Abstract
Ameloblastomas are benign tumors associated with recurrence and morbidity. Mutations in the V-Raf murine sarcoma viral oncogene homolog B1 (BRAF) and smoothened (SMO) genes have been identified in ameloblastomas. The mutation rate of BRAF in different regions and mutation sites of SMO remains controversial. This study assesses the relationship between these mutations and clinicopathological features of ameloblastoma in Chinese patients. Ameloblastomas were surgically removed from 30 patients and BRAF gene polymorphisms were detected using DNA sequencing analysis. We also examined the relationship between BRAF or SMO mutations and clinicopathological parameters. BRAF V600E mutation was detected in 18 of 30 ameloblastomas. No significant correlation was found between BRAF V600E mutation and age, sex, location, histologic type, capsular invasion, or tumor recurrence. Five cases carried an SMO T364N mutation in exon 5, whereas six carried an SMO S590 T mutation in exon 10. The SMO mutation rate in patients aged ≤ 20 years was significantly higher than that in patients aged > 20 years. SMO T364N and S590 T mutations were closely related to age and capsular invasion. These findings indicate that BRAF V600E, SMO T364N, and SMO S590 T mutations play important roles in the pathogenesis of ameloblastoma.
Key highlights
The BRAF V600E mutation was highly prevalent in Chinese patients with ameloblastoma.
SMO T364N and S590 T mutations are closely related to age and capsular invasion.
The BRAF V600E, SMO T364N, and SMO S590 T mutations play important roles in the pathogenesis of ameloblastoma.
Introduction
Ameloblastoma is a benign odontogenic neoplasm that is locally infiltrative and is associated with a high risk of recurrence; however, its etiology is not fully understood (Siar et al. Citation2015; Ghafouri-Fard et al. Citation2021). Surgical excision of the tumor remains the standard treatment for ameloblastomas (Petrovic et al. Citation2018). When surgical resection is conservative, the recurrence rate is significantly higher; however, complete jaw resection can result in significant facial deformity (Srivastava et al. Citation2022). When the tumor is located in the maxilla, it may have a growth that makes the surgical procedure difficult, especially if it is close to the base of the skull, and may even compromise the life of the patient (Boffano et al. Citation2021). Therefore, it is essential to explore newer treatment methods.
Kurppa et al. (Citation2014) reported that the V-Raf murine sarcoma viral oncogene homolog B1 (BRAF) mutation (V600E), at a rate of 63%, was found in ameloblastoma cases. Novel molecular findings have provided new insights into the mechanisms, pathogenesis, and management of ameloblastomas (Kokubun et al. Citation2022; Yoshimoto et al. Citation2022). Most studies have reported no significant association between the BRAF V600E mutation and any clinicopathological features (do Canto et al. Citation2019; Derakhshan et al. Citation2020; Kokubun et al. Citation2022; Kunmongkolwut and Chaisuparat Citation2022); however, data on the mutation rate and relationship between mutation occurrence, clinical pathology, and biological behavior have been inconsistent.
Sweeney et al. (Citation2014) reported smoothened (SMO) gene mutation rate as 39%. Additional studies have focused on SMO gene mutations at the L412F and W535L mutation sites, but the mutation site and rate were reported to be different (Brown et al. Citation2014; Diniz et al. Citation2015; Heikinheimo et al. Citation2019). Gültekin et al. (Citation2018) reported that SMO mutations are predominantly observed in maxillary tumors, and SMO-mutated tumors are more common in older patients. Thus, the mutation rate of SMO and its relationship with clinicopathological characteristics remain to be explored.
Interestingly, SMO and BRAF mutations tend to be mutually exclusive in ameloblastomas (Brown et al. Citation2014). Kokubun et al. (Citation2022) recently performed a simultaneous search for BRAF and SMO L412F mutations in ameloblastoma specimens and reported a high frequency of BRAF mutations (83%). However, the SMO L412F mutation was not observed in these populations. The different results of previous studies indicate that further investigation is necessary to determine the association between SMO gene mutations and ameloblastoma.
Therefore, this study investigated BRAF V600E and SMO mutations in ameloblastomas, and evaluated the relationship between these mutations and various clinicopathological features.
Patients and methods
Study participants and samples
This study was conducted in accordance with the tenets of the Declaration of Helsinki, after obtaining approval from the Ethics Committee of the Hospital of Stomatology, Hebei Medical University [No.(2021)006]. All patients gave their informed consent at the time of hospitalization for the surgical specimens to be stored and later used for research purposes. Additional informed consent was then obtained from patients prior to the commencement of this study through phone calls or in person.
Thirty patients diagnosed with ameloblastoma who visited the Hospital of Stomatology, Hebei Medical University, between January 2006 and December 2016, were included in this study. All patients underwent local bone resection, including that of the lesion. The specimens were divided into two groups. The first piece of each specimen was fixed in 10% formalin without decalcification, embedded in paraffin, and serially sectioned at 4 μm thickness for histopathological diagnosis. The second piece was immediately frozen in liquid nitrogen and stored at −80 °C for later use; only these were used for DNA sequencing analysis studies.
The pathological diagnosis of ameloblastoma was confirmed by two pathologists. The tissues were diagnosed based on the WHO classification of odontogenic tumors (Soluk-Tekkesin and Wright Citation2022). Histologically, the 30 cases of ameloblastoma included 10 follicular, 9 plexiform, and 11 unicystic types.
Complete clinicopathological data of all patients were collected. Ten normal human gingival mucosa samples were used as controls. These samples were from impacted or embedded teeth extra gingival flaps. Written, informed consent was obtained, and was covered by the ethics committee approval [No.(2021)006].
Preparation of DNA template
Genomic DNA was extracted using a QIAamp DNA Mini Kit (QIAGEN, Hilden, Germany), according to the manufacturer’s instructions.
Primer design and synthesis
A mismatch primer (BRAF M) was designed based on BRAF gene mutation V600E. The 3’ end of the primer contained a mismatch site; thus, the wild-type BRAF gene could not be amplified. Thromboxane A synthase 1 (TBXAS1) was selected for further analyses. This gene was used as an internal reference gene, which differed in length from the BRAF V600E-specific mutation product. It was then used for denaturing high-performance liquid chromatography analysis. Primers (BRAF N) containing the V600E mutation site in exon 15 of BRAF and all 12 exons of SMO, were designed according to previous studies (Wang et al. Citation2013; Rui et al. Citation2014) using Primer 5.0 (Premier Biosoft Inc., Palo Alto, CA, USA) (Tables and ).
Table 1. Primer sequence of BRAF gene for DHPLC and Sanger sequencing.
Table 2. Primer sequence of SMO gene.
Polymerase chain reaction (PCR)
BRAF and TBXAS1 genes were subjected to multiplex PCR in one amplification system, with a reaction volume of 25 μl. A GoTaq Green System was used as the reaction system and a total of 12.5 μl of BRAF M, and 0.5 μl (0.2 μM) of upstream and downstream TBXAS1 primers were used.
The following PCR amplification mix was used: 25 μl of GoTaq Green Master Mix (Promega, Madison, WI, USA); 1.5 μl (0.3 μM) primer F; 1.5 μl (0.3 μM) primer R; 5 μl (250 ng) DNA template; and 17 μl of nuclease-free water. The PCR cycling conditions were as follows: 30 cycles of pre-denaturation for 2 min at 95 °C; 95 °C for 30 s, 58 °C for 30 s, and 72 °C for 30 s (30 cycles); and a final extension at 72 °C for 10 min. PCR products were electrophoresed on a 2% agarose gel for 2.5 h at 60 V, and visualized and photographed using a gel imaging system (Bio-Rad ChemiDoc; USA).
Denaturing high-performance liquid chromatography (DHPLC) detection
PCR products were denatured at 95 °C for 10 min, followed by slow reduction (at a rate of −0.5 °C/20 s) to room temperature. Then, 5μl of the PCR product was injected into DNASep® of the WAVEDNA Fragment Analysis System (Transgenomic, Omaha, NE, USA), and equilibrated using 0.1 mol/l triethylammonium acetate in a DHPLC column. DNA was removed from the column at a constant flow rate of 0.9 ml/min using a linear acetonitrile gradient, achieved by mixing buffer A with buffer B. Buffer A and B contained 0.1 M triethylammonium acetate and 25% acetonitrile, respectively. BRAF V600E heteroduplex was separated from the homoduplex at 50 °C. Eluted DNA was spectrophotometrically detected at a wavelength of 260 nm within 10 min. All results were analyzed using the WAVEMAKERTM software (Transgenomic, Omaha, NE, USA).
Polymerase chain reaction-single-strand conformation polymorphism (PCR-SSCP) detection
Five microliters of the PCR product were mixed with 5 μl of SSCP loading buffer dye and denatured at 95 °C for 10 min, snap-frozen on ice for 5 min, and loaded onto 10% polyacrylamide gels. Gels were run at 250 V for 6 min and then at 60 V for 12–14 h in a buffer containing 1× Tris-borate-EDTA buffer (TBE). The polyacrylamide gel plate was removed and rinsed three times with double-distilled water for approximately 15 s. The gel was then fixed in silver stain fixative solution for 6 min, washed thrice with double distilled water for 10 s, stained with 0.1% silver nitrate for 20 min, washed with double distilled water three to five times, and the color was developed in chromogenic solution for 7 min. The above steps were carried out on a shaker, protected from light, in stop solution for 10 min, then rinsed with water, and the DNA bands were visualized using a lightbox. Amplified products of the normal gingival mucosa were used as controls. If the electrophoretic bands increased, decreased, or shifted in the tumor specimen, it was considered an abnormal swimming band, indicating the presence of gene mutations in the sample.
DNA sequencing analysis
Isolated PCR samples were purified using the SK1131 Gel Extraction Kit (Sangon Biotech Co., Ltd., Shanghai, China), according to the manufacturer's instructions. Subsequently, DNA was dissolved in 12 μl of template suppression reagent, centrifuged at 10,000 rpm for 1 min at 4 °C, heated to 95 °C for 2 min, and quenched on ice. Capillaries were installed according to the instrument operation manual and a sequencing file was established. The instrument automatically filled the capillaries with gel. A DNA electrophoresis system (ABI 3770 XL DNA Analyzer; Applied Biosystems, Inc., Carlsbad, CA, USA) was used to detect and analyze the sequencing ladder. Data collection and image analysis were performed using software supplied by the model 3730 DNA sequencer (Applied Biosystems, Foster City, CA, USA).
Statistical analyses
The Chi-square test and Fisher’s exact test were used to analyze the relationship between gene mutations and clinicopathological features of ameloblastoma. Statistical significance was set at P < 0.05. Statistical analysis was performed using SPSS 25.0 (SPSS, Inc., Chicago, IL, USA).
Results
Clinicopathological features
A total of 16 males and 14 females aged 15–60 years formed the study population. Of the 30 cases, 27 involved the mandible, while 3 involved the maxilla. Tumor recurrence was observed in 11 patients (36.7%), with an average recurrence time of 2.59 years and a range of 10 months to 6 years after surgery. Five (16.7%) cases showed intracapsular invasion of the tumor cells.
DHPLC detection
The same band was detected in the PCR amplification products of the ameloblastoma and gingiva via electrophoresis. The BRAF V600E gene mutation curve showed two elution peaks with DHPLC: the first peak was the TBXAS1 gene amplification product (100 bp), and the second was the BRAF mutated gene amplification product (126 bp). Positive and negative controls were used. The BRAF V600E mutation was detected in 18 of 30 (60%) cases using DHPLC (Figure (A–E)).
Figure 1. (A). PCR amplification of exon 15 of BRAF gene. M: DNA marker, 1: negative control, 2: gingiva, 3–7: ameloblastoma specimens; (B). DHPLC chromatograms of exon 15 of BRAF gene with no mutation: negative control; (C). DHPLC chromatograms of exon 15 of BRAF gene with V600E mutation: positive control; (D). DHPLC chromatograms of exon 15 of BRAF gene in normal gingiva; (E). DHPLC chromatograms of exon 15 of BRAF gene mutation in ameloblastoma specimens; (F). Sequencing analysis of exon 15 of BRAF gene in normal gingiva (arrow, GTG); (G). Sequencing analysis of exon 15 of BRAF gene in ameloblastoma specimens (arrow, GAG). PCR, polymerase chain reaction
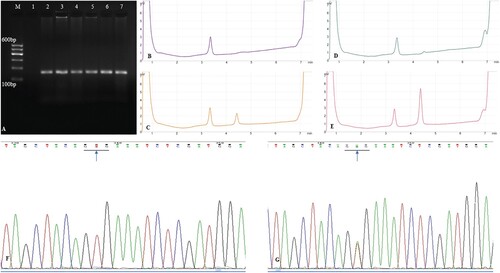
PCR-SSCP detection
The same band was detected in the PCR amplification products of the ameloblastoma and gingiva via electrophoresis. Of the 30, 9 cases of abnormal SSCP migration bands were detected in exons 3, 5, 6, and 10. No altered SSCP bands were detected in other exons or normal gingiva (Figure (A–H)).
Figure 2. (A). PCR amplification of exon 3 of SMO gene. M: DNA marker, 1: negative control, 2: gingiva, 3–7: ameloblastoma specimens; (B). PCR amplification of exon 5 of SMO gene. M: DNA marker, 1: negative control, 2: gingiva, 3–7: ameloblastoma specimens; (C). PCR amplification of exon 6 of SMO gene. M: DNA marker, 1: negative control, 2: gingiva, 3–7: ameloblastoma specimens; (D). PCR amplification of exon 10 of SMO gene. M: DNA marker, 1: negative control, 2: gingiva, 3–7: ameloblastoma specimens; (E). PCR-SSCP analysis of exon 3 of SMO gene. M: DNA marker, 1: negative control, gingiva: 2–8: ameloblastoma specimens. The result of lane 3 cannot be interpreted. Abnormal migrational bands (white arrow); (F). PCR-SSCP analysis of exon 5 of SMO gene. M: DNA marker, 1: negative control, gingiva: 2–8: ameloblastoma specimens. Abnormal migrational bands (white arrow); (G). PCR-SSCP analysis of exon 6 of SMO gene. M: DNA marker, 1: negative control, gingiva: 2–8: ameloblastoma specimens. Abnormal migrational bands (white arrow)’; (H). PCR-SSCP analysis of exon 10 of SMO gene. M: DNA marker, 1: negative control, gingiva: 2–8: ameloblastoma specimens. Abnormal migrational bands (white arrow). PCR-SSCP, polymerase chain reaction-single-strand conformation polymorphism; PCR, polymerase chain reaction
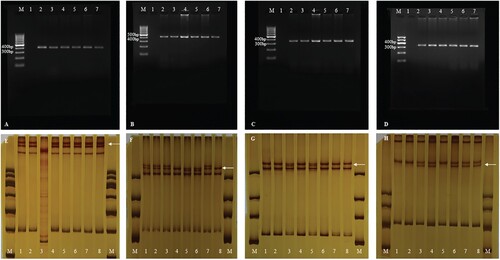
DNA sequencing analysis
BRAF DNA sequencing analysis
BRAF V600E mutation was detected in 18 (60%) of the 30 ameloblastoma specimens. and no BRAF V600E mutation was detected in the normal gingiva. The BRAF V600E mutation is a T1799A transversion that results in the substitution of valine with glutamic acid at codon 600 (V600E) (Figure (F, G)).
SMO DNA sequencing analysis
A synonymous SMO mutation, from A to G, was located at nucleotide position 808 in exon 2, which did not result in an amino acid change at E176E. The SMO E194E mutation from A to G, which was located at nucleotide position 862 of exon 3, was detected in nine cases. Of the 11 mutations in exon 5, five were identified as missense mutations from C to A: position 1371, T364N, while six were synonymous mutations from G to A: position 1417 and A379A. Twelve SMO mutations, from A to G, were located at nucleotide position 1444 of exon 6 and did not result in an amino acid change at G383G. In exon 10, six cases were identified with SMO missense mutations from G to C: position 2049 and S590 T (Figures and ; Table ).
Figure 3. (A). Sequencing analysis of exon 2 of SMO gene in wild type ameloblastoma specimens; (B). Sequencing analysis of exon 2 of SMO gene in mutated ameloblastoma specimens (blue arrow, GAA→GAG); (C). Sequencing analysis of exon 3 of SMO gene in wild type ameloblastoma specimens; (D). Sequencing analysis of exon 3 of SMO gene in mutated ameloblastoma specimens (blue arrow, GAA→GAG); (E). Sequencing analysis of exon 5 of SMO gene in wild type ameloblastoma specimens; (F). Sequencing analysis of exon 5 of SMO gene in mutated ameloblastoma specimens (blue arrow, GCG→GCA).
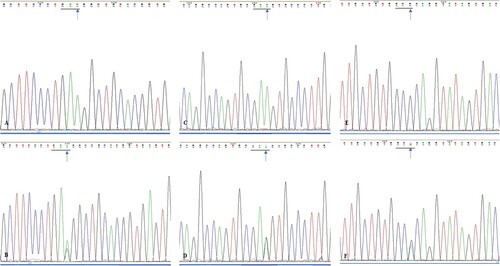
Figure 4. (A). Sequencing analysis of exon 5 of SMO gene in wild type ameloblastoma specimens; (B). Sequencing analysis of exon 5 of SMO gene in mutated ameloblastoma specimens (blue arrow, ACC→AAC); (C). Sequencing analysis of exon 6 of SMO gene in wild type ameloblastoma specimens; (D). Sequencing analysis of exon 6 of SMO gene in mutated ameloblastoma specimens (blue arrow, GGC→GGG); (E). Sequencing analysis of exon 10 of SMO gene in wild type ameloblastoma specimens; (F). Sequencing analysis of exon 10 of SMO gene in mutated ameloblastoma specimens (blue arrow, AGC→ACC).
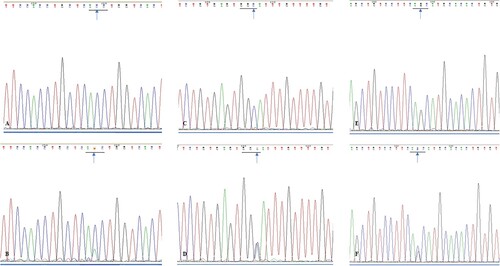
Table 3. SMO gene mutations in ameloblastoma.
Clinicopathological features and mutations
Clinicopathological features and BRAF mutation
No significant correlation was found between BRAF V600E mutation and age, sex, location, histological type, capsular invasion, or tumor recurrence (Table ).
Table 4. Relation between BRAF gene mutations and clinical pathology in 30 cases.
Clinicopathological features and SMO mutations
No significant correlation was found between SMO mutations and sex, location, histological type, or tumor recurrence. However, SMO mutations were closely related to age and capsular invasion. The SMO mutation rate in patients aged ≤ 20 years (100%) was significantly higher than that in patients aged > 20 years (29.6%) (P < 0.05). The SMO mutation rate in cases with capsular invasion (80.0%) was significantly higher than in cases with non-capsular invasion (28.0%) (P < 0.05) (Table ).
Table 5. Relation between SMO gene mutations and clinical pathology in 30 cases.
Discussion
Ameloblastoma is a benign, slow-growing, and locally invasive tumor (Soltani et al. Citation2018). A novel treatment approach might help in avoiding extensive surgical treatment. Several genes have been investigated in patients with ameloblastomas. Kurppa et al. (Citation2014) reported that the BRAF V600E mutation was found in 15 of 24 ameloblastoma cases. Since then, research on this mutation in ameloblastoma has continued to increase. This mutation induces the homeostatic activation of BRAF; the mitogen-activated protein kinase/extracellular signal-regulated kinase (MAPK/ERK) pathway is intimately involved in regulating the proliferative potential of tumor cells (Guimarães et al. Citation2021). BRAF V600E mutations occur in 33.3–94% of ameloblastomas (Kurppa et al. Citation2014; Sweeney et al. Citation2014; Shirsat et al. Citation2018; Heikinheimo et al. Citation2019; Derakhshan et al. Citation2020). The presence and prevalence of the BRAF V600E mutation in ameloblastomas have been reported in patients from various regions (Kurppa et al. Citation2014; Heikinheimo et al. Citation2019; Derakhshan et al. Citation2020). This study reported a high frequency (60%, 18/30) of BRAF V600E mutation in the Chinese population. Factors that might affect the mutation rate include the gene itself and other factors, such as race and ethnicity; specimen processing factors, such as freshly frozen tumor specimens or paraffin-embedded specimens; tumor cells; detection techniques, such as immunohistochemistry, quantitative real-time PCR analysis, and Sanger sequencing technology (Oh et al. Citation2019). Additional studies in patients from different races using the same detection method and preservation conditions could help evaluate the relationship between the BRAF V600E mutation rate and race in ameloblastoma. Meanwhile, because the number of specimens was limited in our study, further validation with a larger number of samples is warranted.
Many studies have focused on the relationship between the BRAF V600E mutation and clinicopathological features. Divergence also exists in terms of age, location, and recurrence in patients with ameloblastomas. Owosho et al. (Citation2021) reported that BRAF V600E mutation preferentially occurs in mandibular ameloblastomas, especially non-plexiform ameloblastomas. Meanwhile, Togni et al. (Citation2022) reported a significant association between the BRAF V600E mutation and tumor development at the mandibular site (ρ = 0.627; P < 0.001) and unicystic histological type.
BRAF V600E mutations in ameloblastomas have reportedly been correlated with younger age and mandibular predilection (Bonacina et al. Citation2022). Shirsat et al. (Citation2018) reported the lowest frequency (33.3%) of BRAF V600E immunoexpression among mandibular ameloblastomas in an Indian population. Moreover, BRAF V600E immunoexpression is significantly associated with recurrence, and plexiform pattern ameloblastomas without BRAF V600E mutations show an increased rate of local recurrence (Heikinheimo et al. Citation2019).
Our study showed no correlation between the BRAF V600E mutation and age, sex, location, histological subtype, capsular invasion, or clinical recurrence. This finding is consistent with that of previous studies (Oh et al. Citation2019; Derakhshan et al. Citation2020). The BRAF V600E mutation was detected in 10 of the 12 recurrent cases (83.3%). However, this finding remains controversial. The statistical data of postoperative recurrence could have been biased due to different tumor sizes and locations, inconsistent surgical methods, and the length of the postoperative follow-up period. Future research should overcome these limitations and ensure a larger sample size, for more definitive results.
The hedgehog (HH) pathway is an important signaling pathway involved in the regulation of embryonic development, tissue and organ formation, stem cell differentiation, and tumorigenesis (Mota and Shevde Citation2020). Smoothened (SMO) gene/protein is a co-receptor in the HH signaling pathway, located at 7q32.3, comprises 12 exons, and participates in the formation of a 787-amino acid transmembrane glycoprotein (Rui et al. Citation2014). SMO activation initiates glioma (GLI)-dependent expression of downstream target genes through complex translocation processes (Malatesta et al. Citation2013). However, the canonical HH signaling pathway involves GLI transcription factors, and its sequence similarity with class A G-protein coupled receptors (GPCRs) is less than 10% (Wang et al. Citation2013). Aberrant HH signaling results in tumorigenesis and supports aggressive phenotypes of human cancers, such as neoplastic transformation, tumor progression, metastasis, and drug resistance (Doheny et al. Citation2020). Moreover, gene mutations are the driving force of tumorigenesis and progression (Bisogno and Keene Citation2018). Environmental and genetic carcinogenic factors can cause DNA damage, resulting in the activation of oncogenes and/or inactivation of tumor suppressor genes, which triggers cascade reactions and over-proliferation of cells, ultimately resulting in tumor formation (Abu-Ghazaleh et al. Citation2021). Several critical components are involved in the HH signaling pathway, including SMO, which are expressed at relatively higher levels during tooth development, indicating that the SMO gene involved in the HH signaling pathway is closely related to odontogenic epithelium and odontogenesis (Hosoya et al. Citation2020). In addition, sonic HH signaling has been reported to be upregulated in adamantinomatous craniopharyngiomas (Gomes et al. Citation2015). Craniopharyngiomas, derived from the remnants of Rathke's pouch or a misplaced enamel organ, are also comparable to odontogenic tumors (Lee and Kim Citation2013). Thus, activation of the HH pathway is important for embryogenesis. It can also be speculated that SMO may be related to the occurrence of odontogenic tumors.
Brown et al. conducted a genetic study of 84 ameloblastoma specimens and reported the SMO L412F mutation in four, the W535L mutation in three, and the G416E mutation in one specimen (Brown et al. Citation2014). Sweeney et al. (Citation2014) reported that 39% (11/28) of tumors had SMO mutations, including the L412F mutation in ten cases and the W535L mutation in one case. The authors also found that the majority (8/10) of plexiform variants had an SMO mutation. Moreover, SMO mutations exhibited a marked preponderance in maxillary ameloblastomas (9/11 cases), and a trend toward earlier recurrence of tumors with SMO mutations has been noted. Gültekin et al. (Citation2018) found SMO mutations in eight of 57 ameloblastomas (14%). They reported the exclusive occurrence of BRAF mutations in mandibular tumors. Moreover, SMO mutations are predominantly observed in maxillary tumors, and SMO-mutated tumors are more common in older patients.
However, SMO L412F and W535L mutations in ameloblastomas have not been observed in several studies, including ours (Diniz et al. Citation2015; Shimura et al. Citation2020; Kokubun et al. Citation2022). Since the entire exon of the SMO gene was sequenced in this study for the first time, the same mutation sites as those reported in previous studies were not found. Therefore, there is no value in discussing clinicopathological features and comparing them with those reported in previous literature. The relationship between SMO gene mutations and sex, location, histological type, and tumor recurrence was not statistically significant; however, SMO mutations are closely related to age and capsule invasion. The mutation rate of SMO in patients ≤ 20 years of age (3/3) was higher than that in patients > 20 years of age (8/27). Moreover, the mutation rate of SMO in cases with tumor cell envelope invasion (4/5) was higher than that in cases without envelope invasion (7/25).
Our study identified two SMO missense mutations [T364N in exon 5 (5/30) and S590 T in exon 10 (6/30)] and four synonymous mutations [E176E in exon 2(1/30), E194E in exon 3(9/30), A379A in exon 5(6/30), and G388G in exon 10 (12/30)]. A previous study on SMO gene mutations in odontogenic keratocysts reported that the mutation sites were concentrated in exons 2, 3, 5, 6, and 10, indicating that the genetic status of these exons may be related to the occurrence of odontogenic tumors (Rui et al. Citation2014). Similar SMO mutation sites have been reported in other studies of odontogenic keratocysts, including synonymous and missense mutations of E194E, T364N, A379A, G388G, and S590 T (Rui et al. Citation2014), which provides more evidence for the inference that specific sites of the SMO gene play important roles in the development of odontogenic epithelium and the formation of odontogenic tumors. However, the frequency of mutations found in this study was different from that of odontogenic keratocysts (Rui et al. Citation2014), and there are new sites that indicate differences in the occurrence and biological behavior of ameloblastomas and odontogenic keratocysts.
Interestingly, BRAF V600E mutation and the L412F and W535L mutations in the SMO gene are mutually exclusive (Sweeney et al. Citation2014). Our study showed that 8 of the 11 tumor specimens with missense SMO mutations (T364N, S590T) also had a BRAF V600E mutation. These findings contradict those of previous studies (Sweeney et al. Citation2014). This inconsistency may have been due to different gene mutation sites, selection of different tumor specimens, differences in the number of research cases, and errors in the interpretation of experimental conditions. Therefore, it is necessary to evaluate additional cases of ameloblastomas in the future.
PCR-SSCP analysis was used to detect SMO polymorphisms. The co-occurrence rate between PCR-SSCP analysis and DNA sequencing results was 94.8%. The PCR-SSCP method is an effective method for screening gene polymorphisms in laboratory settings (Zhang et al. Citation2017). DHPLC is used for high-throughput screening of DNA sequence variations (Kim et al. Citation2013). This method has several advantages, such as high-throughput detection, high efficiency, high sensitivity, and specificity. The sensitivity of DHPLC was 99.9%, which is comparable to that of direct sequencing. However, at present, the overall cost of the equipment remains relatively high. In this study, the detection results of BRAF V600E mutation in 30 cases of ameloblastoma and ten cases of gingival mucosa were consistent with the results of direct sequencing, and the detection results were clear and reliable.
Due to the limited number of tumor samples, this study strictly screened the follicular type and plexiform type in the histological classification of ameloblastoma, the cystic type did not separate specific subtypes. The relationship between the ratio of mutations and clinicopathological characteristics may still need to be further studied, based on further increasing the number of samples to refine the pathological classification. At the same time, since the effect of protein expression after gene mutation is not involved, the relationship between gene mutation and protein expression is also an issue that needs to be paid attention to in future research.
Conclusions
BRAF and SMO mutations in 30 Chinese patients with ameloblastoma were detected, of which, the BRAF V600E mutation was observed in 18. Moreover, we found that two missense mutations [T364N (5/30) and S590T (6/30)] in the SMO gene and BRAF V600E mutation with SMO T364N and S590T mutations, were not mutually exclusive. SMO mutations were closely associated with age and capsular invasion. Since the association between clinicopathological features and BRAF V600E and SMO mutations in ameloblastoma remains questionable, further studies are required to identify molecular targets, and explain the relationship between these mutation sites and the clinicopathological features of ameloblastoma.
Author contributions
Wang Jie and Zhang Xudong designed the study. Li Xiangjun and Sun Xu provided the clinical ameloblastoma specimens. Li Hexiang, Hou Yali and Wang Jie were involved in tumor diagnosis. Chen Ruixue and Zhang Xudong conducted all the experiments and prepared the manuscript. All authors have contributed to the discussion and approval of the manuscript.
Disclosure statement
No potential conflict of interest was reported by the authors.
Data availability statement
The data that support the findings of this study are available in the Zenodo repository at https://doi.org/10.5281/zenodo.8267153.
Additional information
Funding
References
- Abu-Ghazaleh N, Chua WJ, Gopalan V. 2021. Intestinal microbiota and its association with colon cancer and red/processed meat consumption. J Gastroenterol Hepatol. 36(1):75–88. doi:10.1111/jgh.15042.
- Bisogno LS, Keene JD. 2018. RNA regulons in cancer and inflammation. Curr Opin Genet Dev. 48:97–103. doi:10.1016/j.gde.2017.11.004.
- Boffano P, Cavarra F, Tricarico G, Masu L, Brucoli M, Ruslin M, Forouzanfar T, Ridwan-Pramana A, Rodríguez-Santamarta T, Rui Ranz M, et al. 2021. The epidemiology and management of ameloblastomas: a European multicenter study. J Cranio-Maxillofacial Surg. 49(12):1107–1112. doi:10.1016/j.jcms.2021.09.007.
- Bonacina R, Indini A, Massazza G, Rulli E, Gianatti A, Mandalà M, Ameloblastoma Cooperative Group. 2022. Correlation of BRAF mutational status with clinical characteristics and survival outcomes of patients with ameloblastoma: the experience of 11 Italian centres. J Clin Pathol. 75(8):555–559. doi:10.1136/jclinpath-2021-207527.
- Brown NA, Rolland D, McHugh JB, Weigelin HC, Zhao L, Lim MS, Elenitoba-Johnson KSJ, Betz BL. 2014. Activating FGFR2–RAS–BRAF mutations in ameloblastoma. Clin Cancer Res. 20(21):5517–5526. doi:10.1158/1078-0432.CCR-14-1069.
- Derakhshan S, Aminishakib P, Karimi A, Aminishakib P, Karimi A, Saffar H, Abdollahi A, Mohammadpour H, Fard MJK, Memarha A. 2020. High frequency of BRAF V600E mutation in Iranian population ameloblastomas. Med Oral Patología Oral y Cirugia Bucal. 25(4):e502–e507. doi:10.4317/medoral.23519.
- Diniz MG, Gomes CC, Guimarães BV, Gomes CC, Guimarães BVA, Castro WH, Lacerda JCT, Cardoso SV, de Faria PR, Dias FL, et al. 2015. Assessment of BRAFV600E and SMOF412E mutations in epithelial odontogenic tumours. Tumor Biol. 36(7):5649–5653. doi:10.1007/s13277-015-3238-0.
- do Canto AM, da Silva Marcelino BMR, Schussel JL, Wastner BF, Sassi LM, Corrêa L, de Freitas RR, Hasséus B, Kjeller G, Junior CAL, et al. 2019. Immunohistochemical analysis of BRAF V600E mutation in ameloblastomas. Clin Oral Investig. 23(2):779–784. doi:10.1007/s00784-018-2494-y.
- Doheny D, Manore SG, Wong GL, Lo H-W. 2020. Hedgehog signaling and truncated GLI1 in cancer. Cells. 9(9):2114. doi:10.3390/cells9092114.
- Ghafouri-Fard S, Atarbashi-Moghadam S, Taheri M. 2021. Genetic factors in the pathogenesis of ameloblastoma, dentigerous cyst and odontogenic keratocyst. Gene. 771: 145369. doi:10.1016/j.gene.2020.145369.
- Gomes DC, Jamra SA, Leal LF, Colli LM, Campanini ML, Oliveira RS, Martinelli Jr CE, Elias PCL, Moreira AC, Machado HR, et al. 2015. Sonic hedgehog pathway is upregulated in adamantinomatous craniopharyngiomas. Eur J Endocrinol. 172(5):603–608. doi:10.1530/EJE-14-0934.
- Guimarães LM, Coura BP, Gomez RS, Gomes CC. 2021. The molecular pathology of odontogenic tumors: expanding the spectrum of MAPK pathway driven tumors. Frontiers Oral Health. 2:740788. doi:10.3389/froh.2021.740788.
- Gültekin SE, Aziz R, Heydt C, Sengüven B, Zöller J, Safi AF, Kreppel M, Buettner R. 2018. The landscape of genetic alterations in ameloblastomas relates to clinical features. Virchows Arch. 472(5):807–814. doi:10.1007/s00428-018-2305-5.
- Heikinheimo K, Huhtala JM, Thiel A, Kurppa KJ, Heikinheimo H, Kovac M, Kragelund C, Warfvinge G, Dawson H, Elenius K, et al. 2019. The mutational profile of unicystic ameloblastoma. J Dent Res. 98(1):54–60. doi:10.1177/0022034518798810.
- Hosoya A, Shalehin N, Takebe H, SHimo T, Irie K. 2020. Sonic hedgehog signaling and tooth development. Int J Mol Sci. 21(5):1587. doi:10.3390/ijms21051587.
- Kim TE, Jung ES, Jung CK, Bae JS, Kim SN, Kim GS, Lee HN, Kang CS, Choi YJ. 2013. DHPLC is a highly sensitive and rapid screening method to detect BRAFV600E mutation in papillary thyroid carcinoma. Exp Mol Pathol. 94(1):203–209. doi:10.1016/j.yexmp.2012.05.011.
- Kokubun K, Yamamoto K, Akashi Y, Chujo T, Nakajima K, Matsuzaka K. 2022. Genetic study of BRAF V600E and SMO L412F mutations in Japanese patients with ameloblastoma. Int J Surg Pathol. 30(4):378–384. doi:10.1177/10668969211064203.
- Kunmongkolwut S, Chaisuparat R. 2022. Analysis of BRAF V600E expression and disease-free survival in patients with ameloblastoma. Int J Oral Maxillofac Surg. 51(8):1034–1042. doi:10.1016/j.ijom.2021.12.011.
- Kurppa KJ, Catón J, Morgan PR, Ristimäki A, Ruhin B, Kellokoski J, Elenius K, Heikinheimo K. 2014. High frequency of BRAF V600E mutations in ameloblastoma. J Pathol. 232(5):492–498. doi:10.1002/path.4317.
- Lee SK, Kim YS. 2013. Current concepts and occurrence of epithelial odontogenic tumors: I. ameloblastoma and adenomatoid odontogenic tumor. Korean J Pathol. 47(3):191–202. doi:10.4132/KoreanJPathol.2013.47.3.191.
- Malatesta M, Steinhauer C, Mohammad F, Pandey DP, Squatrito M, Helin K. 2013. Histone acetyltransferase PCAF is required for hedgehog-Gli-dependent transcription and cancer cell proliferation. Cancer Res. 73(20):6323–6333. doi:10.1158/0008-5472.CAN-12-4660.
- Mota M, Shevde LA. 2020. Merlin regulates signaling events at the nexus of development and cancer. Cell Commun Signal. 18(1):63. doi:10.1186/s12964-020-00544-7.
- Oh K-Y, Cho S-D, Yoon H-J, Lee JI, Ahn S-H, Hong S-D. 2019. High prevalence of BRAF V600E mutations in Korean patients with ameloblastoma: clinicopathological significance and correlation with epithelial-mesenchymal transition. J Oral Pathol Med. 48(5):413–420. doi:10.1111/jop.12851.
- Owosho AA, Ladeji AM, Adebiyi KE, Olajide MA, Okoye ISI, Kehinde T, Nwizu NN, Summersgill KF. 2021. BRAF v600e mutation-specific immunohistochemical analysis in ameloblastomas: a 44-patient cohort study from a single institution. Eur Arch Oto-Rhino-Laryngol. 278(8):3065–3071. doi:10.1007/s00405-020-06491-w.
- Petrovic ID, Migliacci J, Ganly I, Patel S, Xu B, Ghossein R, Huryn J, Shah J. 2018. Ameloblastomas of the mandible and maxilla. Ear Nose Throat J. 97(7):233–232. doi:10.1177/014556131809700704.
- Rui Z, Li-Ying P, Jia-Fei Q, Ying-Ying H, Feng C, Tie-Jun L. 2014. Smoothened gene alterations in keratocystic odontogenic tumors. Head Face Med. 10:36. doi:10.1186/1746-160X-10-36.
- Shimura M, Nakashiro KI, Sawatani Y, Hasegawa T, Kamimura R, Izumi S, Komiyama Y, Fukumoto C, Yagisawa S, Yaguchi E, et al. 2020. Whole exome sequencing of SMO, BRAF, PTCH1 and GNAS in odontogenic diseases. In Vivo. 34(6):3233–3240. doi:10.21873/invivo.12159.
- Shirsat PM, Bansal S, Prasad P, Desai RS. 2018. Low frequency of BRAF V600E immunoexpression in mandibular ameloblastomas: an institutional study. J Oral Maxillofac Pathol. 22(3):353–359. doi:10.4103/jomfp.JOMFP_174_17.
- Siar CH, Ishak I, Ng KH. 2015. Podoplanin, E-cadherin, β-catenin, and CD44v6 in recurrent ameloblastoma: their distribution patterns and relevance. J Oral Pathol Med. 44(1):51–58. doi:10.1111/jop.12203.
- Soltani M, Tabatabaiefar MA, Mohsenifar Z, Pourreza MR, Moridnia A, Shariati L, Razavi SM. 2018. Genetic study of the BRAF gene reveals new variants and high frequency of the V600E mutation among Iranian ameloblastoma patients. J Oral Pathol Med. 47(1):86–90. doi:10.1111/jop.12610.
- Soluk-Tekkesin M, Wright JM. 2022. The world health organization classification of odontogenic lesions: a summary of the changes of the 2022 (5th) edition. Turkish J Pathol. 38(2):168–184. doi:10.5146/tjpath.2022.01573.
- Srivastava G, Padhiary SK, Nayak TK, Mishra N. 2022. Rehabilitation using implants in free fibula flap of a patient with ameloblastoma: case report with 4-year follow-up. Natl J Maxillofac Surg. 13(Suppl 1):s194–s198. doi:10.4103/njms.NJMS_239_20.
- Sweeney RT, McClary AC, Myers BR, Biscocho J, Neahring L, KAQu K, Gong K, Ng X, Jones T, D C, et al. 2014. Dentification of recurrent SMO and BRAF mutations in ameloblastomas. Nat Genet. 46(7):722–725. doi:10.1038/ng.2986.
- Togni L, Zizzi A, Mazzucchelli R, Santarelli A, Rubini C, Mascitti M. 2022. Identification of BRAF V600E mutation in odontogenic tumors by high-performance MALDI-TOF analysis. Int J Oral Sci. 14(1):22. doi:10.1038/s41368-022-00170-8.
- Wang C, Wu H, Katritch V, Han GW, Huang X-P, Liu W, Siu FY, Roth BL, Cherezov V, Stevens RC. 2013. Structure of the human smoothened receptor bound to an antitumour agent. Nature. 497(7449):338–343. doi:10.1038/nature12167.
- Yoshimoto S, Morita H, Okamura K, Hiraki A, Hashimoto S. 2022. αTAT1-induced tubulin acetylation promotes ameloblastoma migration and invasion. Lab Invest. 102(1): 80–89. doi:10.1038/s41374-021-00671-w.
- Zhang X, Wang J, Liu H, Zhang Y, Dong F. 2017. The clinicopathologic relevance of RECK gene polymorphisms in ameloblastoma. Arch Oral Biol. 79:77–86. doi:10.1016/j.archoralbio.2017.03.008.