), carbon metabolism (ko01200), starch and sucrose metabolism (ko00500), porphyrin and chlorophyll metabolism (ko00860), and carotenoid biosynthesis pathways (ko00906).
DEGs involved in photosynthesis and carbon metabolism
Transcriptome sequencing technology can provide a large amount of information on the DEGs involved in specific biological responses. Our results showed that carbon metabolism, porphyrin and chlorophyll metabolism, and photosynthesis were lower in PEG-treated tobacco than in well-watered tobacco (Figure (a–e)), which was consistent with the low Pn in PEG-treated tobacco seedlings. The expression levels of genes involved in carbon metabolism, such as FBP (Nitab4.5_0002152g0090), those involved in photosynthesis, such as PSBO (Nitab4.5_0000108g0110), PSBR (Nitab4.5_0002475g0030), and PSAO (Nitab4.5_0003068g0060), and those involved in photosynthesis-antenna proteins, such as IHCA-P4 (Nitab4.5_0001128g0010), LHC4.2 (Nitab4.5_0000476g0110), and CAB50 (Nitab4.5_0000592g0320), were consistently suppressed under drought stress in flue-cured tobacco and upregulated after SA spraying. To explore the reason why SA was able to alleviate the damage caused by drought stress in flue-cured tobacco, we also analyzed the differences in porphyrin and chlorophyll metabolism gene expression levels of. The results showed that the genes of POR1 (Nitab4.5_0002526g0100), HEMC (Nitab4.5_0001791g0020), HEMA1 (Nitab4.5_0000377g0190), and CHLM (Nitab4.5_0000976g0010) were consistently and significantly downregulated under drought stress and upregulated in SA-treated tobacco (Figure (d)), which may also be the reason for the higher photosynthesis in SA-treated tobacco. Supplementary Table S1-S5 provides information on the pathways and DEGs.
Figure 5. (a) Expression level of genes related to photosynthesis; (b) Expression of genes related to photosynthesis-antenna proteins; (c) Gene expression related to porphyrin and chlorophyll metabolism; (d) Expression levels of genes related to carbon metabolism; (e) Expression pattern of genes related to starch and sucrose metabolism.
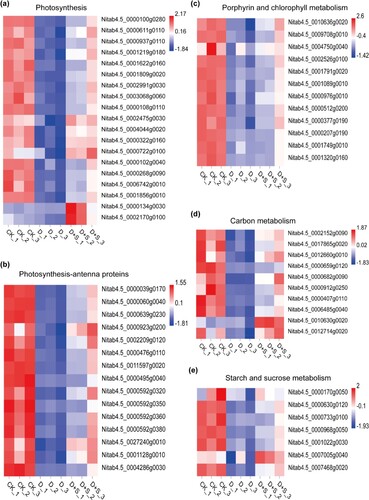
Discussion
In recent years, drought stress has become one of the most serious environmental factors affecting the productivity and quality of tobacco. Our investigation revealed that the application of SA protected the tobacco seedlings from the damage caused by drought stress and its corresponding mechanisms; this finding can have significant practical applications. Under drought stress conditions, a large amount of ROS accumulates inside the plant, resulting in increased MDA production, cell membrane damage, cell aging, and in some cases, plant death (Sohag et al. Citation2020; Ahmad et al. Citation2021). Therefore, MDA levels are used as indicators of cellular component damage. Plants have evolved multifaceted adaptation mechanisms to rapidly recognize and adapt to drought stress conditions; these mechanisms include an increase in antioxidant enzyme activities and osmotic regulatory substances (Rajput et al. Citation2021). In wheat, exogenous SA application has been reported to play an important role in improving the activity of antioxidant enzymes and decreasing the MDA content (Ma et al. Citation2014; Shemi et al. Citation2021). As important osmotic regulators in plants, proline and soluble proteins can improve the cell osmotic function and alleviate the damage caused by drought stress (Ozturk et al. Citation2021). Positive effects of SA in triggering the synthesis of soluble proteins and proline to improve plant drought resistance were reported by Lu et al. (Citation2020). Ahmad et al. (Citation2021) reported that SA had a beneficial effect on plant growth by increasing antioxidant enzyme activities and membrane stability. Khalvandi et al. (Citation2021) reported that foliar application of SA increased the antioxidant enzyme activities (SOD, POD, and CAT) and reduced the MDA content under stress conditions. In ROS-scavenging systems, antioxidant enzymes play the key roles, with SOD catalyzing the dismutation of the O2 radical to O2 and H2O2, which are then scavenged by CAT and POD (Miller et al. Citation2010). In our study, an increase in MDA, soluble protein, and proline contents, as well as an increase in antioxidant enzyme activities, were observed in PEG drought-stressed tobacco. However, SA application decreased the MDA content and enhanced the antioxidant enzyme activities, soluble protein and proline accumulation in tobacco plants under drought stress conditions (Figure (a–f)). The decrease in MDA content in SA-treated plants may be associated with the induction of antioxidant responses, which protect the plant from damage. Beside antioxidant enzymes, SA treatment helped to overcome oxidative stress in drought-stressed tobacco plants by proline accumulation, since proline acts as ROS scavenger. And Sharma et al. (Citation2023) suggested that exogenous application of SA improves proline content due to increasing the activity of pyrroline-5-carboxylate reductase, which eventually elevates synthesis of proline, by converting pyrroline-5-carboxylate to proline. These results proved that SA can enhance plant antioxidant defense systems and increase the levels of osmotic-regulating substances to eliminate ROS, which is in accordance with our observation that ROS and MDA levels were markedly reduced in plants after the SA treatment.
Photosynthesis is the key factor affecting plant yield and plays an important role in crop growth. Also, Pn is regarded as an effective indicator of plant drought resistance (Pérez-López et al. Citation2012). Previous studies have reported that the decrease in photosynthesis is usually caused by stomatal limitation when both Gs and Ci decline, whereas non-stomatal limitation was found to be the main reason for the decrease in photosynthesis when Ci increased and Gs decreased (Pérez-López et al. Citation2012; Zhou et al. Citation2013). SA is part of several plant processes and its role in improving Pn has already been reported. For example, water deficit reduced CO2 assimilation, while SA application increased gas exchange characteristics in plants (Shemi et al. Citation2021). Saheri et al. (Saheri et al. Citation2020) also demonstrated that foliar application of SA improved the adaptation of the purslane plant to drought stress by improving photosynthetic pigments and CO2 assimilation. Khalvandi et al. (Khalvandi et al. Citation2021) reported that SA treatments effectively alleviated the negative effects of drought stress on Sardari ecotypes through improving the photosynthetic performance. In the present study, the data clearly showed a reduction in Pn, Tr, and Gs accompanied by an increase in Ci under PEG-drought stress (Figure (a–d)). These results indicated that non-stomatal limitation primarily led to a decrease in Pn under PEG-induced drought stress. While SA application significantly reversed this trend under PEG-drought stress, Shemi et al. (Citation2021) reported that the Chla, Chlb, and total Chl contents, as well as the relative water content (RWC), were improved by SA, which is beneficial for enhancing the Pn of tobacco. Khalvandi et al. (Citation2021) concluded that drought stress caused damage to the membrane system and led to a decline in Pn, whereas SA treatments effectively ameliorated the negative effects of drought stress by improving photosynthetic performance. This SA-induced increase in photosynthetic performance results from the enhancement in activities of rubisco and rubisco activase enzymes under drought resistance conditions (Shao et al. Citation2018).
The decrease in photosynthesis can lead to plants absorbing more light energy than the level necessary for photosynthetic carbon fixation. This excess energy has the potential to trigger an increase in ROS production, which has been proven to damage the photosystems, decrease the activity of the photosynthetic electron transport chain, and hinder pigment synthesis (Wang et al. Citation2018). To better understand the mechanism of SA-induced increase in photosynthesis rate, molecular technologies were used in the present study. Under drought stress, most of the downregulated DEGs were correlated with the chlorophyll biosynthetic process, photosynthesis, light harvesting, chloroplast, and photosystem II (Figure (a)). Our KEGG pathway analysis also showed that the downregulated DEGs were mainly enriched in starch and sucrose metabolism, photosynthesis-antenna proteins, and porphyrin and chlorophyll metabolism (Figure (c)). However, the application of SA increased the expression of genes related to photosystem II, photosystem I, chloroplast thylakoid, photosynthetic electron transport chain, chloroplast thylakoid membrane, carbon fixation, and synthesis of carotenoids (Figure (b, d)), indicating that it was effective in protecting the cell membrane and photosystems from damage. This can consequently enhance the photosynthesis rate and pigment synthesis. Further investigation of the downregulated DEGs between watered and drought-treated plants as well as the upregulated DEGs between drought-treated and SA-sprayed plants suggested that SA application promoted pigment biosynthesis, photosynthesis, and carbon fixation, and protected the photosystems from damage (Figure (a–c)). In addition, some key genes related to photosynthesis, photosynthesis-antenna proteins, porphyrin and chlorophyll metabolism, and carbon metabolism were upregulated after SA application (Figure (a–e)). These include the RCA1 genes, which play an important role in the activation of the rubisco enzyme (Nagarajan and Gill Citation2018), lhcA-P4, which encodes a key subunit of the light-harvesting complex and converts light energy into chemical energy (Jansson Citation1999), AGPS1 and SS1, which catalyze the limiting steps in starch synthesis (Chunduri et al. Citation2021), as well as photosystem II genes (PSBO, PSBR, PSBS), photosystem I genes (PSAF, PSAG, PSAK, PSAO), POR1, and CHLP, which play crucial roles in chlorophyll biosynthesis. Rubisco, a key enzyme in the Calvin cycle, assimilates atmospheric CO2 into the biosphere (Lo et al. Citation2019). A previous study showed that the overexpression of rubisco activase gene (RCA) was able to maintain photosynthesis under drought stress, which contributed to the higher drought tolerance in transgenic plants (Wijewardene et al. Citation2020). Therefore, we speculated that enhanced transcription of RCA mRNA under drought condition caused an increase in the photosynthesis after SA treatment. Together, these gene products may contribute to the adaptation or tolerance of tobacco to drought stress.
Conclusion
In conclusion, drought stress significantly decreased photosynthesis characteristics due to nonstomatal limitations and increased the MDA content. However, SA exerts a positive effect on photosynthesis and antioxidant enzymes under drought condition, which occurs mainly through stimulating the genes involved in photosynthesis, photosynthesis-antenna proteins, photosystem II, photosystem I, carbon metabolism, porphyrin, and chlorophyll metabolism. Therefore, we concluded that SA application under drought stress is an effective method for alleviating the damage caused by drought stress. Further research on the cellular signaling is necessary to obtain more data about how SA treatment interacts with drought effects in tobacco plants. Moreover, further such investigations as field trials and natural drought experiments could be beneficial in developing a sustainable crop production system.
Supplemental Material
Download MS Word (482.1 KB)Acknowledgments
We would like to thank Editage (www.editage.cn) for English language editing. The pre-print of the study can be available online at: https://assets.researchsquare.com/files/rs-406971/v1/90e24f8b-b0ac-4e5a-8c02-330b6f501e5d.pdf?c=1633410856. Author contributions: SHZ performed the conception and design of the research. FYQ and ZYY conducted the research and investigation process. ZJ and LYF analyzed the data and prepared the figures1-6. ZJ and FYQ checked the data. FYQ drafted the manuscript. SHZ completed the revision of manuscript for important intellectual content. All authors read and approved the final manuscript.
Disclosure statement
No potential conflict of interest was reported by the author(s).
Data availability statement
The sequencing data were deposited in the National Center of Biotechnology Information database (https://www.ncbi.nlm.nih.gov/bioproject/PRJNA747725). The rest of the raw data that support the findings of this study are openly available in Science Data Bank at https://doi.org/10.57760/sciencedb.08474.
Additional information
Funding
References
- Ahmad A, Aslam Z, Naz M, Hussain S, Javed T, Aslam S, Raza A, Ali HM, Siddiqui MH, Salem MZM, et al. 2021. Exogenous salicylic acid-induced drought stress tolerance in wheat (Triticum aestivum L.) grown under hydroponic culture. PloS one. 16(12):e0260556. doi:10.1371/journal.pone.0260556.
- Behnamnia M, Kalantari KM, Ziaie J. 2009. The effects of brassinosteroid on the induction of biochemical changes in Lycopersicon esculentum under drought stress. Turk J Botany. 33(6):417–428. doi:10.3906/bot-0806-12.
- Bolger AM, Lohse M, Usadel B. 2014. Trimmomatic: a flexible trimmer for Illumina sequence data. Bioinformatics. 30(15):2114–2120. doi:10.1093/bioinformatics/btu170.
- Chunduri V, Kaur A, Kaur S, Kumar A, Sharma S, Sharma N, Singh P, Kapoor P, Kaur S, Kumari A, et al. 2021. Gene expression and proteomics studies suggest an involvement of multiple pathways under day and day–night combined heat stresses during grain filling in wheat. Front Plant Sci. 12: 66046. doi:10.3389/fpls.2021.660446.
- Demiralay M, Sag˘lam A, Kadioglu A. 2013. Salicylic acid delays leaf rolling by inducing antioxidant enzymes and modulating osmoprotectant content in Ctenanthe setosa under osmotic stress. Turk J Biol. 37(1):49–59. doi:10.3906/biy-1205-16.
- Fan SQ, Liang SW. 1999. Guidance of modern plant physiology. Shanghai: Science Press; p. 303–306.
- Gao DH, Gao Q, Xu HY, Ma F, Zhao CM, Liu JQ. 2009. Physiological responses to gradual drought stress in the diploid hybrid Pinus densata and its two parental species. Trees. 23(4):717–728. doi:10.1007/s00468-009-0314-3.
- Jansson S. 1999. A guide to the Lhc genes and their relatives in Arabidopsis. Trends Plant Sci. 4:236–240. doi:10.1016/S1360-1385(99)01419-3.
- Kanehisa M, Araki M, Goto S, Hattori M, Hirakawa M, Itoh M, Katayama T, Kawashima S, Okuda S, Tokimastu T, Yamanishi Y. 2007. KEGG for linking genomes to life and the environment. Nucleic Acids Res. 36(suppl 1):D480–D484. doi:10.1093/nar/gkm882.
- Kaya C, Ashraf M, Alyemeni MN, Corpas FJ, Ahmad P. 2020. Salicylic acid-induced nitric oxide enhances arsenic toxicity tolerance in maize plants by upregulating the ascorbate-glutathione cycle and glyoxalase system. J Hazard Mater. 399:123020. doi:10.1016/j.jhazmat.2020.123020.
- Khalvandi M, Siosemardeh A, Roohi E, Keramati S. 2021. Salicylic acid alleviated the effect of drought stress on photosynthetic characteristics and leaf protein pattern in winter wheat. Heliyon. 7(1):e05908. doi:10.1016/j.heliyon.2021.e05908.
- Khan R, Zhou PL, Ma XH, Zhou L, Wu YY, Ullah Z, Wang SS. 2019. Transcriptome profiling, biochemical and physiological analyses provide new insights towards drought tolerance in Nicotiana tabacum L. Genes. 10(12):1041. doi:10.3390/genes10121041.
- Kim D, Langmead B, Salzberg SL. 2015. HISAT: a fast spliced aligner with low memory requirements. Nat Methods. 12(4):357–360. doi:10.1038/nmeth.3317.
- Li HJ, Yang Y, Chang P, Zheng JC, Chen L, Hu YG. 2019. Transcriptome and photosynthetic trait analysis of TaER over-expression wheat under drought stress. J Triticeae Crops. 39(08):941–949.
- Li HS, Sun Q, Zhao SJ, Zhang WH. 2000. Principle and technology of plant physiological and biochemical experiments. Beijing: Higher Education Press.
- Liu HL, Piao XM, Xu WH, Bai XF, Cheng ZH, Jin F, Wang L, Han YZ. 2020. Effect of low temperature control in greenhouse on cold tolerance of high quality rice at seedling stage. North Rice. 50(06):27–29. doi:10.16170/j.cnki.1673-6737.2020.06.008.
- Lo SC, Huang CC, Ho TY, Yang YT. 2019. Detailed profiling of carbon fixation of in silico synthetic autotrophy with reductive tricarboxylic acid cycle and Calvin-Benson-Bassham cycle in Esherichia coli using hydrogen as an energy source. Synthetic and Systems Biotechnology. 4(3):165–172. doi:10.1016/j.synbio.2019.08.003.
- Love MI, Huber W, Anders S. 2014. Moderated estimation of fold change and dispersion for RNAseq data with DESeq2. Genome Biol. 15(12):1–21. doi:10.1186/s13059-014-0550-8.
- Lu X, Zhou X, Cao Y, Zhou MX, Mcneil D, Liang S, Yang CW. 2017. RNA-Seq analysis of cold and drought responsive transcriptomes of Zea mays ssp. mexicana L. Front Plant Sci. 8:136. doi:10.3389/fpls.2017.00136.
- Lu YZ, Li N, Zhang EH, Yin X, Zhao KT, Lan XZ. 2020. Effects of soaking seeds with salicylic acid on seed germination and drought resistance of Dracocephalum tanguticum. J Henan Agric Sci. 49(03):63–69. doi:10.15933/j.cnki.1004-3268.2020.03.009.
- Ma C, Wang ZQ, Zhang LT, Sun MM, Lin TB. 2014. Photosynthetic responses of wheat (Triticum aestivum L.) to combined effects of drought and exogenous methyl jasmonate. Photosynthetica. 52(3):377–385. doi:10.1007/s11099-014-0041-x.
- Maruri-López I, Aviles-Baltazar NY, Buchala A, Serrano M. 2019. Intra and extracellular journey of the phytohormone salicylic acid. Front Plant Sci. 10:423. doi:10.3389/fpls.2019.00423.
- Michel BE, Kaufmann MR. 1973. The osmotic potential of polyethylene glycol 6000. Plant Physiol. 51(5):914–916. doi:10.1104/pp.51.5.914.
- Miller G, Suzuki N, Ciftci-Yilmaz S, Mittler R. 2010. Reactive oxygen species homeostasis and signalling during drought and salinity stresses. Plant Cell Environ. 33(4):453–467. doi:10.1111/j.1365-3040.2009.02041.x.
- Mohammadi H, Rahimpour B, Pirasteh-Anosheh H, Race M. 2022. Salicylic acid manipulates ion accumulation and distribution in favor of salinity tolerance in Chenopodium quinoa. Int J Environ Res Public Health. 19(3):1576. doi:10.3390/ijerph19031576.
- Nagarajan R, Gill KS. 2018. Evolution of Rubisco activase gene in plants. Plant Mol Biol. 96(1):69–87. doi:10.1007/s11103-017-0680-y.
- Ozturk M, Turkyilmaz Unal B, García-Caparrós P, Khursheed A, Gul A, Hasanuzzaman M. 2021. Osmoregulation and its actions during the drought stress in plants. Physiol Plant. 172(2):1321–1335. doi:10.1111/ppl.13297.
- Patel RK, Jain M. 2012. NGS QC toolkit: a toolkit for quality control of next generation sequencing data. PLoS One. 7(2):e30619. doi:10.1371/journal.pone.0030619.
- Pérez-López U, Robredo A, Lacuesta M, Mena-Petite A, Muñoz-Rueda A. 2012. Elevated CO2 reduces stomatal and metabolic limitations on photosynthesis caused by salinity in Hordeum vulgare. Photosynth Res. 111(3):269–283. doi:10.1007/s11120-012-9721-1.
- Pirasteh-Anosheh H, Emam Y, Hashemi SE, Gaur A. 2020. Chapter 9 - Role of chlormequat chloride and salicylic acid in improving cereal crops production under saline conditions. In: Improving cereal productivity through climate smart practices. Sawston: Woodhead Publishing Series in Food Science, Technology and Nutrition; p. 145–158. doi:10.1016/B978-0-12-821316-2.00009-1.
- Pirasteh-Anosheh H, Rahimpour B, Mohammadi H, Ranjbar G, Race M. 2023. Induced salinity tolerance by salicylic acid through physiological manipulations. In: Phytohormones and stress responsive secondary metabolites. San Diego: Academic Press; p. 99–109. doi:10.1016/B978-0-323-91883-1.00017-6.
- Pirasteh-Anosheh H, Ranjbar G, Hasanuzzaman M, Khanna K, Bhardwaj R, Ahmad P. 2022. Salicylic acid-mediated regulation of morpho-physiological and yield attributes of wheat and barley plants in deferring salinity stress. J Plant Growth Regul. 41(3):1291–1303. doi:10.1007/s00344-021-10358-7.
- Qi JS, Song CP, Wang BS, Zhou JM, Kangasjärvi J, Zhu JK, Gong ZZ. 2018. Reactive oxygen species signaling and stomatal movement in plant responses to drought stress and pathogen attack. J Integr Plant Biol. 60(9):805–826. doi:10.1111/jipb.12654.
- Rajput VD, Singh RK, Verma KK, Sharma L, Quiroz-Figueroa FR, Meena M, Gour VS, Minkina T, Sushkova S, Mandzhieva S. 2021. Recent developments in enzymatic antioxidant defence mechanism in plants with special reference to abiotic stress. Biology. 10(4):267. doi:10.3390/biology10040267.
- Saheri F, Barzin G, Pishkar L, Boojar MMA, Babaeekhou L. 2020. Foliar spray of salicylic acid induces physiological and biochemical changes in purslane (Portulaca oleracea L.) under drought stress. Biologia. 75(12):2189–2200. doi:10.2478/s11756-020-00571-2.
- Shao RX, Xin LF, Guo JM, Zheng HF, Mao J, Han XP, Jia L, Du CG, Song R, Yang QH, Elmore RW. 2018. Salicylic acid-induced photosynthetic adaptability of Zea mays L. to polyethylene glycol-simulated water deficit is associated with nitric oxide signaling. Photosynthetica. 56:1370–1377. doi:10.1007/s11099-018-0850-4.
- Sharma A, Kohli SK, Khanna K, Ramakrishnan M, Kumar V, Bhardwaj R, Brestic M, Skalicky M, Landi M, Zheng BS. 2023. Salicylic acid: A phenolic molecule with multiple roles in salt-stressed plants. J Plant Growth Regul. 42 (2):1–25. doi:10.1007/s00344-022-10902-z.
- Sharma A, Zheng BS. 2019. Melatonin mediated regulation of drought stress: physiological and molecular aspects. Plants. 8(7):190. doi:10.3390/plants8070190.
- Shemi R, Wang R, Gheith ES, Hussain HA, Hussain S, Irfan M, Cholidah L, Zhang KP, Zhang S, Wang LC. 2021. Effects of salicylic acid, zinc and glycine betaine on morpho-physiological growth and yield of maize under drought stress. Sci Rep. 11(1):1–14. doi:10.1038/s41598-021-s82264-7.
- Shinozaki K, Yamaguchi-Shinozaki K. 2006. Gene networks involved in drought stress response and tolerance. J. Exp. Bot. 58(2):221–227. doi:10.1093/jxb/erl164.
- Sohag AAM, Tahjib-Ul-Arif M, Brestic M, Afrin S, Sakil MA, Hossain MT, Hossain MA, Hossain MA. 2020. Exogenous salicylic acid and hydrogen peroxide attenuate drought stress in rice. Plant Soil Environ. 66(1):7–13. doi:10.17221/472/2019-PSE.
- Su XH, Wei FJ, Huo YJ, Xia ZL. 2017. Comparative physiological and molecular analyses of two contrasting flue-cured tobacco genotypes under progressive drought stress. Front Plant Sci. 8:827. doi:10.3389/fpls.2017.00827.
- Takahashi S, Murata N. 2008. How do environmental stresses accelerate photoinhibition? Trends Plant Sci. 13(4):178–182. doi:10.1016/j.tplants.2008.01.005.
- Trapnell C, Roberts A, Goff L, Pertea G, Kim D, Kelley DR, Pimentel H, Salzberg SL, Rinn JL, Pachter L. 2012. Differential gene and transcript expression analysis of RNA-Seq experiments with TopHat and cufflinks. Nat Protoc. 7:562–578. doi:10.1038/nprot.2012.016.
- Wang F, Sang XH, Gu W, Chao JG, Zhou C, Tian R. 2020. Effects of salicylic acid on photosynthetic characteristics and physiological indexes of Atractylodes lancea (Thumb. DC). under drought stress. Mol Plant Breed. 18(09):3060–3067. doi:10.13271/j.mpb.018.003060.
- Wang FJ, Tan HF, Huang LH, Cai C, Ding YF, Bao H, Chen ZX, Zhu C. 2021. Application of exogenous salicylic acid reduces Cd toxicity and Cd accumulation in rice. Ecotoxicol Environ Saf. 207:111198. doi:10.1016/j.ecoenv.2020.111198.
- Wang ZB, Li GF, Sun HQ, Ma L, Guo YP, Zhao ZY, Gao H, Mei LX. 2018. Effects of drought stress on photosynthesis and photosynthetic electron transport chain in young apple tree leaves. Biol Open. 7:11. doi:10.1242/bio.035279.
- Wijewardene I, Mishra N, Sun L, Smith J, Zhu XL, Payton P, Shen GX, Zhang H. 2020. Improving drought-, salinity-, and heat-tolerance in transgenic plants by co-overexpressing Arabidopsis vacuolar pyrophosphatase gene AVP1 and Larrea Rubisco Activase gene RCA. Plant Sci. 296:110499. doi:10.1016/j.plantsci.2020.110499.
- Xiong B, Wang Y, Zhang Y, Ma MM, Gao YF, Zhou ZY, Wang BZ, Wang T, Lv XL, Wang X, et al. 2020. Alleviation of drought stress and the physiological mechanisms in Citrus cultivar (Huangguogan) treated with methyl jasmonate. Biosci Biotechnol Biochem. 84(9):1958–1965. doi:10.1080/09168451.2020.1771676.
- Yoo YH, Chandran AKN, Park JC, Gho YS, Lee SW, An G, Jung KH. 2017. OsPhyB-mediating novel regulatory pathway for drought tolerance in rice root identified by a global RNA-Seq transcriptome analysis of rice genes in response to water deficiencies. Front Plant Sci. 8:580. doi:10.3389/fpls.2017.00580.
- Yordanova R, Baydanova V, Peeva V. 2017. Nitric oxide mediates the stress response induced by cadmium in maize plants. Genetics Plant Physiol. 7(3-4):121–134.
- Zhang CL, Cao S, Man LL, Xiang DJ, Liu P. 2019. Analysis of PEG stress on drought tolerance and related response genes expression in soybean seedlings of two varieties. Mol Plant Breed. 17(18):5891–5898. doi:10.13271/j.mpb.017.005891.
- Zhou SX, Duursma RA, Medlyn BE, Kelly JW, Prentice IC. 2013. How should we model plant responses to drought? An analysis of stomatal and non-stomatal responses to water stress. Agric For Meteorol. 182-183:204–214. doi:10.1016/j.agrformet.2013.05.009.