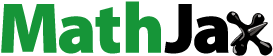
Abstract
We investigated the cytotoxic and genotoxic effects of the organic UVA filter avobenzone (AVB), and the UVB filter octocrylene (OCT), found alone or in combination in different sunscreen formulations, on normal human skin fibroblast cells (CCD-1118Sk). To achieve this purpose, we used XTT viability assay, single cell gel electrophoresis (comet) assay and reactive oxygen species (ROS) assay. For cytotoxicity, CCD-1118Sk cells were exposed to various concentrations of AVB (32–800 µM), and OCT (130–6500 μM). The IC50 values were 100.2 and 1390.95 µM for AVB and OCT, respectively. IC12.5, IC25 and IC50 concentrations were chosen for genotoxicity testing. The comet assay revealed DNA damage at the two highest concentrations of AVB and all OCT concentrations. The rate of DNA damage was significantly higher than in the control groups. Co-treatment with AVB and OCT increased the level of DNA damage and intracellular oxidative stress compared with AVB and OCT treatment alone. Finally, our study showed that the UVA filter AVB, with the UVB filter OCT, may induce cytotoxic and genotoxic effects on human skin fibroblast cells.
Highlights
Avobenzone and octocrylene are UV filters used in sunscreen products.
Healthy human skin fibroblast cell line CCD-1118Sk was used for cytotoxic and genotoxic assays.
Cotreatment with avobenzone and octocrylene caused more severe DNA damage than their treatment alone.
Our findings will contribute to the limited number of cytotoxicity and genotoxicity studies and lead to more detailed studies on the use of these two chemicals.
Introduction
In recent years, sunscreens have become the most popular means of global protection against ultraviolet radiation (UVR). The increasing use of artificial tanning devices, development of holiday habits, as well as tanning for cosmetic purposes through sunbathing, have led to an increase in daily exposure of the skin to UV light. Because UVR (UVA and UVB) levels rise with a declining ozone layer, skin cancer rates are expected to increase worldwide (Barnes et al. Citation2022).
Sunscreen precautions include the frequent use of sunscreens, especially during outdoor recreation, sports, or water activities (Gilaberte et al. Citation2022). The active ingredients in sunscreen products decrease UV rays impacting the skin through absorbing, reflecting, and scattering the radiation, while some protect the skin and avoid UV-induced oxidative damage (Nohynek and Schaefer Citation2001). As both UVA (320–400 nm) and UVB (290–320 nm) radiation contribute to sunlight-induced skin damage, combinations of UVA and UVB filters are used in the formulation of preparations to block UVA (Armeni et al. Citation2004; de la Coba et al. Citation2019). Another important parameter for the efficacy of sunscreen products is a high photostability because their light-induced degradation leads to a reduction in their blocking capacity through sun exposure and degraded products can generate reactive oxygen species in the skin (CEC Citation2006; Dondi et al. Citation2006; Herzog et al. Citation2009; Gonzalez et al. Citation2022). There are two main types of UV protectors: organic and inorganic. Octocrylene (OCT) (2-ethylhexyl-2-cyano-3, 3-diphenylacrylate), phenylbenzimidazole sulfonic acid (PBSA), homosalate, 2-ethoxyethyl p-methoxycinnamate, 2-ethylhexyl salicylate, 2-ethyl-hexyl-4-trimethoxy cinnamate (EHMC), and butyl methoxydibenzoylmethane, (BMDM – avobenzone) are widely-used organic filters in sunscreens and personal products (Brooke et al. Citation2008). Individual organic filters have a narrow absorption spectrum. Thus, they are usually combined with other UV filters for protection against the UVR spectrum (Grabicova et al. Citation2013; Abou-Dahech et al. Citation2022).
Avobenzone (AVB) is a UVA filter exhibiting maximum absorption at 360 nm long wavelengths (DeLeo et al. Citation2009). However, the protection capacity of AVB was shown to weaken by 60% to 50% in one hour after sunlight exposure. In addition to the decrease in protection capacity, the decomposition of AVB facilitates the formation of free radicals in the skin: this results in DNA strand breaks and oxidative damage in the cells (Damiani et al. Citation1999; Armeni et al. Citation2004). OCT is also commonly used as a UVB filter in sunscreens and cosmetics, and as a photostabilizing agent of AVB (Manová et al. Citation2013). There are no cyto/genotoxicity studies previously conducted with these two filters (UVA and UVB). Therefore, we aimed to determine the cytotoxic and genotoxic effects of AVB and OCT either alone or in combination on CCD-1118Sk cells.
Materials and methods
In this study, we investigated the cytotoxic and genotoxic effects of AVB and OCT included in several sunscreen formulations single or in combination, and their ability to produce intracellular reactive oxygen species (ROS) using CCD-1118Sk (ATCC CRL-2466) human healthy skin fibroblast cells. We used XTT formazan dye viability assay, comet assay and intracellular ROS assay for cytotoxicity and genotoxicity.
Chemicals
The chemicals and their sources were as follows: AVB (crystal) and OCT (dense liquid) were purchased from FLUKA (Fluka Chemie GmbH, Buchs, Switzerland). DMEM (Dulbecco’s Modified Eagle’s Medium), DPBS (Dulbecco’s phosphate buffered saline), l-glutamine, low melting point agarose, normal melting point agarose, EDTA, Triton X-100, ethidium bromide (EB), and tris-(hydroxymethyl)aminomethane-HCl were purchased from Sigma (Steinheim, Germany). Fetal calf serum was purchased from Biochrom (Berlin, Germany); penicillin–streptomycin from PAN Biotech (Aidenbach, Germany); and XTT cell proliferation assay kit from Biological Industries (Beit-Haemek, Israel).
Cell culture
Normal human skin fibroblast cell line (CCD-1118Sk) obtained from American type culture collection (ATCC – CRL2466™, Manassas, United States) was kindly provided by Dr. E. Ulukaya (University of Uludag, Turkey). Cells were maintained at 37°C and 5% CO2/ 95% air in DMEM supplemented with non-essential aminoacids (1%), 2 mM l-glutamine, penicillin–streptomycin (50 U/ml–50 µg/ml) and 10% (v/v) fetal bovine serum and cultured as adherent monolayers, routinely split 1:4 every 3–4 days and used for each experiment at the third passage.
Cytotoxicity assay (XTT)
The viability of human skin fibroblasts exposed to AVB and OCT was measured by XTT assay. The concentrations of avobenzone were 32, 65, 130, 200, 400 and 800 µM respectively. OCT also was given as the concentrations of 130, 260, 750, 1300, 1950, 2600, 3900, 5200 and 6500 µM. DMSO (maximum final concentration 1.24% (v/v)) and dH2O (maximum final concentration 1.5% (v/v)) were used as solvent controls for AVB and OCT because of AVB dissolved in DMSO and OCT diluted in dH2O. H2O2 (500 µM) was used as a positive control.
Cells were seeded in sterile 96-well flat-bottomed plates in triplicate at 5 × 103 cells/well. After 24 h incubation at 37°C with 5% CO2 and 95% relative humidity, cells were treated with AVB or OCT with the different concentrations mentioned above. After 24 h exposure, wells were washed with PBS and 100 μL of fresh culture medium was added to each well. The activated XTT solution (50 μL per well) was applied and incubated for 4 h. Absorbance was measured using a microplate reader (BioTek ELx800, Vermont, USA) at a wavelength of 450 nm, and cell proliferation curve was drawn. After blank subtraction, percent growth inhibition of cells was calculated as follows, and the cell proliferation curve was drawn using the percent cell viability (Gi-100).
Then, IC50 values were calculated using the proliferation curve formed from the values obtained from the experiment repetitions. The IC50 values were determined by the X axis values obtained by giving 50 to the Y axis value in the function formula of the linear graphs determined by choosing at least four points with linear regression in the proliferation curve.
Comet assay
CCD-1118Sk cells were seeded in T25 tissue culture flasks at a density of 54 × 103 cells/flask and allowed to grow for 48 h. Cells were washed with DPBS. Cells were exposed to IC12.5, IC25 and IC50 values of AVB and OCT determined by XTT assay, for 24 h. Furthermore, because AVB and OCT are present together in the UV filter formulations, we also used two different combined concentrations of AVB and OCT considering their lower cytotoxic concentrations from XTT assay (IC12.5 and IC25). For the comet assay we used DMSO (maximum final concentration 1.24% (v/v)) and dH2O (maximum final concentration 1.5% (v/v)) as solvent control groups. H2O2 (150 µM) was used as a positive control.
After treatments, the alkaline comet assay was performed according to previous studies (Singh et al. Citation1988), with some modifications (Costa et al. Citation2008). CCD-1118Sk cells were harvested following incubation with 0.25% trypsin-EDTA solution for 5–8 min, collected with complete growth medium, and after being twice washed with PBS cells, embedded in 0.8% low melting agarose on slides pre-coated with normal melting point agarose. Slides were then placed in precooled lysis solution (2.5 M NaCl, 0.1 M EDTA, 10 mM Tris base, pH 10) with 1% Triton X for 1 h at 4°C. DNA from cells was then denatured in alkaline buffer (0.3 M NaOH, 1 mM EDTA) for 30 min in the dark at room temperature. Electrophoresis was performed at 25 V and 300 mA for 20 min. The slides were immersed in neutralization buffer (0.5 M Tris-HCl, pH 7.5) for 10 min followed by dehydration in 70% ethanol. The slides were air dried and stained with 100 ml ethidium bromide (20 mg/ml). Slides and comets were examined under Nikon epifluorescence microscope (Eclipse I-80) equipped with a digital camera (Kameram 21™, İstanbul, Türkiye) using an image processing software (ARGENIT Comet Assay Software™, İstanbul, Türkiye). The comet parameters used to measure DNA damage were tail length (μm), % tail DNA and Olive tail moment (OTM).
Intracellular ROS assay
In this study, we used 2′ 7′- dichlorodihydrofluorescein diacetate (DCFH-DA) to determine intracellular ROS levels. DCFH-DA is not fluorescent by itself diffused into cells, and is hydrolyzed to 2′ 7′- dichlorodihydrofluorescein (DCFH) by cellular esterase. DCFH is oxidized to the highly fluorescent 2′ 7′- dichlorodihydrofluorescein (DCF) by intracellular ROS. Cells were seeded in sterile 96-well microplates for fluorescence-based assays in triplicate at 5 × 103 cells/well and 24 h incubation at 37°C with 5% CO2 and 95% relative humidity. Then cells pre-incubated with DCFH-DA for 2 h. Cells were washed with DPBS. DCFH-DA loaded cells were treated with AVB (IC12.5, IC25 and IC50 concentrations), OCT (IC12.5, IC25 and IC50 concentrations) and combined AVB + OCT (IC12.5 + IC12.5, IC25 + IC25) for 12 h. DMSO, dH2O were used as solvent control and H2O2 as positive control. The DCF fluorescence intensity of each well was measured with a fluorescence plate reader (Thermoscientific Fluoroskan Ascent FL) in 480 nm (Excitation)/ 530 nm (Emission). The ROS contents were determined using relative fluorescent units (RFU).
Statistical analysis
The data are represented as means ± SE. Statistical analyses were performed using SPSS Statistics for Windows 22.0 software (IBM Corp. Armonk, NY., USA). Statistical significance was determined by the Kolmogorov–Smirnov test and the Mann–Whitney U test. Significance was ascribed at P < 0.05.
Results
Cell viability
The cytotoxic effects of AVB and OCT was evaluated by performing the XTT assay. The IC50 value was 100.20 µM (R2 = 0.9521) for AVB and 1390.95 µM (R2 = 0.9986) for OCT: the IC12.5, IC25 and IC50 concentrations were used for the comet and intracellular ROS assay. IC12.5 and IC25 values were 25.05 and 50.10 µM for AVB and 347.74 and 695.48 µM for OCT, respectively. Data show the rate of cell viability decreasing significantly (P < 0.001) with higher concentrations of two UV filters, vs. the control group (Figures and ).
Damaging effects of AVB and OCT on DNA
We used comet assay to determine the damaging effects on DNA from AVB, OCT, and combined treatments; we also measured tail length, % tail DNA, and OTM parameters.
Tail length, the percent of tail DNA and OTM values significantly increased at IC25 and IC50 doses of AVB compared to the DMSO control (P < 0.01). But all OCT concentrations (IC12.5, IC25 and IC50; P < 0.01) had significantly elevated comet tail parameters compared to solvent controls (dH2O).
Moreover, tail length values increased significantly with two UV filter combinations for IC12.5 + IC12.5 concentrations, compared to single AVB treatment at the same concentration (P < 0.01), but not with single OCT treatment. Tail lengths were significantly elevated in AVB + OCT (IC25 concentrations) combinations, compared to AVB only (IC25) and OCT only (IC25) (P < 0.01) (Table and Figure ).
Figure 3. Undamaged and damaged DNA observed by Comet assay (x 200). a: growth control b: solvent control (DMSO) c: solvent control (dH2O) d: positive control (H2O2) e: IC25 (A) f: IC25 (O) g: IC25 (A)+IC25 (O).
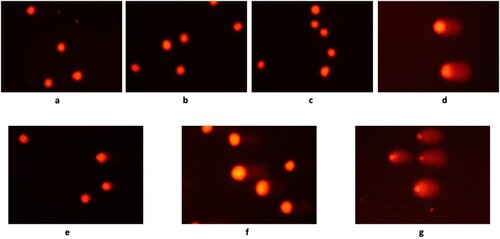
Table 1. Comet values in DNA damage assay.
The percent of tail DNA and OTM data (Table and Figure ) was significantly elevated in the IC50 concentration of AVB, compared to the DMSO control (P < 0.01). As such, the % tail DNA and OTM significantly increased in all OCT concentrations (IC12.5, IC25, IC50; P < 0.01) compared to the dH2O control group. Significant elevations were observed in the % tail DNA and OTM values for AVB + OCT combinations (IC12.5 + IC12.5 and IC25 + IC25) compared to IC12.5 and IC25 concentrations of AVB only, as well as IC12.5 and IC25 concentrations of OCT only (P < 0.01).
Intracellular ROS
The oxidative stress inducing potentials of AVB, OCT and their combinations were assessed by measuring the intracellular ROS levels in human skin fibroblast cells.
AVB at IC25 and IC50 concentrations elevated the ROS level compared to the DMSO control (P < 0.01). Three OCT concentrations (IC12,5; IC25 and IC50) significantly increased the intracellular ROS level compared to the solvent control (dH2O; P < 0.01). AVB-IC12.5 + OCT-IC12.5 and AVB-IC25 + OCT-IC25 concentrations combinations affected the ROS level compared to each of the AVB-IC12.5, IC25 and OCT-IC12.5, and IC25 single concentrations (P < 0.01) (Figure ).
Figure 4. Intracellular ROS values. GC; untreated control, SC; solvent control, PC; positive control (100 µM H2O2), A; avobenzone, O; octocrylene, RFU; relative fluorescence unit, **P < 0.01 compared with SC (DMSO for A, dH2O for O) ##P < 0.01 compared with IC12.5 A and O. ++P < 0.01 compared with IC25 A and O.
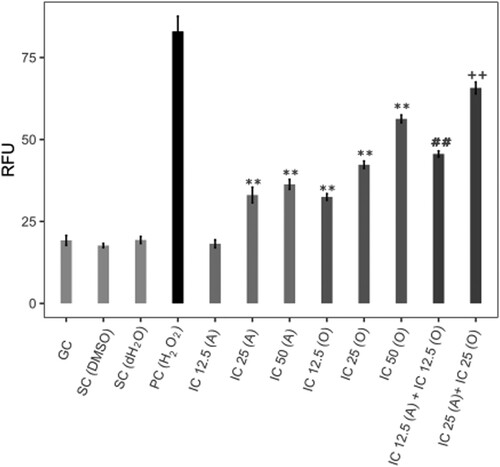
Discussion
UVR from sunlight affects all skin to a certain extent, but the skin’s response varies from person to person (D’Orazio et al. Citation2013). The harmful effects of UV light depend on the duration and frequency of UV exposure, skin color, and phototype (Al-Jamal et al. Citation2014). In addition to the direct effect of UVR on cellular DNA, the formation of photoaging in the skin, the indirect effect of free radicals and ROS, caused by UVA and UVB, are also responsible (Markiewicz and Idowu Citation2019). In this way, both natural and chemical filters are widely used as sunscreen. Organic UV filters such as AVB and OCT used to prefer in sunscreen formulas are known for absorbing UVA and UVB radiation (Jesus et al. Citation2022). The combination of UV filters elicits synergistic interactions for both protection and stabilization (Nunes et al. Citation2018). Prolonged sunscreen use on the skin, along with its high chemical content, may cause cytotoxic and genotoxic effects (Ruszkiewicz et al. Citation2017). The main goal of our study is to examine cytotoxic effects and abilities to cause DNA damage of sunscreen filters AVB and OCT in the CCD-1118Sk human healthy skin fibroblast cell line. Thus, the IC50 concentration and that below the two concentrations were selected for each chemical, both used alone and in combination with each other. When the UVA filter AVB and the UVB filter OCT are applied together, they significantly increased the formation of cytotoxic, genotoxic, and oxidative damage in human skin fibroblast cells, compared to those created alone.
AVB produce cytotoxic effects in collagen-secreting fibroblast cells (human skin fibroblast cell line) in the dermis layer of the skin and we found the IC50 concentration of AVB to be 100.2 µM. Although the other filter OCT is a UVB filter, it is always used as a photostability enhancing agent of AVB in sunscreen formulations. Without OCT, AVB is degraded by 50% upon 1 h of UV exposure, rendering the sunscreen less effective (Mori and Wang Citation2021). As a result of the XTT viability test performed with OCT, the IC50 concentration was determined to be 1390.95 µM. According to this result, OCT produced low cytotoxicity in CCD-1118Sk skin fibroblast cells.
Alkaline comet is a well-known genotoxicity testing method to determine single and double- strand DNA breaks as a result of chemical and physical exposure (Tice et al. Citation2000; Cordelli et al. Citation2021). We determined that AVB significantly increased tail length, % tail DNA, and OTM percentages in the alkaline comet test, as compared to the solvent control in IC25 and IC50 concentrations. Also all concentrations of OCT increased DNA damage significantly in tail length, % tail DNA, and the OTM ratio compared to control. When AVB and OCT are used in combination for comet assay they both exhibited remarkable DNA damage. Although fibroblast cells are located in the dermis layer, it is suspected that AVB is absorbed in the lower layers of the skin after topical application (Scalia et al. Citation2011; Ruskiewicz et al. Citation2017). It is claimed that oil soluble UV filters, such as AVB, are better absorbed by upper layers of the epidermis after being applied, but their potential can also reach the lower layers of the skin due to concentration levels (Lazar et al. Citation1996; Egambaram et al. Citation2020). OCT has an oily viscous structure and can penetrate the lower layers of the epidermis (Tampucci et al. Citation2018).
Our findings with the comet test seem compatible with how AVB may cause oxidative damage, due to its transformation into a triplet radical form on contact with UVA radiation, because we also found that AVB induced high levels of intracellular ROS in human skin fibroblasts. Photodegradation products, benzyl and aryl glyoxal, are formed after AVB is exposed to UV light, as these products have shown cytotoxic and skin sensitizing activity (Manová et al. Citation2013). Indeed, possible genotoxic effects of AVB such as plasmid DNA breaks and oxidative damage on albumin have been shown (Paris et al. Citation2009).
In our study, three concentrations of OCT increased the ROS level against the control group in human skin fibroblasts. We suggest that the increase of genotoxic damage by OCT can be caused by ROS formation in epidermal cells. UV filters may induce genotoxicity without direct contact with DNA and may exhibit an indirect effect on DNA through their ability to produce intracellular ROS (Ruszkiewicz et al. Citation2017). One significant aspect of the study is that when AVB and OCT are used in combination for comet and ROS assays, the rate of DNA damage and intracellular ROS levels were higher compared to the ones induced alone. The IC50 + IC50 combination group were not included in these tests, since it would be too cytotoxic. Greater damage ratios and ROS levels were observed in the single OCT group, while single AVB creates less DNA damage and ROS, vs. OCT and combination groups. Thus, we suggest that OCT shows a synergistic effect with AVB in combination applications, causing an excessive increase in genotoxic and oxidative damage.
The concentration of AVB varies between 1.5% and 2% in sunscreen formulations. The IC50 concentration we determined, 100 µM, can be converted into 31 µg/ml. This is close to the 2% AVB concentration (40 µg) in 2 mg/cm 2 cream formulations, recommended for each topical application. In this case, we assume that AVB is cytotoxic in human skin cells and that the safe use of UVA filter in sunscreen creams must be reassessed.
It is recommended that OCT be used at 10% in sunscreen creams and lotions. In this study, the IC50 value of OCT, which we found as 1390.95 µM, can be expressed as 534 µg/ml. When we consider that 5 g of OCT is in 50 g cream, this IC50 concentration does not seem cytotoxic. In our study, however, OCT elevated DNA damage ratios and ROS levels at concentration of 534 µg/ml as well as below in the comet test. Thus, although OCT does not appear to be cytotoxic, it should be approached cautiously, given its DNA damage even at low concentrations.
Consequently, OCT has a low cytotoxic effect, but was found to increase the genotoxic effect and oxidative damage if used with AVB, with its synergistic effect. The other compound, AVB, showed cytotoxic, genotoxic, and increased ROS effects in human skin fibroblast cells. Care should be taken with sunscreen creams that use both compounds. In general, although it is difficult for consumers to understand detailed product content lists, we think that consumers should generally realize which UV filter is available and read the product content lists in detail in order to make informed decisions (Manová et al. Citation2013). Further, it would be appropriate to conduct cytotoxicity and genotoxicity studies on in vivo animal models with two UV filters, along with mechanism determination studies to illuminate these effects.
Authors contribution statement
Ö.V., designed the studies, performed the statistical and lab analysis, analyzed results, wrote and revised the manuscript. D.S. performed lab analysis and a literature review. D.Y., performed lab analysis. A.C.A., analyzed, interpreted results and reviewed the manuscript. Ö.T., ensured the continuity of cell culture, performed XTT assay. N.O., ensured the continuity of cell culture, performed ROS assay. H.H., ensured the continuity of cell culture, tracked the materials, performed lab analysis. T.Ç., analyzed results and prepared the manuscript. N.Ç., designed and supervised the studies, wrote and reviewed the manuscript. All authors agree to the work done and for its publication.
Acknowledgements
This manuscript was edited by American Manuscript Editors for proper English language, grammar, spelling, and style.
Data availability statement
The data that support the findings of this study are available in Mendeley data, V4 at www.doi.org/10.17632/224cwbbkk8.4.
Disclosure statement
No potential conflict of interest was reported by the author(s).
Additional information
Funding
References
- Abou-Dahech M, Boddu SH, Bachu RD, Babu RJ, Shahwan M, Al-Tabakha MM, Tiwari AK. 2022. A mini-review on limitations associated with UV filters. Arab J Chem. 15:104212. ISSN 1878-5352. doi:10.1016/j.arabjc.2022.104212.
- Al-Jamal MS, Griffith JL, Lim HW. 2014. Photoprotection in ethnic skin. Dermatologica Sin. 32(4):217–224. doi:10.1016/j.dsi.2014.09.001.
- Armeni T, Damiani E, Battino M, Greci L, Principato G. 2004. Lack of in vitro protection by a common sunscreen ingredient on UVA-induced cytotoxicity in keratinocytes. Toxicology. 203(1-3):165–178. doi:10.1016/j.tox.2004.06.008.
- Barnes PW, Robson TM, Neale PJ, Williamson CE, Zepp RG, Madronich S, Wilson SR, Andrady AL, Heikkilä AM, Bernhard GH, et al. 2022. Environmental effects of stratospheric ozone depletion, UV radiation, and interactions with climate change: UNEP environmental effects assessment panel, update 2021. Photochem Photobiol Sci. 21(3):275–301. doi:10.1007/s43630-022-00176-5.
- Brooke DN, Burns JS, Crookes MJ. 2008. UV-filters in cosmetics – prioritisation for environmental assessment. SCHO1008BPAY-E-P. Bristol: Environment Agency, Rio House, Waterside Drive, Aztec West, Almondsbury.
- Commission of the European Communities (CEC). 2006. Commission recommendation of 22 September 2006 on the efficacy of sunscreen products and the claims made relating thereto. Off J Eur Union. 39–43. [accessed 2006 Sept 22]. https://eur-lex.europa.eu/legalcontent/IT/TXT/PDF/?uri=CELEX:32006H0647&from=EN.
- Cordelli E, Bignami M, Pacchierotti F. 2021. Comet assay: a versatile but complex tool in genotoxicity testing. Toxicol Res. 10(1):68–78. doi:10.1093/toxres/tfaa093.
- Costa S, Coelho P, Costa C, Silva S, Mayan O, Santos LS, Gaspar J, Teixeira JP. 2008. Genotoxic damage in pathology anatomy laboratory workers exposed to formaldehyde. Toxicology. 252(1-3):40–48. doi:10.1016/j.tox.2008.07.056.
- Damiani E, Greci L, Parsons R, Knowland J. 1999. Nitroxide radicals protect DNA from damage when illuminated in vitro in the presence of dibenzoylmethane and a common sunscreen ingredient. Free Radic Biol Med. 26(7-8):809–816. doi:10.1016/S0891-5849(98)00292-5.
- De la Coba F, Aguilera J, Korbee N, de Gálvez MV, Herrera-Ceballos E, Álvarez-Gómez F, Figueroa FL. 2019. UVA and UVB photoprotective capabilities of topical formulations containing mycosporine-like amino acids (MAAs) through different biological effective protection factors (BEPFs). Mar Drugs. 17(1):55. doi:10.3390/md17010055.
- DeLeo VA, Clark S, Fowler J, Poncet M, Loesche C, Soto P. 2009. A new ecamsule-containing SPF 40 sunscreen cream for the prevention of polymorphous light eruption: a double-blind, randomized, controlled study in maximized outdoor conditions. Cutis. 83(2):95–103.
- Dondi D, Albini A, Serpone N. 2006. Interactions between different solar UVB/UVA filters contained in commercial suncreams and consequent loss of UV protection. Photochem Photobiol Sci. 5(9):835–843. doi:10.1039/b606768a.
- D’Orazio J, Jarrett S, Amaro-Ortiz A, Scott T. 2013. UV radiation and the skin. Int J Mol Sci. 14(6):12222–12248. doi:10.3390/ijms140612222.
- Egambaram OP, Kesavan Pillai S, Ray SS. 2020. Materials science challenges in skin UV protection: a review. Photochem Photobiol. 96:779–797. doi:10.1111/php.13208.
- Gilaberte Y, Trullàs C, Granger C, de Troya-Martín M. 2022. Photoprotection in outdoor sports: a review of the literature and recommendations to reduce risk among athletes. Dermatol Ther (Heidelb). 12(2):329–343. doi:10.1007/s13555-021-00671-0.
- González S, Aguilera J, Berman B, Calzavara-Pinton P, Gilaberte Y, Goh CL, Lim HW, Schalka S, Stengel F, Wolf P, Xiang F. 2022. Expert recommendations on the evaluation of sunscreen efficacy and the beneficial role of Non-filtering ingredients. Front Med (Lausanne). 9:790207. doi:10.3389/fmed.2022.790207.
- Grabicova K, Fedorova G, Burkina V, Steinbach C, Schmidt-Posthaus H, Zlabek V, Kroupova HK, Grabic R, Randak T. 2013. Presence of UV filters in surface water and the effects of phenylbenzimidazole sulfonic acid on rainbow trout (Oncorhynchus mykiss) following a chronic toxicity test. Ecotoxicol Environ Saf. 96:41–47. doi:10.1016/j.ecoenv.2013.06.022.
- Herzog B, Wehrle M, Quass K. 2009. Photostability of UV absorber systems in sunscreens. Photochem Photobiol. 85(4):869–878. doi:10.1111/j.1751-1097.2009.00544.x.
- Jesus A, Sousa E, Cruz MT, Cidade H, Lobo JMS, Almeida IF. 2022. Uv filters: challenges and prospects. Pharmaceuticals. 15(3):263. doi:10.3390/ph15030263.
- Lazar GM, Baillet A, Fructus AE. 1996. Evaluation of in vitro percutaneous absorption of UV filters used in sunscreen formulations. Drug Cosmet Ind. 158:50–62.
- Manová E, Goetz NV, Hauri U, Bogdal C, Hungerbühler K. 2013. Organic UV filters in personal care products in Switzerland: a survey of occurrence and concentrations. Int J Hyg Environ Health. 216(4):508–514. doi:10.1016/j.ijheh.2012.08.003.
- Markiewicz E, Idowu OC. 2019. DNA damage in human skin and the capacities of natural compounds to modulate the bystander signalling. Open Biol. 9(12):190208. doi:10.1098/rsob.190208.
- Mori S, Wang SQ. 2021. Sunscreens. In: Wolverton SE, editor. Comprehensive dermatologic drug therapy. 4th ed. Elsevier; p. 565–575.e2, ISBN 9780323612111. doi:10.1016/B978-0-323-61211-1.00050-4.
- Nohynek GJ, Schaefer H. 2001. Benefit and risk of organic ultraviolet filters. Regul Toxicol Pharmacol. 33(3):285–299. doi:10.1006/rtph.2001.1476.
- Nunes AR, Vieira ÍGP, Queiroz DB, Leal ALAB, Morais SM, Muniz DF, Calixto-Junior JT, Coutinho HDM. 2018. Use of flavonoids and cinnamates, the main photoprotectors with natural origin. Adv Pharmacol Sci. 28:5341487. doi:10.1155/2018/5341487.
- Paris C, Lhiaubet-Vallet V, Jiménez O, Trullas C, Miranda MÁ. 2009. A blocked diketo form of avobenzone: photostability, photosensitizing properties and triplet quenching by a triazine-derived UVB-filter. Photochem Photobiol. 85(1):178–184. doi:10.1111/j.1751-1097.2008.00414.x.
- Ruszkiewicz JA, Pinkas A, Ferrer B, Peres TV, Tsatsakis A, Aschner M. 2017. Neurotoxic effect of active ingredients in sunscreen products, a contemporary review. Toxicol Rep. 4:245–259. doi:10.1016/j.toxrep.2017.05.006.
- Scalia S, Coppi G, Iannuccelli V. 2011. Microencapsulation of a cyclodextrin complex of the UV filter, butyl methoxydibenzoylmethane: In vivo skin penetration studies. J Pharm Biomed Anal. 54(2):345–350. doi:10.1016/j.jpba.2010.09.018.
- Singh NP, McCoy MT, Tice RR, Schneider EL. 1988. A simple technique for quantitation of low levels of DNA damage in individual cells. Exp Cell Res. 175(1):184–191. doi:10.1016/0014-4827(88)90265-0.
- Tampucci S, Burgalassi S, Chetoni P, Monti D. 2018. Cutaneous permeation and penetration of sunscreens: formulation strategies and In vitro methods. Cosmetics. 5(1):1. MDPI AG. doi:10.3390/cosmetics5010001.
- Tice RR, Agurell E, Anderson D, Burlinson B, Hartmann A, Kobayashi H, Miyamae Y, Rojas E, Ryu JC, Sasaki YF. 2000. Single cell gel/comet assay: guidelines for in vitro and in vivo genetic toxicology testing. Environ Mol Mutagen. 35(3):206–221. doi:10.1002/(SICI)1098-2280(2000)35:3<206::AID-EM8>3.0.CO;2-J.